Interventional pulmonology approaches in the diagnosis and treatment of early stage non small cell lung cancer
Background of interventional pulmonology (IP)
At the dawn of the 20th century, respiratory physicians largely provided medical care in sanatoriums tending to patients infected with tuberculosis. TB was the second leading cause of death in that era behind pneumonias and influenza infections (1,2). Advances in antimicrobials, including isoniazid and penicillin, led to significant improvement in outcomes. During the subsequent decades, tobacco smoking became widespread in the United States. It was not until the mid-20th century that lung cancer was strongly linked to smoking with epidemiological data (3). Since then, pulmonologists have managed the myriad of lung ailments consequent to tobacco addiction, such as emphysema. As lung cancer became the leading cause of cancer deaths in both men and women, the pulmonologist’s role in lung cancer care has evolved. Smoking cessation remains the most important role a pulmonologist assumes to prevent lung cancers; as one fifth of U.S. population are smokers, and among the economically disadvantaged, the number increases to about forty percent (4). On the other hand, the management role of pulmonologists in lung cancer has also evolved from diagnosis of late stage lung cancers and risk stratification for surgery, to management of early stage disease with personalized approach in a multidisciplinary setting.
Interventional pulmonology concentrates on the use and development of diagnostic and therapeutic endobronchial techniques (5-7). The IP armatorium consists of (and is not limited to) rigid bronchoscopy, endobronchial laser therapy, electrocautery, cryotherapy brachytherapy and endobronchial or tracheal stent placement and the advanced diagnostic techniques available to pulmonologists such as endobronchial ultrasound (EBUS) and navigational bronchoscopy (NB) (Table 1).
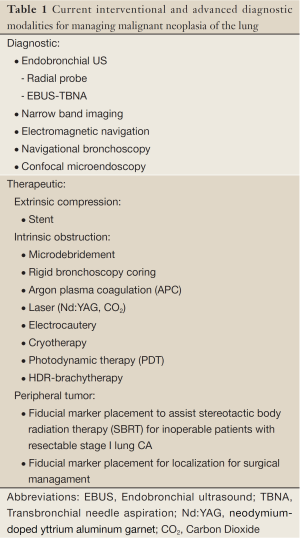
Full Table
It is important to bear in mind that the field of IP depends on close-working and complementary relationships with the thoracic radiologist, radiation oncologist, and the thoracic surgeon as part of a multidisciplinary team. This review will cover diagnostic and therapeutic techniques that are being used in the management of early stage lung cancer.
Bronchoscopic early detection of malignancy
Lung cancer is the most lethal of solid tumors. Up to 85% are attributed largely to heavy smoking. Furthermore, despite smoking cessation many are still at risk for several years since their last cigarette (8). In the last decade evidence in favor of lung cancer screening with low-dose CT (LDCT) has been shown to be superior to CXR (8).
Henscke et al. demonstrated in a prospective observational study of 31,567 asymptomatic patients, low dose CT screening resulted in the diagnosis of lung cancer in 484 patients, 85% of whom had Stage I disease, and who after treatment had a 10-year survival rate of 88% (95% CI, 88-95%) (9). The National Cancer Institute-Sponsored Lung Screening Trial that followed supported its findings. The NSLT was a randomized control trial in which 53,454 patients were randomized to three years of annual low dose CT screening versus plain chest X-ray (8). After three years the NSLT investigators had achieved their primary objective, which was a 20% relative reduction in mortality from lung cancer. The corresponding number needed to screen (NNS) to prevent 1 death after 1 year of screening is 320. Although questions remain about trial design, generalizability, applicability and cost-effectiveness of LDCT in the community, the goal of detecting early stage lung cancer with concomitant reduction in cancer specific mortality has become achievable.
As a result of NLST, the U.S. Preventive Services Task Force has recently updated its original 2004 recommendations regarding lung cancer screening. Its main recommendation, based on the NLST, provides LDCT screening to high risk individuals with at least a 30 pack-year smoking history between the ages of 55-79 years. The prospective efficacy of these recommendations will depend on the ability of the pulmonologist to meet the demands of accurate and timely diagnosis, proper patients risk stratifications, tissue sampling when appropriate, and familiarity thoracic oncologists’ needs beyond tumor types, i.e. molecular profiling
Early detection of malignancies arising from the central airways by bronchoscopy has also been evaluated over the past decade. Squamous cell carcinomas of the central airways have shown to develop through several stages from metaplasia, dysplasia, carcinoma in-situ (CIS) and advanced invasion (10). The cellular transformation of bronchial carcinomas has been described as a spectrum of lesions from basal layer hyperplasia, metaplasia, dysplasia, and CIS (11). CIS is characterized by involvement of the entire epithelium with marked cytologic atypia. Bronchoscopic follow-up by autofluorescence bronchoscopy (AFB) and biopsy data among patients with high suspicion for lung cancer either from positive sputum cytology or prior upper respiratory cancers has shown that severe dysplastic lesions were more likely to progress towards CIS and further invasive cancer (12,13). Furthermore, Bota et al. showed 75% of CIS lesions, which persisted at 3 months required therapy (13). Currently the American College of Chest Physicians (ACCP) recommendations regarding known CIS and high-grade dysplastic lesions suggest performing follow-up white-light bronchoscopy (WLB) to rule out endobronchial lesions with use of AFB if available (14). However, relatively higher false-positive rates and suboptimal specificity of AFB, likely due to failure to distinguish inflammatory airways from dysplasia, limit its potential to preclude the need for unnecessary biopsies (15).
Narrow band imaging (NBI)
NBI utilizes narrow wavelengths of blue (400-430 & 420-470 nm; B1 & B2, respectively) and green (560-590 nm) light to enhance visualization of abnormal collections of submucosal capillaries (16,17). This strategy has been shown to be highly effective in differentiating normal mucosa from highly vascular precancerous lesions such as angiogenic squamous dysplasia (ASD). ASD is characterized by abnormal collections of microvessels projecting into dysplastic cells within the bronchial mucosa (18). NBI has shown improved sensitivity in detecting ASD not readily seen with WLB or AFB (19-21). Diagnostic yield was similar between NBI and AFB without any increased false-positives suggesting that NBI may serve as an alternative tool in early lung cancer detection. NBI’s unique ability to detect early angiogenesis undetectable by AFB with high specificity (85-90%) and negative predictive value (>90%) has the potential for influencing therapeutic decision-making (22). Currently, the ACCP recommends NBI or AFB, when available, be used to delineate tumor margins in patients who are candidates for early lung cancer resection (23).
Lung cancer diagnosis and staging
The solitary pulmonary nodule (SPN)
The solitary pulmonary nodule, commonly detected incidentally, is among the top reasons for referral to a pulmonologist. Concurrently, as the work on early lung cancer screening evolves, it behooves the pulmonary specialist to become an expert in the management of the solitary lung nodule.
