Hippo/YAP pathway for targeted therapy
Introduction
The Hippo signaling pathway controls organ size through the regulation of cell cycle, proliferation and apoptosis (1,2). It was discovered and linked for the first time to human cancer in 2002 (3). In this review we will briefly introduce this pathway, with an emphasis on components that are altered in human cancers, and then we will focus on its role in malignant pleural mesothelioma (MPM) and finally present potential implication for the therapy of MPM.
The Hippo/Yes-associated protein (YAP) pathway in cancer
The mammalian components of Hippo pathway (Figure 1) include Serine/threonine kinase 3 and 4 (STK3 and 4, also called MST2 and 1, orthologs of Drosophila Hippo), SAV1, and serine/threonine kinase large tumor suppressor 1 and 2 (LATS1 and 2) (4). Neurofibromin 2 (NF2) (product of NF2 gene), also called merlin, a member of the Ezrin ezrin/radixin/moesin protein family, promotes plasma membrane association of LATS which results in phosphorylation and activation of LATS1/2 by MST and other not yet known kinases (5). Activation of LATS inhibits the transcriptional co-activator YAP and the co-activator with PDZ-binding motif (TAZ) through their phosphorylation. Indeed, phosphorylated YAP/TAZ cannot accumulate into the nucleus and this hinders their co-transcriptional activity. The dysfunction of Hippo pathway, which leads to increased YAP/TAZ activity with an underphosphorylated form in the nucleus (6), induces oncogenic transformation due to the activation of transcription factors including transcription enhancers activation domain (TEAD) family members (7). In mammals, there are four TEAD family members: TEAD1-4 which have a distinct but not mutually exclusive expression pattern (8). TEAD on its own is unable to induce gene expression and requires additional factors or co-activators for gene expression (8). Upon binding TEADs YAP/TAZ up-regulates the expression of several growth promoting factors, including secretory proteins connective tissue growth factor (CTGF) and Cyr61 (7), AXL receptor tyrosine kinase (9), c-myc and survivin (10,11).
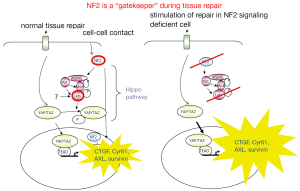
Amplification of YAP-containing chromosome 11q22 amplicon is frequently observed in several human tumors. High levels of YAP are observed in human liver tumors and YAP is a key driver of hepatocellular carcinoma tumorigenesis (12,13). High YAP levels are also seen in about 15% of ovarian cancers where it has been correlated with poor patient prognosis (14). Similarly, a correlation between high YAP expression and poor prognosis has been identified in non-small cell lung cancer and esophageal squamous cell carcinoma (15,16). YAP overexpression is also seen in medulloblastoma (17) intracranial ependymoma (18) and oral squamous cell carcinoma (19). Also, YAP plays a role in human colorectal cancer progression (20). Mutations in Mst1, Mst2, LATS1 and LATS2 are not common but epigenetic silencing of these genes has been reported (21-23).
Hippo pathway activity may also be altered though crosstalk with other signalling pathways, which harbour oncogenic alteration [reviewed in (24)]. The Hippo pathway has been implicated in cell contact inhibition, as YAP/TAZ display a dramatic cell density dependent subcellular localization and phosphorylation (25). In addition, mechanic stress has also been shown to modulate YAP/TAZ activity (26). Recent studies have shown that YAP activity is regulated by G-protein-coupled receptor signaling (27,28). In this context it is of particular interest the activation of YAP/TAZ by thrombin and protease-activated receptor PAR1 (29), since thrombin is generated at sites of tissue injury to promote wound healing. In addition inhibition of mevalonate pathway with simvastatin decreases nuclear YAP (30).
The interaction with sonic hedgehog stem signalling pathway in mesothelioma will be detailed below.
The Hippo/YAP pathway in MPM
MPM is an aggressive human malignancy (31). MPM is mostly associated with asbestos exposure and the latency period after initial exposure is typically longer than 30 years (32). MPM is a rare disease with a 15-year cumulative frequency during 1994-2008 in the 56 countries reporting MPM to be 174,300 (33); however the real incidence of MPM is unknown, since there are countries in which MPM mortality is not reported, including asbestos-producing countries such as Russia, Kazakhstan, China and India (33). MPM mortality rates are estimated to increase by 5-10% per year in most industrialized countries until about 2020 (34). Despite treatment with chemotherapy, radiation therapy or surgery, the disease carries a poor prognosis. The median survival time of patients after diagnosis is only 7-12 months (35).
