Drug development against the hippo pathway in mesothelioma
Introduction
Malignant pleural mesothelioma (MPM) is a deadly disease with a poor prognosis that remains a treatment challenge. The diffuse nature of malignant cells throughout the entire pleural cavity preclude a complete surgical resection and therefore developing successful treatments to eradicate all microscopic sites of disease is the only way by which we can hope to achieve long-term survival. However, MPM is notoriously resistant to conventional cytotoxic chemotherapy regimens. Surveillance, epidemiology, and end results (SEER) medicare data show that medicare patients treated with only first-line chemotherapy of carboplatin or cisplatin plus pemetrexed have a median survival of only 7 months (1).
Drug development challenges
In the last 20 years there has been little progress to develop effective new therapies or dramatically improve survival. Countless failed clinical trials for novel chemotherapy agents against mesothelioma have floundered with bleak overall survival times and few patients responding to therapy. A review of British phase I MPM clinical trials between 2003 and 2015 had an 8-month median survival time with only 6% of patients having RECIST partial responses to therapy and 40% having RECIST stable disease for more than 3 months (2). The Mesothelioma Avastin Cisplatin Pemetrexed Study (MAPS) trial represented the first true clinical benefit in many years when it demonstrated a survival benefit of adding bevacizumab to cisplatin and pemetrexed with median survival times of 18.8 months among patients treated with the triple drug regimen versus 16.1 in patients treated with cisplatin and pemetrexed alone (3). Most recently, the explosion of immunotherapy has dramatically reshaped the oncology landscape for all cancers including MPM. Preliminary abstract data in MPM has been promising for some patients and immunotherapy has become an area of intense interest.
Importance of cancer stem cell pathways
Cancer stem cells are defined by their properties of self-renewal, pluripotency, a high proliferative capacity and the ability to resist standard chemotherapy and radiation. Cancer stem cell subpopulations having been identified in MPM using multiple cancer stem cell markers including aldehyde dehydrogenase (ALDH) and CD44 and by an OCT4/SOX2 reporter approach. These cancer stem cell subpopulations of cells have been shown to be resistant to cisplatin in multiple MPM cell lines and these cancer stem cells have been shown to be more tumor-initiating in xenograft mouse models (4,5). The presence of cancer stem cells and the stem cell like behavior of MPM account for some of the difficulty in treating MPM and blocking these stem cell pathways is a critical component of future successful MPM treatments.
Preclinical work is underway in several of these pathways including the Sonic Hedgehog (SHH) pathway where is has been shown rate models that vismodegib, a smoothened inhibitor approved in basal cell cancer, impairs MPM growth. In a rat xenograft model, vismodegib has been shown to downregulate SHH target genes of Gli1 and Ptch1 as well as reducing tumor volume and tumor growth (6). Combining stem cell pathway inhibitors with standard chemotherapy can improve the efficacy of chemotherapy, for example in the Wnt stem cell pathway combining cisplatin with Wnt pathway inhibitors in vitro induces synergistic cell cycle arrest and colony formation (7).
Focal adhesion kinase (Fak) is critical for cancer stem cell survival and maintenance and the molecule Fak inhibitor defactinib or VS-6063 showed promising results in preclinical studies. Fak is overexpressed in epithelial and mesenchymal tumors and regulates cell adhesion, proliferation, migration and survival. There was much anticipation of Fak as a drug target in MPM because cells with Merlin deficiency, commonly lost in mesothelioma, are very sensitive to Fak inhibition (8). Also encouraging was that cancer stem cell enriched MPM cell subpopulations have been shown to be more sensitive to defactinib in vitro (4). Fak signaling is associated with resistance to cytotoxic chemotherapy and Fak inhibition also enhances cancer cell sensitivity to taxanes in vitro (9). However a phase II clinical trial of defactinib in mesothelioma was ended in late 2015 after interim analysis failed to show any benefit. There may be a future for clinical testing of Fak inhibitors with the addition of predictive biomarkers, such as Merlin deficiency, to better identify MPM patients who will respond to Fak inhibition (10). Targeting cancer stem cell pathways remains an important area of active research.