The current ACCP guidelines recommend, depending on patient and SPN features, observation, tissue biopsy and direct surgical excision. The role for pulmonologists for biopsies will be expanding with advancing bronchoscopic techniques. Currently, with more peripheral lesions where tissue biopsy is indicated, the guidelines recommend trans-thoracic needle biopsy (TTNB) as the preferred modality as the diagnostic yield is slightly below 90% (23-25). Traditional transbronchial biopsy with bronchoscopy has a diagnostic yield of only 14-63% (26). In 2012, Wang et al. published a meta-analysis of 39 pooled studies (n=patients >3,000) of all available guided-techniques (discussed below) that demonstrated a pooled diagnostic yield of 70% (25). While this is improved compared to standard bronchoscopy, it still remains below the diagnostic yield of TTNB. Concurrently, Wang reported a pneumothorax rate in TTNB of 25% (15% requiring a chest tube) versus less than 2% (less than 1% requiring a chest tube) in bronchoscopic techniques. The three main techniques used in this field of specialized bronchoscopy are radial probe-EBUS (RP-EBUS), virtual bronchoscopic navigation (VBN) and electromagnetic navigational bronchoscopy (ENB™) (5).
Radial probe EBUS (RP-EBUS)
RP-EBUS uses ultrasound to take samples sequentially. It allows excellent visualization of the bronchial walls and a tumor in situ. It is also the only technology that allows for real-time confirmation that the target lesion has been reached which translates to improved yield over conventional transbronchial biopsy with or without fluoroscopy (27-31). In a prospective cohort study of 131 patients, RP-EBUS demonstrated better sensitivity and accuracy (89% and 100%, respectively) at detecting bronchial wall invasion over CT (75% and 51%, respectively) (32). RP-EBUS uses a flexible bronchoscope to access the bronchiole closest to the nodule, and then a miniaturized radial probe and sheath are passed through the working channel until the nodule is visualized. The probe is removed leaving the sheath in position, then biopsy forceps is inserted through the guide sheath and the nodule sampled (Figure 1). Steinfort et al. showed in a meta-analysis of 13 studies and 1,090 patients, that RP-EBUS in SPNs had a point specificity of 1.00 (95% CI, 0.99-1.0) and point sensitivity of 0.73 (95% CI, 0.70-0.76) (33). Eberthardt et al. in a randomized control trial showed that RP-EBUS with a guide sheath alone had a diagnostic yield of 69% (34). This increased to 88% when combined with electromagnetic navigational bronchoscopy (ENB™); a diagnostic yield comparable to TTNB and SPNs with a mean diameter of 25 mm.
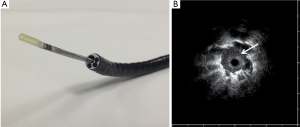
Electromagnetic navigational bronchoscopy (ENB™)
ENB™ is a technology developed for access to peripheral nodules beyond the reach of conventional bronchoscopes. Akin to global positioning systems (GPS), ENB™ is able to provide real-time orientation of a proprietary sensor probe by utilizing an electromagnetic field generated by a board underneath the patient (16,35). Pre-procedural planning involves importing the patient’s CT data and assigning anatomical landmarks including the target lesion. The sensor probe (1 mm diameter × 8 mm long) is loaded into a flexible catheter, and then passed through the working channel of a standard bronchoscope. Guidance is provided by a matched virtual bronchoscopy image aside the real-time video bronchoscopy overlain with pre-determined pathway markers. Once the bronchoscope is wedged into the segment of interest the flexible catheter with the sensor probe is advanced until the target lesion is reached. At this point the sensor probe is retracted leaving the flexible catheter in place to act as an extended working channel (Figure 2). The diagnostic yield of ENB™ alone is reported to range from 59-74% (36-38). While early studies postulated that target lesion size might be significant, recently it is believed that CT-body divergence (a measure of image data registration accuracy) may determine navigational success (36,38). Other factors related to local anatomy and distance influence overall success (39,40). The presence of a bronchus sign significantly improved success to 79% in series of 51 patients by Seijo and colleagues (39).
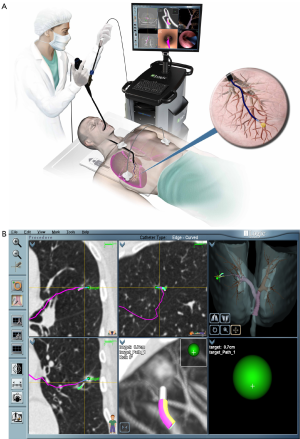
Recently, a randomized-controlled trial by Asano and colleagues demonstrated virtual bronchoscopy navigational guidance (VBN, a computerized guidance system without electromagnetic correlation) with an ultrathin scope significantly improves diagnostic yield in the right upper lobe, peripheral third and lesions invisible on chest X-ray (41). However, the main limitation of this technique is the lack of real-time confirmation that a nodule has been reached. Addition of radial EBUS has shown to overcome this by increasing diagnostic yield to 88-93% (42).
Lung cancer staging with convex-EBUS (EBUS-TBNA)
Staging and confirmation of nodal status is central to the diagnosis and management of non-small cell lung cancer (NSCLC) (Figure 3). The treatment of choice of stage I and II disease is surgical resection in operable candidates, whereas combined chemo and radiotherapy is indicated for patients with Stage III disease and above (43). In academic settings, a multimodality approach can be considered for functional IIIA patients to undergo surgical resection after neoadjuvant therapy (23). Mediastinal nodal metastases are detected non-invasively with CT and/or PET scanning followed by tissue sampling of any pathologic nodes. Depending on population prevalence, non-invasive imaging alone is inadequate as histological staging is obligatory to prognosticate and stratify management options (44,45). For example, in geographic regions where histoplasmosis infection is endemic, granulomatous infection can lead to PET-avidity in the mediastinum and lung lesions. Conversely, Altorki et al. conducted a retrospective review of 224 patients identified with clinical stage I NSCLC by CT and PET scan. At resection they found that 6.5% of clinical T1 patients had occult N2 disease (46).