The mechanism of development of MPM after exposure to asbestos fibres is not well understood. Few hypotheses can be proposed based on experimental data and observation of clinical samples, some of which has been detailed in several reviews (36-42). Chronic tissue repair activates stem cell signalling pathways to regenerate the tissue but, because of persistent system stimulation, oncogenic events occur leading to the formation of a tumor (43). Cells that are stimulated to proliferate upon asbestos fibres exposure may be undifferentiated precursor cells, as it has been shown for mesothelial regeneration after injury (44). Undifferentiated precursor cells have been recently described in normal mesothelial primary cultures (45). The activation of stem cell signalling would normally be kept under control, but, because of persistent system stimulation, oncogenic events occur leading to the formation of a tumor (43). In line with such hypothesis one would expect the oncogenic events to occur within the components responsible for homeostasis in tissue repair and in control of stem cell signalling. Activated stem cell signalling has already been suggested in MPM by the presence of an 11-gene signature, correlated with a stem-cell-like expression profile, which is associated with a poor prognosis in patients with MPM (46). Knowledge about common alterations observed in MPM, which are detailed below, confirms that alteration in NF2 signalling, which is responsible for homeostasis in tissue repair and in the control of stem cell signalling, is the ideal target for an oncogenic event to occur during the development of MPM. This hypothesis is supported by the observation that Hippo pathway restricts the oncogenic potential of intestinal regeneration program induced after injury by dextran sodium sulfate (47).
Data mining of version 68 of the catalogue of somatic mutations in cancer (COSMIC, http://www.sanger.ac.uk/cosmic) (48) reveals that the genes that are mostly mutated in MPM (Figure 2) are cyclin-dependent kinase activator inhibitor (CDKN2A), neurofibromatosis type 2 (NF2) and BRCA-associated protein 1 (BAP1). Although the total number of samples (<500, status February 2014) screened is too low to confidently predict mutation frequencies this estimate is nevertheless useful to infer a general MPM profile. CDKN2A and NF2 are the two most abundantly mutated genes in MPM. Indeed, MPM lack expression of both CDKN2A encoded proteins p16 and ARF (49,50) due to gene deletion (51-53) or methylation (54-56). Mutations in NF2 gene have been found in about 40% of mesothelioma (57-59). In MPM tumors with no detectable genetic alterations of NF2, its activity is downregulated. Indeed, the activity of NF2 is controlled by phosphorylation (Figure 3), which depending on the phosphorylated amino acid, leads to functional inactivation (60,61) or AKT-dependent degradation (62). Experimental animal models indicate that disruption of the NF2 signalling pathway, together with a deficiency in CDKN2A, is essential for mesothelioma development (63-65). NF2 is an upstream regulator of the Hippo signaling cascade, which is conserved from Drosophila to mammalians (10). The NF2/Hippo connection is supported by mouse genetics, wherein heterozygosity of Yap greatly suppresses the Nf2-deficient phenotype (66).
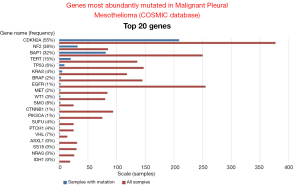
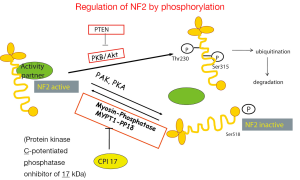
It has been proposed that genes which are inactivated in a given tumor type and directly regulate tumor growth by either inhibiting growth or promoting death are “gatekeeper” genes (67). According to the data mentioned above, NF2 does correspond to this definition and should be considered a “gatekeeper” in mesothelioma. Indeed, NF2 mediates contact-dependent inhibition of proliferation by both sensing cell-cell contact and intercepting mitogenic signalling initiated at the plasma membrane (68). In cultured mammalian cells, NF2 inhibits internalization, effector complexing and downstream signalling of activated EGFR upon cell-cell contact. This is consistent with the idea that NF2/merlin normally sequesters EGFR into non-signalling plasma membrane compartments (69). In addition, NF2 is required for the assembly, but not the maintenance, of apico-lateral junctional complexes (70) which means that NF2 loss will be most important when it occurs in dividing cells, for example during tissue repair. Cells that cannot form apico-lateral junctional complexes will be unable to form a well-organized tissue and will be resistant to contact-dependent growth arrest. A role of NF2 in tissue repair is further supported by the observation that the active form of NF2 suppresses tumorigenesis by migrating into the nucleus where it inhibits the E3 ubiquitin ligase CRL4 and through that controls a subset of Hippo pathway target genes (71). This recent observation is consistent with previous evidence of NF2 signaling-dependent activation of the Hippo pathway (72).