The hippo pathway and mesothelioma
The Hippo pathway is a highly conserved regulator of organ size and of stem cell proliferation and maintenance (11). Only a small handful of genes are frequently mutated in MPM and many of these gene are in Hippo pathway suggesting that Hippo plays a critically important role in the development and growth of MPM (12). One of the most frequently mutated genes in MPM is Neurofibromatosis type 2 (NF2) tumor suppressor, located at chromosome 22q12, and is detected in 40% to 50% of MPM tumors (12,13). Large tumor suppressor homolog 2 (LAST2) gene, which is located at chromosome 13q12, is another frequently inactivated gene that is detected in 13% of MPM tumors (12,14). Inactivation of NF2 and LATS2 by deletion and/or mutation often contributes to dysregulation of Hippo pathway (15). In addition to LATS2, its closest gene family member LATS1 another Hippo pathway gene, has also recently been identified to be dysregulated in MPM, though less commonly than LATS2. LATS1 is located on chromosome 6 and changes in copy number variation and fusions to Presenilin 1 (PSEN1) on chromosome 14 have been observed in MPM (12,16). Mammalian sterile-20 like kinase 1 (MST1) is an important upstream kinase in the Hippo pathway that has also been found to be dysregulated in MPM (16). The largest and most comprehensive genomic analysis to date of transcriptomes, whole exomes and targeted exomes from 216 MPM samples found Hippo pathway signaling to be the number one most significantly mutated pathway in mesothelioma with a Q-value of 1.70E-17, driven by mutations, copy number variations and fusions in NF2, LATS1, LATS2 and MST1 (Figure 1).
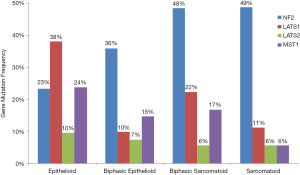
The Hippo pathway controls organ size primarily by regulating cell contact inhibition (17). In normal cells, Merlin, a protein encoded by NF2, and LATS2 contribute to the phosphorylation of the transcription factor Yes-associated protein (YAP) at S127 (18), resulting in YAP ubiquitination and activation of Hippo pathway to control cell proliferation. In MPM tumor cells, inactivation of NF2 and LATS2 prevent the phosphorylation of YAP at S127, which results in YAP relocation from the cytosol to nucleus where it interacts with TEA domain transcription factors (TEAD). In addition, constitutively activation of YAP has been identified in over 70% of primary MPM tumors (12,15,19), and YAP activation leads to Hippo signaling attenuation and transcription of downstream target genes, such as connective tissue growth factor (CTGF) and Cyr61 (17). Low Merlin expression (NF2), results in YAP1 activation, and has been shown to be associated with worse clinical outcomes with shorter times to recurrence and shorter overall survival times in patients with MPM (20). Blocking YAP activation therefore may be an important novel drug target.
Upstream signals that regulate YAP activation
There are multiple upstream signals and pathways which regulate the Hippo pathway activity that might be exploited as novel therapeutic targets to inhibit YAP in MPM (Figure 2). These include specific G-protein coupled receptors (GPCR), Rho kinase (ROCK), the epidermal growth factor receptor (EGFR) pathway, direct interruption of YAP-TEAD mediated transcriptional activity, cyclin-dependant kinase 1 (CKD1) and cyclin-dependant kinase 9 (CKD9).
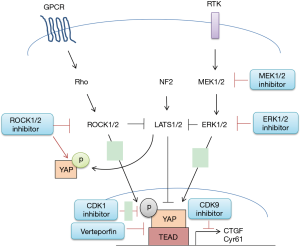
Gα12/13, Gαq11, Gαs and GPCR signaling
GPCRs function as transducers, by using heterotrimeric G proteins, to activate internal signal transduction pathways in response to specific extracellular signals. There are thousands of tissue specific GPCR heterodimers, that couple to fifteen different Gα proteins. Recent studies have highlighted the capability of GPCR signaling to regulate the inhibition or activation of the Hippo pathway depending on the grouped Gα proteins. For instance, activating Gα12/13 or Gαq11 coupled receptors by serum-borne lysophosphatidic acid (LPA), sphingosine 1-phosphophate (S1P), thrombin and protease-activated receptor (PAR) agonist peptides resulted in inhibition of LATS1/2 kinases and activation of YAP (21-23). Conversely, activating Gαs coupled receptors by adrenaline, glucagon and dihydrexidine turns on the cAMP-dependent protein kinase A (PKA) signaling pathway and promotes LAST1/2 activity, resulted in YAP suppression (24,25). Therapeutic approaches targeting GPCR to attenuate YAP/Hippo pathway in cancers have been evaluated in clinic trials, including S1P-blocking antibody Sphingomab and Phosphatase-resistant LPA analog (26,27). In pre-clinical studies, G protein-coupled β-adrenergic receptor agonist dobutamine has been proposed as anti-cancer treatments (28). In MPM cells, LPA has been shown to stimulate YAP activation and promote cell proliferation (29), and PAR1 expression has been shown to contribute to MPM tumor growth in vivo (30). These studies suggest that targeting GPCR, specifically by activating Gαs or inhibiting Gα12/13 or Gαq11 coupled receptors to suppress YAP could be an effective therapeutic approach in human mesothelioma.
