Classification of pulmonary neuroendocrine tumors: new insights
Introduction
Neuroendocrine tumors of the lung (Lu-NETs) make up a heterogeneous family of neoplasms ranging from quite indolent lesions with long-term life expectancy to extremely aggressive tumors with very poor prognosis. The 2015 WHO classification has grouped the four histologic variants of Lu-NETs, namely typical carcinoid (TC), atypical carcinoid (AC), large cell neuroendocrine carcinoma (LCNEC) and small cell lung carcinoma (SCLC), into a unique box of neuroendocrine (NE) cell proliferations to facilitate their taxonomy and improve diagnostic recognition (1,2). Behaviorally, TC are low-grade tumors with good prognosis usually cured by surgery alone, AC intermediate-grade tumors with a more aggressive clinical course benefitting from multimodality therapy, and LCNEC and SCLC high-grade full-blown carcinomas with dismal prognosis usually treated by chemo-radiotherapy (1). Although tumor grading is included into the current classification scheme, as considerable clinical and epidemiologic data have been validating a pathologic four-tier, clinical three-tier spectrum of NE-differentiated tumors (1,3), a grading system independent of histology could prove useful in the setting of a metastatic disease and/or small-sized diagnostic material, where morphology alone could not match adequately with the pathologic and clinical grade to support the best therapy choices (4,5).
Tumors homologous with Lu-NENs arise in the thymus (T-NENs) and gastro-entero-pancreatic tract (GEP-NENs), likely due to their common origin from endoderm-derived precursors/stem cells of the foregut according to specific molecular gene pathway alterations (6-11). Significant differences, however, in the biological characteristics of these tumors make a direct comparison with Lu-NETs clinically unwarranted (12-23). Suffice it to say that a backbone observation regarding NENs is that they behave diversely according to the different anatomical sites (23,24), associated risk factors and underlying molecular mechanisms (24-29). However, differences in cell lineage or the diverse application of defining criteria may cause some inconsistency to arise in the diagnostic, prognostic and predictive interpretation (8-11). This bewildering situation becomes even more challenging and frustrating when dealing with metastatic NENs of unproven or uncertain origin and/or small-sized diagnostic material, where morphology alone may be a major pitfall in the management of tumor patients (5,30-36).
The classification of Lu-NETs is a stepwise process based on a constellation of cytological and histological traits alongside the evaluation of mitotic count and necrosis extent (1,2). At variance with its established role in GEP NENs according to existing guidelines (37,38) and the forthcoming 2017 WHO classification on non-pulmonary neuroendocrine tumors, immunohistochemistry (IHC) for NE markers is not strictly required to render an ultimate diagnosis of Lu-NETs. Nonetheless, it is recommended to confirm their NE nature and, particularly, to separate LCNEC from other histologic mimickers, such as large cell carcinoma with NE morphology (LCC-NEM) and basaloid carcinoma, or to identify combined variants with non-small cell carcinoma (NSCC) components (1,2).
Lung TC and AC as a whole are pragmatically equated to well-differentiated neoplasms in opposition to SCLC and LCNEC, which are collectively poorly differentiated tumors closest to the NE carcinoma (NEC) category of GEP NENs (3,8-11). This dichotomy of morphologic classification is consistent with the current assertion that TC and AC in the lung are molecularly distinct from SCLC and LCNEC (1,2,39-43). Major differences in the somatic mutation rate and engagement of diverse gene pathway alterations have been observed in these two main groupings of Lu-NETs (31,42-52); while there is some inter-tumor heterogeneity of molecular events within a category, particularly AC and LCNEC (39,40,53-55). These observations support the view that different patient subsets exist within each variant of Lu-NETs as defined upon histology, with some degrees of overlap among the categories (25,27), suggesting some commonality in their origin, developmental mechanisms, prognosis or treatment options (5,8,9,53-57).
Browsing the recent literature, this review is focused on current Lu-NETs nosology, immunophenotyping, proliferation indices and molecular alterations, trying to place into context all current information for the best management of the patients.
Diagnosis and classification of pulmonary neuroendocrine tumors
TC and AC in the lung retain morphologic criteria of well-differentiated NETs (1,3,58). They are close to the normal NE elements present in the respiratory mucosa, hyperplastic NE cells as seen in chronic inflammation and the diffuse idiopathic pulmonary NE cell hyperplasia (DIPNECH), a pre-invasive lesion with a potential towards the development of carcinoids (1,59-61). Defining criteria of these tumors include organoid growth patterns (rosettes, trabeculae, ribbons, festoons, lobular nests, palisading), absent to focal punctate necrosis (not just apoptotic bodies), up to 10 mitoses per 2 mm2 and a consistent labeling for pan-NE IHC markers, sometimes less intense and uneven in AC (1,3,58,62,63). On the contrary, SCLC and LCNEC are clustered into poorly differentiated tumors showing trabecular to solid to diffuse growth patterns, extensive/geographic necrosis, mitotic count higher than 10 mitoses per 2 mm2 with no theoretical upper limit and uneven cell decoration for pan-NE markers, especially those based on dense-core granules (1,3,62-65). Tumor architecture and cytological details serve further to separate LCNEC from SCLC, even though inter-observer reproducibility remains disappointingly low (66-70). LCNEC is a tumor category defined upon pan-NE IHC markers to exclude histological mimics such as LCC-NEM and basaloid carcinoma, or identify non-NE components in combined variants (1,71). The diagnosis of SCLC in turn relies primarily upon morphology in both the lung and elsewhere, although IHC has been recommended to either reduce the rate of misdiagnosis in challenging cases for technical reasons or to increase the diagnostic confidence of pathologists for complex differential diagnoses (65).
In surgical as well as in biopsy/cytology specimens, general markers of NE differentiation provide a reliable profile for all histologic variants of Lu-NETs, while single hormones are less useful even in the metastatic setting or when facing with unknown origin lesions because of the lack of specific substances produced in the lower respiratory tract (72). Current guidelines and longstanding experience recommend using a couple of well-established pan-NE markers to cross the entire spectrum of Lu-NETs (1-3,65,73). Among plentiful pan-NE markers, the most currently used are cytoplasm-based molecules contained in dense core granules, such as chromogranin A, whose presence closely parallels the biogenesis of secretory granules and hence cell differentiation (74), or small clear synaptic vesicles, such as synaptophysin, which regulates the kinetics of synaptic vesicle endocytosis (75) and whose expression is still retained in high-grade Lu-NETs (1-3,65,73). As poorly differentiated Lu-NET down-regulate chromogranin A, its expression should be evaluated at high-power magnification to appreciate even faint granular signals in scattered tumor cells (1). Embryonic nuclear determinants of NE differentiation in the lung, such as human achaete-scute homolog 1 (hASH1), are usually lost or poorly expressed in carcinoids but retained by high-grade tumors, especially SCLC (76,77), whereas membrane-based NCAM/CD56 is sensitive but less specific for NE differentiation (1,78). The latter is expressed in all Lu-NETs, especially SCLC (40,77-79), but can turn out positive also in conventional NSCC (80), various sarcomas (81,82) and malignant mesothelioma (83). High molecular weight keratins (CK), such as those recognized by the clone 34βE12 (CK1, CK5, CK10 and CK14), are consistently negative in Lu-NETs (84). The squamous differentiation determinant DeltaNp63/p40 is unreactive in all variants of Lu-NETs (85), although its expression is found rarely in some LCNEC exhibiting morphology and molecular alterations more akin to SCLC (40). These tumors with focal (≤10% tumor cells) positivity for DeltaNp63/p40 but no overt squamous differentiation harbor a high prevalence of KEAP1-NFE2L2 alterations, suggesting that they are somewhat linked to squamous cell carcinoma rather than conventional SCLC (40).
Another nuclear determinant of NE differentiation, i.e., insulinoma-associated protein 1 (INSM1), has recently been proposed to stain consistently all variants of Lu-NET regardless of histology, but not conventional adenocarcinoma or squamous cell carcinoma (86,87). This marker is potentially expected to work better than the usual pan-NE antigens because of its wide range of expression across the entire spectrum of Lu-NETs, and could become a reference molecule for NE differentiation in the near future. In general, nuclear markers are less likely affected by cellular damage, thus remain intact on small-sized and/or crushed tissue samples, while organelle- or membrane-associated markers may suffer from non-specific staining due to extracellular extravasation or may not consistently reflect their own locations in the cytoplasm (88). Albeit rare in the lung but less in the mediastinum, nuclear in testis (NUT) midline carcinoma (NMC) (88,89), Ewing sarcoma family (ESF) (90) and desmoplastic small round cell tumor (DSRCT) (91) should also be accounted for in the differential diagnosis of high-grade NETs especially when facing with large unresectable or metastatic lesions, due to close similarities in histologic appearance and striking overlap of IHC markers with more conventional thoracic NETs. In this regard, an appropriate IHC for NUT protein in NMC and specific molecular investigation for diagnostic translocations in ESF and DSRCT allow the correct diagnosis to be rendered in most cases with high rate of confidence.
Identifying the origin of neuroendocrine tumors
The identification of thoracic NEN origin plays an important role in adopting the most appropriate terminology, correctly classifying metastatic tumors and offering the best therapeutic options (1,2,92), given that lung and thymus NETs differ in tumor presentation (7,12,14), associated endocrine syndromes (12,13,16,18,19,21,93,94), underlying risk factors (1,15) and molecular alterations (1,95). The differentiation of Lu-NETs from T-NETs proves to be particularly challenging in the setting of low- to intermediate-grade tumors displaying large unresectable or metastatic lesions at the time of diagnosis (96). Whether IHC is able to play some role in this task is outlined below.