Data from the group of Sekido (73) and the group of Ladanyi (74) indicate that NF2/Hippo signalling is disrupted in most MPM. It is important to note that downstream of such a disrupted signalling, TEAD1/YAP1 are activated and YAP/TEAD1 are necessary, but not sufficient (75), for the overexpression of mesothelin. Mesothelin is expressed in normal mesothelium (76) and is a marker of epithelioid mesothelioma (77).
YAP is constitutively active in more than 70% of primary MPM (73), it has been originally described in an inducible transgenic model to be involved in organ size control paralleled by a 30-fold increase in survivin expression (10). A recent study has showed that it controls survivin expression in MPM (78). We have observed that Hedgehog signaling is activated in MPM, consistent with the re-activation of a signalling known to be essential during embryonic mesothelium development (79). Treatment with an inhibitor of Hedgehog pathway (HhAntag) of mesothelioma cells, grown in cell culture conditions favoring stemness, was arresting cells growth and this was accompanied by decreased levels of survivin (80). Survivin is not described as a direct target downstream Hedgehog pathway. Hence, we sought for other transcription activators known to be expressed in MPM and to regulate survivin expression and the most obvious was YAP. We confirmed nuclear expression of YAP in MPM and observed that HhAntag reduced YAP protein levels. Transient transfection of a constitutively active YAP (81) rescued HhAntag-dependent survivin decrease, confirming the interaction between Hedgehog and YAP signaling. Tumor bearing mice were randomized in two groups receiving either solvent or HhAntag. The in vivo HhAntag treatment dosage (38 mg/kg bw, administered twice daily by oral gavage, 5 d/week) was chosen based on therapeutic range reported in the literature (82). HhAntag led to a significant 35% decrease of the tumor volume after the two weeks of treatment. At the end of dosing regimen, animals were euthanized in order to collect tumor tissue for RNA extraction and immunohistochemical analysis. A different expression profile indicating changes in both tumor and stromal tissue were obtained in the two groups. The effect of HhAntag on tumor volumes was also accompanied by a significant 43% decrease in Ki-67 labelling index. Furthermore, consistent with in vitro experiments, we observed a significant 32% decrease in nuclear YAP immunostaining in HhAntag treated tumors. The observation that HhAntag decreases YAP protein is consistent with the role of Hedgehog signaling in maintaining YAP protein stability (17).
More recently, the modulation of YAP by the AJUBA family has been investigated in MPM (83). The mammalian Ajuba family comprises three proteins AJUBA, LIMD1, and WTIP characterized by a so-called, LIM domain. The LIM domain defines a cysteine-rich double zinc finger initially identified in three developmentally important transcription factors, Caenorhabditis elegans Lin-11, rat Isl-1, and C. elegans mec-3, from which the acronym LIM is derived (84). Although in Drosophila the unique AJUBA family member ortholog activates YAP by binding LATS (85), in MPM the three different LIM family members seem not to have all the same YAP-activating properties (83).