ROCK
The YAP/Hippo pathway is involved in contact inhibition and mechanotransduction in MPM cells. Cellular components of adherens and tight junctions have been reported to inhibit YAP nuclear accumulation (18,31). It has also been demonstrated that F-actin promotes YAP nuclear translocation and inhibits the Hippo pathway, and that destabilization of F-actin results in the nuclear exportation of YAP (32,33). The clinical use of anti-cytoskeletal therapies has been limited due to toxicity (34). Current therapeutic approaches to target mechanotransduction signals in cancer work through inhibiting Rho-associated kinase (ROCK) signaling (34,35). ROCK inhibition was recently reported to suppress YAP activity in MPM cells harboring LAST2 mutation, suggesting that ROCK could regulate the Hippo pathway through a LATS2-independent mechanism (36). These findings also suggest that targeting ROCK could be an effective therapeutic strategy in MPM.
EGFR
A direct link between EGFR signaling and YAP has been reported. Studies have shown that YAP regulates EGF-mediated cell migration and promotes transcription of EGF-like growth factor AREG (37-39). In addition, inhibition of MAPK/ERK Kinase (MEK1/2) or extracellular signal-regulated kinases (ERK1/2) accelerates YAP degradation, reduces transcriptional activities of downstream genes, and decreases the abilities of migration and invasion in non-small cell lung cancer (NSCLC) cells (40). These studies suggest that targeting EGFR signaling could effectively inhibit YAP activation in cancer cells; however, YAP activation can also regulate drug resistance to EGFR inhibitor erlotinib in NSCLC (41), implying the complexity of YAP regulatory mechanisms in human cancer.
Direct interruption of YAP-TEAD mediated transcriptional activity VP
One way to disrupt YAP transcriptional mechanisms is to block the interaction of YAP to TEAD transcription factors. Two small molecule inhibitors that block the YAP-TEAD interaction were identified from John Hopkins Drug Library by drug screening (42). Both Verteporfin (VP, trade name Visudyne by Novartis) and protoporphyrin IX (PPIX) inhibit the YAP-TEAD complex formation at 10 µM in Co-immunoprecipitation assays (43). VP is a FDA-approved photosensitizer in the photodynamic therapy of neovascular macular degeneration; however, when used as an inhibitor of the YAP-TEAD interaction, light activation is not required (43,44). VP alters YAP conformation when binding to it, and abolished its interaction to TEAD (45). In addition, VP was shown to inhibit the oncogenic activity of YAP in vivo and reduced cell growth and viability in various human malignancies (42,46-48). Moreover, VP treatment has been shown to inhibit cell proliferation, viability, and invasion of MPM cells (36,49).
Cyclin-dependent kinase 1
Posttranslational modification of YAP during mitosis and the mechanism that controls YAP phosphorylation at different locations have been an area of interest (50-52). In eukaryotic cells, the cell cycle is controlled by cyclin dependent kinases (CDKs), and CDK1 was shown to phosphorylate YAP at multiple sites during the G2/M phase of the cell cycle (50-52). YAP phosphorylation at T119 and S289 by CDK1 during G2/M phase was shown to enhance cell migration and invasion of immortal cell lines (52); however, YAP phosphorylation at S128 by CDK1 during anti-tubulin treatment was shown to induce cancer cell death (53). The physiological outcome of CDK1 induced YAP phosphorylation highlighted the complexity mechanisms of CDK1 induced YAP phosphorylation in cancer cells.
Cyclin-dependent kinase 9 (CDK9)
The interaction of YAP and TEAD recruits a transcriptional mediator complex and promotes elongation of downstream mRNA molecules (54). YAP has been shown to promote acetylation of histones located in enhancers and depletion of YAP reduces recruitment of the mediator complex and promoter-proximal polymerase II levels in cells (54). In addition, phosphorylation of CDK9, a key component of transcription mediators and the elongation complex, promotes transcriptional activation driven by YAP (54). CDK9 inhibitors including flavopiridol, dinaciclib, seliciclib, SNS-032 and RGB-286638 have been evaluated in clinical studies (55,56). It has recently been reported in MPM studies in vitro, that CDK9 inhibition reduces cell proliferation and viability (36).
