Diagnostic and prognostic biomarkers for malignant mesothelioma: an update
Introduction
Malignant mesothelioma (MM), is an aggressive cancer arising from the surfaces of the pleural and peritoneal cavities, and it is predominantly caused by exposure to asbestos or asbestos-like fibers (1). These carcinogenic fibers induce cell necrosis, with consequent release of high mobility group protein B1 (HMGB1) and activation of the Nalp3 inflammasome, leading to chronic inflammation, DNA damage and carcinogenesis (2-4). There are approximately 3,200 cases of MM per year in the United States and at least 34,000 cases worldwide in 2013 (1,5). Asbestos continues to be used worldwide, particularly in newly industrializing countries, such as China and India (6,7), therefore the incidence of MM is expected to increase during the course of the next decades.
Exposure to asbestos fibers and to carcinogenic mineral fibers not commonly classified as asbestos is considered as the main cause of MM (1,4,5,8-10). However, additional factors including SV40 infection (11-13), exposure to radiation, especially high doses radiotherapy of lymphoma and other chest malignancies (1), may also cause mesothelioma, possibly in concert with asbestos (14,15).
Moreover, we demonstrated that germline heterozygous inactivating mutations of the BRCA1 associated protein-1 (BAP1) gene cause the high penetrance hereditary BAP1 cancer syndrome (16,17). The individuals carrying the germline heterozygous BAP1 mutations develop multiple cancers including mesothelioma, even if not occupationally exposed to asbestos (8), suggesting that they may have acquired an increased susceptibility to very low levels of asbestos exposure, otherwise harmless to the general population. This hypothesis was supported by the findings in germline BAP1 heterozygous mice, where minimal exposure to carcinogenic fibers highly increased the risk of mesothelioma (15).
MM is classified into three histological subtypes. The epithelioid subtype characterizes about 70% of all MMs and is less aggressive than the sarcomatoid type, which is highly resistant to chemotherapy and associated with the poorest survival. The biphasic subtype has intermediate characteristics and possibly corresponds to a transition between the other two histological subtypes (18). However, differential diagnosis of MM is challenging, because MM morphology is similar to other cancers. In the epithelioid MM subtype morphology can be confused with that of non-small cell lung carcinomas, renal cell carcinomas, and others (19). The morphology of biphasic MMs can be similar to the one of synovial sarcomas and other biphasic malignancies, whilst sarcomatoid mesothelioma is often morphologically indistinguishable from other spindle cell tumors, including carcinosarcoma. This diagnostic uncertainty is a serious and critical issue because patients with different cancers need different treatments and may have different prognosis. The accuracy of MM diagnosis has been improved by using a set of immunohistochemical (IHC) markers, including mesothelial markers (calretinin, the most sensitive, and WT-1, the most specific) and carcinoma-related markers (CEA, CD15, Ber-EP4, MOC-31, TTF-1) for differential diagnosis of carcinoma (19,20). It is by combining the results obtained with these markers together that a correct diagnosis can be obtained, because—as an example—calretinin and WT1 staining is not exclusively specific for MM, but can be found also in other malignancies. Additional IHC markers can be used to distinguish MM from other malignancies: PAX8 positivity in a pleural tumor allows identifying metastatic renal cell carcinoma, and positive staining for WT1, estrogen receptor (ER), and progesterone receptor (PR) in a peritoneal malignancy allows a differential diagnosis of ovarian carcinoma from MM. Notably, these tumors are morphologically indistinguishable and both stain positively for WT1. However, although these IHC diagnostic tools are of undeniable help to diagnose correctly most MMs, there are still about 10% of cases in which the diagnosis remains dubious because, for example, in both MM and lung carcinoma markers result as either positive or negative, or only a fraction of tumor cells are positive (19). Moreover, in the absence of evident MM tumor cell invasion into the surrounding normal tissues, it is particularly challenging to distinguish atypical mesothelial hyperplasia from epithelioid type MM, as well as biphasic and sarcomatoid MM subtypes from organizing pleuritis (21,22). Misdiagnosis of MM is a general problem worldwide. In France, the accuracy of initial diagnosis of MM was reported only in 67% of cases (23). We recently reviewed 92 pathological diagnoses of MM from China and found that the diagnostic accuracy rate was about 56.6% (24). In our experience, there are about 1/10 cases of MMs misdiagnosed in the US. Most of the times, these misdiagnoses can be attributed to pathologists who have rarely seen this malignancy, who attempt diagnosis on very small biopsies and/or cytology specimens, or who make a diagnosis with an insufficient set of IHC markers. Misdiagnosis leads to delayed treatment, negatively impacting on patient survival. Therefore, more specific and sensitive diagnostic biomarkers for MM are urgently needed to increase the accuracy of diagnosis of this aggressive and rapidly progressing cancer.
MM develops with a latency of 20–60 years from asbestos exposure (1). Because the tumor is difficult to diagnose and the initial symptoms can be insidious, the disease is often diagnosed at an advanced stage, and this delay is associated with a median survival of about 12 months (5,18). Moreover, MM, in general, is poorly responsive to current therapies, but this is especially true in advanced stages of the disease. Monotherapy and combination therapy have been widely investigated for the treatment of MM however, all therapeutic strategies failed to significantly benefit patients so far (25-28). On the other hand, it has been shown that when patients are diagnosed and treated at an early stage (stage I) of MM, the overall median survival is significantly improved (29,30). Therefore, the relevance of early diagnosis of MM is very high for a better response to therapy. However, only 5% of MM patients are currently diagnosed at an early stage (5).
Monitoring cohorts at high risk of developing MM, because of documented exposure to asbestos or other mineral fibers, or because they carry germline BAP1 mutations, can be a helpful strategy to make MM diagnoses at earlier stages, when the malignancy may be more susceptible to therapy. In this perspective, the availability of specific and sensitive biomarkers for asbestos exposure and/or MM would facilitate monitoring cohorts over the course of the years.
A biomarker generally refers to a measurable indicator of some biological state or condition, including a pathogenic process. Different novel biomarkers have been utilized in pre-clinical studies and diagnosis to predict, detect and monitor cancers along several last decades.