Implications for therapy
Under the hypothesis that disruption of NF2 function acts as “driver” in MPM, therapeutic intervention on genes that are normally kept under control by NF2 and the Hippo pathway such as, e.g., survivin, would be a reasonable approach. However, this might not be easy to implement. In the context of MPM harboring mutated NF2/Hippo pathway, cancer cells may be addicted to the activity of YAP. Liu-Chittenden et al. (86) screened a Johns Hopkins Drug Library, a collection of >3,300 drugs, for compounds that could inhibit the transcriptional activity of YAP in vitro. Three compounds related to porphyrin were identified with this assay. One of these, verteporfin, is in clinical use as a photosensitizer in photocoagulation therapy for macular degeneration. Verteporfin was moderately effective at blocking mouse Yap1-overexpression- or loss of Nf2-driven hepatic tumorigenesis. These data suggest the application of these compounds as anticancer therapies independently of their photosensitizing roles.
Downstream G-coupled receptor signalling we mentioned above YAP activation via thrombin/PAR1 (29) and this activation could be relevant in MPM that depend on PAR1 for growth (87). In these cases one potential option for therapy might be PAR-1 antagonists pepducins such as P1pal-12 (88) which is a cell-penetrating peptide derived from the third intracellular loop of PAR-1. Once inserted into the plasma membrane it is delivered to the PAR-1 intracellular surface, thereby interfering with the receptor/G-protein interaction. Lysophosphatidic acid (LPA) stimulates YAP activity (27) and has also been described to stimulate MPM growth (89), offering another opportunity for intervention.
Simvastatin decreases nuclear YAP and induces growth arrest by interfering with protein geranylgeranylation (30). The same mechanism may participate to lovastatin-mediated protective effect against cisplatin in proliferating normal mesothelial cells (90).
Other druggable targets, which might be relevant in other cancer types, have been summarized in two recent reviews (91,92).
In MPM the strategy may depend on upstream signalling: activated stem signalling, thrombin/PAR1 and LPA/LPA receptor are all potentially interesting targets to inhibit in view of interfering with YAP activation. Nevertheless, for the time being, only for Hedgehog stem signalling a direct link with YAP activation has been demonstrated (80).
Acknowledgements
The work in the Laboratory of Molecular Oncology is supported by the Stiftung für Angewandte Krebsforschung, Baugarten Foundation and the Swiss National Science Foundation.
Disclosure: The authors declare no conflict of interest.
References
- Huang J, Wu S, Barrera J, et al. The Hippo signaling pathway coordinately regulates cell proliferation and apoptosis by inactivating Yorkie, the Drosophila Homolog of YAP. Cell 2005;122:421-34. [PubMed]
- Badouel C, Garg A, McNeill H. Herding Hippos: regulating growth in flies and man. Curr Opin Cell Biol 2009;21:837-43. [PubMed]
- Tapon N, Harvey KF, Bell DW, et al. Salvador promotes both cell cycle exit and apoptosis in Drosophila and is mutated in human cancer cell lines. Cell 2002;110:467-78. [PubMed]
- Zhao B, Lei QY, Guan KL. The Hippo-YAP pathway: new connections between regulation of organ size and cancer. Curr Opin Cell Biol 2008;20:638-46. [PubMed]
