Combining stereotactic body radiation therapy with immunotherapy: current data and future directions
Introduction
Stereotactic body radiation therapy (SBRT) combines conformal radiation dose-shaping, tumor motion management, and on-board daily imaging to deliver high doses of radiation in five or fewer treatments (1,2). These technological advances in radiation delivery over the last decade have allowed safer and more effective dose escalation to patients with non-small cell lung cancer (NSCLC). For early-stage, medically non-operable NSCLC, SBRT has become the standard of care (3). RTOG 0236 established SBRT could achieve an impressive 5-year tumor control rate of 93% (4,5), with minimal pulmonary toxicity (6).
However, systemic progression remains problematic. Regional and distant failure rates occur in at least 30% of patients, with even higher rates with increasing tumor size (5,7-11). Medically inoperable patients often cannot safely receive adjuvant chemotherapy to help with distant control. Immunotherapy, which is better tolerated than chemotherapy (12), has recently been heralded as the “fourth pillar” of oncologic treatment (13). Food and Drug Administration (FDA)-approved antibody drugs currently target cytotoxic T-lymphocyte protein 4 (CTLA-4), programmed cell-death protein 1 (PD-1), and programmed death-ligand 1 (PD-L1). Activation of CTLA-4 and PD-1 receptors on T cells downregulates the adaptive immune response. This prevents auto-immunity, but checkpoint inhibition can also be hijacked by tumors seeking to avoid immune surveillance (14). By targeting these checkpoint inhibitors, immunotherapy has the potential to take the brakes off one’s own immune system to seek out and destroy cancer cells.
Immunotherapy has had mixed success in the locally advanced and metastatic NSCLC setting. Nivolumab, a PD-1 inhibitor, was compared in a phase 3 trial to platinum-based chemotherapy for metastatic or recurrent NSCLC with PD-L1 expression ≥5% (15). There was no difference in progression-free survival (PFS) or overall survival (OS), but patients tolerated nivolumab better (grades 3–4 adverse event 18% vs. 51%). Similarly, ipilimumab, a CTLA-4 inhibitor, did not improve PFS or OS when added to carboplatin/paclitaxel in metastatic NSCLC (16). In contrast, KEYNOTE 24 tested pembrolizumab, a PD-1 inhibitor, compared to platinum-based chemotherapy in metastatic NSCLC patients with tumor PD-L1 expression ≥50%, and both PFS and OS were significantly improved with a 45% response rate (17). The PACIFIC trial tested adjuvant durvalumab, a PD-L1 inhibitor, against placebo after definitive chemoradiation for stage III NSCLC (18). Durvalumab significantly improved median PFS from 5.6 to 16.8 months. The PFS benefit was seen even when the tumor had PD-L1 expression <25%. Atezolizumab, also a PD-L1 inhibitor, improved OS compared to docetaxel in metastatic NSCLC regardless of PD-L1 expression (19). The overall success of checkpoint inhibitors is tempered by the variable response rate, which may be improved upon when combined with radiation therapy. Several excellent reviews on this subject have been recently published and we refer you to them for additional references (20-24). In this fast-changing field of immuno-radiation therapy, we will highlight updates from ongoing clinical trials and offer our perspective for future trials.
Rationale for combining SBRT with immunotherapy
SBRT tumor debulking may improve immunotherapy response. A recent publication in 29 patients with stage IV melanoma treated with pembrolizumab found 74% of patients had an immunologic response seen in peripheral blood draws, but only 38% achieved a radiographic clinical response (25). Using Ki-67 as a marker of proliferation of PD-1+ T cells, the authors measured the Ki-67 percentage cell staining to tumor burden (sum of the long-axis of all measurable lesions) ratio after patients received pembrolizumab. A ratio >1.9 was associated with improved response and OS. One rationale for tumor debulking lies in T cell exhaustion, a phenomenon whereby inhibitory signals from the tumor overwhelm T cell activation (26). In patients with limited or oligometastatic disease, SBRT could reduce the tumor burden and allow re-invigorated T cells to find and destroy micrometastatic disease.