For MM diagnosis biomarkers include metabolites, proteins, and microRNAs (miRNAs). An ideal biomarker should selectively detect MM patients from other malignancies or asbestos-exposed subjects from non-exposed individuals. The ideal sample types for detecting biomarkers are blood and pleural effusion (PE), which can be easily collected. This review focuses on some of the most frequently used and promising biomarkers for early detection and diagnosis of MM.
Soluble mesothelin-related peptides (SMRPs)
Mesothelin is a 71-kDa precursor protein, which is physiologically cleaved into two mature proteins: the 31-kDa NH2-terminal megakaryocyte potentiating factor (MPF), secreted into the blood, and the 40-kDa COOH-terminal glycosylated phosphatidylinositol-linked glycoprotein, which is a plasma membrane-bound protein. After further processing, the SMRPs is released from the cell, and this is the most studied and so far the only Food and Drug Administration (FDA)-approved biomarker for MM (31,32). Mesothelin is expressed at low levels in normal mesothelial cells and is undetectable in most normal tissues. On the contrary, mesothelin is overexpressed in several human cancers, including MM (33,34), pancreatic adenocarcinoma (35), ovarian (36) and lung cancers (37). The level of serum SMRPs has been proposed for identifying MM patients among asbestos-unexposed/exposed individuals and individuals with benign pleural diseases, with a sensitivity of 60–90% and specificity of 80–85% (38-41). Furthermore, it has been proposed that serum SMRPs levels may also differentiate MM patients from patients with pleural metastases of different types of carcinomas (39,40,42). However, serum mesothelin levels are elevated also in patients with renal impairment, and therefore renal function has to be taken into account during the interpretation of this assay (43).
SMRPs in PEs may also be a useful diagnostic biomarker for MM. SMRPs levels were evaluated in MM PE by enzyme-linked immunosorbent assay (ELISA) for their diagnostic performance in differentiating MM from benign pathologies and non-MM pleural metastasis and proposed as a novel diagnostic tool for MM (44). Furthermore, SMRPs levels detected in pleural effusion (PE-SMRPs) were compared with those detected in serum (S-SMRPs) for their performance in diagnosis of patients with MM, non-MM pleural metastases, and benign pleural diseases. The result suggested that PE-SMRPs had a higher diagnostic performance than S-SMRPs in identifying MM (45).
Two meta-analysis evaluated sensitivity, specificity, and accuracy of serum SMRPs in the diagnosis of MM. A systematic review and meta-analysis of 12 studies in 717 MM patients and 2,851 control subjects including healthy control and patients with non-MM diseases. This study revealed 64% sensitivity [95% confidence interval (CI) 61–68%] and 89% specificity (95% CI 88–90%) of serum SMRPs in the diagnosis of MM (46). The meta-analysis of Hollevoet et al. examined 16 studies in 4,491 controls and 1,026 MM patients (47). The results showed a sensitivity of 32% (95% CI 26–40%) and 95% specificity for mesothelin. These results indicate that, although SMRPs may help to identify MM, the sensitivity of the assay is inadequate, limiting its application in early diagnosis of MM (47).
MPF
MPF is a 31-kDa secreted cytokine, which derives from the cleavage of mesothelin, similarly to SMRPs (31). Like SMRPs, MPF was evaluated by ELISA in serum samples from MM patients and different control subjects. Higher serum MPF levels were detected in MM patients, compared to healthy subjects, individuals with benign asbestos-related diseases, or lung cancer patients (48,49).
A prospective multicenter study was performed to measure the diagnostic performance of MPF in MM (50). This study enrolled 507 participants, grouped into six cohorts: 101 healthy controls, 46 individuals with benign respiratory disease, 89 healthy asbestos-exposed individuals, 123 patients with benign asbestos-related diseases, 63 lung cancer and 85 MM patients. The result of this large study revealed that both serum SMRPs and MPF levels allow differentiating MM patients from the other cohorts (P<0.001). Moreover, no significant difference was found between SMRPs and MPF (SMRPs =0.871, MPF =0.849; P=0.28). A further study confirmed equivalent diagnostic performances of SMRPs and MPF in distinguishing MM from other diseases, using samples from PE and/or serum (31).
The influence of clinical covariates on SMRPs and MPF levels and their diagnostic value were examined by a multicenter study in a total of 594 participants, including 106 MM patients and 488 control subjects, which found that SMRPs and MPF levels were independently associated with age, glomerular filtration rate (GFR), and body mass index (BMI) in control subjects, and with GFR and tumor stage in patients with MM. Moreover, the diagnostic performances of SMRPs and MPF were significantly affected by the distribution of these covariates, implying that age, GFR, and BMI should be routinely recorded when measuring these biomarkers. The definition of the approaches to account for these covariates requires further validation (51).
Osteopontin (OPN)
OPN is a secreted glycoprotein that plays critical roles in several biological processes, such as cell-matrix interaction, immunological regulation, tumor development, and cell migration (52-55). The circulating serum OPN levels are elevated in several cancers, including MM (56), colon (57), lung (58), and breast cancer (59). Therefore, serum OPN has been identified as a potential biomarker for early detection of MM (60,61). In particular, Pass et al. discovered the link between increased OPN levels and MM (60), by measuring OPN levels in tumor tissues and sera of 49 controls (with no documented exposure to asbestos), 69 asbestos-exposed individuals and 76 MM patients (60). This study showed that there was no significant difference in serum OPN levels between subjects with asbestos exposure and with no asbestos exposure. However, OPN levels in MM were significantly elevated compared to controls and asbestos-exposed individuals. The performance of serum OPN assay was good with 77.6% sensitivity and 85.5% specificity, pointing at OPN as a promising biomarker for the identification of MM patients (60).A study comparing serum OPN levels from 96 patients with MM and 112 healthy asbestos-exposed subjects showed that assays based on serum OPN levels displayed good sensitivity and specificity to distinguish MM patients from asbestos-exposed individuals (62). However, the OPN assay was unable to distinguish MM from pleural metastases, carcinomas or benign pleural lesions associated with asbestos exposure (BPLAE or pleural plaques) (62). The diagnostic performance of OPN for MM was further investigated in several other studies (63-67) with conflicting results. Some of the discrepancies can be explained by the source of the sample used for the OPN assay. Indeed, two separate studies comparing serum and plasma OPN revealed that the diagnostic performance of the OPN assay was higher when performed in plasma than in serum (65,68), because of the lower stability of serum OPN caused by thrombin cleavage during the coagulation process, which may yield unreliable results (62,69).A more recent systematic review and meta-analysis evaluated the overall diagnostic accuracy of serum OPN measurement for diagnosing MM (64). The results of this study showed that pooled sensitivity was 57% (95% CI 52–61%), and specificity was 81% (95% CI 79–84%), the area under the curve (AUC) of the receiver operating characteristic (ROC) curve being 0.80. These data suggested that OPN was a helpful diagnostic biomarker for MM (64).