- Yin F, Yu J, Zheng Y, et al. Spatial organization of hippo signaling at the plasma membrane mediated by the tumor suppressor merlin/NF2. Cell 2013;154:1342-55. [PubMed]
- Striedinger K, VandenBerg SR, Baia GS, et al. The neurofibromatosis 2 tumor suppressor gene product, merlin, regulates human meningioma cell growth by signaling through YAP. Neoplasia 2008;10:1204-12. [PubMed]
- Zhao B, Ye X, Yu J, et al. TEAD mediates YAP-dependent gene induction and growth control. Genes Dev 2008;22:1962-71. [PubMed]
- Pobbati AV, Hong W. Emerging roles of TEAD transcription factors and its coactivators in cancers. Cancer Biol Ther 2013;14:390-8. [PubMed]
- Xu MZ, Chan SW, Liu AM, et al. AXL receptor kinase is a mediator of YAP-dependent oncogenic functions in hepatocellular carcinoma. Oncogene 2011;30:1229-40. [PubMed]
- Dong J, Feldmann G, Huang J, et al. Elucidation of a universal size-control mechanism in Drosophila and mammals. Cell 2007;130:1120-33. [PubMed]
- Lu L, Li Y, Kim SM, et al. Hippo signaling is a potent in vivo growth and tumor suppressor pathway in the mammalian liver. Proc Natl Acad Sci U S A 2010;107:1437-42. [PubMed]
- Zender L, Spector MS, Xue W, et al. Identification and validation of oncogenes in liver cancer using an integrative oncogenomic approach. Cell 2006;125:1253-67. [PubMed]
- Xu MZ, Yao TJ, Lee NP, et al. Yes-associated protein is an independent prognostic marker in hepatocellular carcinoma. Cancer 2009;115:4576-85. [PubMed]
- Zhang X, George J, Deb S, et al. The Hippo pathway transcriptional co-activator, YAP, is an ovarian cancer oncogene. Oncogene 2011;30:2810-22. [PubMed]
- Muramatsu T, Imoto I, Matsui T, et al. YAP is a candidate oncogene for esophageal squamous cell carcinoma. Carcinogenesis 2011;32:389-98. [PubMed]
- Wang Y, Dong Q, Zhang Q, et al. Overexpression of yes-associated protein contributes to progression and poor prognosis of non-small-cell lung cancer. Cancer Sci 2010;101:1279-85. [PubMed]
- Fernandez-L A, Northcott PA, Dalton J, et al. YAP1 is amplified and up-regulated in hedgehog-associated medulloblastomas and mediates Sonic hedgehog-driven neural precursor proliferation. Genes Dev 2009;23:2729-41. [PubMed]
- Modena P, Lualdi E, Facchinetti F, et al. Identification of tumor-specific molecular signatures in intracranial ependymoma and association with clinical characteristics. J Clin Oncol 2006;24:5223-33. [PubMed]
- Snijders AM, Schmidt BL, Fridlyand J, et al. Rare amplicons implicate frequent deregulation of cell fate specification pathways in oral squamous cell carcinoma. Oncogene 2005;24:4232-42. [PubMed]
- Avruch J, Zhou D, Bardeesy N. YAP oncogene overexpression supercharges colon cancer proliferation. Cell Cycle 2012;11:1090-6. [PubMed]
- Seidel C, Schagdarsurengin U, Blumke K, et al. Frequent hypermethylation of MST1 and MST2 in soft tissue sarcoma. Mol Carcinog 2007;46:865-71. [PubMed]
- Jiang Z, Li X, Hu J, et al. Promoter hypermethylation-mediated down-regulation of LATS1 and LATS2 in human astrocytoma. Neurosci Res 2006;56:450-8. [PubMed]
- Takahashi Y, Miyoshi Y, Takahata C, et al. Down-regulation of LATS1 and LATS2 mRNA expression by promoter hypermethylation and its association with biologically aggressive phenotype in human breast cancers. Clin Cancer Res 2005;11:1380-5. [PubMed]
- Harvey KF, Zhang X, Thomas DM. The Hippo pathway and human cancer. Nat Rev Cancer 2013;13:246-57. [PubMed]