Thyroid transcription factor-1 (TTF-1) is a useful marker of pulmonary lineage only when positive in the group of well-differentiated NETs. Of note, only a minority of well-differentiated Lu-NETs, especially those composed of spindle cells arising from peripheral bronchioles, are TTF-1 positive (1,97-102), so as some of DIPNECH or NE cell hyperplasia of the lung (103). The main limitation to the use of this marker in high-grade NECs as a descriptor of lung origin is that most of them demonstrate a diffuse reactivity regardless of their pulmonary or extra-pulmonary derivation (1,102,104). Moreover, some T-NETs (93) may be reactive for TTF-1 even when using the most specific clone 8G7G3/1, thus TTF-1 may not be a reliable maker to confirm the pulmonary origin in thoracic NETs. Many other nuclear transcription factors, which are frequently used to differentiate well differentiated NETs in diverse organs, namely Islet-1 (103,105,106), PAX-8 (101,105) and CDX2 (106), can be aberrantly and illegitimately expressed in high-grade NETs regardless of their anatomical locations (31,107). Similarly, CD117 does not separate reliably lung from thymic NETs because is often expressed in both anatomical compartments, especially less differentiated lesions (1,108,109) as well as conventional NSCC (110) and thymic carcinoma (1,92). Conversely, CD5 expression is exceedingly rare in NETs of either anatomical site (23), while CD5 reacts with about a half of thymic squamous cell carcinomas (1,92,111) and a non-negligible fraction of NSCLC (112). Potential confounding factor is represented by combined variants of NETs not only at the level of the IHC characterization (due to co-expression of unexpected profiles and lack of protein expression characteristic of either tumor type), but also at the molecular level (113). It has recently been noted that mixed NE/non-NE carcinomas are molecularly different from their pure NE and non-NE counterparts in the lung and a variety of extra-pulmonary sites when analyzed for cDNA quantification of ribonucleotide reductase, large subunit 1, excision repair cross-complementation group 1 and thymidylate synthase (114). In this setting of a predominant non-NE component, the administration of adjuvant therapy in addition to surgery and a high thymidylate synthase expression in non-NET components were significantly associated with a lower risk of patient death, thereby improving the clinical strategies for the treatment of these rare and underestimated tumors (114).
The role of IHC becomes particularly relevant in the scenario of small biopsy or cytology samples, where it is clinically warranted to separate NE from other non-NE tumors or unrelated malignancies mimicking NETs in either the lung or thymus. In a recent international study carried out on biopsy samples, the rate of agreement on SCLC diagnosis was increased from 64.7% obtained by the solely morphology to 77.5% with a judicious use of a variety of IHC markers, such as cytokeratin cocktail, pan-NE markers, TTF1, p16 (expressed by high-grade NETs, especially SCLC), retinoblastoma protein and Ki-67 antigen, with Cohen’s kappa coefficient scores on IHC being 0.60 and 0.64 in resected specimens and biopsy samples, respectively (65). While the differentiation of SCLC from other unrelated mimics (undifferentiated carcinomas, small cell sarcomas or lymphomas) requires further IHC markers and molecular assays, the single most reliable marker in NETs to get insights into their clinical behavior remains the Ki-67 antigen (1,30,115). This marker is particularly reliable on small biopsy or cytology samples in the presence of scarce material or crush artifacts (32).
Identifying the clinical aggressiveness of neuroendocrine tumors and the role of Ki-67 antigen
Ki-67 antigen has been extensively evaluated in Lu-NET with several diagnostic, prognostic and grading implications [reviewed in (115)]. Since the Ki-67 antigen identifies proliferating cells spanning from G1 to M phase (116-118), Ki-67 nuclear expression is proportional to the mitotic count, but reveals more proliferating tumor cells than the latter, which remains the backbone of defining criteria in Lu-NETs and T-NETs according to existing guidelines (1). The method to quantify Ki-67 expression and that to count mitoses are different; a percentage of Ki-67 positive cells (labeling index, LI) is usually obtained in hot spot regions with highest staining, while the mitotic count is based on the number of mitotic figures in pre-defined tumor areas of highest activity (high power fields or square millimeters). The difference may account in part for inconsistent results between these proliferation indexes (4). Indeed, once mitoses are expressed as mitotic index rather than mitotic count (119), the correlation rate with Ki-67 LI increases considerably (120,121). Furthermore, another source of inconsistency is the alleged lack of inter-observer reproducibility of Ki-67 LI, which is likely attributed to the differences in regions (randomly selected fields; hot spot areas of highest labeling; entire tumor area) and methods (few hundreds to thousands of tumors cells; eyeball, manual or picture-based count evaluation or automated analysis system adoption) for counting Ki-67 positive tumor cells, once pre-analytical issues (fixation, staining systems, reagents) are equated (8,115,122-124). Another conundrum is the intra-tumor heterogeneous distribution of Ki-67 antigen likely due to the unpredictable occurrence of differentially regulated tumor cell subsets in tumors (115,120), but the consistent assessment of labeling indexes in hot spot regions will lead to more reproducible results (115). This biological phenomenon becomes particularly challenging on biopsy/cytology specimens in comparison with resection specimens (30,33,125) because of unpredictable sampling issues (the so-called tip-of-the-iceberg effect or the part of the whole). Therefore, the reproducibility and clinical usefulness of Ki-67 LI in Lu-NETs have been seriously argued, with alleged superiority of mitotic count, which has remained the only proliferation criterion in tumor classifications over time (1,126,127), with no current diagnostic role for Ki-67 (1). However, reproducibility of results for Ki-67 LI is not worse than any other IHC markers when tested for quantification (128,129) and even mitotic count shows large inter-observer variability in Lu-NETs classification of either carcinoids (130) or high-grade tumors (66-70). The only obvious difference is that mitotic count is traditional, largely known by pathologists everywhere and carried out simultaneously with hematoxylin-eosin diagnosis, whereas Ki-67 antigen evaluation requires additional IHC on new paraffin sections and a numeric quantification. Hopefully, the localization of the same tumor areas as those assessed for mitotic count will make results biologically more reasonable (115). Last but not least, the unavailability of IHC in some pathology laboratories would prefer mitotic count over Ki-67 LI in the daily practice. In any event, Ki-67 LI should not be used as a surrogate of mitotic count, since it is not a current defining criterion, but rather a complementary tool. In contrast, Ki-67 LI is a backbone of the grading system of GEP tract NENs, once tumors are split into well differentiated and poorly differentiated categories based on morphological grounds (8-11,37,131).
A recent standardization of Ki-67 LI on biopsy samples and the corresponding surgical specimens of Lu-NETs have demonstrated that results were quite superimposable with minimal deviation, even among different observers, provided that precise methodology rules were a priori established and used in either type of material (30). In keeping with other comparative studies (33,125), it was important to start identifying hot spot regions in either type of material in order to obtain overlapping results when counting 2,000 cells, 2-mm2-spanning areas or the entire biopsy fragment(s) (30). In this way, it was possible to attribute to unpredictable sampling, tissue sizing, intra-tumor heterogeneous distribution of Ki-67 antigen and inter-observer discordance when Ki-67 LI were discrepant between biopsies and the corresponding resection specimens (30). This study also suggests that different methods for the Ki-67 LI assessment but not an inherent unreliability of the marker as a biological predictor affect its clinical meaning (30). Recent reproducibility studies in Lu-NET have revealed that there is less than 1.5% of variability when evaluating Ki-67 LI, with an out-performance over mitotic count with regard to inter-observer agreement (132,133).
Although Ki-67 LI is not currently accredited with Lu-NET subtyping due to some overlap of cut-off thresholds among biologically adjacent tumors (TC vs. AC, AC vs. LCNEC, LCNEC vs. SCLC), its differential distribution between low- to intermediate-grade and high-grade tumors has made it an irreplaceable discriminator especially on biopsy/cytology samples, and recommended even on surgical specimens (1,32,115). A Ki-67 LI up to 20–25% has the highest specificity and sensitivity for low- to intermediate-grade versus high-grade tumors, whilst other IHC markers or a combination of necrosis and mitotic count have lower specificity and sensitivity (1,30,32,115). Given the reported prognostic role of the Ki-67 LI within the same group, such as TC and/or AC, Ki-67 LI has emerged as a reliable criterion for clinical decision-making (132,134-137), in particular, in the setting of metastatic disease. It is important to note that Ki-67 LI closely reflects tumor biology, while the role of the more fallacious morphology is limited in small-sized and/or crushed diagnostic materials (138). Such an advantage holds particularly true for AC and LCNEC, the most clinically challenging categories of Lu-NETs likely due to the large range of diagnostic criteria (2–10 mitoses in AC; no upper limit over 10 mitoses alongside a wide spectrum of morphology for LCNEC) (1,40,66,67,69,139). Conversely, diagnostic criteria for TC (virtual absence of mitosis and necrosis) and SCLC (undifferentiated morphology, plentiful mitoses, extensive/geographic necrosis) are consistent with biologically and behaviorally more homogeneous categories that occupy the ends of the Lu-NET spectrum (1,73). Not unexpectedly, Ki-67 is typically 5% or less in TC and usually 80% or more in SCLC (118). Our recent observations showed that AC with Ki-67 LI of 10% or more consisted of tumors with more prominent proliferation activity within the allowed range of mitotic count and necrosis, and are associated with worse prognosis than predicted within the tumor category (140). It has also been noted that LCNEC with molecular alterations akin to NSCLC or AC feature the mean Ki-67 LI lower than that of LCNEC with molecular alterations similar to SCLC (i.e., SCLC-like LCNEC), which in turn feature cytomorphology in the SCLC spectrum (40). Other studies have revealed that AC behaviorally can overlap with either TC, SCLC or LCNEC when using Ki-67 LI thresholds specifically designed for the lung (4).