Fibulin-3
Human fibulin-3 is a secreted glycoprotein encoded by the epidermal growth factor (EGF)-containing fibulin-like extracellular matrix protein-1 (EFEMP-1) gene, involved in the regulation of MM cell proliferation and migration (70).
To assess fibulin-3 as a potential diagnostic marker for MM, its levels of expression were evaluated (71). In this study, plasma fibulin-3 levels, PE fibulin-3 levels, and tumor tissue fibulin-3 levels were all analyzed. The results showed that fibulin-3 preferentially stained tumor cells and that the ROC for fibulin-3 displayed a sensitivity of 96.7% and a specificity of 95.5%, findings suggesting that fibulin-3 was a valuable biomarker for MM. However, other research teams reported a much lower sensitivity of fibulin-3 for MM diagnosis (32,72).
A comprehensive meta-analysis was conducted on 7 studies in 468 MM cases to establish the clinical diagnostic value of fibulin-3 for MM (70). The pooled sensitivity and specificity of the fibulin-3 assay was 62% (95% CI 45–77%) and of 82% (95% CI 73–89%), respectively and MM cases were discriminated from controls with AUC of ROC of 0.81 (70). These data demonstrate the high diagnostic efficacy of fibulin-3, supporting previous evidence of the role of this protein as a promising diagnostic biomarker for MM (5,71,73).
High Mobility Group Box 1 protein (HMGB1)
HMGB1 is a damage-associated molecular pattern (DAMP) and mediates several biological processes such as transcription, cell proliferation, DNA repair and inflammation (74,75). HMGB1 has been extensively studied as nuclear protein, however, acetylation of HMGB1 prevents nuclear translocation, leading to its accumulation in the cytoplasm. Inflammatory cells, such as granulocytes and macrophages, can release acetylated HMGB1 from the cytosol in the extracellular milieu, where it displays pro-inflammatory activity (76,77). HMGB1 is also passively released by cells undergoing programmed cell necrosis. We discovered that exposure of primary human mesothelial cells to asbestos fibers induces programmed necrosis and consequent release of HMGB1, which in turn triggers the process of cell transformation (3,4,78). Moreover, we demonstrated that MM cells become addicted to HMGB1 for growth and invasion. Accordingly, MM cells actively secrete HMGB1 in an autocrine fashion, as demonstrated by the interference of HMGB1 antagonists on MM growth in vitro and in vivo (78).
Based on these findings we hypothesized that the serum of individuals exposed to asbestos contain mainly non-acetylated HMGB1 (as expected by cells undergoing asbestos-induced programmed necrosis), while the serum of patients with MM contains mainly hyperacetylated HMGB1, as expected following active secretion of this cytokine by cancer cells. Consistently, we found that HMGB1 levels in serum and plasma were higher in MM patients compared to healthy individuals (Figure 1) (78) and these findings were confirmed by other studies reporting significantly higher serum HMGB1 levels in MM patients compared to individuals with benign asbestos-related diseases (79,80). A systematic review and meta-analysis established HMGB1 as a prognostic marker for MM (79,81).
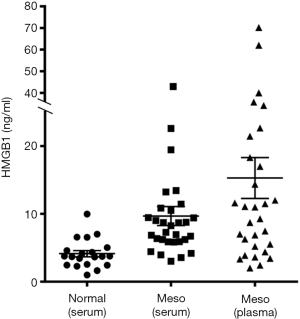
More recently, we discovered that hyperacetylated HMGB1 was significantly higher in MM patients compared to asbestos-exposed individuals and healthy controls and that HMGB1 levels do not appear to be influenced by tumor stage (82). The sensitivity and specificity of serum hyper-acetylated HMGB1 in discriminating MM patients and asbestos-exposed individuals and healthy controls was 100%, outperforming any other previously studied biomarkers. The combination of HMGB1 and fibulin-3 produced a better sensitivity and specificity in differentiating MM patients from patients with benign or malignant non-MM PE. These findings suggest that hyperacetylated HMGB1 is a valuable biomarker to identify MM patients among unexposed individuals or individuals occupationally exposed to asbestos (82). Validation studies are in progress.
microRNAs (miRNAs)
miRNAs are small non-coding RNA molecules of 18-22 nucleotides, which regulate gene expression at the post-transcriptional level by binding the 3’-untranslated regions of target miRNAs (83,84); 2,588 human miRNAs have been identified so far. These miRNAs are expected to target about 50% of human miRNAs (85), regulating many cellular activities, such as proliferation, differentiation, metabolism, apoptosis, senescence, angiogenesis, invasion (86). Deregulated miRNAs commonly occur in many cancers and different miRNAs have been proposed as promising diagnostic biomarkers in many cancers, including MM. In general miRNAs are excellent biomarkers because are stable and can be analyzed in routinely processed tissue samples (87), as well as in blood samples (88,89).
