Integrating immunotherapy into chemoradiation regimens for medically inoperable locally advanced non-small cell lung cancer
Introduction
For patients with inoperable stage II–III non-small cell lung cancer (NSCLC), the backbone of curative intent therapy is concurrent chemoradiotherapy (CRT). The supportive evidence for the use of combined CRT is rooted in several decades of methodical clinical studies that established the superiority of concurrent CRT compared to either modality alone or sequential delivery of chemotherapy followed by radiotherapy (RT) (1-5). The customary platform for localized and inoperable NSCLC consists of concurrent chemoradiation with a platinum-based doublet and 60 Gy of RT delivered daily over 6 weeks followed by consideration of two cycles of consolidative chemotherapy, particularly for carboplatin and paclitaxel regimens (6,7). Although consolidative chemotherapy was not found to demonstrate an obvious survival benefit for inoperable, locally advanced NSCLC (8,9), its incorporation into RTOG 0617 has led to its acceptance as the de facto standard of care (7). Despite its acceptance as a curative intent treatment, concurrent CRT results in relatively meager treatment outcomes with median survival rates of 20–28 months and 5-year overall survival (OS) rates of 15–20%.
The advent of novel immunotherapy agents affords patients and clinicians therapeutic modalities to improve patient longevity and avenues to study innovative combinations of therapies (10-13). Incorporation of immunotherapy with standard therapy provides the potential to build upon the gains of the well-established regimen of CRT for inoperable NSCLC. Since checkpoint inhibitors have shown clinical benefit in the setting of metastatic NSCLC, additional study will be necessary to understand their role in combined modality CRT. When integrating immunotherapy with RT for cure, clinicians will need to consider synergy, timing, doses, and safety among the combined therapies. This article seeks to review data evaluating interactions, temporal sequencing, fractionation, and overlapping toxicity profiles of CRT and immunotherapy.
Immune modulation in the setting of RT
Local ionizing radiation can interact with the host’s immune system by increasing the tumor antigen specific effector cells that traffic to a tumor. In a study comparing xenografts with B16-F0 tumors, irradiated mice (treated with 15 Gy) had greater ability to present tumor antigens and specific T-cells and tumor infiltrating lymphocytes than non-irradiated mice (14). In melanoma murine models, tumor control increased with the size of the RT dose as did tumor-reactive T cells, but a dose of 7.5 Gy per fraction proved to be the regimen with the optimal tumor control and tumor immunity with the lowest number of T-regulatory cells (T-regs) (15).
RT modulates the immune system and can help to mount an immune response that can result in immunogenic cell death. Radiation releases tumor antigens and facilitates tumor antigen release by dendritic cells (DC) and cross-complementation on major histocompatibility complex-1 (MHC-1) (16). RT potentiates calretinin’s exposure on the cell surface and release of ATP and high mobility group box 1 (HMGB1), which seems to be required for DC activation and immune priming against malignant cells (17). Therefore, RT also acts as an in-situ tumor vaccine and may immunize the patient against their neoplasm and can provide immunologic memory which may endure for the host’s lifetime (18,19).
RT also provides a pro-immunogenic effect on the tumor microenvironment (18). RT elicits activation of both innate and adaptive immunity (20), and these immune responses are potentiated by the cellular damage caused by RT and the cascade of interleukin-1β (IL-1β), tumor necrosis-factor-α (TNF-α), and chemokine (C-C-C motif) ligand 16 (CXCL16), MHC molecules, adhesion molecules and death receptors (21). RT also can reprogram macrophage differentiation to an iNOS+/M1 phenotype that orchestrates effective T cell immunotherapy (18,22).