Radiation has also been shown to upregulate immunogenic cell surface markers. MHC class I is a molecule that presents intracellular antigens to the cell surface for T cells to recognize foreign peptides. Their expression is down-regulated in tumors to evade immune recognition (20). Reits et al. were able to show that radiation increases MHC class I expression in a dose dependent manner, and mice exposed to both radiation and immunotherapy had a prolonged tumor response compared to mice receiving either therapy alone (27). Calreticulin and HMGB1 are other antigen-presenting proteins that have been found to be upregulated by radiation (28). Thus, radiation may synergize with immunotherapy by helping unmask tumor antigens.
Radiation can also engage the innate immune system. FAS is a death receptor that catalyzes the apoptotic cascade when it encounters FAS ligand, found on activated T cells. Chakraborty et al. found that one 8 Gy dose of radiation upregulated FAS on tumor cells in situ for up to 11 days and increased T cell infiltration and killing (29). Natural killer cells can also be alerted to kill tumor cells by radiation-induced NKG2D expression (30). There is thus a halo effect, where tumor cells primed to be recognized by undergoing apoptosis after radiation are engulfed in an overwhelming immune response from neighboring activated immune cells.
Radiation, unfortunately, is a double-edged sword. Prolonged fractionated radiation courses to large vascular volumes have been shown to deplete circulating lymphocytes in all body sites, sometimes up to a year after radiation (31-34). Lymphocytes are among the most radiosensitive cells in the body, with in vitro data showing 50% cell killing after 2 Gy and 10% cell killing after 0.5 Gy (35). In locally advanced lung cancer, both cumulative lung and heart dose were associated with worsening lymphopenia and poor survival (34,36). Hypofractionation or SBRT could potentially reduce this iatrogenic immunosuppression by limiting the blood pool volume exposed to daily low-intermediate dose radiation (37,38). Furthermore, radiation up-regulates cell surface PD-L1 expression (39), which by itself can limit the immunogenic cell death desired for optimal local control. However, Deng et al. has shown blockade of PD-L1 after irradiation diminishes the infiltration of tumor suppressor cells (39), further rationalizing the combination of hypofractionated radiation with checkpoint inhibitors.
The abscopal effect is a much-discussed hope of many radiation oncologists. Simply put, can we radiate a tumor and create anti-tumor effects outside of the irradiated field? The mechanism of the abscopal effect is hypothesized to be immune-mediated. Tumor-specific antigens revealed by irradiation need to be recognized and picked up by dendritic cells, which then activate T cells at neighboring lymph nodes (40). Efforts to understand and exploit the abscopal effect have ramped up since checkpoint inhibitors were found to have increased immunomodulatory activity compared to activating cytokines (e.g., IL-2) and infusions of activated immune cells. However, it is still unclear what the optimal radiation dose, fractionation schedule, and timing with immunotherapy is to induce an abscopal effect. Most pre-clinical studies have used single dose or hypofractionated courses with large (≥6 Gy) doses per fraction (40). In order to better study this, clinically relevant models of cancer development beyond orthotopic tumor models need to be developed.
Clinical data combining radiation with immunotherapy
The introduction of immunotherapy for metastatic cancer treatment has corresponded with increasing clinical case reports of the abscopal effect. Reynders et al. compiled data from 23 case reports, in an effort to generate momentum in studying this phenomenon (41). Radiation dose and fractionation varied, ranging from 14.4 Gy in 12 fractions to 18–26 Gy in a single fraction. Time to documented abscopal response ranged between less than 1 and 24 months, with a median reported time of 5 months. Once an abscopal response was achieved, a median time of 13 months went by before disease progression occurred or the reported follow-up ended (range, 3–39 months). Of four reported cases of abscopal effect in a patient with a primary lung tumor, two were in combination with immunotherapy.
The combination of immunotherapy and radiation therapy has been studied more systematically in the metastatic setting as well. A secondary analysis of 98 metastatic NSCLC patients treated on the phase 1 KEYNOTE-001 pembrolizumab trial from the University of California, Los Angeles found that PFS [hazard ratio (HR) =0.56, 95% confidence interval (CI), 0.34–0.91] and OS (HR =0.58, 95% CI, 0.36–0.94) were improved in patients that had received any prior radiation (43%) (42). There was no difference in positive PD-L1 status in patients receiving prior radiation vs. not (71% vs. 80%, respectively, P=0.75). Of the patients receiving prior radiation, 91% was to extracranial sites; 74% was delivered with palliative intent; SBRT was used in 29% of cases. However, the group with prior radiation had been exposed to more lines of systemic therapy (P=0.02) and time from diagnosis to starting pembrolizumab was longer (26 vs. 17 months, P=0.04).