Several groups explored the miRNA expression profiles in MM tissues using microarrays (90-95). An initial analysis of miRNAs expression in MM (94) reported 12 miRNAs overexpressed and 9 miRNAs down-regulated in MM tissues compared with normal tissues. Interestingly, among over-expressed miRNAs, miR-30b*, miR-32*, miR-483-3p, miR-584, and miR-885-3p were predicted to regulate the tumor suppressor genes CDKN2A and NF2, while down-regulated miRNAs, miR-9, miR-7-1* and miR-203 were expected to target the oncogenes HGF, PDGFA, EGF and JUN (94). In a different study, the first miRNA signature of MM and mesothelial cells was obtained, revealing 10 miRNAs overexpressed and 19 miRNAs down-regulated in MM cells (93). The validation of these miRNAs by qRT-PCR in 24 MM specimens (epithelioid, biphasic, and sarcomatoid) significantly linked several overexpressed miRNAs (miR-17-5p, miR-21, miR-29a, miR-30c, miR-30e-5p, miR-106a, and miR-143) to histopathological subtypes of MM. In addition, the expression of miR-17-5p and miR-30c was correlated with survival in sarcomatoid MM (93). Another study assigned to miR-29c* elevated expression a significantly higher survival of MM patients (96), and loss of miR-31 (linked with frequent homozygous loss of 9p21.3 chromosome in MM) was associated with tumor suppressor activity (97). Finally, a survey was performed on a panel of formalin-fixed paraffin embedded (FPPE) specimens of MM patients undergoing extrapleural pneumonectomy (EPP) or palliative surgery (P/D), to explore the association between miRNA expression and patient overall survival. The results generated the miR-Score, a signature of 6 miRNAs (miR-21-5p, miR-23a-3p, miR-30e-5p, miR-221-3p, miR-222-3p, and miR-31-5p), which allowed predicting long survival patients. The performance of the miR-Score was evaluated by ROC curve analysis that revealed 92.3% and 71.9% accuracy for patients undergoing EPP and P/D, respectively (98). These data suggested that deregulated miRNAs can be promising diagnostic biomarkers and prognostic factors for MM as well.
Also cell-free, circulating miRNAs have been suggested as biomarkers for MM (99-101). A plasma miRNA profiling was performed by comparing samples from MM patients and those from healthy controls. Three prognostic miRNA (miR-29c*, miR-92a, and the newly identified miR-625-3p) were validated as promising diagnostic markers for MM (92). Notably, miR29c* was already identified also as a prognostic marker in a previous study (96). Two distinct serum miRNA signatures were identified in MM patients with both diagnostic and prognostic significance (90).
Proteomics
The proteome is the complete set of proteins expressed by an organism or a system, at a certain time and under defined physiological or pathological conditions. A proteomics strategy is a high-throughput approach yielding a protein signature, which has been recently exploited for the effective screening of a high number biomarkers, significantly improving the diagnostic accuracy in different cancers (102), including MM (103,104).
The SOMAmer proteomic technology (105) has been used to screen serological diagnostic markers for MM in a multicenter case-control study including 117 MM patients and 142 control subjects with asbestos exposure (104). Over 1,000 proteins were screened, 64 candidate biomarkers were discovered, and a 13-marker random forest classifier was developed from the candidates, including inflammatory and proliferative proteins. This random forest model differentiated MM from controls with AUC of 0.99, both sensitivity and specificity of >90%, superior to the performance of mesothelin (AUC 0.82, sensitivity 66%, specificity 88%) (104). The potency of this proteomics approach, providing a multiplex biomarker signature, is likely a promising MM diagnostic tool (106).
A seven glycopeptide signature was identified by selected reaction monitoring (SRM) assay technology in MM cells and used to investigate surfaceome derived serum candidate biomarker panels for MM (107). The seven glycopeptide panel accurately discriminated MM from healthy controls and, in combination with mesothelin ELISA, significantly improved the diagnostic accuracy of mesothelin in differentiating MM from non-small-cell lung cancer (NSCLC) (107). Tissue-based proteomics studies in MM and benign biopsies (103) and in MM-derived exosomes (108) identified both already known biomarkers and novel potential candidates, whose biological significance need to be validated by further investigations.
Conclusions
Many million people have been exposed to asbestos in the US and worldwide, causing continuing increase of MM morbidity and mortality and a high number of individuals at risk of developing MM (82). MM is mostly diagnosed at an advanced stage when it is poorly responsive to the current therapies and it was demonstrated that early diagnosis significantly improves overall survival (5,29,30). However, the currently available tissue and serological diagnostic biomarkers are characterized by relatively poor sensitivity and specificity preventing the use of reliable tools both for identification of individuals exposed to asbestos and other carcinogenic fibers and for early detection in patients who are developing MM (109).
The only FDA-approved diagnostic biomarker for MM, serum SMRPs, has low sensitivity, and it was approved only to monitor tumor recurrence after therapy. Novel, and potentially more sensitive and specific MM biomarkers were evaluated, including MPF(31), OPN (60,64,66), fibulin-3 (70,72,73), HMGB1 (82), with promising results. In addition, a variety of deregulated miRNAs [reviewed in (109)], including the miR-score signature with prognostic significance (98), as well as different approaches based on proteomics technology, have been proposed as suitable MM markers (100,109). It is conceivable that a combination of the most performing and valuable markers validated by the ongoing studies will allow more accurate MM diagnosis and earlier detection in the near future.
Acknowledgements
Funding: This work was supported by NCI-R01 CA160715, DOD CA120355, and The Riviera United 4-a Cure to H.Y, and by NCI-R01 CA198138-01 to M.C., and by the University of Hawai’i Foundation, which received unrestricted donations to support mesothelioma research from Honeywell International Inc., to M.C.
Footnote
Conflicts of Interest: M.C. and H.Y. have pending patent applications on BAP1 and HMGB1; M.C. provides consultation for mesothelioma diagnosis.