The interaction of RT and checkpoint inhibitors or other immunotherapies may lead to an abscopal effect whereby after the administration of RT to one location, a non-responding systemic tumor then displays diffuse systemic response at distant sites from the site of irradiation. This concept has gained much attention in the setting of metastatic melanoma patient who had been maintained on ipilimumab with relatively stable disease subsequently received stereotactic body RT to an enlarging paraspinal mass and was found to have response at their other sites of distant metastases. The authors suggested that the tumor was resistant to T cell mediated antitumor effects until the delivery of RT (23). T cells are thought to be a driver for the abscopal effect, which may require Flt-3 ligand as mice bearing tumor in both flanks responded in both flanks despite irradiation of only one flank when Flt-3 was available (24). This abscopal effect has been demonstrated on multiple occasions but is thought to be relatively infrequent, and no reliable method has been discovered to reproducibly harness these potent series of events clinically.
PD-1 and its interaction with RT
Programmed cell death-1 is an immune checkpoint inhibitory receptor and facilitates immune escape (25). PD-1 primarily curbs the activity of T cells in the periphery during chronic inflammation, infection or cancer and limits autoimmunity. When PD-1 interacts with its ligand PD-L1, it can inhibit T cell growth, survival, and effector function, such as cytokine release and cytotoxicity (26), and leads to tumor specific T cell apoptosis (27), stimulates the differentiation of CD4+ T cells into T-regs (28) and allows for the resistance of tumor cells to cytotoxic T cell (CTL) attack (29).
Inhibition of PD-1, and likely PD-L1, improves tumor rejection. Polyclonal antibody against PD-L1 can promote tumor rejection in models (29). Since PD-1 is expressed directly on tumor surfaces, this is an attractive target for immune-mediated responses. PD-1 blockade can allow for tumor rejection and immune-mediated signaling to allow the immune system to attack the tumor. PD-1 expression is generally increased in tumors with a higher non-synonymous mutational burden in tumors and is associated with improved responses and durable clinical benefit with longer progression-free survival in NSCLC (30). Given the high mutational burden that is often seen in smokers who develop NSCLC, PD-1/PD-L1 inhibition appears to be a logical combination.
Blockade of PD-L1 improves T cell responses leading to tumor rejection (31). PD-L1 can be upregulated in the tumor microenvironment after RT in murine models. The addition of anti-PD-L1 therapy can improve the efficacy of RT through a CTL-dependent mechanism. This combination also reduced tumor-infiltrating myeloid-derived suppressor cells that contribute to altering the tumor microenvironment (32).
Importantly, the interaction of stereotactic RT can augment antigen-specific PD-1 mediated antitumor responses by inducing a more robust immune response and cross-presentation of tumor antigen, which was studied in melanoma and breast cancer models (33). In those models, RT resulted in the development of antigen-specific T cell and B cell-mediated immune responses. These immune stimulating effects of RT were increased when RT was combined with anti-PD-1 therapy or regulatory T cell depletion and resulted in improved local control of the tumor.
As discussed in other articles in this series, anti-PD-1 and anti-PD-L1 therapies have shown clinical activity for NSCLC alone and in combination with chemotherapy. Since the clinical effect of anti-PD-1/anti-PD-L1 therapies is evident without the incorporation of RT, it is plausible that the incorporation of RT may provide combinatorial, abscopal or synergistic effects.
Timing, dose, fractionation of immunotherapy with chemoradiation
To date, clinicians have related many of the abscopal responses to hypofractionated irradiation regimens, often with stereotactic body radiotherapy (SBRT), also termed stereotactic ablative radiotherapy (SABR). Dewan et al. evaluated three RT fractionation schemes: 20 Gy × 1, 8 Gy × 3 or 6 Gy × 5 with or without CTLA blockade. CTLA blockade alone was ineffective, but when combined with any of the RT regimens, growth delay was seen. Abscopal effects were evident with the combination of the fractionated RT designs (34). Clinically, abscopal effects have been seen with 8 Gy × 3, 6 Gy × 5, and 9.5 Gy × 3 fractions (23,35,36). The greatest difference occurred for patients with 8 Gy × 3, and 80% of tumors outside the field regressed (16). Lower RT doses may cause reprogramming of macrophages toward an iNOS+/M1 phenotype, enabling them the ability to allow tumor rejection (37).