As necrosis and mitoses are unreliable criteria on biopsy samples (1,3,30,58), the differentiation of TC from AC or the diagnosis of LCNEC is usually not permissive on the morphologic basis alone or can only be suggested in these samples (1,141). In Lu-NETs, the main clinical question concerns the need of the patient risk stratification on the basis of tumor aggressiveness, especially in the setting of metastatic disease where the morphology on small-sized diagnostic materials could be misleading (5,138). Therefore, together with the overall clinical profiles (radiology and nuclear medicine imaging, tumor burden, symptoms and individual risk for evolving disease) Ki-67 LI could potentially be a decisional factor to stratify patients into more defined clinical categories for precision medicine (5).
Accordingly, metastatic Lu-NETs can be stratified into four main clinical groups by integrating Ki-67 LI and traditional histology (5).
- The first group consist of completely indolent tumors showing Ki-67 LI of 5% or less, which biologically correspond to either TC or AC with a low mitotic count and could be treated with biological drugs (somatostatin analogues or m-TOR pathway inhibitors), if any (3,58).
- The second group includes low-to-moderate malignant tumors showing Ki-67 LI up to 20–25%, which biologically correspond to rare TC, most AC and even some LCNEC with a molecular profile similar to that of carcinoids (40). They are probably managed still with biological drugs and/or peptide receptor radionuclide therapy alone (142-144), though no official guidelines yet exist for them (3,58,145,146).
- The third group consists of moderate to higher malignant tumors with Ki-67 LI ranging from 25% to 50–60%, biologically corresponding to more uncommon aggressive AC or LCNEC with a molecular profile similar to that of NSCLC (40). They can be treated with alkylating drugs or others but not with platinum/etoposide-based chemotherapy, such as gemcitabine, paclitaxel or vinorelbine (93,144).
- The last group is composed of highly malignant tumors with Ki-67 LI ranging from 60% to 100%, biologically corresponding to aggressive SCLC and SCLC-like LCNEC on molecular grounds (41), which should be treated with platinum/etoposide-based chemotherapy (1,144).
Phenotypic/genotypic correlations in Lu-NET showed that a Ki-67 LI over 10% predicts poor prognosis within the AC category outperforming necrosis and mitotic count (140) and that there is a close relationship between proliferation activity and molecular subcategorization of LCNEC (40). For the latter, LCNEC exhibiting a molecular profile similar to that of SCLC had the highest Ki-67 LI (on average 90%), LCNEC harboring NSCLC-like mutations displayed an intermediate value around 60% and those bearing MEN1 mutations presented with the lowest Ki-67 LI around 35% (40). This peculiar distribution of Ki-67 LI as a function of cell morphology and differentiation levels is similar to what has recently been described in the digestive system under the new classification of GEP NEN tumors, which, along with NET G1 and G2, now includes NET G3, distinct from (poorly differentiated) NEC (144).
Neuroendocrine tumors and molecular alterations
It is widely accepted that Lu-NETs and T-NETs consist of biologically distinct groups but not a continuum of neoplasms with common pathogenesis (1,50,92). In both anatomical sites, TC/AC on the one hand and LCNEC/SCLC on the other prove separate malignancies when molecularly dissected, with recurrent and non-random alterations of cell cycle checkpoints, chromatin remodeling and recurrent chromosomal alterations (1,50,92,95). Whether genetic traits are better than morphology or IHC markers to distinguish lung from thymus NENs, especially in the setting of large tumors occupying both anatomical regions and metastatic sites, still remains as an unanswered question due to the lack of organ-specific profiles.
TC and AC in the lung (42,44-46,48,50,55,115,147) and the homologous G1-G2 NETs in the GEP tract (24,148) (data thus far are largely unavailable for T-NETs) display lower somatic mutation rates (<1 per million base pairs) compared to the high-grade counterparts likely due to substantial differences in risk factors (1,149-151), gene pathway activations, levels of differentiation and purported cell derivation (42,44-46,48,50,55,115,147). In the lung, defined candidate driver alterations are identifiable in up to 73% of TC and AC (42). Briefly, RB1 and TP53 mutations are quite uncommon in TC and AC (152), whereas inactivation of genes affecting histone methylation (MEN1) by multiple mechanisms and SWI/SNF complex subunit mutations (ARID1A, SMARC1, SMARCA2, SMRCA4) are found in up to one third of cases (42,50-52,55). Additional alterations include mutations of CBX6, EZH2, EIF1AX and E3 ubiquitin ligases (52).
Among high-grade Lu-NETs, LCNEC constitute a heterogeneous family of tumors, with some of them being classified SCLC-like LCNEC accounting for about 40%, NSCLC-like LCNEC (either adenocarcinoma or squamous cell carcinoma) accounting for about 50% and carcinoid-like LCNEC accounting for about 5% on the basis of the different sets of altered genes (40). SCLC-like LCNEC share molecular alterations with SCLC and show RB1, TP53, CREBBP, EP300 and MLL gene mutations (40,42,49) alongside MYCL1 and FGFR1 amplifications. NSCLC-like LCNEC exhibit CDKN2A deletion, TTF1 amplifications and KEAP1 and STK11 mutations as observed in non-NE tumors (40,42,49) and carcinoid-like LCNEC bear MEN1 mutations (40,153). SCLC has one of the highest mutation rates in cancers, with inactivating mutations of tumor suppressor genes (TP53, RB1) and chromatin remodeling genes (CREBBP, EP300, MLL) being more frequently found (42,46,154). Other recurrent gene aberrations include mutations of PTEN, SLIT2, COBL, EPHA7 and CDKN2A genes, along with amplification of FGFR1, MYCL1, MYCN, MYC, SOX2, KIAA1432, RICTOR, JAK2 and MAD1L1 (42,44,46,48,154-157) and recurrent fusion transcript RLF-MYCL1 (44,48). Inactivation of NOTCH gene upon mutation with simultaneous ASCL1 and canonical WNT signaling engagement in addition to mutual bi-allelic RB1 and TP53 lesions is at the basis of pulmonary and extra-pulmonary small cell carcinoma developing as secondary tumors from preexisting non-NE carcinomas, either spontaneous or induced by therapy (158). Activation of epithelial-mesenchymal transition (EMT) via fascin-induced E-cadherin/β-catenin system alterations that are responsible for nuclear shuttling of non-mutated β-catenin has been documented in a subset of LCNEC and SCLC (159-161). A further level of complexity in the molecular heterogeneity of Lu-NETs is unraveled by differences in gene expression profiling or diverse expression of functional biomarkers, such as CD44, orthopedia transcription factor, CEACAM and vitamin D-binding protein, which are able to identify patient subsets differentially at risk of progression within each histological variant or tumor group (52,54-56,97,159,162).
Molecular alterations of T-NETs are poorly understood and, in particular, only few next generation sequencing (NGS) studies have been conducted. In these tumors, MEN1 genotype/phenotype correlation is less significant than in Lu-NETs suggesting the involvement of other genetic factors (15,17,18,20,21). As a matter of fact, about one fourth of T-NETs are MEN1-related (20,163), whereas only 1–8% of patients with MEN1 syndrome develop T-NET during life (15,17,18,163-167). Most of MEN1-related T-NETs correspond to carcinoids (163), but even poorly differentiated NE carcinomas (18,168) or purported carcinoids with gross areas of necrosis (15) have been reported. Interestingly, two T-NET cases have recently been reported, in which synchronous or metachronous LCNEC arose within a background of preexisting AC. All tumor components, either AC or LCNEC, presented with CTNNB1 mutations, which were likely responsible for cyclin D1-RB1 axis-dependent tumor growth, along with subsequent TP53 and JAK3 mutations in one case and EMT activation in the other case leading to de-differentiation and further tumor expansion (23). Chromosomal imbalances, whether loss or gain (95,169,170), and aneuploidy (171) are differentially distributed among the diverse subtypes of T-NETs, with the mean number of chromosomal imbalances being 0.8, 1.1 and 4.7 in TC (31% aberrant cases), AC (44% aberrant cases) and high-grade T-NET (75% aberrant cases), respectively. Gains of 8q24 mapping to MYC gene locus was the most frequent alteration and one of the overlapping features between carcinoids and high-grade T-NENs (95).
Molecular alterations across Lu-NETs, T-NETs or GEP-NENs, yet with different prevalence rates, have recently been documented as mutations, copy number variations and microRNAs (24-27,95,157), thus supporting innovative insights into the developmental mechanisms of these tumors. The existence of combined variants of NETs in several anatomical locations (1,113) and experimental models on the development of secondary SCLC (158) reveal a high plasticity of cancer stem cells through the activation of multiple genetic and epigenetic mechanisms. It could be hypothesized that the occurrence of common genetic events among diversely classified tumors is functional to maintain shared biological, morphological or functional traits and/or that even low grade tumors with well differentiated morphology have a potential to evolve into high-grade NETs (23). Interestingly, Lu-NETs, T-NETs and even GEP NENs with dual components of high- and low-grade tumors in synchronous or metachronous lesions have been identified (23,40,171-178). Further, these tumors share molecular alterations indicative of an origin from common ancestors of lower grade, with additional gene mutations occurring in the high-grade components over time due to temporally delayed clonal evolution of tumor cells. This combination of low-grade and high-grade components has been documented in the thymus (23,173,174) as secondary high-grade NETs, in the lung as NE carcinoma with carcinoid morphology (172) or carcinoids with proliferation rate progression (179), and in the GEP tract as transformed NET, well differentiated NET featuring high-grade components or well differentiated NET with high-grade (G3) progression (180,181). This concept, which would represent a paradigm shift from accepted pathogenesis schemes, has not been included in the current WHO classification of Lu-NETs where carcinoids or NETs are not thought to be usual early forerunners of high-grade lesions (1) and is probably an under-recognized phenomenon in these tumors. The reverse, i.e., down-grading of poorly differentiated NETs, is not supported by the clinical behavior of these tumors (usually more aggressive than lower grade counterparts but less lethal than high-grade tumors) and by the occurrence of the same molecular alterations in both tumor components alongside further aberrations in high-grade elements promoting tumor dedifferentiation, growth and invasion (23). The recently described categories of NET G3 within the GEP tract (113,180) with a preserved well-differentiated NE morphology yet showing a mitotic count above 20 mitoses per 2 mm2 and Ki-67 LI over 20%, the carcinoid-like LCNEC and NE carcinoma with carcinoid morphology (40,172), and synchronous or metachronous thymus LCNEC retaining AC components (23) are instances of secondary high-grade NETs (23) derived from preexisting G1/G2 GEP NETs or lung and thymus TC/AT. CTNNB1 gene mutations could be one of the molecular alterations underlying the progression in the thymus (23) and GEP tract (26). Similar occurrences, however, have been documented in secondary glioblastoma from long-standing astrocytoma (182,183) or triple negative breast cancer from adenoid cystic carcinoma (184).