References
- Carbone M, Ly BH, Dodson RF, et al. Malignant mesothelioma: facts, myths, and hypotheses. J Cell Physiol 2012;227:44-58. [Crossref] [PubMed]
- Dostert C, Petrilli V, Van Bruggen R, et al. Innate immune activation through Nalp3 inflammasome sensing of asbestos and silica. Science 2008;320:674-7. [Crossref] [PubMed]
- Yang H, Rivera Z, Jube S, et al. Programmed necrosis induced by asbestos in human mesothelial cells causes high-mobility group box 1 protein release and resultant inflammation. Proc Natl Acad Sci U S A 2010;107:12611-6. [Crossref] [PubMed]
- Qi F, Okimoto G, Jube S, et al. Continuous exposure to chrysotile asbestos can cause transformation of human mesothelial cells via HMGB1 and TNF-alpha signaling. Am J Pathol 2013;183:1654-66. [Crossref] [PubMed]
- Carbone M, Kanodia S, Chao A, et al. Consensus Report of the 2015 Weinman International Conference on Mesothelioma. J Thorac Oncol 2016;11:1246-62. [Crossref] [PubMed]
- Burki T. Health experts concerned over India's asbestos industry. Lancet 2010;375:626-7. [Crossref] [PubMed]
- Mao W, Zhang X, Guo Z, et al. Association of Asbestos Exposure With Malignant Mesothelioma Incidence in Eastern China. JAMA Oncol 2017;3:562-4. [Crossref] [PubMed]
- Baumann F, Flores E, Napolitano A, et al. Mesothelioma patients with germline BAP1 mutations have 7-fold improved long-term survival. Carcinogenesis 2015;36:76-81. [Crossref] [PubMed]
- Baumann F, Ambrosi JP, Carbone M. Asbestos is not just asbestos: an unrecognised health hazard. Lancet Oncol 2013;14:576-8. [Crossref] [PubMed]
- Carbone M, Emri S, Dogan AU, et al. A mesothelioma epidemic in Cappadocia: scientific developments and unexpected social outcomes. Nat Rev Cancer 2007;7:147-54. [Crossref] [PubMed]
- Gazdar AF, Carbone M. Molecular pathogenesis of malignant mesothelioma and its relationship to simian virus 40. Clin Lung Cancer 2003;5:177-81. [Crossref] [PubMed]
- Carbone M, Rizzo P, Pass H. Simian virus 40: the link with human malignant mesothelioma is well established. Anticancer Res 2000;20:875-7. [PubMed]
- Carbone M. Simian virus 40 and human tumors: It is time to study mechanisms. J Cell Biochem 1999;76:189-93. [Crossref] [PubMed]
- Kroczynska B, Cutrone R, Bocchetta M, et al. Crocidolite asbestos and SV40 are cocarcinogens in human mesothelial cells and in causing mesothelioma in hamsters. Proc Natl Acad Sci U S A 2006;103:14128-33. [Crossref] [PubMed]
- Napolitano A, Pellegrini L, Dey A, et al. Minimal asbestos exposure in germline BAP1 heterozygous mice is associated with deregulated inflammatory response and increased risk of mesothelioma. Oncogene 2016;35:1996-2002. [Crossref] [PubMed]
- Testa JR, Cheung M, Pei J, et al. Germline BAP1 mutations predispose to malignant mesothelioma. Nat Genet 2011;43:1022-5. [Crossref] [PubMed]
- Carbone M, Yang H, Pass HI, et al. BAP1 and cancer. Nat Rev Cancer 2013;13:153-9. [Crossref] [PubMed]
- Pass HI, Carbone M, Krug LM, et al. Benign and Malignant Mesothelioma. In: De Vita VT, Hellmann, S, Rosemberg, S.A., editor. Cancer, Principles & Practice of Oncology. 10th ed. Baltimore: Lippincott Williams & Wilkins; 2014:1738-60.
- Carbone M, Shimizu D, Napolitano A, et al. Positive nuclear BAP1 immunostaining helps differentiate non-small cell lung carcinomas from malignant mesothelioma. Oncotarget 2016;7:59314-21. [PubMed]
- Betta PG, Magnani C, Bensi T, et al. Immunohistochemistry and molecular diagnostics of pleural malignant mesothelioma. Arch Pathol Lab Med 2012;136:253-61. [Crossref] [PubMed]
- Churg A, Sheffield BS, Galateau-Salle F. New Markers for Separating Benign From Malignant Mesothelial Proliferations: Are We There Yet? Arch Pathol Lab Med 2016;140:318-21. [Crossref] [PubMed]
- Zimling ZG, Jorgensen A, Santoni-Rugiu E. The diagnostic value of immunohistochemically detected methylthioadenosine phosphorylase deficiency in malignant pleural mesotheliomas. Histopathology 2012;60:E96-105. [Crossref] [PubMed]
- Goldberg M, Imbernon E, Rolland P, et al. The French National Mesothelioma Surveillance Program. Occup Environ Med 2006;63:390-5. [Crossref] [PubMed]
- Guo Z, Carbone M, Zhang X, et al. Improving the Accuracy of Mesothelioma Diagnosis in China. J Thorac Oncol 2017;12:714-23. [PubMed]
- Hoang CD. Surgical controversies in mesothelioma: MesoVATS addresses the role of surgical debulking. Transl Lung Cancer Res 2016;5:82-4. [PubMed]
- Abdel-Rahman O. Role of postoperative radiotherapy in the management of malignant pleural mesothelioma: A propensity score matching of the SEER database. Strahlenther Onkol 2017;193:276-84. [Crossref] [PubMed]
- Blackwell C, Sherk C, Fricko M, et al. Inhibition of FGF/FGFR autocrine signaling in mesothelioma with the FGF ligand trap, FP-1039/GSK3052230. Oncotarget 2016;7:39861-71. [PubMed]
- Kaufman AJ, Pass HI. Current concepts in malignant pleural mesothelioma. Expert Rev Anticancer Ther 2008;8:293-303. [Crossref] [PubMed]
- Rusch VW, Chansky K, Kindler HL, et al. The IASLC Mesothelioma Staging Project: Proposals for the M Descriptors and for Revision of the TNM Stage Groupings in the Forthcoming (Eighth) Edition of the TNM Classification for Mesothelioma. J Thorac Oncol 2016;11:2112-9. [Crossref] [PubMed]
- Nowak AK, Chansky K, Rice DC, et al. The IASLC Mesothelioma Staging Project: Proposals for Revisions of the T Descriptors in the Forthcoming Eighth Edition of the TNM Classification for Pleural Mesothelioma. J Thorac Oncol 2016;11:2089-99.