Also, concurrent platinum and RT cause calretinin translocation from dying tumor cells at dosages tested in a dose-dependent manner. Calretinin translocation increased due to platinum but remained stable after adding RT. Nevertheless, platinum and RT cause release of HMGB1 from dying tumor cells. When RT was combined with paclitaxel, adding RT caused immunogenic cell death (18).
A study by Gulley et al., demonstrated the possible efficacy of standard radiation fractionation of 1.8–2 Gy per day in combination with a poxviral vaccine. T cell responses were seen in the tumor antigens and not in the vaccine, suggesting irradiation promoted the activation of T cells (16,38). Therefore, the combination of standard fractionation CRT and immunotherapy may be effective when they are used together.
Ongoing trials will help to elucidate the role and timing of PD-L1 or PD-1 blockade for inoperable NSCLC treated with definitive chemoradiation (Table 1).
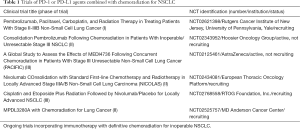
Full table
Additional immunotherapy combinations
For locally advanced NSCLC, other agents have been investigated, including tecemotide (L-BLP25), a mucin 1 (MUC1) specific agent that induced T cell responses to MUC1. The phase III START trial was a double-blind phase III trial that randomly assigned 1,006 subjects to tecemotide and 507 to placebo. Median overall survival (OS) was 25.6 months with tecemotide vs. 22.3 months with placebo (HR 0.88, 95% CI: 0.75–1.03, P=0.123). In patients who received prior concurrent CRT, median OS for those who received tecemotide was 30.8 months compared to 20.6 months for the control group (HR 0.78, P=0.016), whereas patients who received sequential CRT did not benefit in terms of OS (39,40). In the group of patients who received prior CRT, high soluble MUC1 and antinuclear antibodies correlated with tecemotide benefit (41). However, in a subsequent study by Katakami et al., which randomized Japanese patients (n=172) with stable or clinical responses after CRT to receive adjuvant tecemotide vs. placebo, no apparent trend toward increased OS or other secondary endopoint with tecemotide was observed (42).
Additionally, study of GV1001, a telomerase peptide vaccine, was administered after CRT in a phase I/II trial of 23 patients. A GV1001-specific immune response developed in 16/20 evaluable patients and long-term immunomonitoring showed persisting responses in 13 patients. Immune responders demonstrated a median progression-free survival of 19 months compared to 3.5 months for nonresponders (P<0.001). Responders all harbored durable GV1001-specific T-cell memory responses with high IFNγ, and low IL-4 and IL-10 levels (43,44).
Toxicities of immunotherapy overlap with RT side effects
Administration of thoracic RT places patients at higher risk of radiation-induced pneumonitis, and the clinical presentation is similar to immunotherapy-induced pneumonitis with dry cough, fever, dyspnea, and tachycardia. Urgent initiation of steroid therapy is often required. A study evaluating 915 patients who were treated with PD-1/PD-L1 antibody demonstrated that 43 patients developed pneumonitis (about 5% of patients). Pneumonitis was more likely to occur when anti-PD-1/anti-PD-L1 and another simultaneous immunotherapy were administered, such as concurrent CTLA-4 therapies. Pneumonitis is a toxicity of variable onset clinically, and in the aforementioned study, it ranged from 9 days to 19.2 months (45). Concern for a pneumonitis requires urgent evaluation with imaging and often rapid initiation of steroids to avoid severe and potentially life-threatening respiratory compromise.
Another complication that can occur with both RT and immunotherapy is myocarditis. Unlike pericarditis that can occur in the acute or subacute setting, radiotherapeutic injury to the myocardium is thought to be a delayed effect with long-term toxicities such as coronary artery disease and valvular injury. In contrast, fulminant cases of myocarditis from immunotherapy have been described, particularly with the combination of nivolumab and ipilimumab. The incidence of fatal myocarditis with nivolumab alone is <0.01% and with dual nivolumab and ipilimumab is 0.17%. The incidence of any myocarditis with single-agent nivolumab is 0.06% compared to 0.27% with dual agent therapy. In post-mortem examination of the cardiac tissue of immune checkpoint mediated myocarditis, increased expression of PD-L1 was found in the injured myocardium of patients, consistent with the upregulation of myocardial PD-L1 studies in mice. Investigators have hypothesized that PD-L1 upregulation in the myocardium is a cytokine-induced cardioprotective mechanism that is abrogated by immune checkpoint blockade (46).