Similarly, a retrospective analysis of 164 metastatic NSCLC patients treated with PD-1/PD-L1 inhibitors from Massachusetts General Hospital compared outcomes in patients receiving thoracic radiation (n=73) or not (n=91) (43). Most patients were treated with a definitive radiation dose (median 60 Gy, range, 44–79.1 Gy), and the median time to checkpoint inhibitor initiation after radiation was 8.6 months. In multivariable analysis, the thoracic radiation group trended to improved OS (HR =0.66, 95% CI, 0.42–1.01) despite including fewer favorable adenocarcinoma patients (49% vs. 75%, P=0.001) with targetable mutation (4% vs. 16%, P=0.01). Thus, the two prior studies suggest there may be clinical synergy between checkpoint inhibitors and radiation therapy.
In an interesting retrospective study from China, outcomes of patients with a variety of advanced malignancies receiving SBRT combined with adoptive immunotherapy were better than outcomes of patients receiving SBRT alone (44). In the study, re-injection of the patient’s own interferon-activated monocytes combined with SBRT improved the total response rate (RECIST partial or complete response) compared to SBRT alone (66.8% vs. 60.2%, P<0.05). A median total dose of 43 Gy (range, 18–65 Gy) given in 4–18 Gy per fraction was prescribed. However, this was a heterogeneous group of patients and tumor response outside of the irradiated field was not specified, which limits interpretation and generalization for this review.
The first phase I–II prospective trial combining SBRT with immunotherapy was updated last year. Tang et al. from MD Anderson Cancer Center designed a trial testing concurrent vs. sequential ipilimumab (4 cycles every 3 weeks, 3 mg/kg) and SBRT (50 Gy in 4 fractions or 60 Gy in 10 fractions) targeted to liver or lung metastases. In the phase I portion, 7/31 (23%) had clinical benefit, either a partial response (3 patients) or stable disease lasting more than 6 months (4 patients) outside the irradiated field, but 34% of patients experienced grade 3 toxicity (most commonly colitis, liver toxicity, and a maculopapular rash). Liver SBRT produced greater T cell activation, which was associated with clinical benefit (45). In the phase 2 update at the 2017 American Society for Radiation Oncology (ASTRO) annual conference, 100 patients had been enrolled. Clinical benefit, defined as stable disease or response, was seen in 67% of patients with NSCLC. Stable disease was seen in 60% of the sequential 60 Gy group, 50% of the sequential 50 Gy lung group, 45% of the concurrent 50 Gy lung group, 35% of the concurrent 50 Gy liver group, and 30% of the sequential 50 Gy liver group. In contrast to the phase I results, patients who received sequential radiation to lung metastases rather than to liver metastases had better PFS (P=0.055, 95% CI, 3.7–6.4) and OS (P=0.059, CI, 7.9–20.0). No differences were found between the concurrent lung or liver groups for PFS (P=0.2) or OS (P=0.3) (46).
Most recently, the University of Chicago published a phase I study of multisite SBRT followed by pembrolizumab in metastatic solid tumor patients (47). Two to four metastases (94.5% were two metastases) were targeted with SBRT to 30–50 Gy in 3–5 fractions, and pembrolizumab 200 mg every 3 weeks was initiated 7 days after completion of SBRT. The cohort of 73 patients was heavily pre-treated, with a median of five prior therapies. Out of 151 metastases irradiated, 68 were in the lung, 24 in the liver, 28 in other abdomen/pelvis sites, 16 in the bone, and 15 near the spine. The abscopal response rate (RECIST, 30% reduction) using the aggregate diameter of all nonirradiated target metastases was 13.5%; however, the response rate in any single nonirradiated target metastasis was an impressive 26.9%. Median PFS was 3.1 months, but it was not reported if patients with an initial response had a sustained response. Grade 3 toxicity was seen in 6 patients (pneumonitis n=3; colitis n=2; hepatic toxicity n=1). These promising results in a heterogenous group of tumors with agnostic PD-L1 status support further studies combining checkpoint inhibitors with SBRT.