- Creaney J, Sneddon S, Dick IM, et al. Comparison of the diagnostic accuracy of the MSLN gene products, mesothelin and megakaryocyte potentiating factor, as biomarkers for mesothelioma in pleural effusions and serum. Dis Markers 2013;35:119-27. [Crossref] [PubMed]
- Creaney J, Dick IM, Meniawy TM, et al. Comparison of fibulin-3 and mesothelin as markers in malignant mesothelioma. Thorax 2014;69:895-902. [Crossref] [PubMed]
- Servais EL, Colovos C, Rodriguez L, et al. Mesothelin overexpression promotes mesothelioma cell invasion and MMP-9 secretion in an orthotopic mouse model and in epithelioid pleural mesothelioma patients. Clin Cancer Res 2012;18:2478-89. [Crossref] [PubMed]
- Creaney J, Olsen NJ, Brims F, et al. Serum mesothelin for early detection of asbestos-induced cancer malignant mesothelioma. Cancer Epidemiol Biomarkers Prev 2010;19:2238-46. [Crossref] [PubMed]
- Hassan R, Laszik ZG, Lerner M, et al. Mesothelin is overexpressed in pancreaticobiliary adenocarcinomas but not in normal pancreas and chronic pancreatitis. Am J Clin Pathol 2005;124:838-45. [Crossref] [PubMed]
- Chang K, Pastan I. Molecular cloning of mesothelin, a differentiation antigen present on mesothelium, mesotheliomas, and ovarian cancers. Proc Natl Acad Sci U S A 1996;93:136-40. [Crossref] [PubMed]
- Ho M, Bera TK, Willingham MC, et al. Mesothelin expression in human lung cancer. Clin Cancer Res 2007;13:1571-5. [Crossref] [PubMed]
- Amati M, Tomasetti M, Scartozzi M, et al. Profiling tumor-associated markers for early detection of malignant mesothelioma: an epidemiologic study. Cancer Epidemiol Biomarkers Prev 2008;17:163-70. [Crossref] [PubMed]
- Scherpereel A, Grigoriu B, Conti M, et al. Soluble mesothelin-related peptides in the diagnosis of malignant pleural mesothelioma. Am J Respir Crit Care Med 2006;173:1155-60. [Crossref] [PubMed]
- Hassan R, Remaley AT, Sampson ML, et al. Detection and quantitation of serum mesothelin, a tumor marker for patients with mesothelioma and ovarian cancer. Clin Cancer Res 2006;12:447-53. [Crossref] [PubMed]
- Rodríguez Portal JA, Rodriguez Becerra E, Rodriguez Rodriguez D, et al. Serum levels of soluble mesothelin-related peptides in malignant and nonmalignant asbestos-related pleural disease: relation with past asbestos exposure. Cancer Epidemiol Biomarkers Prev 2009;18:646-50. [Crossref] [PubMed]
- Beyer HL, Geschwindt RD, Glover CL, et al. MESOMARK: a potential test for malignant pleural mesothelioma. Clin Chem 2007;53:666-72. [Crossref] [PubMed]
- Boudville N, Paul R, Robinson BW, et al. Mesothelin and kidney function--analysis of relationship and implications for mesothelioma screening. Lung Cancer 2011;73:320-4. [Crossref] [PubMed]
- Canessa PA, Franceschini MC, Ferro P, et al. Evaluation of soluble mesothelin-related peptide as a diagnostic marker of malignant pleural mesothelioma effusions: its contribution to cytology. Cancer Invest 2013;31:43-50. [Crossref] [PubMed]
- Ferro P, Canessa PA, Battolla E, et al. Mesothelin is more useful in pleural effusion than in serum in the diagnosis of pleural mesothelioma. Anticancer Res 2013;33:2707-13. [PubMed]
- Luo L, Shi HZ, Liang QL, et al. Diagnostic value of soluble mesothelin-related peptides for malignant mesothelioma: a meta-analysis. Respir Med 2010;104:149-56. [Crossref] [PubMed]
- Hollevoet K, Reitsma JB, Creaney J, et al. Serum mesothelin for diagnosing malignant pleural mesothelioma: an individual patient data meta-analysis. J Clin Oncol 2012;30:1541-9. [Crossref] [PubMed]
- Shiomi K, Miyamoto H, Segawa T, et al. Novel ELISA system for detection of N-ERC/mesothelin in the sera of mesothelioma patients. Cancer Sci 2006;97:928-32. [Crossref] [PubMed]
- Onda M, Nagata S, Ho M, et al. Megakaryocyte potentiation factor cleaved from mesothelin precursor is a useful tumor marker in the serum of patients with mesothelioma. Clin Cancer Res 2006;12:4225-31. [Crossref] [PubMed]
- Hollevoet K, Nackaerts K, Thimpont J, et al. Diagnostic performance of soluble mesothelin and megakaryocyte potentiating factor in mesothelioma. Am J Respir Crit Care Med 2010;181:620-5. [Crossref] [PubMed]
- Hollevoet K, Nackaerts K, Thas O, et al. The effect of clinical covariates on the diagnostic and prognostic value of soluble mesothelin and megakaryocyte potentiating factor. Chest 2012;141:477-84. [Crossref] [PubMed]
- Chen RX, Xia YH, Xue TC, et al. Osteopontin promotes hepatocellular carcinoma invasion by up-regulating MMP-2 and uPA expression. Mol Biol Rep 2011;38:3671-7. [Crossref] [PubMed]
- Tajima K, Ohashi R, Sekido Y, et al. Osteopontin-mediated enhanced hyaluronan binding induces multidrug resistance in mesothelioma cells. Oncogene 2010;29:1941-51. [Crossref] [PubMed]
- Ohashi R, Tajima K, Takahashi F, et al. Osteopontin modulates malignant pleural mesothelioma cell functions in vitro. Anticancer Res 2009;29:2205-14. [PubMed]