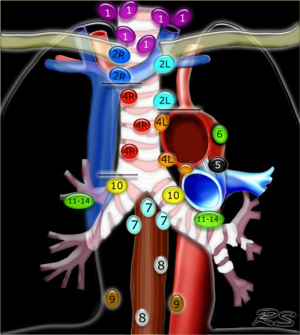
Surgical mediastinoscopy is the gold standard for confirming CT/PET negative mediastinal metastases with a sensitivity of 78%. However, it has some limitations. Convex EBUS is a bronchoscopic technique that compliments mediastinoscopy (23) (Table 2). EBUS is minimally invasive, performed under conscious sedation or with general anesthesia in the outpatient setting, Lymph node sampling occurs under direct real-time ultrasound guidance with the convex probe EBUS (CP-EBUS) allowing a much greater diagnostic yield over blind sampling (47,48) (Figure 4). In a prospective cohort study of 108 patients, CP-EBUS-TBNA successfully sampled 163 mediastinal lymph nodes and demonstrated sensitivity and specificity of 94.6% and 100%, respectively, and a diagnostic accuracy of 96% (49). Several systematic reviews confirm equivalent sensitivity for EBUS-TNA to Mediastinoscopy (23,50-52). Yasufuku performed a prospective controlled comparison of EBUS-TBNA and mediastinoscopy in 153 patients with potentially resectable NSCLC (prevalence of N2/N3 disease 35%). They found sensitivities for mediastinoscopy and EBUS-TBNA were 79% and 85%, respectively, with comparable specificity (100%) and no significant differences in detecting true pathological N stage (McNemar test P=0.78) (53). In most community hospitals surgical mediastinoscopy remains the only available mediastinal staging technique. Many of the aforementioned studies were conducted at tertiary referral centers and it is uncertain how generalizable the results are.
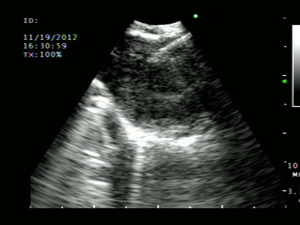
EBUS combined with EUS/ with mediastinoscopy
EBUS-TBNA has the ability to access most of the mediastinum (the anterior and superior), however the presence of paraesophageal, inferior and posterior mediastinal lymph nodes may require combined EBUS with endoscopic ultrasound guided fine needle aspiration (EUS-FNA) (Table 2). The combination improves the diagnostic yield compared to either procedure alone (48,54). Annema et al. in 2010 performed a multi-center randomized control trial in 241 patients with resectable NSCLC comparing mediastinoscopy alone with combined endosonography (EBUS-TBNA and/or EUS-FNA) approach followed by mediastinoscopy if no nodal metastases were found (55). The sensitivities of surgical staging compared to endosonography alone were 79% and 85%, respectively (P=0.47). Sensitivity improved to 94% if endosonography was followed by mediastinoscopy (P=0.02). This combined endosonographic and surgical approach resulted in greater sensitivity and fewer unnecessary thoracotomies.
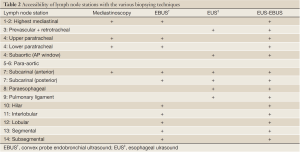
Full Table
Adequacy and techniques for molecular profiling
There have been dramatic advances in our understanding of the molecular makeup of NSCLCs, particularly in non-smokers or smokers with lower cumulative dose. Driver mutations in NSCLC that can be targeted have caused a shift away from a uniform therapeutic approach to a more personalized approach (56-60). In this era of personalized medicine, there is a need to provide high quality biopsy samples not only for pathologic diagnosis but also for the detailed molecular analyses that are becoming important to patient care. Initial studies in small populations suggested that EBUS-TBNA samples can be used for molecular analysis; EGFR, K-ras, p53 and EML4-ALK mutations (61,62). Navani et al. conducted a large multicenter study of 774 patients and confirmed these results. While the appropriate triaging of small biopsy specimens for cytologic, pathologic, and molecular analysis is vital there are as yet no guidelines for managing EBUS-TBNA samples. It is extremely important that the bronchoscopist obtaining samples do so in a manner that optimizes the diagnostic yield from molecular analyses (63,64). Rapid on-site cytologic evaluation (ROSE) of EBUS-TBNA has been shown to increase sensitivity from 80-88% without any added time to the procedure. Where available, ROSE allows repeated sampling of confirmed high-yield sites for triaging of specimens to cytologic diagnosis, immunohistochemisty or molecular analysis with clear communication of these goals to pathologist (61,65-68). In addition to ROSE, a few specialist centers are examining what additional procedural steps can be taken to maximize yield from small biopsy samples. This is crucial as more emerging genes are being identified that affect NSCLC carcinogenesis, such as ROS (crizotinib sensitive), Met, PI3K, etc. (61,64). As minimally invasive diagnosis and staging, as well as therapeutic modalities with driver mutations now becoming available there is increasing need to maximize and refine the technology and processes for tissue sampling (i.e., multiplex sampling, to afford our patients the best treatment options).
Re-biopsy
The European Respiratory Society (ERS) has published a statement that at biopsy it is desirable to obtain as much useful tissue as possible to avoid time consuming delays (dead time) due to molecular analysis or having to re-biopsy (69) (Figure 5). In order to avoid a molecular analysis delay, many facilities have introduced reflex testing, for example any biopsy sample identified as an adenocarcinoma and as primary lung origin is automatically sent for EGFR analysis (along with other chosen molecular markers) without requiring a release from the physician. Sampling techniques, such as ROSE ensure that there is sufficient sample for these various molecular techniques, which also translates to avoiding any associated procedural delays with re-biopsy.
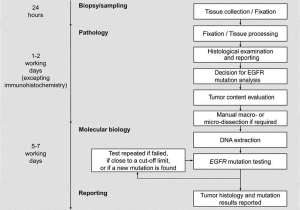
This does not mean however, that patients should never be re-biopsied. There is a growing realization that in patients in whom a driver mutation has been discovered there is tumor heterogeneity and dynamism. An EGFR mutant does not remain static, especially under the selective forces of EGFR-Tyrosine kinase inhibition (TKI). Although initially demonstrating a dramatic response to TKIs most patients will eventually experience treatment failure usually through acquired resistance to EGFR TKI.
Arcila et al. re-biopsied 121 patients with known EGFR mutations and tumor progression and discovered the T790M mutation in 70% with persistence of the original EGFR mutation in all patients (70). Similar results have been found in studies by Sequist, Oxnam and Ohashi et al., including other axonal additions, deletions, SCLC conversions, BRAF mutations and many more. It is clear that identification of the molecular mechanisms will be vital to overcoming EGFR TKI resistance. It is growing apparent that a static biopsy is inadequate to guide therapeutic decision-making during a patient’s treatment course. Re-biopsy at the time of disease progression is becoming standard. Pulmonologists must be available to re-biopsy at progression to assess mutational status (71-76).