- Zhao B, Wei X, Li W, et al. Inactivation of YAP oncoprotein by the Hippo pathway is involved in cell contact inhibition and tissue growth control. Genes Dev 2007;21:2747-61. [PubMed]
- Halder G, Dupont S, Piccolo S. Transduction of mechanical and cytoskeletal cues by YAP and TAZ. Nat Rev Mol Cell Biol 2012;13:591-600. [PubMed]
- Yu FX, Zhao B, Panupinthu N, et al. Regulation of the Hippo-YAP pathway by G-protein-coupled receptor signaling. Cell 2012;150:780-91. [PubMed]
- Yu FX, Zhang Y, Park HW, et al. Protein kinase A activates the Hippo pathway to modulate cell proliferation and differentiation. Genes Dev 2013;27:1223-32. [PubMed]
- Mo JS, Yu FX, Gong R, et al. Regulation of the Hippo-YAP pathway by protease-activated receptors (PARs). Genes Dev 2012;26:2138-43. [PubMed]
- Wang Z, Wu Y, Wang H, et al. Interplay of mevalonate and Hippo pathways regulates RHAMM transcription via YAP to modulate breast cancer cell motility. Proc Natl Acad Sci U S A 2014;111:E89-98. [PubMed]
- Carbone M, Yang H. Molecular pathways: targeting mechanisms of asbestos and erionite carcinogenesis in mesothelioma. Clin Cancer Res 2012;18:598-604. [PubMed]
- Carbone M, Kratzke RA, Testa JR. The pathogenesis of mesothelioma. Semin Oncol 2002;29:2-17. [PubMed]
- Park EK, Takahashi K, Hoshuyama T, et al. Global magnitude of reported and unreported mesothelioma. Environ Health Perspect 2011;119:514-8. [PubMed]
- Carbone M, Ly BH, Dodson RF, et al. Malignant mesothelioma: facts, myths, and hypotheses. J Cell Physiol 2012;227:44-58. [PubMed]
- Robinson BW, Lake RA. Advances in malignant mesothelioma. N Engl J Med 2005;353:1591-603. [PubMed]
- Jaurand MC. Mechanisms of fiber-induced genotoxicity. Environ Health Perspect 1997;105 Suppl 5:1073-84. [PubMed]
- Mossman B, Light W, Wei E. Asbestos: mechanisms of toxicity and carcinogenicity in the respiratory tract. Annu Rev Pharmacol Toxicol 1983;23:595-615. [PubMed]
- Miserocchi G, Sancini G, Mantegazza F, et al. Translocation pathways for inhaled asbestos fibers. Environ Health 2008;7:4. [PubMed]
- Donaldson K, Murphy FA, Duffin R, et al. Asbestos, carbon nanotubes and the pleural mesothelium: a review of the hypothesis regarding the role of long fibre retention in the parietal pleura, inflammation and mesothelioma. Part Fibre Toxicol 2010;7:5. [PubMed]
- Kamp DW. Asbestos-induced lung diseases: an update. Transl Res 2009;153:143-52. [PubMed]
- Heintz NH, Janssen-Heininger YM, Mossman BT. Asbestos, lung cancers, and mesotheliomas: from molecular approaches to targeting tumor survival pathways. Am J Respir Cell Mol Biol 2010;42:133-9. [PubMed]
- Sekido Y. Genomic abnormalities and signal transduction dysregulation in malignant mesothelioma cells. Cancer Sci 2010;101:1-6. [PubMed]
- Beachy PA, Karhadkar SS, Berman DM. Tissue repair and stem cell renewal in carcinogenesis. Nature 2004;432:324-31. [PubMed]
- Carmona R, Cano E, Grueso E, et al. Peritoneal repairing cells: a type of bone marrow derived progenitor cells involved in mesothelial regeneration. J Cell Mol Med 2011;15:1200-9. [PubMed]
- Lansley SM, Searles RG, Hoi A, et al. Mesothelial cell differention into osteoblast- and adipocyte-like cells. J Cell Mol Med 2011;15:2095-105. [PubMed]
- Glinsky GV, Berezovska O, Glinskii AB. Microarray analysis identifies a death-from-cancer signature predicting therapy failure in patients with multiple types of cancer. J Clin Invest 2005;115:1503-21. [PubMed]
- Cai J, Zhang N, Zheng Y, et al. The Hippo signaling pathway restricts the oncogenic potential of an intestinal regeneration program. Genes Dev 2010;24:2383-8. [PubMed]