Conclusions
The Hippo pathway has proven to be of critical importance in MPM. Mutations along this pathway, specifically in NF2 and LATS lead to YAP activation in the majority of MPM tumors. Blocking YAP activity, either via upstream inhibition of one of the several pathways that regulate YAP and Hippo or via direct YAP/TEAD inhibition is an area of active interest. Several novel small molecule YAP inhibitors are in preclinical development with promising results and may enter clinical trials in the near future.
Acknowledgements
None.
Footnote
Conflicts of Interest: The authors have no conflicts of interest to declare.
References
- Beebe-Dimmer JL, Fryzek JP, Yee CL, et al. Mesothelioma in the United States: a Surveillance, Epidemiology, and End Results (SEER)-Medicare investigation of treatment patterns and overall survival. Clin Epidemiol 2016;8:743-50. [Crossref] [PubMed]
- Papadatos-Pastos D, Roda D, De Miguel Luken MJ, et al. Clinical outcomes and prognostic factors of patients with advanced mesothelioma treated in a phase I clinical trials unit. Eur J Cancer 2017;75:56-62. [Crossref] [PubMed]
- Zalcman G, Mazieres J, Margery J, et al. Bevacizumab for newly diagnosed pleural mesothelioma in the Mesothelioma Avastin Cisplatin Pemetrexed Study (MAPS): a randomised, controlled, open-label, phase 3 trial. Lancet 2016;387:1405-14. [Crossref] [PubMed]
- Blum W, Pecze L, Felley-Bosco E, et al. Stem Cell Factor-Based Identification and Functional Properties of In Vitro-Selected Subpopulations of Malignant Mesothelioma Cells. Stem Cell Reports 2017;8:1005-17. [Crossref] [PubMed]
- Cortes-Dericks L, Froment L, Boesch R, et al. Cisplatin-resistant cells in malignant pleural mesothelioma cell lines show ALDH(high)CD44(+) phenotype and sphere-forming capacity. BMC Cancer 2014;14:304. [Crossref] [PubMed]
- Meerang M, Bérard K, Felley-Bosco E, et al. Antagonizing the Hedgehog Pathway with Vismodegib Impairs Malignant Pleural Mesothelioma Growth In Vivo by Affecting Stroma. Mol Cancer Ther 2016;15:1095-105. [Crossref] [PubMed]
- Uematsu K, Seki N, Seto T, et al. Targeting the Wnt signaling pathway with dishevelled and cisplatin synergistically suppresses mesothelioma cell growth. Anticancer Res 2007;27:4239-42. [PubMed]
- Shapiro IM, Kolev VN, Vidal CM, et al. Merlin deficiency predicts FAK inhibitor sensitivity: a synthetic lethal relationship. Sci Transl Med 2014;6:237ra68. [Crossref] [PubMed]
- Sulzmaier FJ, Jean C, Schlaepfer DD. FAK in cancer: mechanistic findings and clinical applications. Nat Rev Cancer 2014;14:598-610. [Crossref] [PubMed]
- Lee BY, Timpson P, Horvath LG, et al. FAK signaling in human cancer as a target for therapeutics. Pharmacol Ther 2015;146:132-49. [Crossref] [PubMed]
- Ramos A, Camargo FD. The Hippo signaling pathway and stem cell biology. Trends Cell Biol 2012;22:339-46. [Crossref] [PubMed]
- Miyanaga A, Masuda M, Tsuta K, et al. Hippo pathway gene mutations in malignant mesothelioma: revealed by RNA and targeted exon sequencing. J Thorac Oncol 2015;10:844-51. [Crossref] [PubMed]
- Sekido Y. Molecular pathogenesis of malignant mesothelioma. Carcinogenesis 2013;34:1413-9. [Crossref] [PubMed]
- Murakami H, Mizuno T, Taniguchi T, et al. LATS2 is a tumor suppressor gene of malignant mesothelioma. Cancer Res 2011;71:873-83. [Crossref] [PubMed]
- Felley-Bosco E, Stahel R. Hippo/YAP pathway for targeted therapy. Transl Lung Cancer Res 2014;3:75-83. [PubMed]
- Bueno R, Stawiski EW, Goldstein LD, et al. Comprehensive genomic analysis of malignant pleural mesothelioma identifies recurrent mutations, gene fusions and splicing alterations. Nat Genet 2016;48:407-16. [Crossref] [PubMed]