Therapeutic endobronchial tumor management
Navigational bronchoscopy/RP-EBUS in fiducial placement for SBRT
Navigational bronchoscopy has been utilized to assist the radiation oncologist for stereotactic body radiation therapy (SBRT). Small peripheral lung lesions that were previously inaccessible can be sampled and fiducial markers can be placed in the same procedure in anticipation for SBRT (77,78). Standard therapy for early stage (I and II) NSCLC is a lobectomy with ipsilateral hilar lymph node dissection. While parenchyma-preserving surgeries such as sublobar resection (wedge resection & segmentectomy) have advanced over the years with improved outcomes, there is still a significant subgroup of patients with poor lung function or other comorbidities that cannot tolerate surgery (79,80). Radiation therapy for this inoperable group of patients with potentially curable disease is an attractive option. SBRT has the ability to deliver high doses of radiation with fidelity to generate margins of 1 cm. SBRT trials consistently report loco-regional and 3-year overall survival rates of 78-87% and 55-88% respectively, both comparable to surgery (81,82). Continuous tracking of respiratory motion using fiducials improves the fidelity of SBRT even further, allowing it to deliver beams with tumor margins of 5 mm. To date, there are three ways to deliver fiducials to or near the target lesion: transthoracic, intravascular and bronchoscopically. Currently, CT-guided transthoracic placement has been generally used, but it has a high pneumothorax rate. CT guided lung biopsy has a pneumothorax rate of 15%, while in some studies this is as high as 38%. This is certainly deleterious in a population of patients selected by their marginal pulmonary health (25,83). Intravascular placement also has its problems too. Intravascular fiducial placement cause pleurisy (13-33%), pulmonary infarcts (5%) and groin hematomas (3%) (78,84,85). ENB placement of fiducials has the advantages of successful delivery of markers with great fidelity and with the low complication rates of bronchoscopy (Figure 6).
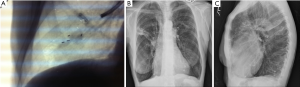
Several studies have looked at the use of radial EBUS and ENB use for fiducial placement, and have found a high success rate (Anatham found that 88% were able to be delivered to within the tumor itself) with very minimal migration of fiducials (78). Indeed, the 10% migration rate seen in studies using linear fiducials was greatly reduced if coil-spring fiducials were employed. Schroeder reported a pneumothorax complication rate of 5.3% (86). A meta-analysis by Wang et al. of over 3,000 bronchoscopies reports a much lower pneumothorax rate (25,87). Larger comparison studies need to confirm the role of ENB and fiducials for inoperable, early stage cancer patients. Currently, results are pending from an interventional trial-RTOG 0618-in comparing tumor control between SBRT and surgery among operable stage I/II patients.
Fiducial markers for localization for surgical biopsy
Navigational bronchoscopy can assist the thoracic surgeon with biopsy of small lesions that are difficult to palpate during video-assisted thoracoscopic surgery (VATS) or thoracotomy, particularly ground glass nodules that warrant histological confirmation of malignancy prior to anatomical resection or parenchymal sparing surgeries. CT-guidance can place fiducial markers with precision around the lesion prior to surgery with confirmation of position (Figure 7). Larger observational and interventional trials are needed to evaluate the efficacy of this complementary approach.
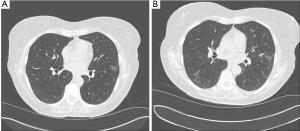
Palliative management in nonsurgical candidates
High dose rate brachytherapy
Henschke introduced the concept and technique of endobronchial brachytherapy in the 1960s as a method of introducing a radioactive source via a thin catheter (afterloader) intraluminally to targeted malignant tissue within the airways (16,88,89). A computerized, remote, ‘afterloading’ technique allows for safe delivery of radioactive material to endobronchial lesions at high doses in short periods of time while greatly minimizing radioactive exposure to staff. The most common radioisotope used is iridium-192 manufactured as a thin, flexible wire. The highly localized field of radiation around the flexible catheter allows for sparing of the surrounding tissue. High-dose rate endobronchial brachytherapy (HDREB) involves delivery of high-energy radiation over short periods (16). Although the available evidence for optimal radiation dosing is currently limited, the American Brachytherapy Society recommends 3 weekly fractions of 7.5 Gy each, 2 fractions of 10 Gy each, or 4 fractions of 6 Gy prescribed at 1 cm (16,90). These outpatient sessions are often well tolerated and rapid with a response to therapy within 4-6 weeks (91). HDREB has shown to benefit patients with hemoptysis, dyspnea, post-obstructive pneumonia, and cough with centrally located lesions typically providing the best outcomes (16,90,91). Symptom control has been shown to be durable up to 6 months. Other potential indications for HDREB include patients who are poor surgical candidates; those who have maximized external beam radiation (EBR) doses; sole treatment for localized bronchial carcinomas; and carcinoma in-situ or pre-cancerous lesions (16,89,90,92). Although overall EBR alone has been more effective than HDREB in terms of durable palliation, combination of EBR with HDREB has also been shown to provide significant symptomatic control especially among patients with inoperable tumors or endobronchial obstruction causing atelectasis (89,91,93).
Photodynamic therapy
The targeted strategy of using photodynamic therapy (PDT) against malignant tissue has been in practice since the 1980s (16,94-96). It is an alternative treatment for cancer that involves administration of a systemic photosensitizing agent that preferentially accumulates in tumor cells. Palliative PDT for obstructive endobronchial tumors have been shown to be an effective strategy for patients experience persistent cough, progressive dyspnea, atelectasis and post-obstructive pneumonia (16,97-99). Results are optimal if the obstructive lesion is found in the segmental and subsegmental airways (100). PDT is generally well tolerated and can be administered regardless of prior chemotherapy, radiation or surgery. The most common photosensitizing agents used in lung cancer are hematoporphyrin derivatives, porfimer sodium (Photofrin®) and talaporfin sodium (Laserphyrin®). These agents are administered intravenously and peak extravascular concentration in tissues is achieved in 24 hours. While concentrations of the photosensitizing agent within peripheral organs decline over the next 2-3 days, tumors have been shown to selectively retain the chemical for much longer periods (16,101). For this reason the next stage of photoactivation typically does not occur until 24-72 hours when the tumor-normal tissue concentration ratio is optimal. During photosensitization a diode laser source emitting red or near-infrared light from a quartz catheter is delivered via flexible bronchoscopy to the endoluminal tumor cells. Currently the FDA recommended light dose is 200 J/cm with a total exposure time of 500 s (16,95,97,101). In addition PDT induces a thrombotic state within tumor microvessels leading to ischemic damage (16,96). As tumor cell death progresses necrotic tissue and debris accumulate in the airways in the next 48 hours after photoactivation. Repeat bronchoscopy is recommended at this point for debridement and prevention of obstruction (16,95,100). Further PDT sessions can be administered up to a maximum of 3 sessions within a 30-day period for residual tumor cells. Although PDT is generally well tolerated photosensitivity reaction in the form of sunburns can persist for up to 6 weeks after injection (16,96,101). The major disadvantage of PDT similar to brachytherapy is a delayed response after photoactivation. Thus this is not a feasible modality if rapid resolution of airway obstruction is needed (16,96,97,101).
Cryotherapy
Cryotherapy is an alternative method of controlling and debulking malignant endobronchial lesions by utilizing extreme cold energy to induce a cascade of events leading to tumor cell death (102,103). The principle of cryotherapy is the delivery of focused extreme cold energy via rapid expansion of compressed liquid nitrogen (the Joule-Thompson effect) at the tip of a cryoprobe. The high vascularity and water content of tumor tissue make it exceptionally vulnerable to extreme cold energy (16,102,104,105).