The hallmark of such secondary high-grade NETs in the thymus (23) and GEP tract (NV, personal communication) is the presence of concurrent and variably intermingled areas with low or high Ki-67 LI/mitotic counts within a tumor. These evolving tumors would be usually associated with an intermediate clinical course (23,181), unless additional, adverse molecular alterations, such as TP53 inactivation, took place over time (23). An instance of such a tumor arising in the lung is depicted in Figure 1, which emphasizes once again the role of Ki-67 LI for diagnosis and biological interpretation. This heterogeneous intra-tumor distribution of Ki67 LI likely results from diversely tuned cell subsets causing hot and cold spot areas to appear within individual tumors. Heterogeneity of Ki-67 LI at metastatic sites and among different metastasis locations as compared with paired primaries was first described in pancreatic NETs (120), but has recently been indicated to occur in stage IV lung carcinoids only in an abstract form (179).
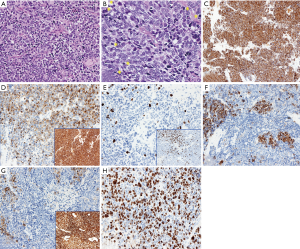
At this point, the clinical and biological questions are related to the frequency of this event and to the level of diagnostic awareness by pathologists. It has been observed that carcinoids and high-grade NE carcinomas in the lung share most of the altered genes, such as mutations, copy number variations and microRNA, even though at different prevalence (24-27,95,157), and that common mutations or chromosomal changes may unexpectedly cluster tumor regardless of histology. This supports a transition from low to high grade in a non-negligible fraction of Lu-NETs (GP, personal experience). To partly explain the apparent contradiction to the widely reported differential distribution of molecular events among diverse NETs in both the lung and elsewhere (1,50,92,95), it should be kept in mind that most of existing molecular data have been derived from the analysis of surgical specimens, where up to one fourth/one third of high-grade NET patients experience longer survival (56). This implies that histologically poorly differentiated neoplasms amenable of complete resection and running a more favorable clinical course could harbor these evolving secondary high-grade NE tumors. It will be essential to clarify if these tumors correspond to progressing lesions, which are in turn highlighted by heterogenous Ki-67 LI, in order to understand their pathological basis and explore the most appropriate clinical management.
Conclusions
Our understanding of Lu-NET is rapidly expanding, especially regarding diagnosis, IHC marker choice and pathogenetic mechanisms, although adapting the morphology-based classification to the personalized and precision medicine is still challenging. This holds true in NETs of other organs as well. The assumption of differential genomic alterations between well-differentiated NE tumors and high-grade NE carcinomas of the lung and thymus is substantiated by multiple studies, but reassembling the existing data under the concept of secondary high-grade NETs has led to a possible paradigm shift in the pathogenesis of NETs.
Acknowledgements
This work is dedicated with special mention to the memory of Carlotta, an extraordinarily lively girl who ultimately died of cancer in the prime of life.
Footnote
Conflicts of Interest: The authors have no conflicts of interest to declare.
References
- Travis W, Brambilla E, Burke A, et al. WHO Classification of Tumours of the Lung, Pleura, Thymus and Heart. Fourth Ed. ed. World Health Organization Classification of Tumours. Lyon: IARC Press, 2015.
- Travis WD, Brambilla E, Nicholson AG, et al. The 2015 World Health Organization Classification of Lung Tumors: Impact of Genetic, Clinical and Radiologic Advances Since the 2004 Classification. J Thorac Oncol 2015;10:1243-60. [Crossref] [PubMed]
- Caplin ME, Baudin E, Ferolla P, et al. Pulmonary neuroendocrine (carcinoid) tumors: European Neuroendocrine Tumor Society expert consensus and recommendations for best practice for typical and atypical pulmonary carcinoids. Ann Oncol 2015;26:1604-20. [Crossref] [PubMed]
- Rindi G, Klersy C, Inzani F, et al. Grading the neuroendocrine tumors of the lung: an evidence-based proposal. Endocr Relat Cancer 2013;21:1-16. [Crossref] [PubMed]
- Pelosi G, Pattini L, Morana G, et al. Grading lung neuroendocrine tumors: Controversies in search of a solution. Histol Histopathol 2017;32:223-41. [PubMed]
- Falkmer S. Phylogeny and ontogeny of the neuroendocrine cells of the gastrointestinal tract. Endocrinol Metab Clin North Am 1993;22:731-52. [PubMed]
- Moran CA, Suster S. Neuroendocrine carcinomas (carcinoid tumor) of the thymus. A clinicopathologic analysis of 80 cases. Am J Clin Pathol 2000;114:100-10. [Crossref] [PubMed]
- Klimstra DS. Pathologic Classification of Neuroendocrine Neoplasms. Hematol Oncol Clin North Am 2016;30:1-19. [Crossref] [PubMed]
- Klimstra DS, Beltran H, Lilenbaum R, et al. The spectrum of neuroendocrine tumors: histologic classification, unique features and areas of overlap. Am Soc Clin Oncol Educ Book 2015.92-103. [Crossref] [PubMed]
- Klimstra DS, Modlin IR, Adsay NV, et al. Pathology reporting of neuroendocrine tumors: application of the Delphic consensus process to the development of a minimum pathology data set. Am J Surg Pathol 2010;34:300-13. [Crossref] [PubMed]
- Klimstra DS, Modlin IR, Coppola D, et al. The pathologic classification of neuroendocrine tumors: a review of nomenclature, grading, and staging systems. Pancreas 2010;39:707-12. [Crossref] [PubMed]
- Ahn S, Lee JJ, Ha SY, et al. Clinicopathological analysis of 21 thymic neuroendocrine tumors. Korean J Pathol 2012;46:221-5. [Crossref] [PubMed]
- Cardillo G, Rea F, Lucchi M, et al. Primary neuroendocrine tumors of the thymus: a multicenter experience of 35 patients. Ann Thorac Surg 2012;94:241-5; discussion 245-6. [Crossref] [PubMed]
- de Montpréville VT, Macchiarini P, Dulmet E. Thymic neuroendocrine carcinoma (carcinoid): a clinicopathologic study of fourteen cases. J Thorac Cardiovasc Surg 1996;111:134-41. [Crossref] [PubMed]
- Ferolla P, Falchetti A, Filosso P, et al. Thymic neuroendocrine carcinoma (carcinoid) in multiple endocrine neoplasia type 1 syndrome: the Italian series. J Clin Endocrinol Metab 2005;90:2603-9. [Crossref] [PubMed]
- Fukai I, Masaoka A, Fujii Y, et al. Thymic neuroendocrine tumor (thymic carcinoid): a clinicopathologic study in 15 patients. Ann Thorac Surg 1999;67:208-11. [Crossref] [PubMed]
- Goudet P, Dalac A, Le Bras M, et al. MEN1 disease occurring before 21 years old: a 160-patient cohort study from the Groupe d'etude des Tumeurs Endocrines. J Clin Endocrinol Metab 2015;100:1568-77. [Crossref] [PubMed]
- Goudet P, Murat A, Cardot-Bauters C, et al. Thymic neuroendocrine tumors in multiple endocrine neoplasia type 1: a comparative study on 21 cases among a series of 761 MEN1 from the GTE (Groupe des Tumeurs Endocrines). World J Surg 2009;33:1197-207. [Crossref] [PubMed]
- Liu RX, Wang WQ, Ye L, et al. p21-activated kinase 3 is overexpressed in thymic neuroendocrine tumors (carcinoids) with ectopic ACTH syndrome and participates in cell migration. Endocrine 2010;38:38-47. [Crossref] [PubMed]
- Teh BT. Thymic carcinoids in multiple endocrine neoplasia type 1. J Intern Med 1998;243:501-4. [Crossref] [PubMed]
- Thevenon J, Bourredjem A, Faivre L, et al. Unraveling the intrafamilial correlations and heritability of tumor types in MEN1: a Groupe d'etude des Tumeurs Endocrines study. Eur J Endocrinol 2015;173:819-26. [Crossref] [PubMed]
- Sullivan JL, Weksler B. Neuroendocrine Tumors of the Thymus: Analysis of Factors Affecting Survival in 254 Patients. Ann Thorac Surg 2017;103:935-9. [Crossref] [PubMed]
- Fabbri A, Cossa M, Sonzogni A, et al. Thymus neuroendocrine tumors with CTNNB1 gene mutations, disarrayed ss-catenin expression, and dual intra-tumor Ki-67 labeling index compartmentalization challenge the concept of secondary high-grade neuroendocrine tumor: a paradigm shift. Virchows Arch 2017;471:31-47. [Crossref] [PubMed]