Finally, short-term safety of combining thoracic SBRT and immunotherapy was recently explored in a combined analysis of two prospective trials ongoing at MD Anderson (48). The first was the phase 1–2 trial testing SBRT with ipilimumab detailed above. The second was another ongoing phase 1–2 trial testing SBRT with concurrent pembrolizumab in metastatic NSCLC. SBRT dose was 50 Gy in 4 fractions or 60 Gy in 10 fractions. Out of 60 patients with a median follow up of 6.9 months (range, 0.5–30.9 months), there were no grade ≥4 toxicities. There were 34 grade 3 toxicities experienced by 15 patients (9 grade 3 pulmonary toxicities experienced by 4 patients) with no difference between the ipilimumab and pembrolizumab groups. These short-term pulmonary toxicity rates were on par with RTOG 0236, in which 8 of 55 patients developed grade 3 respiratory events (4). Notably, no iatrogenic leukopenia was seen with SBRT, suggesting SBRT and immunotherapy have non-intersecting and complementary toxicity profiles.
Future directions
As more interest is generated in combining radiation with immunotherapy, it is important to recognize that tumors with a higher mutational burden like NSCLC and melanoma appear to be more likely to respond to protocols involving immunotherapy (49). Prior trials combining ipilimumab with a palliative 8 Gy single dose of radiation in metastatic prostate cancer (50,51) were possibly doomed by choosing a non-ablative dose in a mutationally quiet cancer (52). The optimal dose and fractionation may also differ for each cancer type. A recent case series of 47 metastatic melanoma patients found an abscopal effect was most likely to occur when fraction sizes ≤3 Gy were utilized (53). Preliminary evidence from the MD Anderson phase II trial indicate that treating the largest lesion in metastatic NSCLC with 60 Gy in 10 fractions may have the most activity when combined with ipilimumab (46). Pre-clinical data supports using a hypofractionated regimen of 3–5 fractions with dose per fraction <10–12 Gy, but dose and fractionation will need to be further evaluated in clinical trials (54). Response rates and clinical benefit of other immune checkpoint inhibitors are also actively being investigated (Table 1), and the optimal combination of therapy and sequencing with SBRT is still to be determined.
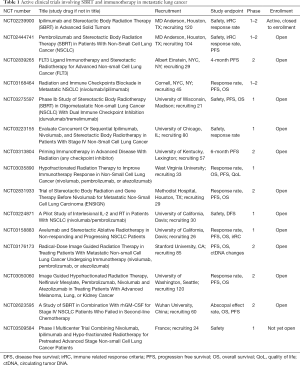
Full table
SBRT and immunotherapy protocols are now in development for non-metastatic NSCLC patients (Table 2). Since decreased tumor burden has been associated with increased response to immunotherapy (25), this strategy is promising. Results from the PACIFIC trial already showed adjuvant immunotherapy after definitive chemoradiation in stage III NSCLC improved PFS (18), regardless of tumor PD-L1 expression. This suggests that radiation may work synergistically with immunotherapy, as prior trials in the metastatic setting using immunotherapy alone were mostly only positive in tumors with positive PD-L1 expression. However, the local failure rate of conventionally fractionated radiation with concurrent chemotherapy approaches 40%, and efforts to dose-escalate conventional radiation have been limited by cardiac and pulmonary toxicity (55-58). The future may combine the conformal ablative power of an SBRT boost to residual PET-avid disease (59) to further maximize the efficacy of adjuvant immunotherapy.
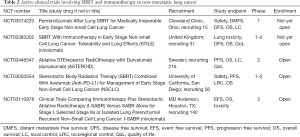
Full table
In early stage NSCLC, the rationale for adding adjuvant immunotherapy to SBRT is even greater. Regional and distant failure rates of at least 30% (11) demand a tolerable systemic solution in this medically frail population. We hypothesize that use of adjuvant immunotherapy will reduce locoregional and distant recurrence with a resultant improvement in OS following SBRT for early stage NSCLC. Compared with cytotoxic chemotherapy, we expect that this will be readily tolerable in this patient population, and lead to minimal impact in quality of life. NRG Oncology is developing a phase 3 trial of adjuvant durvalumab after SBRT in early stage NSCLC, which will test this hypothesis. In the marriage of SBRT with immunotherapy, we are still in the honeymoon period. Time will tell if these early promises will last.
Acknowledgements
None.
Footnote
Conflicts of Interest: The authors have no conflicts of interest to declare.