Therefore, combinatorial therapy of CRT and immunotherapy must be approached with caution and careful clinical evaluation in prospective clinical trials.
Acknowledgements
None.
Footnote
Conflicts of Interest: Dr. Jabbour has research funding from Merck Sharp & Dohme. The other authors have no conflicts of interest to declare.
References
- Dillman RO, Herndon J, Seagren SL, et al. Improved survival in stage III non-small-cell lung cancer: seven-year follow-up of cancer and leukemia group B (CALGB) 8433 trial. J Natl Cancer Inst 1996;88:1210-5. [Crossref] [PubMed]
- Sause WT, Scott C, Taylor S, et al. Radiation Therapy Oncology Group (RTOG) 88-08 and Eastern Cooperative Oncology Group (ECOG) 4588: preliminary results of a phase III trial in regionally advanced, unresectable non-small-cell lung cancer. J Natl Cancer Inst 1995;87:198-205. [Crossref] [PubMed]
- Le Chevalier T, Arriagada R, Quoix E, et al. Radiotherapy alone versus combined chemotherapy and radiotherapy in unresectable non-small cell lung carcinoma. Lung cancer 1994;10 Suppl 1:S239-44. [Crossref] [PubMed]
- Schaake-Koning C, van den Bogaert W, Dalesio O, et al. Effects of concomitant cisplatin and radiotherapy on inoperable non-small-cell lung cancer. N Engl J Med 1992;326:524-30. [Crossref] [PubMed]
- Jeremic B, Shibamoto Y, Acimovic L, et al. Hyperfractionated radiation therapy with or without concurrent low-dose daily carboplatin/etoposide for stage III non-small-cell lung cancer: a randomized study. J Clin Oncol 1996;14:1065-70. [Crossref] [PubMed]
- Curran WJ Jr, Paulus R, Langer CJ, et al. Sequential vs. concurrent chemoradiation for stage III non-small cell lung cancer: randomized phase III trial RTOG 9410. J Natl Cancer Inst 2011;103:1452-60. [Crossref] [PubMed]
- Bradley JD, Paulus R, Komaki R, et al. Standard-dose versus high-dose conformal radiotherapy with concurrent and consolidation carboplatin plus paclitaxel with or without cetuximab for patients with stage IIIA or IIIB non-small-cell lung cancer (RTOG 0617): a randomised, two-by-two factorial phase 3 study. Lancet Oncol 2015;16:187-99. [Crossref] [PubMed]
- Hanna N, Neubauer M, Yiannoutsos C, et al. Phase III study of cisplatin, etoposide, and concurrent chest radiation with or without consolidation docetaxel in patients with inoperable stage III non-small-cell lung cancer: the Hoosier Oncology Group and U.S. Oncology. J Clin Oncol 2008;26:5755-60. [Crossref] [PubMed]
- Vokes EE, Senan S, Treat JA, et al. PROCLAIM: A phase III study of pemetrexed, cisplatin, and radiation therapy followed by consolidation pemetrexed versus etoposide, cisplatin, and radiation therapy followed by consolidation cytotoxic chemotherapy of choice in locally advanced stage III non-small-cell lung cancer of other than predominantly squamous cell histology. Clin Lung Cancer 2009;10:193-8. [Crossref] [PubMed]
- Brahmer J, Reckamp KL, Baas P, et al. Nivolumab versus Docetaxel in Advanced Squamous-Cell Non-Small-Cell Lung Cancer. N Engl J Med 2015;373:123-35. [Crossref] [PubMed]
- Borghaei H, Paz-Ares L, Horn L, et al. Nivolumab versus Docetaxel in Advanced Nonsquamous Non-Small-Cell Lung Cancer. N Engl J Med 2015;373:1627-39. [Crossref] [PubMed]