Endobronchial cryotherapy can be performed either via rigid or flexible bronchoscopy. It is considered a very safe procedure and is generally well tolerated. Patients who may be candidates for this procedure have advanced stage cancers and are poor surgical candidates. Similar to indications for photodynamic or brachytherapy, these patients require alleviation of symptoms attributed to endobronchial obstruction such as hemoptysis, atelectasis, intractable cough or post-obstructive pneumonia.
A recent systematic review noted mean response rates of 80% with minimal complications (0-11%) (106). In a series of 476 patients by Maiwand and colleagues, palliative cryotherapy has shown to provide significant alleviation of hemoptysis, cough, dyspnea and chest pain (76.4%, 69%, 59.2%, and 42.6%, respectively) in addition to improvements in Karnofsky performance scores (59.6 to 75.2) (103,106). Although the available data have shown variable survival rates, median survival time has not shown to be worse than other palliative-focuses endobronchial therapies (102,104-106). The major disadvantage of cryotherapy is its delayed response time and need for repeat treatments relative to other palliative endobronchial procedures such as Nd:YAG laser, electrocautery and microdebridement. Tumor necrosis may continue for days after the initial treatment. Therefore cryotherapy is not optimal for patients experiencing massive hemoptysis or extensive endobronchial tumor involvement (16).
Rigid Bronchoscopy and stenting
Nearly one-third of lung cancer patients experience some form of central airway obstruction (CAO) due to external compression, endoluminal disease or bulky lymphadenopathy (107-110). The quality of life and performance status of patients with CAO is significantly compromised due to dyspnea, stridor, hemorrhage and/or obstructive pneumonias. These airway-related symptoms may preclude the operability of a patient with early lung cancer. Endobronchial stenting has been shown to significantly relieve symptoms and improve quality of life among patients with malignant obstruction (107,111). While this technique has largely been used in advanced lung cancers it is, however, a palliative method that can be utilized in early lung cancer patients with poor functional status. Furthermore early stenting in this patient population may provide an additional survival advantage in addition to symptom relief (112). This will allow those who cannot tolerate surgery to undergo other definitive treatments such as brachytherapy or external radiation.
Conclusions
The last decade has seen many advances in lung cancer diagnosis and management options. Improvements in surgical techniques such as VATS, video mediastinoscopy and parenchymal sparing surgeries; discoveries of new targeted therapies for specific gene mutations in lung adenocarcinomas; and stereotactic radiotherapy for early stage lung cancers all contribute to improvement of quality of life and outcomes for patients with variable performance status. Furthermore, low-dose CT screening for early detection of lung cancers will inevitably revolutionize how lung cancer will be approached. It is paramount that the interventional pulmonologist integrate the armamentarium of minimally invasive approaches described in this review, coupled with sound clinical judgments to collaborate with all specialists of the lung cancer multidisciplinary team.
Acknowledgements
Disclosure: The authors declare no conflict of interest.
References
- Smoking and tobacco use: fast facts. Atlanta: Centers for Disease Control and Prevention. Available online: http://www.cdc.gov/tobacco/data_statistics/fact_sheets/fast_facts
- Jemal A, Siegel R, Xu J, et al. Cancer statistics, 2010. CA Cancer J Clin 2010;60:277-300. [PubMed]
- Wynder EL, Graham EA. Tobacco smoking as a possible etiologic factor in bronchiogenic carcinoma; a study of 684 proved cases. J Am Med Assoc 1950;143:329-36. [PubMed]
- Schmidt H, Voigt K, Emanuel EJ. The ethics of not hiring smokers. N Engl J Med 2013;368:1369-71. [PubMed]
- Silvestri GA, Feller-Kopman D, Chen A, et al. Latest advances in advanced diagnostic and therapeutic pulmonary procedures. Chest 2012;142:1636-44. [PubMed]
- Seijo LM, Sterman DH. Interventional pulmonology. N Engl J Med 2001;344:740-9. [PubMed]
- Levine DJ, Angel LF. Role of the Interventional Pulmonologist. Clin Pulm Med 2006;13:128-41.
- National Lung Screening Trial Research Team, Aberle DR, Adams AM, et al. Reduced lung-cancer mortality with low-dose computed tomographic screening. N Engl J Med 2011;365:395-409. [PubMed]
- International Early Lung Cancer Action Program Investigators, Henschke CI, Yankelevitz DF, et al. Survival of patients with stage I lung cancer detected on CT screening. N Engl J Med 2006;355:1763-71. [PubMed]
- Rivera MP. Preinvasive lesions of the bronchus. Clin Chest Med 2011;32:693-702. [PubMed]
- Travis WD, Brambilla E, Noguchi M, et al. International association for the study of lung cancer/american thoracic society/european respiratory society international multidisciplinary classification of lung adenocarcinoma. J Thorac Oncol 2011;6:244-85. [PubMed]
- Breuer RH, Pasic A, Smit EF, et al. The natural course of preneoplastic lesions in bronchial epithelium. Clin Cancer Res 2005;11:537-43. [PubMed]
- Bota S, Auliac JB, Paris C, et al. Follow-up of bronchial precancerous lesions and carcinoma in situ using fluorescence endoscopy. Am J Respir Crit Care Med 2001;164:1688-93. [PubMed]
- Kennedy TC, McWilliams A, Edell E, et al. Bronchial intraepithelial neoplasia/early central airways lung cancer: ACCP evidence-based clinical practice guidelines (2nd edition). Chest 2007;132:221S-233S.
- Ikeda N, Hayashi A, Iwasaki K, et al. Comprehensive diagnostic bronchoscopy of central type early stage lung cancer. Lung Cancer 2007;56:295-302. [PubMed]
- Principles and Practice of Interventional Pulmonology. Springer, New York, 2013.
- Zaric B, Perin B. Use of narrow-band imaging bronchoscopy in detection of lung cancer. Expert Rev Med Devices 2010;7:395-406. [PubMed]
- Keith RL, Miller YE, Gemmill RM, et al. Angiogenic squamous dysplasia in bronchi of individuals at high risk for lung cancer. Clin Cancer Res 2000;6:1616-25. [PubMed]
- Zaric B, Perin B, Becker HD, et al. Combination of narrow band imaging (NBI) and autofluorescence imaging (AFI) videobronchoscopy in endoscopic assessment of lung cancer extension. Med Oncol 2012;29:1638-42. [PubMed]
- Vincent BD, Fraig M, Silvestri GA. A pilot study of narrow-band imaging compared to white light bronchoscopy for evaluation of normal airways and premalignant and malignant airways disease. Chest 2007;131:1794-9. [PubMed]
- Herth FJ, Eberhardt R, Anantham D, et al. Narrow-band imaging bronchoscopy increases the specificity of bronchoscopic early lung cancer detection. J Thorac Oncol 2009;4:1060-5. [PubMed]
- Zaric B, Becker HD, Perin B, et al. Narrow band imaging videobronchoscopy improves assessment of lung cancer extension and influences therapeutic strategy. Jpn J Clin Oncol 2009;39:657-63. [PubMed]
- Detterbeck FC, Lewis SZ, Diekemper R, et al. Executive Summary: Diagnosis and management of lung cancer, 3rd ed: American College of Chest Physicians evidence-based clinical practice guidelines. Chest 2013;143:7S-37S.