- Scarpa A, Chang DK, Nones K, et al. Whole-genome landscape of pancreatic neuroendocrine tumours. Nature 2017;543:65-71. [Crossref] [PubMed]
- Simbolo M, Mafficini A, Sikora KO, et al. Lung neuroendocrine tumours: deep sequencing of the four World Health Organization histotypes reveals chromatin-remodelling genes as major players and a prognostic role for TERT, RB1, MEN1 and KMT2D. J Pathol 2017;241:488-500. [Crossref] [PubMed]
- Vijayvergia N, Boland PM, Handorf E, et al. Molecular profiling of neuroendocrine malignancies to identify prognostic and therapeutic markers: a Fox Chase Cancer Center Pilot Study. Br J Cancer 2016;115:564-70. [Crossref] [PubMed]
- Vollbrecht C, Werner R, Walter RF, et al. Mutational analysis of pulmonary tumours with neuroendocrine features using targeted massive parallel sequencing: a comparison of a neglected tumour group. Br J Cancer 2015;113:1704-11. [Crossref] [PubMed]
- Nieser M, Henopp T, Brix J, et al. Loss of Chromosome 18 in Neuroendocrine Tumors of the Small Intestine: The Enigma Remains. Neuroendocrinology 2017;104:302-12. [Crossref] [PubMed]
- Karpathakis A, Dibra H, Pipinikas C, et al. Prognostic Impact of Novel Molecular Subtypes of Small Intestinal Neuroendocrine Tumor. Clin Cancer Res 2016;22:250-8. [Crossref] [PubMed]
- Fabbri A, Cossa M, Sonzogni A, et al. Ki-67 labeling index of neuroendocrine tumors of the lung has a high level of correspondence between biopsy samples and surgical specimens when strict counting guidelines are applied. Virchows Arch 2017;470:153-64. [Crossref] [PubMed]
- Pelosi G, Fabbri A, Cossa M, et al. What clinicians are asking pathologists when dealing with lung neuroendocrine neoplasms? Semin Diagn Pathol 2015;32:469-79. [Crossref] [PubMed]
- Pelosi G, Rodriguez J, Viale G, et al. Typical and atypical pulmonary carcinoid tumor overdiagnosed as small-cell carcinoma on biopsy specimens: a major pitfall in the management of lung cancer patients. Am J Surg Pathol 2005;29:179-87. [Crossref] [PubMed]
- Barnes J, Johnson SJ, French JJ. Correlation of Ki-67 indices from biopsy and resection specimens of neuroendocrine tumours. Ann R Coll Surg Engl 2017;99:193-7. [Crossref] [PubMed]
- Clay V, Papaxoinis G, Sanderson B, et al. Evaluation of diagnostic and prognostic significance of Ki-67 index in pulmonary carcinoid tumours. Clin Transl Oncol 2017;19:579-86. [Crossref] [PubMed]
- Sampathirao N, Basu S. MIB-1 Index-Stratified Assessment of Dual-Tracer PET/CT with 68Ga-DOTATATE and 18F-FDG and Multimodality Anatomic Imaging in Metastatic Neuroendocrine Tumors of Unknown Primary in a PRRT Workup Setting. J Nucl Med Technol 2017;45:34-41. [Crossref] [PubMed]
- van Velthuysen ML, Groen EJ, van der Noort V, et al. Grading of neuroendocrine neoplasms: mitoses and Ki-67 are both essential. Neuroendocrinology 2014;100:221-7. [Crossref] [PubMed]
- Bosman F, Carneiro F, Hruban R, et al. WHO classification of tumours of the digestive system. Lyon: IARC Press, 2010.
- Rindi G, Petrone G, Inzani F. The 2010 WHO classification of digestive neuroendocrine neoplasms: a critical appraisal four years after its introduction. Endocr Pathol 2014;25:186-92. [Crossref] [PubMed]
- Miyoshi T, Umemura S, Matsumura Y, et al. Genomic Profiling of Large-Cell Neuroendocrine Carcinoma of the Lung. Clin Cancer Res 2017;23:757-65. [Crossref] [PubMed]
- Rekhtman N, Pietanza MC, Hellmann MD, et al. Next-Generation Sequencing of Pulmonary Large Cell Neuroendocrine Carcinoma Reveals Small Cell Carcinoma-like and Non-Small Cell Carcinoma-like Subsets. Clin Cancer Res 2016;22:3618-29. [Crossref] [PubMed]
- Fernandez-Cuesta L, McKay JD. Genomic architecture of lung cancers. Curr Opin Oncol 2016;28:52-7. [Crossref] [PubMed]
- Fernandez-Cuesta L, Peifer M, Lu X, et al. Frequent mutations in chromatin-remodelling genes in pulmonary carcinoids. Nat Commun 2014;5:3518. [Crossref] [PubMed]
- George J, Lim JS, Jang SJ, et al. Comprehensive genomic profiles of small cell lung cancer. Nature 2015;524:47-53. [Crossref] [PubMed]
- Iwakawa R, Takenaka M, Kohno T, et al. Genome-wide identification of genes with amplification and/or fusion in small cell lung cancer. Genes Chromosomes Cancer 2013;52:802-16. [Crossref] [PubMed]
- Kunz PL. Carcinoid and neuroendocrine tumors: building on success. J Clin Oncol 2015;33:1855-63. [Crossref] [PubMed]
- Peifer M, Fernandez-Cuesta L, Sos ML, et al. Integrative genome analyses identify key somatic driver mutations of small-cell lung cancer. Nat Genet 2012;44:1104-10. [Crossref] [PubMed]
- Pelosi G, Hiroshima K, Mino-Kenudson M. Controversial issues and new discoveries in lung neuroendocrine tumors. Diagn Histopathol 2014;20:392-7. [Crossref]
- Rudin CM, Durinck S, Stawiski EW, et al. Comprehensive genomic analysis identifies SOX2 as a frequently amplified gene in small-cell lung cancer. Nat Genet 2012;44:1111-6. [Crossref] [PubMed]
- Seidel D, Zander T, Heukamp L, et al. A genomics-based classification of human lung tumors. Sci Transl Med 2013;5:209ra153. [PubMed]
- Swarts DR, Ramaekers FC, Speel EJ. Molecular and cellular biology of neuroendocrine lung tumors: Evidence for separate biological entities. Biochim Biophys Acta 2012;1826:255-71.
- Swarts DR, Scarpa A, Corbo V, et al. MEN1 Gene Mutation and Reduced Expression Are Associated With Poor Prognosis in Pulmonary Carcinoids. J Clin Endocrinol Metab 2014;99:E374-8. [Crossref] [PubMed]
- Swarts DR, Van Neste L, Henfling ME, et al. An exploration of pathways involved in lung carcinoid progression using gene expression profiling. Carcinogenesis 2013;34:2726-37. [Crossref] [PubMed]
- Swarts DR, Henfling ME, Van Neste L, et al. CD44 and OTP are strong prognostic markers for pulmonary carcinoids. Clin Cancer Res 2013;19:2197-207. [Crossref] [PubMed]
- Toffalorio F, Belloni E, Barberis M, et al. Gene expression profiling reveals GC and CEACAM1 as new tools in the diagnosis of lung carcinoids. Br J Cancer 2014;110:1244-9. [Crossref] [PubMed]
- Swarts DR, Claessen SM, Jonkers YM, et al. Deletions of 11q22.3-q25 are associated with atypical lung carcinoids and poor clinical outcome. Am J Pathol 2011;179:1129-37. [Crossref] [PubMed]
- Jones MH, Virtanen C, Honjoh D, et al. Two prognostically significant subtypes of high-grade lung neuroendocrine tumours independent of small-cell and large-cell neuroendocrine carcinomas identified by gene expression profiles. Lancet 2004;363:775-81. [Crossref] [PubMed]
- Pelosi G, Pastorino U, Pasini F, et al. Independent prognostic value of fascin-1 immunoreactivity in stage I non-small cell lung cancer. Br J Cancer 2003;88:537-47. [Crossref] [PubMed]
- Hendifar AE, Marchevsky AM, Tuli R. Neuroendocrine Tumors of the Lung: Current Challenges and Advances in the Diagnosis and Management of Well-Differentiated Disease. J Thorac Oncol 2017;12:425-36. [Crossref] [PubMed]
- Marchevsky AM, Wirtschafter E, Walts AE. The spectrum of changes in adults with multifocal pulmonary neuroendocrine proliferations: what is the minimum set of pathologic criteria to diagnose DIPNECH? Hum Pathol 2015;46:176-81. [Crossref] [PubMed]
- Marchevsky AM, Walts AE. Diffuse idiopathic pulmonary neuroendocrine cell hyperplasia (DIPNECH). Semin Diagn Pathol 2015;32:438-44. [Crossref] [PubMed]
- Rossi G, Cavazza A, Spagnolo P, et al. Diffuse idiopathic pulmonary neuroendocrine cell hyperplasia syndrome. Eur Respir J 2016;47:1829-41. [Crossref] [PubMed]
- Schmitt AM, Blank A, Marinoni I, et al. Histopathology of NET: Current concepts and new developments. Best Pract Res Clin Endocrinol Metab 2016;30:33-43. [Crossref] [PubMed]
- Wick MR, Marchevsky AM. Neuroendocrine neoplasms of the lung: Concepts and terminology. Semin Diagn Pathol 2015;32:445-55. [Crossref] [PubMed]
- Marchevsky AM, Wick MR. Diagnostic difficulties with the diagnosis of small cell carcinoma of the lung. Semin Diagn Pathol 2015;32:480-8. [Crossref] [PubMed]