- Garon EB, Rizvi NA, Hui R, et al. Pembrolizumab for the treatment of non-small-cell lung cancer. N Engl J Med 2015;372:2018-28. [Crossref] [PubMed]
- Reck M, Rodriguez-Abreu D, Robinson AG, et al. Pembrolizumab versus Chemotherapy for PD-L1-Positive Non-Small-Cell Lung Cancer. N Engl J Med 2016;375:1823-33. [Crossref] [PubMed]
- Lugade AA, Moran JP, Gerber SA, et al. Local radiation therapy of B16 melanoma tumors increases the generation of tumor antigen-specific effector cells that traffic to the tumor. J Immunol 2005;174:7516-23. [Crossref] [PubMed]
- Schaue D, Ratikan JA, Iwamoto KS, et al. Maximizing tumor immunity with fractionated radiation. Int J Radiat Oncol Biol Phys 2012;83:1306-10. [Crossref] [PubMed]
- Demaria S, Formenti SC. Radiation as an immunological adjuvant: current evidence on dose and fractionation. Front Oncol 2012;2:153. [Crossref] [PubMed]
- Golden EB, Formenti SC. Is tumor (R)ejection by the immune system the "5th R" of radiobiology? Oncoimmunology 2014;3:e28133. [Crossref] [PubMed]
- Golden EB, Frances D, Pellicciotta I, et al. Radiation fosters dose-dependent and chemotherapy-induced immunogenic cell death. Oncoimmunology 2014;3:e28518. [Crossref] [PubMed]
- Formenti SC, Demaria S. Radiation therapy to convert the tumor into an in situ vaccine. Int J Radiat Oncol Biol Phys 2012;84:879-80. [Crossref] [PubMed]
- McBride WH, Chiang CS, Olson JL, et al. A sense of danger from radiation. Radiat Res 2004;162:1-19. [Crossref] [PubMed]
- Formenti SC, Demaria S. Combining radiotherapy and cancer immunotherapy: a paradigm shift. J Natl Cancer Inst 2013;105:256-65. [Crossref] [PubMed]
- Klug F, Prakash H, Huber PE, et al. Low-dose irradiation programs macrophage differentiation to an iNOS(+)/M1 phenotype that orchestrates effective T cell immunotherapy. Cancer cell 2013;24:589-602. [Crossref] [PubMed]
- Postow MA, Callahan MK, Barker CA, et al. Immunologic correlates of the abscopal effect in a patient with melanoma. N Engl J Med 2012;366:925-31. [Crossref] [PubMed]
- Demaria S, Ng B, Devitt ML, et al. Ionizing radiation inhibition of distant untreated tumors (abscopal effect) is immune mediated. Int J Radiat Oncol Biol Phys 2004;58:862-70. [Crossref] [PubMed]
- Okazaki T, Honjo T. The PD-1-PD-L pathway in immunological tolerance. Trends Immunol 2006;27:195-201. [Crossref] [PubMed]
- Tseng SY, Otsuji M, Gorski K, et al. B7-DC, a new dendritic cell molecule with potent costimulatory properties for T cells. J Exp Med 2001;193:839-46. [Crossref] [PubMed]
- Dong H, Strome SE, Salomao DR, et al. Tumor-associated B7-H1 promotes T-cell apoptosis: a potential mechanism of immune evasion. Nat Med 2002;8:793-800. [Crossref] [PubMed]
- Wang L, Pino-Lagos K, de Vries VC, et al. Programmed death 1 ligand signaling regulates the generation of adaptive Foxp3+CD4+ regulatory T cells. Proc Natl Acad Sci U S A 2008;105:9331-6. [Crossref] [PubMed]
- Iwai Y, Ishida M, Tanaka Y, et al. Involvement of PD-L1 on tumor cells in the escape from host immune system and tumor immunotherapy by PD-L1 blockade. Proc Natl Acad Sci U S A 2002;99:12293-7. [Crossref] [PubMed]
- Rizvi NA, Hellmann MD, Snyder A, et al. Cancer immunology. Mutational landscape determines sensitivity to PD-1 blockade in non-small cell lung cancer. Science 2015;348:124-8. [Crossref] [PubMed]