- Schreiber G, McCrory DC. Performance characteristics of different modalities for diagnosis of suspected lung cancer: summary of published evidence. Chest 2003;123:115S-128S. [PubMed]
- Wang Memoli JS, Nietert PJ, et al. Meta-analysis of guided bronchoscopy for the evaluation of the pulmonary nodule. Chest 2012;142:385-93. [PubMed]
- Baaklini WA, Reinoso MA, Gorin AB, et al. Diagnostic yield of fiberoptic bronchoscopy in evaluating solitary pulmonary nodules. Chest 2000;117:1049-54. [PubMed]
- Kurimoto N, Murayama M, Yoshioka S, et al. Assessment of usefulness of endobronchial ultrasonography in determination of depth of tracheobronchial tumor invasion. Chest 1999;115:1500-6. [PubMed]
- Baba M, Sekine Y, Suzuki M, et al. Correlation between endobronchial ultrasonography (EBUS) images and histologic findings in normal and tumor-invaded bronchial wall. Lung Cancer 2002;35:65-71. [PubMed]
- Tanaka F, Muro K, Yamasaki S, et al. Evaluation of tracheo-bronchial wall invasion using transbronchial ultrasonography (TBUS). Eur J Cardiothorac Surg 2000;17:570-4. [PubMed]
- Gildea TR, Mazzone PJ, Karnak D, et al. Electromagnetic navigation diagnostic bronchoscopy: a prospective study. Am J Respir Crit Care Med 2006;174:982-9. [PubMed]
- Makris D, Scherpereel A, Leroy S, et al. Electromagnetic navigation diagnostic bronchoscopy for small peripheral lung lesions. Eur Respir J 2007;29:1187-92. [PubMed]
- Herth F, Ernst A, Schulz M, et al. Endobronchial ultrasound reliably differentiates between airway infiltration and compression by tumor. Chest 2003;123:458-62. [PubMed]
- Steinfort DP, Khor YH, Manser RL, et al. Radial probe endobronchial ultrasound for the diagnosis of peripheral lung cancer: systematic review and meta-analysis. Eur Respir J 2011;37:902-10. [PubMed]
- Eberhardt R, Anantham D, Ernst A, et al. Multimodality bronchoscopic diagnosis of peripheral lung lesions: a randomized controlled trial. Am J Respir Crit Care Med 2007;176:36-41. [PubMed]
- Ernst A, Anantham D. Update on interventional bronchoscopy for the thoracic radiologist. J Thorac Imaging 2011;26:263-77. [PubMed]
- Eberhardt R, Anantham D, Herth F, et al. Electromagnetic navigation diagnostic bronchoscopy in peripheral lung lesions. Chest 2007;131:1800-5. [PubMed]
- Eberhardt R, Anantham D, Ernst A, et al. Multimodality bronchoscopic diagnosis of peripheral lung lesions: a randomized controlled trial. Am J Respir Crit Care Med 2007;176:36-41. [PubMed]
- Makris D, Scherpereel A, Leroy S, et al. Electromagnetic navigation diagnostic bronchoscopy for small peripheral lung lesions. Eur Respir J 2007;29:1187-92. [PubMed]
- Seijo LM, de Torres JP, Lozano MD, et al. Diagnostic yield of electromagnetic navigation bronchoscopy is highly dependent on the presence of a Bronchus sign on CT imaging: results from a prospective study. Chest 2010;138:1316-21. [PubMed]
- Lamprecht B, Porsch P, Wegleitner B, et al. Electromagnetic navigation bronchoscopy (ENB): Increasing diagnostic yield. Respir Med 2012;106:710-5. [PubMed]
- Asano F, Shinagawa N, Ishida T, et al. Virtual bronchoscopic navigation combined with ultrathin bronchoscopy. A randomized clinical trial. Am J Respir Crit Care Med 2013;188:327-33. [PubMed]
- Eberhardt R, Kahn N, Gompelmann D, et al. LungPoint--a new approach to peripheral lesions. J Thorac Oncol 2010;5:1559-63. [PubMed]
- Rusch VW, Asamura H, Watanabe H, et al. The IASLC lung cancer staging project: a proposal for a new international lymph node map in the forthcoming seventh edition of the TNM classification for lung cancer. J Thorac Oncol 2009;4:568-77.
- De Leyn Lardinois D. ESTS guidelines for preoperative lymph node staging for non-cmall cell lung cancer. Eur J Cardiothoracic Surg 2007;32:1-8.
- Hwangbo B, Kim SK, Lee HS, et al. Application of endobronchial ultrasound-guided transbronchial needle aspiration following integrated PET/CT in mediastinal staging of potentially operable non-small cell lung cancer. Chest 2009;135:1280-7. [PubMed]
- Lee PC, Port JL, Korst RJ, et al. Risk factors for occult mediastinal metastases in clinical stage I non-small cell lung cancer. Ann Thorac Surg 2007;84:177-81. [PubMed]
- Herth F, Becker HD, Ernst A. Conventional vs endobronchial ultrasound-guided transbronchial needle aspiration: a randomized trial. Chest 2004;125:322-5. [PubMed]
- Wallace MB, Pascual JM, Raimondo M, et al. Minimally invasive endoscopic staging of suspected lung cancer. JAMA 2008;299:540-6. [PubMed]
- Yasufuku K, Chiyo M, Koh E, et al. Endobronchial ultrasound guided transbronchial needle aspiration for staging of lung cancer. Lung Cancer 2005;50:347-54. [PubMed]
- Adams K, Shah PL, Edmonds L, et al. Test performance of endobronchial ultrasound and transbronchial needle aspiration biopsy for mediastinal staging in patients with lung cancer: systematic review and meta-analysis. Thorax 2009;64:757-62. [PubMed]
- Gu P, Zhao YZ, Jiang LY, et al. Endobronchial ultrasound-guided transbronchial needle aspiration for staging of lung cancer: a systematic review and meta-analysis. Eur J Cancer 2009;45:1389-96. [PubMed]
- Varela-Lema L, Fernández-Villar A, Ruano-Ravina A. Effectiveness and safety of endobronchial ultrasound-transbronchial needle aspiration: a systematic review. Eur Respir J 2009;33:1156-64. [PubMed]
- Yasufuku K, Pierre A, Darling G, et al. A prospective controlled trial of endobronchial ultrasound-guided transbronchial needle aspiration compared with mediastinoscopy for mediastinal lymph node staging of lung cancer. J Thorac Cardiovasc Surg 2011;142:1393-400.e1.