- Forbes SA, Bindal N, Bamford S, et al. COSMIC: mining complete cancer genomes in the Catalogue of Somatic Mutations in Cancer. Nucleic Acids Res 2011;39:D945-50. [PubMed]
- Kratzke RA, Otterson GA, Lincoln CE, et al. Immunohistochemical analysis of the p16INK4 cyclin-dependent kinase inhibitor in malignant mesothelioma. J Natl Cancer Inst 1995;87:1870-5. [PubMed]
- Yang CT, You L, Yeh CC, et al. Adenovirus-mediated p14(ARF) gene transfer in human mesothelioma cells. J Natl Cancer Inst 2000;92:636-41. [PubMed]
- Cheng JQ, Jhanwar SC, Klein WM, et al. p16 alterations and deletion mapping of 9p21-p22 in malignant mesothelioma. Cancer Res 1994;54:5547-51. [PubMed]
- Xio S, Li D, Vijg J, et al. Codeletion of p15 and p16 in primary malignant mesothelioma. Oncogene 1995;11:511-5. [PubMed]
- Prins JB, Williamson KA, Kamp MM, et al. The gene for the cyclin-dependent-kinase-4 inhibitor, CDKN2A, is preferentially deleted in malignant mesothelioma. Int J Cancer 1998;75:649-53. [PubMed]
- Toyooka S, Pass HI, Shivapurkar N, et al. Aberrant methylation and simian virus 40 tag sequences in malignant mesothelioma. Cancer Res 2001;61:5727-30. [PubMed]
- Wong L, Zhou J, Anderson D, et al. Inactivation of p16INK4a expression in malignant mesothelioma by methylation. Lung Cancer 2002;38:131-6. [PubMed]
- Destro A, Ceresoli GL, Baryshnikova E, et al. Gene methylation in pleural mesothelioma: Correlations with clinico-pathological features and patient's follow-up. Lung Cancer 2008;59:369-76. [PubMed]
- Bianchi AB, Mitsunaga SI, Cheng JQ, et al. High frequency of inactivating mutations in the neurofibromatosis type 2 gene (NF2) in primary malignant mesotheliomas. Proc Natl Acad Sci U S A 1995;92:10854-8. [PubMed]
- Sekido Y, Pass HI, Bader S, et al. Neurofibromatosis type 2 (NF2) gene is somatically mutated in mesothelioma but not in lung cancer. Cancer Res 1995;55:1227-31. [PubMed]
- Deguen B, Goutebroze L, Giovannini M, et al. Heterogeneity of mesothelioma cell lines as defined by altered genomic structure and expression of the NF2 gene. Int J Cancer 1998;77:554-60. [PubMed]
- Jin H, Sperka T, Herrlich P, et al. Tumorigenic transformation by CPI-17 through inhibition of a merlin phosphatase. Nature 2006;442:576-9. [PubMed]
- Thurneysen C, Opitz I, Kurtz S, et al. Functional inactivation of NF2/merlin in human mesothelioma. Lung Cancer 2009;64:140-7. [PubMed]
- Tang X, Jang SW, Wang X, et al. Akt phosphorylation regulates the tumour-suppressor merlin through ubiquitination and degradation. Nat Cell Biol 2007;9:1199-207. [PubMed]
- Fleury-Feith J, Lecomte C, Renier A, et al. Hemizygosity of Nf2 is associated with increased susceptibility to asbestos-induced peritoneal tumours. Oncogene 2003;22:3799-805. [PubMed]
- Lecomte C, Andujar P, Renier A, et al. Similar tumor suppressor gene alteration profiles in asbestos-induced murine and human mesothelioma. Cell Cycle 2005;4:1862-9. [PubMed]
- Jongsma J, van Montfort E, Vooijs M, et al. A conditional mouse model for malignant mesothelioma. Cancer Cell 2008;13:261-71. [PubMed]
- Zhang N, Bai H, David KK, et al. The Merlin/NF2 tumor suppressor functions through the YAP oncoprotein to regulate tissue homeostasis in mammals. Dev Cell 2010;19:27-38. [PubMed]
- Kinzler KW, Vogelstein B. Cancer-susceptibility genes. Gatekeepers and caretakers. Nature 1997;386:761, 763.