- Liang H, Deng L, Chmura S, et al. Radiation-induced equilibrium is a balance between tumor cell proliferation and T cell-mediated killing. J Immunol 2013;190:5874-81. [Crossref] [PubMed]
- Deng L, Liang H, Burnette B, et al. Irradiation and anti-PD-L1 treatment synergistically promote antitumor immunity in mice. J Clin Invest 2014;124:687-95. [Crossref] [PubMed]
- Sharabi AB, Nirschl CJ, Kochel CM, et al. Stereotactic Radiation Therapy Augments Antigen-Specific PD-1-Mediated Antitumor Immune Responses via Cross-Presentation of Tumor Antigen. Cancer Immunol Res 2015;3:345-55. [Crossref] [PubMed]
- Dewan MZ, Galloway AE, Kawashima N, et al. Fractionated but not single-dose radiotherapy induces an immune-mediated abscopal effect when combined with anti-CTLA-4 antibody. Clin Cancer Res 2009;15:5379-88. [Crossref] [PubMed]
- Stamell EF, Wolchok JD, Gnjatic S, et al. The abscopal effect associated with a systemic anti-melanoma immune response. Int J Radiat Oncol Biol Phys 2013;85:293-5. [Crossref] [PubMed]
- Golden EB, Demaria S, Schiff PB, et al. An abscopal response to radiation and ipilimumab in a patient with metastatic non-small cell lung cancer. Cancer Immunol Res 2013;1:365-72. [Crossref] [PubMed]
- Golden EB, Apetoh L. Radiotherapy and immunogenic cell death. Semin Radiat Oncol 2015;25:11-7. [Crossref] [PubMed]
- Gulley JL, Arlen PM, Bastian A, et al. Combining a recombinant cancer vaccine with standard definitive radiotherapy in patients with localized prostate cancer. Clin Cancer Res 2005;11:3353-62. [Crossref] [PubMed]
- Butts C, Socinski MA, Mitchell PL, et al. Tecemotide (L-BLP25) versus placebo after chemoradiotherapy for stage III non-small-cell lung cancer (START): a randomised, double-blind, phase 3 trial. Lancet Oncol 2014;15:59-68. [Crossref] [PubMed]
- Berman AT, Simone CB 2nd. Immunotherapy in locally-advanced non-small cell lung cancer: releasing the brakes on consolidation? Transl Lung Cancer Res 2016;5:138-42. [PubMed]
- Mitchell P, Thatcher N, Socinski MA, et al. Tecemotide in unresectable stage III non-small-cell lung cancer in the phase III START study: updated overall survival and biomarker analyses. Ann Oncol 2015;26:1134-42. [Crossref] [PubMed]
- Katakami N, Hida T, Nokihara H, et al. Phase I/II study of tecemotide as immunotherapy in Japanese patients with unresectable stage III non-small cell lung cancer. Lung cancer 2017;105:23-30. [Crossref] [PubMed]
- Brunsvig PF, Kyte JA, Kersten C, et al. Telomerase peptide vaccination in NSCLC: a phase II trial in stage III patients vaccinated after chemoradiotherapy and an 8-year update on a phase I/II trial. Clin Cancer Res 2011;17:6847-57. [Crossref] [PubMed]
- Hansen GL, Gaudernack G, Brunsvig PF, et al. Immunological factors influencing clinical outcome in lung cancer patients after telomerase peptide vaccination. Cancer Immunol Immunother 2015;64:1609-21. [Crossref] [PubMed]
- Naidoo J, Wang X, Woo KM, et al. Pneumonitis in Patients Treated With Anti-Programmed Death-1/Programmed Death Ligand 1 Therapy. J Clin Oncol 2017;35:709-17. [Crossref] [PubMed]
- Johnson DB, Balko JM, Compton ML, et al. Fulminant Myocarditis with Combination Immune Checkpoint Blockade. N Engl J Med 2016;375:1749-55. [Crossref] [PubMed]