References
- Caillet V, Booth JT, Keall P. IGRT and motion management during lung SBRT delivery. Phys Med 2017;44:113-22. [Crossref] [PubMed]
- Chua KLM, Sin I, Fong KW, et al. Stereotactic body radiotherapy for early stage lung cancer—historical developments and future strategies. Chin Clin Oncol 2017;6:S20. [Crossref] [PubMed]
- Ettinger DS, Wood DE, Aisner DL, et al. Non-Small Cell Lung Cancer, Version 5.2017, NCCN Clinical Practice Guidelines in Oncology. J Natl Compr Canc Netw 2017;15:504-35. [Crossref] [PubMed]
- Timmerman R, Paulus R, Galvin J, et al. Stereotactic body radiation therapy for inoperable early stage lung cancer. JAMA 2010;303:1070-6. [Crossref] [PubMed]
- Timmerman RD, Hu C, Michalski J, et al. Long-term Results of RTOG 0236: A Phase II Trial of Stereotactic Body Radiation Therapy (SBRT) in the Treatment of Patients with Medically Inoperable Stage I Non-Small Cell Lung Cancer. Int J Radiat Oncol 2014;90:S30. [Crossref]
- Stanic S, Paulus R, Timmerman RD, et al. No clinically significant changes in pulmonary function following stereotactic body radiation therapy for early- stage peripheral non-small cell lung cancer: An analysis of RTOG 0236. Int J Radiat Oncol Biol Phys 2014;88:1092-9. [Crossref] [PubMed]
- Timmerman RD, Paulus R, Pass HI, et al. RTOG 0618: Stereotactic body radiation therapy (SBRT) to treat operable early-stage lung cancer patients. J Clin Oncol 2013;31:7523.
- Bezjak A, Paulus R, Gaspar LE, et al. Primary Study Endpoint Analysis for NRG Oncology/RTOG 0813 Trial of Stereotactic Body Radiation Therapy (SBRT) for Centrally Located Non-Small Cell Lung Cancer (NSCLC). Int J Radiat Oncol 2016;94:5-6. [Crossref]
- Videtic GMM, Hu C, Singh AK, et al. A randomized phase 2 study comparing 2 stereotactic body radiation therapy schedules for medically inoperable patients with stage i peripheral non-small cell lung cancer: NRG Oncology RTOG 0915 (NCCTG N0927). Int J Radiat Oncol Biol Phys 2015;93:757-64. [Crossref] [PubMed]
- Robinson CG, Dewees TA, El Naqa IM, et al. Patterns of failure after stereotactic body radiation therapy or lobar resection for clinical stage i non-small-cell lung cancer. J Thorac Oncol 2013;8:192-201. [Crossref] [PubMed]
- Chi A, Liao Z, Nguyen NP, et al. Systemic review of the patterns of failure following stereotactic body radiation therapy in early-stage non-small-cell lung cancer: Clinical implications. Radiother Oncol 2010;94:1-11. [Crossref] [PubMed]
- Brahmer JR, Rodríguez-Abreu D, Robinson AG, et al. Health-related quality-of-life results for pembrolizumab versus chemotherapy in advanced, PD-L1-positive NSCLC (KEYNOTE-024): a multicentre, international, randomised, open-label phase 3 trial. Lancet Oncol 2017;18:1600-9. [Crossref] [PubMed]
- Smyth MJ. Multiple approaches to immunotherapy - The new pillar of cancer treatment. Immunol Cell Biol 2017;95:323-4. [Crossref] [PubMed]
- Sharpe AH. Introduction to checkpoint inhibitors and cancer immunotherapy. Immunol Rev 2017;276:5-8. [Crossref] [PubMed]
- Carbone DP, Reck M, Paz-Ares L, et al. First-Line Nivolumab in Stage IV or Recurrent Non-Small-Cell Lung Cancer. N Engl J Med 2017;376:2415-26. [Crossref] [PubMed]
- Govindan R, Szczesna A, Ahn MJ, et al. Phase III Trial of Ipilimumab Combined With Paclitaxel and Carboplatin in Advanced Squamous Non-Small-Cell Lung Cancer. J Clin Oncol 2017;35:3449-57. [Crossref] [PubMed]