- Thunnissen E, Borczuk AC, Flieder DB, et al. The Use of Immunohistochemistry Improves the Diagnosis of Small Cell Lung Cancer and Its Differential Diagnosis. An International Reproducibility Study in a Demanding Set of Cases. J Thorac Oncol 2017;12:334-46. [Crossref] [PubMed]
- den Bakker MA, Thunnissen FB. Neuroendocrine tumours--challenges in the diagnosis and classification of pulmonary neuroendocrine tumours. J Clin Pathol 2013;66:862-9. [Crossref] [PubMed]
- den Bakker MA, Willemsen S, Grunberg K, et al. Small cell carcinoma of the lung and large cell neuroendocrine carcinoma interobserver variability. Histopathology 2010;56:356-63. [Crossref] [PubMed]
- Franks TJ, Galvin JR. Lung tumors with neuroendocrine morphology: essential radiologic and pathologic features. Arch Pathol Lab Med 2008;132:1055-61. [PubMed]
- Marchevsky AM, Gal AA, Shah S, et al. Morphometry confirms the presence of considerable nuclear size overlap between "small cells" and "large cells" in high-grade pulmonary neuroendocrine neoplasms. Am J Clin Pathol 2001;116:466-72. [Crossref] [PubMed]
- Rekhtman N. Neuroendocrine tumors of the lung: an update. Arch Pathol Lab Med 2010;134:1628-38. [PubMed]
- Rossi G, Bisagni A, Cavazza A. High-grade neuroendocrine carcinoma. Curr Opin Pulm Med 2014;20:332-9. [Crossref] [PubMed]
- Ejaz S, Vassilopoulou-Sellin R, Busaidy NL, et al. Cushing syndrome secondary to ectopic adrenocorticotropic hormone secretion: the University of Texas MD Anderson Cancer Center Experience. Cancer 2011;117:4381-9. [Crossref] [PubMed]
- Travis WD. Advances in neuroendocrine lung tumors. Ann Oncol 2010;21 Suppl 7:vii65-71. [Crossref] [PubMed]
- D'Amico M A, Ghinassi B, Izzicupo P, et al. Biological function and clinical relevance of chromogranin A and derived peptides. Endocr Connect 2014;3:R45-54. [Crossref] [PubMed]
- Kwon SE, Chapman ER. Synaptophysin regulates the kinetics of synaptic vesicle endocytosis in central neurons. Neuron 2011;70:847-54. [Crossref] [PubMed]
- Miki M, Ball DW, Linnoila RI. Insights into the achaete-scute homolog-1 gene (hASH1) in normal and neoplastic human lung. Lung Cancer 2012;75:58-65. [Crossref] [PubMed]
- Ye B, Cappel J, Findeis-Hosey J, et al. hASH1 is a specific immunohistochemical marker for lung neuroendocrine tumors. Hum Pathol 2016;48:142-7. [Crossref] [PubMed]
- Lantuejoul S, Moro D, Michalides RJ, et al. Neural cell adhesion molecules (NCAM) and NCAM-PSA expression in neuroendocrine lung tumors. Am J Surg Pathol 1998;22:1267-76. [Crossref] [PubMed]
- Teicher BA. Targets in small cell lung cancer. Biochem Pharmacol 2014;87:211-9. [Crossref] [PubMed]
- Feng J, Sheng H, Zhu C, et al. Correlation of neuroendocrine features with prognosis of non-small cell lung cancer. Oncotarget 2016;7:71727-36. [Crossref] [PubMed]
- Agaimy A, Wunsch PH. Distribution of neural cell adhesion molecule (NCAM/CD56) in gastrointestinal stromal tumours and their intra-abdominal mesenchymal mimics. J Clin Pathol 2008;61:499-503. [Crossref] [PubMed]
- Olsen SH, Thomas DG, Lucas DR. Cluster analysis of immunohistochemical profiles in synovial sarcoma, malignant peripheral nerve sheath tumor, and Ewing sarcoma. Mod Pathol 2006;19:659-68. [Crossref] [PubMed]
- Lantuéjoul S, Laverriere MH, Sturm N, et al. NCAM (neural cell adhesion molecules) expression in malignant mesotheliomas. Hum Pathol 2000;31:415-21. [Crossref] [PubMed]
- Sturm N, Lantuejoul S, Laverriere MH, et al. Thyroid transcription factor 1 and cytokeratins 1, 5, 10, 14 (34betaE12) expression in basaloid and large-cell neuroendocrine carcinomas of the lung. Hum Pathol 2001;32:918-25. [Crossref] [PubMed]
- Pelosi G, Rossi G, Cavazza A, et al. DeltaNp63 (p40) distribution inside lung cancer: a driver biomarker approach to tumor characterization. Int J Surg Pathol 2013;21:229-39. [Crossref] [PubMed]
- Fujino K, Motooka Y, Hassan WA, et al. Insulinoma-Associated Protein 1 Is a Crucial Regulator of Neuroendocrine Differentiation in Lung Cancer. Am J Pathol 2015;185:3164-77. [Crossref] [PubMed]
- Jia S, Wildner H, Birchmeier C. Insm1 controls the differentiation of pulmonary neuroendocrine cells by repressing Hes1. Dev Biol 2015;408:90-8. [Crossref] [PubMed]
- Pelosi G, Scarpa A, Forest F, et al. The impact of immunohistochemistry on the classification of lung tumors. Expert Rev Respir Med 2016;10:1105-21. [Crossref] [PubMed]
- Stathis A, Zucca E, Bekradda M, et al. Clinical Response of Carcinomas Harboring the BRD4-NUT Oncoprotein to the Targeted Bromodomain Inhibitor OTX015/MK-8628. Cancer Discov 2016;6:492-500. [Crossref] [PubMed]
- Weissferdt A, Moran CA. Primary pulmonary primitive neuroectodermal tumor (PNET): a clinicopathological and immunohistochemical study of six cases. Lung 2012;190:677-83. [Crossref] [PubMed]
- Parkash V, Gerald WL, Parma A, et al. Desmoplastic small round cell tumor of the pleura. Am J Surg Pathol 1995;19:659-65. [Crossref] [PubMed]
- Marx A, Chan JK, Coindre JM, et al. The 2015 World Health Organization Classification of Tumors of the Thymus: Continuity and Changes. J Thorac Oncol 2015;10:1383-95. [Crossref] [PubMed]
- Ferolla P. Medical Therapy of Pulmonary Neuroendocrine Neoplasms: Targeted, Symptomatic and Chemotherapy. Front Horm Res 2015;44:193-7. [Crossref] [PubMed]
- Kamp K, Alwani RA, Korpershoek E, et al. Prevalence and clinical features of the ectopic ACTH syndrome in patients with gastroenteropancreatic and thoracic neuroendocrine tumors. Eur J Endocrinol 2016;174:271-80. [Crossref] [PubMed]
- Ströbel P, Zettl A, Shilo K, et al. Tumor genetics and survival of thymic neuroendocrine neoplasms: a multi-institutional clinicopathologic study. Genes Chromosomes Cancer 2014;53:738-49. [Crossref] [PubMed]
- Weissferdt A, Kalhor N, Liu H, et al. Thymic neuroendocrine tumors (paraganglioma and carcinoid tumors): a comparative immunohistochemical study of 46 cases. Hum Pathol 2014;45:2463-70. [Crossref] [PubMed]
- Nonaka D, Papaxoinis G, Mansoor W. Diagnostic Utility of Orthopedia Homeobox (OTP) in Pulmonary Carcinoid Tumors. Am J Surg Pathol 2016;40:738-44. [Crossref] [PubMed]
- Matoso A, Singh K, Jacob R, et al. Comparison of thyroid transcription factor-1 expression by 2 monoclonal antibodies in pulmonary and nonpulmonary primary tumors. Appl Immunohistochem Mol Morphol 2010;18:142-9. [Crossref] [PubMed]
- Zhang C, Schmidt LA, Hatanaka K, et al. Evaluation of napsin A, TTF-1, p63, p40, and CK5/6 immunohistochemical stains in pulmonary neuroendocrine tumors. Am J Clin Pathol 2014;142:320-4. [Crossref] [PubMed]
- Sturm N, Rossi G, Lantuejoul S, et al. Expression of thyroid transcription factor-1 in the spectrum of neuroendocrine cell lung proliferations with special interest in carcinoids. Hum Pathol 2002;33:175-82. [Crossref] [PubMed]
- Weissferdt A, Tang X, Wistuba II, et al. Comparative immunohistochemical analysis of pulmonary and thymic neuroendocrine carcinomas using PAX8 and TTF-1. Mod Pathol 2013;26:1554-60. [Crossref] [PubMed]
- Kaufmann O, Dietel M. Expression of thyroid transcription factor-1 in pulmonary and extrapulmonary small cell carcinomas and other neuroendocrine carcinomas of various primary sites. Histopathology 2000;36:415-20. [Crossref] [PubMed]
- Graham RP, Shrestha B, Caron BL, et al. Islet-1 is a sensitive but not entirely specific marker for pancreatic neuroendocrine neoplasms and their metastases. Am J Surg Pathol 2013;37:399-405. [Crossref] [PubMed]
- Quinn AM, Blackhall F, Wilson G, et al. Extrapulmonary small cell carcinoma: a clinicopathological study with identification of potential diagnostic mimics. Histopathology 2012;61:454-64. [Crossref] [PubMed]
- Koo J, Mertens RB, Mirocha JM, et al. Value of Islet 1 and PAX8 in identifying metastatic neuroendocrine tumors of pancreatic origin. Mod Pathol 2012;25:893-901. [Crossref] [PubMed]
- Yang Z, Klimstra DS, Hruban RH, et al. Immunohistochemical Characterization of the Origins of Metastatic Well-differentiated Neuroendocrine Tumors to the Liver. Am J Surg Pathol 2017;41:915-22. [Crossref] [PubMed]