- Curto M, McClatchey AI. Nf2/Merlin: a coordinator of receptor signalling and intercellular contact. Br J Cancer 2008;98:256-62. [PubMed]
- Curto M, Cole BK, Lallemand D, et al. Contact-dependent inhibition of EGFR signaling by Nf2/Merlin. J Cell Biol 2007;177:893-903. [PubMed]
- McLaughlin ME, Kruger GM, Slocum KL, et al. The Nf2 tumor suppressor regulates cell-cell adhesion during tissue fusion. Proc Natl Acad Sci U S A 2007;104:3261-6. [PubMed]
- Li W, You L, Cooper J, et al. Merlin/NF2 suppresses tumorigenesis by inhibiting the E3 ubiquitin ligase CRL4(DCAF1) in the nucleus. Cell 2010;140:477-90. [PubMed]
- Lau YK, Murray LB, Houshmandi SS, et al. Merlin is a potent inhibitor of glioma growth. Cancer Res 2008;68:5733-42. [PubMed]
- Murakami H, Mizuno T, Taniguchi T, et al. LATS2 is a tumor suppressor gene of malignant mesothelioma. Cancer Res 2011;71:873-83. [PubMed]
- Bott M, Brevet M, Taylor BS, et al. The nuclear deubiquitinase BAP1 is commonly inactivated by somatic mutations and 3p21.1 losses in malignant pleural mesothelioma. Nat Genet 2011;43:668-72. [PubMed]
- Ren YR, Patel K, Paun BC, et al. Structural analysis of the cancer-specific promoter in mesothelin and in other genes overexpressed in cancers. J Biol Chem 2011;286:11960-9. [PubMed]
- Chang K, Pastan I, Willingham MC. Isolation and characterization of a monoclonal antibody, K1, reactive with ovarian cancers and normal mesothelium. Int J Cancer 1992;50:373-81. [PubMed]
- Ordóñez NG. Application of immunohistochemistry in the diagnosis of epithelioid mesothelioma: a review and update. Hum Pathol 2013;44:1-19. [PubMed]
- Mizuno T, Murakami H, Fujii M, et al. YAP induces malignant mesothelioma cell proliferation by upregulating transcription of cell cycle-promoting genes. Oncogene 2012;31:5117-22. [PubMed]
- Dixit R, Ai X, Fine A. Derivation of lung mesenchymal lineages from the fetal mesothelium requires hedgehog signaling for mesothelial cell entry. Development 2013;140:4398-406. [PubMed]
- Shi Y, Moura U, Opitz I, et al. Role of hedgehog signaling in malignant pleural mesothelioma. Clin Cancer Res 2012;18:4646-56. [PubMed]
- Hao Y, Chun A, Cheung K, et al. Tumor suppressor LATS1 is a negative regulator of oncogene YAP. J Biol Chem 2008;283:5496-509. [PubMed]
- Romer JT, Kimura H, Magdaleno S, et al. Suppression of the Shh pathway using a small molecule inhibitor eliminates medulloblastoma in Ptc1(+/-)p53(-/-) mice. Cancer Cell 2004;6:229-40. [PubMed]
- Tanaka I, Osada H, Fujii M, et al. LIM-domain protein AJUBA suppresses malignant mesothelioma cell proliferation via Hippo signaling cascade. Oncogene 2013. [Epub ahead of print]. [PubMed]
- Goyal RK, Lin P, Kanungo J, et al. Ajuba, a novel LIM protein, interacts with Grb2, augments mitogen-activated protein kinase activity in fibroblasts, and promotes meiotic maturation of Xenopus oocytes in a Grb2- and Ras-dependent manner. Mol Cell Biol 1999;19:4379-89. [PubMed]
- Das Thakur M, Feng Y, Jagannathan R, et al. Ajuba LIM proteins are negative regulators of the Hippo signaling pathway. Curr Biol 2010;20:657-62. [PubMed]
- Liu-Chittenden Y, Huang B, Shim JS, et al. Genetic and pharmacological disruption of the TEAD-YAP complex suppresses the oncogenic activity of YAP. Genes Dev 2012;26:1300-5. [PubMed]
- Keshava S, Sahoo S, Tucker TA, et al. Endothelial cell protein C receptor opposes mesothelioma growth driven by tissue factor. Cancer Res 2013;73:3963-73. [PubMed]
- O’Callaghan K, Kuliopulos A, Covic L. Turning receptors on and off with intracellular pepducins: new insights into G-protein-coupled receptor drug development. J Biol Chem 2012;287:12787-96. [PubMed]
- Yamada T, Yano S, Ogino H, et al. Lysophosphatidic acid stimulates the proliferation and motility of malignant pleural mesothelioma cells through lysophosphatidic acid receptors, LPA1 and LPA2. Cancer Sci 2008;99:1603-10. [PubMed]
- Shi Y, Felley-Bosco E, Marti TM, et al. Differential effects of lovastatin on cisplatin responses in normal human mesothelial cells versus cancer cells: implication for therapy. PLoS One 2012;7:e45354. [PubMed]
- Johnson R, Halder G. The two faces of Hippo: targeting the Hippo pathway for regenerative medicine and cancer treatment. Nat Rev Drug Discov 2014;13:63-79. [PubMed]
- Park HW, Guan KL. Regulation of the Hippo pathway and implications for anticancer drug development. Trends Pharmacol Sci 2013;34:581-9. [PubMed]