- Frey AB, Wali A, Pass H, et al. Osteopontin is linked to p65 and MMP-9 expression in pulmonary adenocarcinoma but not in malignant pleural mesothelioma. Histopathology 2007;50:720-6. [Crossref] [PubMed]
- Turgut Cosan D, Oner C, Mutlu Sahin F. Micro RNA-126 coordinates cell behavior and signaling cascades according to characteristics of breast cancer cells. Bratisl Lek Listy 2016;117:639-47. [PubMed]
- Coppola D, Szabo M, Boulware D, et al. Correlation of osteopontin protein expression and pathological stage across a wide variety of tumor histologies. Clin Cancer Res 2004;10:184-90. [Crossref] [PubMed]
- Rouanne M, Adam J, Goubar A, et al. Osteopontin and thrombospondin-1 play opposite roles in promoting tumor aggressiveness of primary resected non-small cell lung cancer. BMC Cancer 2016;16:483. [Crossref] [PubMed]
- El-Tanani MK, Yuen HF, Shi Z, et al. Osteopontin can act as an effector for a germline mutation of BRCA1 in malignant transformation of breast cancer-related cells. Cancer Sci 2010;101:1354-60. [Crossref] [PubMed]
- Pass HI, Lott D, Lonardo F, et al. Asbestos exposure, pleural mesothelioma, and serum osteopontin levels. N Engl J Med 2005;353:1564-73. [Crossref] [PubMed]
- Ivanov SV, Ivanova AV, Goparaju CM, et al. Tumorigenic properties of alternative osteopontin isoforms in mesothelioma. Biochem Biophys Res Commun 2009;382:514-8. [Crossref] [PubMed]
- Grigoriu BD, Scherpereel A, Devos P, et al. Utility of osteopontin and serum mesothelin in malignant pleural mesothelioma diagnosis and prognosis assessment. Clin Cancer Res 2007;13:2928-35. [Crossref] [PubMed]
- Paleari L, Rotolo N, Imperatori A, et al. Osteopontin is not a specific marker in malignant pleural mesothelioma. Int J Biol Markers 2009;24:112-7. [Crossref] [PubMed]
- Hu ZD, Liu XF, Liu XC, et al. Diagnostic accuracy of osteopontin for malignant pleural mesothelioma: a systematic review and meta-analysis. Clin Chim Acta 2014;433:44-8. [Crossref] [PubMed]
- Creaney J, Yeoman D, Musk AW, et al. Plasma versus serum levels of osteopontin and mesothelin in patients with malignant mesothelioma--which is best? Lung Cancer 2011;74:55-60. [Crossref] [PubMed]
- Cristaudo A, Foddis R, Bonotti A, et al. Comparison between plasma and serum osteopontin levels: usefulness in diagnosis of epithelial malignant pleural mesothelioma. Int J Biol Markers 2010;25:164-70. [PubMed]
- Cappia S, Righi L, Mirabelli D, et al. Prognostic role of osteopontin expression in malignant pleural mesothelioma. Am J Clin Pathol 2008;130:58-64. [Crossref] [PubMed]
- Cristaudo A, Bonotti A, Simonini S, et al. Combined serum mesothelin and plasma osteopontin measurements in malignant pleural mesothelioma. J Thorac Oncol 2011;6:1587-93. [Crossref] [PubMed]
- Myles T, Leung LL. Thrombin hydrolysis of human osteopontin is dependent on thrombin anion-binding exosites. J Biol Chem 2008;283:17789-96. [Crossref] [PubMed]
- Pei D, Li Y, Liu X, et al. Diagnostic and prognostic utilities of humoral fibulin-3 in malignant pleural mesothelioma: Evidence from a meta-analysis. Oncotarget 2017;8:13030-8. [PubMed]
- Pass HI, Levin SM, Harbut MR, et al. Fibulin-3 as a blood and effusion biomarker for pleural mesothelioma. N Engl J Med 2012;367:1417-27. [Crossref] [PubMed]
- Kirschner MB, Pulford E, Hoda MA, et al. Fibulin-3 levels in malignant pleural mesothelioma are associated with prognosis but not diagnosis. Br J Cancer 2015;113:963-9. [Crossref] [PubMed]
- Pass HI, Goparaju C. Fibulin-3 as a biomarker for pleural mesothelioma. N Engl J Med 2013;368:190. [PubMed]
- Scaffidi P, Misteli T, Bianchi ME. Release of chromatin protein HMGB1 by necrotic cells triggers inflammation. Nature 2002;418:191-5. [Crossref] [PubMed]
- Bianchi ME, Beltrame M, Paonessa G. Specific recognition of cruciform DNA by nuclear protein HMG1. Science 1989;243:1056-9. [Crossref] [PubMed]
- Lu B, Antoine DJ, Kwan K, et al. JAK/STAT1 signaling promotes HMGB1 hyperacetylation and nuclear translocation. Proc Natl Acad Sci U S A 2014;111:3068-73. [Crossref] [PubMed]
- Carneiro VC, de Moraes Maciel R, de Abreu da Silva IC, et al. The extracellular release of Schistosoma mansoni HMGB1 nuclear protein is mediated by acetylation. Biochem Biophys Res Commun 2009;390:1245-9. [Crossref] [PubMed]
- Jube S, Rivera ZS, Bianchi ME, et al. Cancer cell secretion of the DAMP protein HMGB1 supports progression in malignant mesothelioma. Cancer Res 2012;72:3290-301. [Crossref] [PubMed]
- Tabata C, Kanemura S, Tabata R, et al. Serum HMGB1 as a diagnostic marker for malignant peritoneal mesothelioma. J Clin Gastroenterol 2013;47:684-8. [Crossref] [PubMed]
- Ying S, Jiang Z, He X, et al. Serum HMGB1 as a Potential Biomarker for Patients with Asbestos-Related Diseases. Dis Markers 2017;2017:5756102.