- Agaimy A, Erlenbach-Wunsch K, Konukiewitz B, et al. ISL1 expression is not restricted to pancreatic well-differentiated neuroendocrine neoplasms, but is also commonly found in well and poorly differentiated neuroendocrine neoplasms of extrapancreatic origin. Mod Pathol 2013;26:995-1003. [Crossref] [PubMed]
- Pelosi G, Masullo M, Leon ME, et al. CD117 immunoreactivity in high-grade neuroendocrine tumors of the lung: a comparative study of 39 large-cell neuroendocrine carcinomas and 27 surgically resected small-cell carcinomas. Virchows Arch 2004;445:449-55. [Crossref] [PubMed]
- Ventura L, Gnetti L, Silini EM, et al. Primary atypical carcinoid tumor of the mediastinum: a very rare finding. J Thorac Dis 2017;9:E367-72. [Crossref] [PubMed]
- Pelosi G, Barisella M, Pasini F, et al. CD117 immunoreactivity in stage I adenocarcinoma and squamous cell carcinoma of the lung: relevance to prognosis in a subset of adenocarcinoma patients. Mod Pathol 2004;17:711-21. [Crossref] [PubMed]
- Thomas de Montpréville V, Ghigna MR, Lacroix L, et al. Thymic carcinomas: clinicopathologic study of 37 cases from a single institution. Virchows Arch 2013;462:307-13. [Crossref] [PubMed]
- Kriegsmann M, Muley T, Harms A, et al. Differential diagnostic value of CD5 and CD117 expression in thoracic tumors: a large scale study of 1465 non-small cell lung cancer cases. Diagn Pathol 2015;10:210. [Crossref] [PubMed]
- La Rosa S, Sessa F, Uccella S. Mixed Neuroendocrine-Nonneuroendocrine Neoplasms (MiNENs): Unifying the Concept of a Heterogeneous Group of Neoplasms. Endocr Pathol 2016;27:284-311. [Crossref] [PubMed]
- Volante M, Monica V, Birocco N, et al. Expression analysis of genes involved in DNA repair or synthesis in mixed neuroendocrine/nonneuroendocrine carcinomas. Neuroendocrinology 2015;101:151-60. [Crossref] [PubMed]
- Pelosi G, Rindi G, Travis WD, et al. Ki-67 antigen in lung neuroendocrine tumors: unraveling a role in clinical practice. J Thorac Oncol 2014;9:273-84. [Crossref] [PubMed]
- Gerdes J, Lemke H, Baisch H, et al. Cell cycle analysis of a cell proliferation-associated human nuclear antigen defined by the monoclonal antibody Ki-67. J Immunol 1984;133:1710-5. [PubMed]
- Gerdes J, Li L, Schlueter C, et al. Immunobiochemical and molecular biologic characterization of the cell proliferation-associated nuclear antigen that is defined by monoclonal antibody Ki-67. Am J Pathol 1991;138:867-73. [PubMed]
- Gerdes J, Schwab U, Lemke H, et al. Production of a mouse monoclonal antibody reactive with a human nuclear antigen associated with cell proliferation. Int J Cancer 1983;31:13-20. [Crossref] [PubMed]
- Pelosi G, Zamboni G. Proliferation Markers and Their Uses in the Study of Endocrine Tumors. Endocr Pathol 1996;7:103-19. [Crossref] [PubMed]
- Pelosi G, Bresaola E, Bogina G, et al. Endocrine tumors of the pancreas: Ki-67 immunoreactivity on paraffin sections is an independent predictor for malignancy: a comparative study with proliferating-cell nuclear antigen and progesterone receptor protein immunostaining, mitotic index, and other clinicopathologic variables. Hum Pathol 1996;27:1124-34. [Crossref] [PubMed]
- Pelosi G, Zamboni G, Doglioni C, et al. Immunodetection of proliferating cell nuclear antigen assesses the growth fraction and predicts malignancy in endocrine tumors of the pancreas. Am J Surg Pathol 1992;16:1215-25. [Crossref] [PubMed]
- Tang LH, Gonen M, Hedvat C, et al. Objective quantification of the Ki67 proliferative index in neuroendocrine tumors of the gastroenteropancreatic system: a comparison of digital image analysis with manual methods. Am J Surg Pathol 2012;36:1761-70. [Crossref] [PubMed]
- Remes SM, Tuominen VJ, Helin H, et al. Grading of neuroendocrine tumors with Ki-67 requires high-quality assessment practices. Am J Surg Pathol 2012;36:1359-63. [Crossref] [PubMed]
- Reid MD, Bagci P, Ohike N, et al. Calculation of the Ki67 index in pancreatic neuroendocrine tumors: a comparative analysis of four counting methodologies. Mod Pathol 2015;28:686-94. [Crossref] [PubMed]
- Yang Z, Tang LH, Klimstra DS. Effect of tumor heterogeneity on the assessment of Ki67 labeling index in well-differentiated neuroendocrine tumors metastatic to the liver: implications for prognostic stratification. Am J Surg Pathol 2011;35:853-60. [Crossref] [PubMed]
- Travis W, Brambilla E, Muller-Hermelink H, et al. Tumours of the lung, pleura, thymus and heart. World Health Organization Classification of Tumours. Lyon: IARC Press, 2004.
- Travis W, Colby T, Corrin B, et al. Hystological typing of lung and pleural tumours. International histological classification of tumours. Berlin Heidelberg New York: Springer Verlag, 1999.
- Avilés-Salas A, Muniz-Hernandez S, Maldonado-Martinez HA, et al. Reproducibility of the EGFR immunohistochemistry scores for tumor samples from patients with advanced non-small cell lung cancer. Oncol Lett 2017;13:912-20. [PubMed]
- Al Diffalha S, Shaar M, Barkan GA, et al. Immunohistochemistry in the workup of prostate biopsies: Frequency, variation and appropriateness of use among pathologists practicing at an academic center. Ann Diagn Pathol 2017;27:34-42. [Crossref] [PubMed]
- Swarts DR, van Suylen RJ, den Bakker MA, et al. Interobserver variability for the WHO classification of pulmonary carcinoids. Am J Surg Pathol 2014;38:1429-36. [Crossref] [PubMed]
- Yang Z, Tang LH, Klimstra DS. Gastroenteropancreatic neuroendocrine neoplasms: historical context and current issues. Semin Diagn Pathol 2013;30:186-96. [Crossref] [PubMed]
- Walts AE, Ines D, Marchevsky AM. Limited role of Ki-67 proliferative index in predicting overall short-term survival in patients with typical and atypical pulmonary carcinoid tumors. Mod Pathol 2012;25:1258-64. [Crossref] [PubMed]
- Warth A, Fink L, Fisseler-Eckhoff A, et al. Interobserver agreement of proliferation index (Ki-67) outperforms mitotic count in pulmonary carcinoids. Virchows Arch 2013;462:507-13. [Crossref] [PubMed]
- Granberg D, Wilander E, Oberg K, et al. Prognostic markers in patients with typical bronchial carcinoid tumors. J Clin Endocrinol Metab 2000;85:3425-30. [PubMed]
- Swarts DR, Rudelius M, Claessen SM, et al. Limited additive value of the Ki-67 proliferative index on patient survival in World Health Organization-classified pulmonary carcinoids. Histopathology 2017;70:412-22. [Crossref] [PubMed]
- Rugge M, Fassan M, Clemente R, et al. Bronchopulmonary carcinoid: phenotype and long-term outcome in a single-institution series of Italian patients. Clin Cancer Res 2008;14:149-54. [Crossref] [PubMed]
- Grimaldi F, Muser D, Beltrami CA, et al. Partitioning of bronchopulmonary carcinoids in two different prognostic categories by ki-67 score. Front Endocrinol (Lausanne) 2011;2:20. [Crossref] [PubMed]
- Derks JL, Speel EJ, Thunnissen E, et al. Neuroendocrine Cancer of the Lung: A Diagnostic Puzzle. J Thorac Oncol 2016;11:e35-8. [Crossref] [PubMed]
- Ha SY, Han J, Kim WS, et al. Interobserver variability in diagnosing high-grade neuroendocrine carcinoma of the lung and comparing it with the morphometric analysis. Korean J Pathol 2012;46:42-7. [Crossref] [PubMed]
- Marchiò C, Gatti G, Massa F, et al. Distinctive pathological and clinical features of lung carcinoids with high proliferation index. Virchows Arch 2017. [Epub ahead of print]. [Crossref] [PubMed]
- Travis WD, Brambilla E, Noguchi M, et al. Diagnosis of lung cancer in small biopsies and cytology: implications of the 2011 International Association for the Study of Lung Cancer/American Thoracic Society/European Respiratory Society classification. Arch Pathol Lab Med 2013;137:668-84. [Crossref] [PubMed]
- Fazio N, Granberg D, Grossman A, et al. Everolimus plus octreotide long-acting repeatable in patients with advanced lung neuroendocrine tumors: analysis of the phase 3, randomized, placebo-controlled RADIANT-2 study. Chest 2013;143:955-62. [Crossref] [PubMed]
- Yao JC, Fazio N, Singh S, et al. Everolimus for the treatment of advanced, non-functional neuroendocrine tumours of the lung or gastrointestinal tract (RADIANT-4): a randomised, placebo-controlled, phase 3 study. Lancet 2016;387:968-77. [Crossref] [PubMed]