- Vilmann P, Krasnik M, Larsen SS, et al. Transesophageal endoscopic ultrasound-guided fine-needle aspiration (EUS-FNA) and endobronchial ultrasound-guided transbronchial needle aspiration (EBUS-TBNA) biopsy: a combined approach in the evaluation of mediastinal lesions. Endoscopy 2005;37:833-9. [PubMed]
- Annema JT, van Meerbeeck JP, Rintoul RC, et al. Mediastinoscopy vs endosonography for mediastinal nodal staging of lung cancer: a randomized trial. JAMA 2010;304:2245-52. [PubMed]
- Fukuoka M, Wu YL, Thongprasert S, et al. Biomarker analyses and final overall survival results from a phase III, randomized, open-label, first-line study of gefitinib versus carboplatin/paclitaxel in clinically selected patients with advanced non-small-cell lung cancer in Asia (IPASS). J Clin Oncol 2011;29:2866-74. [PubMed]
- Kwak EL, Bang YJ, Camidge DR, et al. Anaplastic lymphoma kinase inhibition in non-small-cell lung cancer. N Engl J Med 2010;363:1693-703. [PubMed]
- Pao W, Miller V, Zakowski M, et al. EGF receptor gene mutations are common in lung cancers from "never smokers" and are associated with sensitivity of tumors to gefitinib and erlotinib. Proc Natl Acad Sci U S A 2004;101:13306-11. [PubMed]
- McDermott U, Iafrate AJ, Gray NS, et al. Genomic alterations of anaplastic lymphoma kinase may sensitize tumors to anaplastic lymphoma kinase inhibitors. Cancer Res 2008;68:3389-95. [PubMed]
- Kwak EL, Camidge DR, Clark J, et al. Clinical activity observed in a phase I dose escalation trial of an oral c-MET and ALK inhibitor, PF-02341066. J Clin Oncol 2009;27:abstr 3509.
- Nakajima T, Yasufuku K. How I do it--optimal methodology for multidirectional analysis of endobronchial ultrasound-guided transbronchial needle aspiration samples. J Thorac Oncol 2011;6:203-6. [PubMed]
- Sakairi Y, Nakajima T, Yasufuku K, et al. EML4-ALK fusion gene assessment using metastatic lymph node samples obtained by endobronchial ultrasound-guided transbronchial needle aspiration. Clin Cancer Res 2010;16:4938-45. [PubMed]
- Navani N, Brown JM, Nankivell M, et al. Suitability of endobronchial ultrasound-guided transbronchial needle aspiration specimens for subtyping and genotyping of non-small cell lung cancer: a multicenter study of 774 patients. Am J Respir Crit Care Med 2012;185:1316-22. [PubMed]
- Bulman W, Saqi A, Powell CA. Acquisition and processing of endobronchial ultrasound-guided transbronchial needle aspiration specimens in the era of targeted lung cancer chemotherapy. Am J Respir Crit Care Med 2012;185:606-11. [PubMed]
- Loukeris K, Vazquez MF, Sica G, et al. Cytological cell blocks: Predictors of squamous cell carcinoma and adenocarcinoma subtypes. Diagn Cytopathol 2010. [Epub ahead of print].
- Wallace WA, Rassl DM. Accuracy of cell typing in nonsmall cell lung cancer by EBUS/EUS-FNA cytological samples. Eur Respir J 2011;38:911-7. [PubMed]
- Micames CG, McCrory DC, Pavey DA, et al. Endoscopic ultrasound-guided fine-needle aspiration for non-small cell lung cancer staging: A systematic review and metaanalysis. Chest 2007;131:539-48. [PubMed]
- Nasuti JF, Gupta PK, Baloch ZW. Diagnostic value and cost-effectiveness of on-site evaluation of fine-needle aspiration specimens: review of 5,688 cases. Diagn Cytopathol 2002;27:1-4. [PubMed]
- Pirker R, Herth FJ, Kerr KM, et al. Consensus for EGFR mutation testing in non-small cell lung cancer: results from a European workshop. J Thorac Oncol 2010;5:1706-13. [PubMed]
- Arcila ME, Oxnard GR, Nafa K, et al. Rebiopsy of lung cancer patients with acquired resistance to EGFR inhibitors and enhanced detection of the T790M mutation using a locked nucleic acid-based assay. Clin Cancer Res 2011;17:1169-80. [PubMed]
- Majem M, Remon J. Tumor heterogeneity: evolution through space and time in EGFR mutant non small cell lung cancer patients. Transl Lung Cancer Res 2013;2:226-237.