- Harvey KF, Zhang X, Thomas DM. The Hippo pathway and human cancer. Nat Rev Cancer 2013;13:246-57. [Crossref] [PubMed]
- Zhao B, Wei X, Li W, et al. Inactivation of YAP oncoprotein by the Hippo pathway is involved in cell contact inhibition and tissue growth control. Genes Dev 2007;21:2747-61. [Crossref] [PubMed]
- Wang Y, Dong Q, Zhang Q, et al. Overexpression of yes-associated protein contributes to progression and poor prognosis of non-small-cell lung cancer. Cancer Sci 2010;101:1279-85. [Crossref] [PubMed]
- Meerang M, Bérard K, Friess M, et al. Low Merlin expression and high Survivin labeling index are indicators for poor prognosis in patients with malignant pleural mesothelioma. Mol Oncol 2016;10:1255-65. [Crossref] [PubMed]
- Mo JS, Yu FX, Gong R, et al. Regulation of the Hippo-YAP pathway by protease-activated receptors (PARs). Genes Dev 2012;26:2138-43. [Crossref] [PubMed]
- Yu FX, Zhao B, Panupinthu N, et al. Regulation of the Hippo-YAP pathway by G-protein-coupled receptor signaling. Cell 2012;150:780-91. [Crossref] [PubMed]
- Miller E, Yang J, DeRan M, et al. Identification of serum-derived sphingosine-1-phosphate as a small molecule regulator of YAP. Chem Biol 2012;19:955-62. [Crossref] [PubMed]
- Kim M, Kim M, Lee S, et al. cAMP/PKA signalling reinforces the LATS-YAP pathway to fully suppress YAP in response to actin cytoskeletal changes. EMBO J 2013;32:1543-55. [Crossref] [PubMed]
- Zhang Y, He J, Zhang F, et al. SMO expression level correlates with overall survival in patients with malignant pleural mesothelioma. J Exp Clin Cancer Res 2013;32:7. [Crossref] [PubMed]
- Fleming JK, Wojciak JM, Campbell MA, et al. Biochemical and structural characterization of lysophosphatidic Acid binding by a humanized monoclonal antibody. J Mol Biol 2011;408:462-76. [Crossref] [PubMed]
- Ponnusamy S, Selvam SP, Mehrotra S, et al. Communication between host organism and cancer cells is transduced by systemic sphingosine kinase 1/sphingosine 1-phosphate signalling to regulate tumour metastasis. EMBO Mol Med 2012;4:761-75. [Crossref] [PubMed]
- Bao Y, Nakagawa K, Yang Z, et al. A cell-based assay to screen stimulators of the Hippo pathway reveals the inhibitory effect of dobutamine on the YAP-dependent gene transcription. J Biochem 2011;150:199-208. [Crossref] [PubMed]
- Yamada T, Yano S, Ogino H, et al. Lysophosphatidic acid stimulates the proliferation and motility of malignant pleural mesothelioma cells through lysophosphatidic acid receptors, LPA1 and LPA2. Cancer Sci 2008;99:1603-10. [Crossref] [PubMed]
- Keshava S, Sahoo S, Tucker TA, et al. Endothelial cell protein C receptor opposes mesothelioma growth driven by tissue factor. Cancer Res 2013;73:3963-73. [Crossref] [PubMed]
- Gumbiner BM, Kim NG. The Hippo-YAP signaling pathway and contact inhibition of growth. J Cell Sci 2014;127:709-17. [Crossref] [PubMed]
- Gaspar P, Tapon N. Sensing the local environment: actin architecture and Hippo signalling. Curr Opin Cell Biol 2014;31:74-83. [Crossref] [PubMed]
- Kono K, Tamashiro DA, Alarcon VB. Inhibition of RHO-ROCK signaling enhances ICM and suppresses TE characteristics through activation of Hippo signaling in the mouse blastocyst. Dev Biol 2014;394:142-55. [Crossref] [PubMed]
- Zanconato F, Battilana G, Cordenonsi M, et al. YAP/TAZ as therapeutic targets in cancer. Curr Opin Pharmacol 2016;29:26-33. [Crossref] [PubMed]
- Chin VT, Nagrial AM, Chou A, et al. Rho-associated kinase signalling and the cancer microenvironment: novel biological implications and therapeutic opportunities. Expert Rev Mol Med 2015;17:e17. [Crossref] [PubMed]