- Rinke A, Gress TM. Neuroendocrine Cancer, Therapeutic Strategies in G3 Cancers. Digestion 2017;95:109-14. [Crossref] [PubMed]
- Gridelli C, Rossi A, Airoma G, et al. Treatment of pulmonary neuroendocrine tumours: State of the art and future developments. Cancer Treat Rev 2013;39:466-72. [Crossref] [PubMed]
- Pusceddu S, Lo Russo G, Macerelli M, et al. Diagnosis and management of typical and atypical lung carcinoids. Crit Rev Oncol Hematol 2016;100:167-76. [Crossref] [PubMed]
- Pelosi G, Papotti M, Rindi G, et al. Unraveling Tumor Grading and Genomic Landscape in Lung Neuroendocrine Tumors. Endocr Pathol 2014;25:151-64. [Crossref] [PubMed]
- Francis JM, Kiezun A, Ramos AH, et al. Somatic mutation of CDKN1B in small intestine neuroendocrine tumors. Nat Genet 2013;45:1483-6. [Crossref] [PubMed]
- Valente R, Hayes AJ, Haugvik SP, et al. Risk and Protective Factors for the Occurrence of Sporadic Pancreatic Endocrine Neoplasms. Endocr Relat Cancer 2017;24:405-14. [Crossref] [PubMed]
- Rinzivillo M, Capurso G, Campana D, et al. Risk and Protective Factors for Small Intestine Neuroendocrine Tumors: A Prospective Case-Control Study. Neuroendocrinology 2016;103:531-7. [Crossref] [PubMed]
- Papadopoulos A, Guida F, Leffondre K, et al. Heavy smoking and lung cancer: Are women at higher risk? Result of the ICARE study. Br J Cancer 2014;110:1385-91. [Crossref] [PubMed]
- Beasley MB, Lantuejoul S, Abbondanzo S, et al. The P16/cyclin D1/Rb pathway in neuroendocrine tumors of the lung. Hum Pathol 2003;34:136-42. [Crossref] [PubMed]
- Debelenko LV, Swalwell JI, Kelley MJ, et al. MEN1 gene mutation analysis of high-grade neuroendocrine lung carcinoma. Genes Chromosomes Cancer 2000;28:58-65. [Crossref] [PubMed]
- Pleasance ED, Stephens PJ, O'Meara S, et al. A small-cell lung cancer genome with complex signatures of tobacco exposure. Nature 2010;463:184-90. [Crossref] [PubMed]
- Coe BP, Lee EH, Chi B, et al. Gain of a region on 7p22.3, containing MAD1L1, is the most frequent event in small-cell lung cancer cell lines. Genes Chromosomes Cancer 2006;45:11-9. [Crossref] [PubMed]
- Sakre N, Wildey G, Behtaj M, et al. RICTOR amplification identifies a subgroup in small cell lung cancer and predicts response to drugs targeting mTOR. Oncotarget 2017;8:5992-6002. [PubMed]
- Voortman J, Lee JH, Killian JK, et al. Array comparative genomic hybridization-based characterization of genetic alterations in pulmonary neuroendocrine tumors. Proc Natl Acad Sci U S A 2010;107:13040-5. [Crossref] [PubMed]
- Meder L, Konig K, Ozretic L, et al. NOTCH, ASCL1, p53 and RB alterations define an alternative pathway driving neuroendocrine and small cell lung carcinomas. Int J Cancer 2016;138:927-38. [Crossref] [PubMed]
- Pelosi G, Pasini F, Fraggetta F, et al. Independent value of fascin immunoreactivity for predicting lymph node metastases in typical and atypical pulmonary carcinoids. Lung Cancer 2003;42:203-13. [Crossref] [PubMed]
- Pelosi G, Scarpa A, Puppa G, et al. Alteration of the E-cadherin/beta-catenin cell adhesion system is common in pulmonary neuroendocrine tumors and is an independent predictor of lymph node metastasis in atypical carcinoids. Cancer 2005;103:1154-64. [Crossref] [PubMed]
- Pelosi G, Scarpa A, Veronesi G, et al. A subset of high-grade pulmonary neuroendocrine carcinomas shows up-regulation of matrix metalloproteinase-7 associated with nuclear beta-catenin immunoreactivity, independent of EGFR and HER-2 gene amplification or expression. Virchows Arch 2005;447:969-77. [Crossref] [PubMed]
- Papaxoinis G, Nonaka D, O'Brien C, et al. Prognostic Significance of CD44 and Orthopedia Homeobox Protein (OTP) Expression in Pulmonary Carcinoid Tumours. Endocr Pathol 2017;28:60-70. [Crossref] [PubMed]
- Rosai J, Higa E, Davie J. Mediastinal endocrine neoplasm in patients with multiple endocrine adenomatosis. A previously unrecognized association. Cancer 1972;29:1075-83. [Crossref] [PubMed]
- Gibril F, Chen YJ, Schrump DS, et al. Prospective study of thymic carcinoids in patients with multiple endocrine neoplasia type 1. J Clin Endocrinol Metab 2003;88:1066-81. [Crossref] [PubMed]
- Sakurai A, Imai T, Kikumori T, et al. Thymic neuroendocrine tumour in multiple endocrine neoplasia type 1: female patients are not rare exceptions. Clin Endocrinol (Oxf) 2013;78:248-54. [Crossref] [PubMed]
- Sakurai A, Suzuki S, Kosugi S, et al. Multiple endocrine neoplasia type 1 in Japan: establishment and analysis of a multicentre database. Clin Endocrinol (Oxf) 2012;76:533-9. [Crossref] [PubMed]
- Christakis I, Qiu W, Silva Figueroa AM, et al. Clinical Features, Treatments, and Outcomes of Patients with Thymic Carcinoids and Multiple Endocrine Neoplasia Type 1 Syndrome at MD Anderson Cancer Center. Horm Cancer 2016;7:279-87. [Crossref] [PubMed]
- Pieterman CR, Conemans EB, Dreijerink KM, et al. Thoracic and duodenopancreatic neuroendocrine tumors in multiple endocrine neoplasia type 1: natural history and function of menin in tumorigenesis. Endocr Relat Cancer 2014;21:R121-42. [Crossref] [PubMed]
- Pan CC, Jong YJ, Chen YJ. Comparative genomic hybridization analysis of thymic neuroendocrine tumors. Mod Pathol 2005;18:358-64. [Crossref] [PubMed]
- Rieker RJ, Aulmann S, Penzel R, et al. Chromosomal imbalances in sporadic neuroendocrine tumours of the thymus. Cancer Lett 2005;223:169-74. [Crossref] [PubMed]
- Goto K, Kodama T, Matsuno Y, et al. Clinicopathologic and DNA cytometric analysis of carcinoid tumors of the thymus. Mod Pathol 2001;14:985-94. [Crossref] [PubMed]
- Quinn AM, Chaturvedi A, Nonaka D. High-grade Neuroendocrine Carcinoma of the Lung With Carcinoid Morphology: A Study of 12 Cases. Am J Surg Pathol 2017;41:263-70. [Crossref] [PubMed]
- Moran CA, Suster S. Thymic neuroendocrine carcinomas with combined features ranging from well-differentiated (carcinoid) to small cell carcinoma. A clinicopathologic and immunohistochemical study of 11 cases. Am J Clin Pathol 2000;113:345-50. [Crossref] [PubMed]
- Wick MR, Scheithauer BW. Oat-cell carcinoma of the thymus. Cancer 1982;49:1652-7. [Crossref] [PubMed]
- Scholz DA, Bahn RC. Thymic tumors associated with Cushing's syndrome: review of three cases. Proc Staff Meet Mayo Clin 1959;34:433-41. [PubMed]
- Kuo TT. Carcinoid tumor of the thymus with divergent sarcomatoid differentiation: report of a case with histogenetic consideration. Hum Pathol 1994;25:319-23. [Crossref] [PubMed]
- Sensaki K, Aida S, Takagi K, et al. Coexisting undifferentiated thymic carcinoma and thymic carcinoid tumor. Respiration 1993;60:247-9. [Crossref] [PubMed]
- Paties C, Zangrandi A, Vassallo G, et al. Multidirectional carcinoma of the thymus with neuroendocrine and sarcomatoid components and carcinoid syndrome. Pathol Res Pract 1991;187:170-7. [Crossref] [PubMed]
- Desmeules P, Sabari J, Santos-Zabala ML, et al. Metastatic lung carcinoid tumors: evidence of Proliferation rate progression at metastatic sites (Abstract #1911). Mod Pathol 2017;30:475A.
- Tang LH, Basturk O, Sue JJ, et al. A Practical Approach to the Classification of WHO Grade 3 (G3) Well-differentiated Neuroendocrine Tumor (WD-NET) and Poorly Differentiated Neuroendocrine Carcinoma (PD-NEC) of the Pancreas. Am J Surg Pathol 2016;40:1192-202. [Crossref] [PubMed]
- Tang LH, Untch BR, Reidy DL, et al. Well-Differentiated Neuroendocrine Tumors with a Morphologically Apparent High-Grade Component: A Pathway Distinct from Poorly Differentiated Neuroendocrine Carcinomas. Clin Cancer Res 2016;22:1011-7. [Crossref] [PubMed]
- Aldape K, Zadeh G, Mansouri S, et al. Glioblastoma: pathology, molecular mechanisms and markers. Acta Neuropathol 2015;129:829-48. [Crossref] [PubMed]
- Ohgaki H, Burger P, Kleihues P. Definition of primary and secondary glioblastoma--response. Clin Cancer Res 2014;20:2013. [Crossref] [PubMed]
- Fusco N, Geyer FC, De Filippo MR, et al. Genetic events in the progression of adenoid cystic carcinoma of the breast to high-grade triple-negative breast cancer. Mod Pathol 2016;29:1292-305. [Crossref] [PubMed]