- Sequist LV, Waltman BA, Dias-Santagata D, et al. Genotypic and histological evolution of lung cancers acquiring resistance to EGFR inhibitors. Sci Transl Med 2011;3:75ra26. [PubMed]
- Yano S, Nakataki E, Ohtsuka S, et al. Retreatment of lung adenocarcinoma patients with gefitinib who had experienced favorable results from their initial treatment with this selective epidermal growth factor receptor inhibitor: a report of three cases. Oncol Res 2005;15:107-11. [PubMed]
- Yoshimoto A, Inuzuka K, Kita T, et al. Remarkable effect of gefitinib retreatment in a patient with nonsmall cell lung cancer who had a complete response to initial gefitinib. Am J Med Sci 2007;333:221-5. [PubMed]
- Oxnard GR, Arcila ME, Sima CS, et al. Acquired resistance to EGFR tyrosine kinase inhibitors in EGFR-mutant lung cancer: distinct natural history of patients with tumors harboring the T790M mutation. Clin Cancer Res 2011;17:1616-22. [PubMed]
- Ohashi K, Sequist LV, Arcila ME, et al. Lung cancers with acquired resistance to EGFR inhibitors occasionally harbor BRAF gene mutations but lack mutations in KRAS, NRAS, or MEK1. Proc Natl Acad Sci U S A 2012;109:E2127-33. [PubMed]
- Eberhardt R, Kahn N, Herth FJ. ‘Heat and destroy’: bronchoscopic-guided therapy of peripheral lung lesions. Respiration 2010;79:265-73. [PubMed]
- Anantham D, Feller-Kopman D, Shanmugham LN, et al. Electromagnetic navigation bronchoscopy-guided fiducial placement for robotic stereotactic radiosurgery of lung tumors: a feasibility study. Chest 2007;132:930-5. [PubMed]
- Ginsberg RJ, Rubinstein LV. Randomized trial of lobectomy versus limited resection for T1 N0 non-small cell lung cancer. Lung Cancer Study Group. Ann Thorac Surg 1995;60:615-22; discussion 622-3. [PubMed]
- Narsule CK, Ebright MI, Fernando HC. Sublobar versus lobar resection: current status. Cancer J 2011;17:23-7. [PubMed]
- Timmerman R, McGarry R, Yiannoutsos C, et al. Excessive toxicity when treating central tumors in a phase II study of stereotactic body radiation therapy for medically inoperable early-stage lung cancer. J Clin Oncol 2006;24:4833-9. [PubMed]
- Timmerman R, Papiez L, McGarry R, et al. Extracranial stereotactic radioablation: results of a phase I study in medically inoperable stage I non-small cell lung cancer. Chest 2003;124:1946-55. [PubMed]
- Whyte RI, Crownover R, Murphy MJ, et al. Stereotactic radiosurgery for lung tumors: preliminary report of a phase I trial. Ann Thorac Surg 2003;75:1097-101. [PubMed]
- Prasad V, Chan RP, Faughnan ME. Embolotherapy of pulmonary arteriovenous malformations: efficacy of platinum versus stainless steel coils. J Vasc Interv Radiol 2004;15:153-60. [PubMed]
- Mager JJ, Overtoom TT, Blauw H, et al. Embolotherapy of pulmonary arteriovenous malformations: long-term results in 112 patients. J Vasc Interv Radiol 2004;15:451-6. [PubMed]
- Schroeder C, Hejal R, Linden PA. Coil spring fiducial markers placed safely using navigation bronchoscopy in inoperable patients allows accurate delivery of CyberKnife stereotactic radiosurgery. J Thorac Cardiovasc Surg 2010;140:1137-42. [PubMed]
- Harley DP, Krimsky WS, Sarkar S, et al. Fiducial marker placement using endobronchial ultrasound and navigational bronchoscopy for stereotactic radiosurgery: an alternative strategy. Ann Thorac Surg 2010;89:368-73. [PubMed]
- de Aquino Gorayeb MM, Gregório MG, de Oliveira EQ, et al. High-dose-rate brachytherapy in symptom palliation due to malignant endobronchial obstruction: A quantitative assessment. Brachytherapy 2013;12:471-8. [PubMed]
- Nori D, Allison R, Kaplan B, et al. High dose-rate intraluminal irradiation in bronchogenic carcinoma. Technique and results. Chest 1993;104:1006-11. [PubMed]
- Nag S, Cano ER, Demanes DJ, et al. The American Brachytherapy Society recommendations for high-dose-rate brachytherapy for head-and-neck carcinoma. Int J Radiat Oncol Biol Phys 2001;50:1190-8. [PubMed]
- Guarnaschelli JN, Jose BO. Palliative high-dose-rate endobronchial brachytherapy for recurrent carcinoma: the University of Louisville experience. J Palliat Med 2010;13:981-9. [PubMed]
- Ung YC, Yu E, Falkson C, et al. The role of high-dose-rate brachytherapy in the palliation of symptoms in patients with non-small-cell lung cancer: a systematic review. Brachytherapy 2006;5:189-202. [PubMed]
- Kelly JF, Delclos ME, Morice RC, et al. High-dose-rate endobronchial brachytherapy effectively palliates symptoms due to airway tumors: the 10-year M. D. Anderson cancer center experience. Int J Radiat Oncol Biol Phys 2000;48:697-702. [PubMed]
- Usuda J, Tsutsui H, Honda H, et al. Photodynamic therapy for lung cancers based on novel photodynamic diagnosis using talaporfin sodium (NPe6) and autofluorescence bronchoscopy. Lung Cancer 2007;58:317-23. [PubMed]
- Sutedja TG. Photodynamic therapy in advanced tracheobronchial cancers. Diagn Ther Endosc 1999;5:245-51. [PubMed]
- Owen J, Carroll C, Cooke J, et al. School-linked sexual health services for young people (SSHYP): a survey and systematic review concerning current models, effectiveness, cost-effectiveness and research opportunities. Health Technol Assess 2010;14:1-228, iii-iv. [PubMed]
- Loewen GM, Pandey R, Bellnier D, et al. Endobronchial photodynamic therapy for lung cancer. Lasers Surg Med 2006;38:364-70. [PubMed]
- Martin LK, Otterson GA, Bekaii-Saab T. Photodynamic therapy (PDT) may provide effective palliation in the treatment of primary tracheal carcinoma: a small case series. Photomed Laser Surg 2012;30:668-71. [PubMed]
- Kato H. Our experience with photodynamic diagnosis and photodynamic therapy for lung cancer. J Natl Compr Canc Netw 2012;10 Suppl 2:S3-8. [PubMed]
- Furukawa K, Okunaka T, Yamamoto H, et al. Effectiveness of Photodynamic Therapy and Nd-YAG Laser Treatment for Obstructed Tracheobronchial Malignancies. Diagn Ther Endosc 1999;5:161-6. [PubMed]
- Usuda J, Kato H, Okunaka T, et al. Photodynamic therapy (PDT) for lung cancers. J Thorac Oncol 2006;1:489-93. [PubMed]
- Maiwand MO. The role of cryosurgery in palliation of tracheo-bronchial carcinoma. Eur J Cardiothorac Surg 1999;15:764-8. [PubMed]
- Maiwand MO, Asimakopoulos G. Cryosurgery for lung cancer: clinical results and technical aspects. Technol Cancer Res Treat 2004;3:143-50. [PubMed]
- Homasson JP, Renault P, Angebault M, et al. Bronchoscopic cryotherapy for airway strictures caused by tumors. Chest 1986;90:159-64. [PubMed]
- Asimakopoulos G, Beeson J, Evans J, et al. Cryosurgery for malignant endobronchial tumors: analysis of outcome. Chest 2005;127:2007-14. [PubMed]
- Lee SH, Choi WJ, Sung SW, et al. Endoscopic cryotherapy of lung and bronchial tumors: a systematic review. Korean J Intern Med 2011;26:137-44. [PubMed]
- Chin CS, Litle V, Yun J, et al. Airway stents. Ann Thorac Surg 2008;85:S792-6. [PubMed]
- Theodore PR. Emergent management of malignancy-related acute airway obstruction. Emerg Med Clin North Am 2009;27:231-41. [PubMed]
- Wood D. Airway stenting. Chest Surg Clin N Am 2003;13:211-29. [PubMed]
- Lee P, Kupeli E, Mehta AC. Airway stents. Clin Chest Med 2010;31:141-50, Table of Contents. [PubMed]
- Chhajed PN, Baty F, Pless M, et al. Outcome of treated advanced non-small cell lung cancer with and without central airway obstruction. Chest 2006;130:1803-7. [PubMed]
- Razi SS, Lebovics RS, Schwartz G, et al. Timely airway stenting improves survival in patients with malignant central airway obstruction. Ann Thorac Surg 2010;90:1088-93. [PubMed]