- Zhang WQ, Dai YY, Hsu PC, et al. Targeting YAP in malignant pleural mesothelioma. J Cell Mol Med 2017. [Epub ahead of print]. [Crossref] [PubMed]
- Fan R, Kim NG, Gumbiner BM. Regulation of Hippo pathway by mitogenic growth factors via phosphoinositide 3-kinase and phosphoinositide-dependent kinase-1. Proc Natl Acad Sci U S A 2013;110:2569-74. [Crossref] [PubMed]
- Reddy BV, Irvine KD. Regulation of Hippo signaling by EGFR-MAPK signaling through Ajuba family proteins. Dev Cell 2013;24:459-71. [Crossref] [PubMed]
- Zhang J, Ji JY, Yu M, et al. YAP-dependent induction of amphiregulin identifies a non-cell-autonomous component of the Hippo pathway. Nat Cell Biol 2009;11:1444-50. [Crossref] [PubMed]
- You B, Yang YL, Xu Z, et al. Inhibition of ERK1/2 down-regulates the Hippo/YAP signaling pathway in human NSCLC cells. Oncotarget 2015;6:4357-68. [Crossref] [PubMed]
- Hsu PC, You B, Yang YL, et al. YAP promotes erlotinib resistance in human non-small cell lung cancer cells. Oncotarget 2016;7:51922-33. [PubMed]
- Liu-Chittenden Y, Huang B, Shim JS, et al. Genetic and pharmacological disruption of the TEAD-YAP complex suppresses the oncogenic activity of YAP. Genes Dev 2012;26:1300-5. [Crossref] [PubMed]
- Santucci M, Vignudelli T, Ferrari S, et al. The Hippo Pathway and YAP/TAZ-TEAD Protein-Protein Interaction as Targets for Regenerative Medicine and Cancer Treatment. J Med Chem 2015;58:4857-73. [Crossref] [PubMed]
- Brodowska K, Al-Moujahed A, Marmalidou A, et al. The clinically used photosensitizer Verteporfin (VP) inhibits YAP-TEAD and human retinoblastoma cell growth in vitro without light activation. Exp Eye Res 2014;124:67-73. [Crossref] [PubMed]
- Johnson R, Halder G. The two faces of Hippo: targeting the Hippo pathway for regenerative medicine and cancer treatment. Nat Rev Drug Discov 2014;13:63-79. [Crossref] [PubMed]
- Yu FX, Luo J, Mo JS, et al. Mutant Gq/11 promote uveal melanoma tumorigenesis by activating YAP. Cancer Cell 2014;25:822-30. [Crossref] [PubMed]
- Nguyen LT, Tretiakova MS, Silvis MR, et al. ERG Activates the YAP1 Transcriptional Program and Induces the Development of Age-Related Prostate Tumors. Cancer Cell 2015;27:797-808. [Crossref] [PubMed]
- Feng J, Gou J, Jia J, et al. Verteporfin, a suppressor of YAP-TEAD complex, presents promising antitumor properties on ovarian cancer. Onco Targets Ther 2016;9:5371-81. [Crossref] [PubMed]
- Tranchant R, Quetel L, Tallet A, et al. Co-occurring Mutations of Tumor Suppressor Genes, LATS2 and NF2, in Malignant Pleural Mesothelioma. Clin Cancer Res 2017;23:3191-202. [Crossref] [PubMed]
- Bui DA, Lee W, White AE, et al. Cytokinesis involves a nontranscriptional function of the Hippo pathway effector YAP. Sci Signal 2016;9:ra23. [Crossref] [PubMed]
- Meng Z, Moroishi T, Guan KL. Mechanisms of Hippo pathway regulation. Genes Dev 2016;30:1-17. [Crossref] [PubMed]
- Yang S, Zhang L, Liu M, et al. CDK1 phosphorylation of YAP promotes mitotic defects and cell motility and is essential for neoplastic transformation. Cancer Res 2013;73:6722-33. [Crossref] [PubMed]
- Zhao Y, Khanal P, Savage P, et al. YAP-induced resistance of cancer cells to antitubulin drugs is modulated by a Hippo-independent pathway. Cancer Res 2014;74:4493-503. [Crossref] [PubMed]
- Galli GG, Carrara M, Yuan WC, et al. YAP Drives Growth by Controlling Transcriptional Pause Release from Dynamic Enhancers. Mol Cell 2015;60:328-37. [Crossref] [PubMed]
- Nowicki MW, Walkinshaw MD. CDK9 inhibitors push cancer cells over the edge. Chem Biol 2010;17:1047-8. [Crossref] [PubMed]
- Morales F, Giordano A. Overview of CDK9 as a target in cancer research. Cell Cycle 2016;15:519-27. [Crossref] [PubMed]