- Wu T, Zhang W, Yang G, et al. HMGB1 overexpression as a prognostic factor for survival in cancer: a meta-analysis and systematic review. Oncotarget 2016;7:50417-27. [PubMed]
- Napolitano A, Antoine DJ, Pellegrini L, et al. HMGB1 and Its Hyperacetylated Isoform are Sensitive and Specific Serum Biomarkers to Detect Asbestos Exposure and to Identify Mesothelioma Patients. Clin Cancer Res 2016;22:3087-96. [Crossref] [PubMed]
- Ambros V. The functions of animal microRNAs. Nature 2004;431:350-5. [Crossref] [PubMed]
- Bartel DP. MicroRNAs: genomics, biogenesis, mechanism, and function. Cell 2004;116:281-97. [Crossref] [PubMed]
- Griffiths-Jones S. miRBase: the microRNA sequence database. Methods Mol Biol 2006;342:129-38. [PubMed]
- Reddy KB. MicroRNA (miRNA) in cancer. Cancer Cell Int 2015;15:38. [Crossref] [PubMed]
- Siebolts U, Varnholt H, Drebber U, et al. Tissues from routine pathology archives are suitable for microRNA analyses by quantitative PCR. J Clin Pathol 2009;62:84-8. [Crossref] [PubMed]
- Zhang K, Wu X, Wang J, et al. Circulating miRNA profile in esophageal adenocarcinoma. Am J Cancer Res 2016;6:2713-21. [PubMed]
- Sharova E, Grassi A, Marcer A, et al. A circulating miRNA assay as a first-line test for prostate cancer screening. Br J Cancer 2016;114:1362-6. [Crossref] [PubMed]
- Lamberti M, Capasso R, Lombardi A, et al. Two Different Serum MiRNA Signatures Correlate with the Clinical Outcome and Histological Subtype in Pleural Malignant Mesothelioma Patients. PLoS One 2015;10:e0135331. [Crossref] [PubMed]
- Weber DG, Johnen G, Bryk O, et al. Identification of miRNA-103 in the cellular fraction of human peripheral blood as a potential biomarker for malignant mesothelioma--a pilot study. PLoS One 2012;7:e30221. [Crossref] [PubMed]
- Kirschner MB, Cheng YY, Badrian B, et al. Increased circulating miR-625-3p: a potential biomarker for patients with malignant pleural mesothelioma. J Thorac Oncol 2012;7:1184-91. [Crossref] [PubMed]
- Busacca S, Germano S, De Cecco L, et al. MicroRNA signature of malignant mesothelioma with potential diagnostic and prognostic implications. Am J Respir Cell Mol Biol 2010;42:312-9. [Crossref] [PubMed]
- Guled M, Lahti L, Lindholm PM, et al. CDKN2A, NF2, and JUN are dysregulated among other genes by miRNAs in malignant mesothelioma -A miRNA microarray analysis. Genes Chromosomes Cancer 2009;48:615-23. [Crossref] [PubMed]
- Xu Y, Zheng M, Merritt RE, et al. miR-1 induces growth arrest and apoptosis in malignant mesothelioma. Chest 2013;144:1632-43. [Crossref] [PubMed]
- Pass HI, Goparaju C, Ivanov S, et al. hsa-miR-29c* is linked to the prognosis of malignant pleural mesothelioma. Cancer Res 2010;70:1916-24. [Crossref] [PubMed]
- Ivanov SV, Goparaju CM, Lopez P, et al. Pro-tumorigenic effects of miR-31 loss in mesothelioma. J Biol Chem 2010;285:22809-17. [Crossref] [PubMed]
- Kirschner MB, Cheng YY, Armstrong NJ, et al. MiR-score: a novel 6-microRNA signature that predicts survival outcomes in patients with malignant pleural mesothelioma. Mol Oncol 2015;9:715-26. [Crossref] [PubMed]
- Bononi I, Comar M, Puozzo A, et al. Circulating microRNAs found dysregulated in ex-exposed asbestos workers and pleural mesothelioma patients as potential new biomarkers. Oncotarget 2016;7:82700-11. [PubMed]
- Santarelli L, Staffolani S, Strafella E, et al. Combined circulating epigenetic markers to improve mesothelin performance in the diagnosis of malignant mesothelioma. Lung Cancer 2015;90:457-64. [Crossref] [PubMed]
- Tomasetti M, Staffolani S, Nocchi L, et al. Clinical significance of circulating miR-126 quantification in malignant mesothelioma patients. Clin Biochem 2012;45:575-81. [Crossref] [PubMed]
- Borrebaeck CA. Precision diagnostics: moving towards protein biomarker signatures of clinical utility in cancer. Nat Rev Cancer 2017;17:199-204. [Crossref] [PubMed]
- Giusti L, Da Valle Y, Bonotti A, et al. Comparative proteomic analysis of malignant pleural mesothelioma evidences an altered expression of nuclear lamin and filament-related proteins. Proteomics Clin Appl 2014;8:258-68. [Crossref] [PubMed]
- Ostroff RM, Mehan MR, Stewart A, et al. Early detection of malignant pleural mesothelioma in asbestos-exposed individuals with a noninvasive proteomics-based surveillance tool. PLoS One 2012;7:e46091. [Crossref] [PubMed]
- Gold L, Ayers D, Bertino J, et al. Aptamer-based multiplexed proteomic technology for biomarker discovery. PLoS One 2010;5:e15004. [Crossref] [PubMed]
- Bonotti A, Foddis R, Landi S, et al. A Novel Panel of Serum Biomarkers for MPM Diagnosis. Dis Markers 2017;2017:3510984.
- Cerciello F, Choi M, Nicastri A, et al. Identification of a seven glycopeptide signature for malignant pleural mesothelioma in human serum by selected reaction monitoring. Clin Proteomics 2013;10:16. [Crossref] [PubMed]
- Greening DW, Ji H, Chen M, et al. Secreted primary human malignant mesothelioma exosome signature reflects oncogenic cargo. Sci Rep 2016;6:32643. [Crossref] [PubMed]
- Micolucci L, Akhtar MM, Olivieri F, et al. Diagnostic value of microRNAs in asbestos exposure and malignant mesothelioma: systematic review and qualitative meta-analysis. Oncotarget 2016;7:58606-37. [PubMed]