- Reck M, Rodríguez-Abreu D, Robinson AG, et al. Pembrolizumab versus Chemotherapy for PD-L1-Positive Non-Small-Cell Lung Cancer. N Engl J Med 2016;375:1823-33. [Crossref] [PubMed]
- Antonia SJ, Villegas A, Daniel D, et al. Durvalumab after Chemoradiotherapy in Stage III Non-Small-Cell Lung Cancer. N Engl J Med 2017;377:1919-29. [Crossref] [PubMed]
- Rittmeyer A, Barlesi F, Waterkamp D, et al. Atezolizumab versus docetaxel in patients with previously treated non-small-cell lung cancer (OAK): a phase 3, open-label, multicentre randomised controlled trial. Lancet 2017;389:255-65. [Crossref] [PubMed]
- Sharabi AB, Lim M, DeWeese TL, et al. Radiation and checkpoint blockade immunotherapy: Radiosensitisation and potential mechanisms of synergy. Lancet Oncol 2015;16:e498-509. [Crossref] [PubMed]
- Daly ME, Monjazeb AM, Kelly K. Clinical Trials Integrating Immunotherapy and Radiation for Non-Small-Cell Lung Cancer. J Thorac Oncol 2015;10:1685-93. [Crossref] [PubMed]
- Popp I, Grosu AL, Niedermann G, et al. Immune modulation by hypofractionated stereotactic radiation therapy: Therapeutic implications. Radiother Oncol 2016;120:185-94. [Crossref] [PubMed]
- Taunk NK, Rimner A, Culligan M, et al. Immunotherapy and radiation therapy for operable early stage and locally advanced non-small cell lung cancer. Transl Lung Cancer Res 2017;6:178-85. [Crossref] [PubMed]
- Kumar SS, Higgins KA, McGarry RC. Emerging Therapies for Stage III Non-Small Cell Lung Cancer: Stereotactic Body Radiation Therapy and Immunotherapy. Front Oncol 2017;7:197. [Crossref] [PubMed]
- Huang AC, Postow MA, Orlowski RJ, et al. T-cell invigoration to tumour burden ratio associated with anti-PD-1 response. Nature 2017;545:60-5. [Crossref] [PubMed]
- Wherry EJ. T cell exhaustion. Nat Immunol 2011;12:492-9. [Crossref] [PubMed]
- Reits EA, Hodge JW, Herberts CA, et al. Radiation modulates the peptide repertoire, enhances MHC class I expression, and induces successful antitumor immunotherapy. J Exp Med 2006;203:1259-71. [Crossref] [PubMed]
- Gameiro SR, Jammeh ML, Wattenberg MM, et al. Radiation-induced immunogenic modulation of tumor enhances antigen processing and calreticulin exposure, resulting in enhanced T-cell killing. Oncotarget 2014;5:403-16. [Crossref] [PubMed]
- Chakraborty M, Abrams SI, Coleman CN, et al. External beam radiation of tumors alters phenotype of tumor cells to render them susceptible to vaccine-mediated T-cell killing. Cancer Res 2004;64:4328-37. [Crossref] [PubMed]
- Kim JY, Son YO, Park SW, et al. Increase of NKG2D ligands and sensitivity to NK cell-mediated cytotoxicity of tumor cells by heat shock and ionizing radiation. Exp Mol Med 2006;38:474-84. [Crossref] [PubMed]
- Campian JL, Ye X, Brock M, et al. Treatment-related lymphopenia in patients with stage III non-small-cell lung cancer. Cancer Invest 2013;31:183-8. [Crossref] [PubMed]
- Campian JL, Sarai G, Ye X, et al. The association between severe treatment-related lymphopenia and progression free survival in patients with newly diagnosed squamous cell head and neck cancer. Head Neck 2014;36:1747-53. [Crossref] [PubMed]
- Grossman S, Ellsworth S, Campian J, et al. Survival in Patients With Severe Lymphopenia Following Treatment With Radiation and Chemotherapy for Newly Diagnosed Solid Tumors. J Natl Compr Canc Netw 2015;13:1225-31. [Crossref] [PubMed]
- Tang C, Liao Z, Gomez D, et al. Lymphopenia Association With Gross Tumor Volume and Lung V5 and Its Effects on Non-Small Cell Lung Cancer Patient Outcomes. Int J Radiat Oncol Biol Phys 2014;89:1084-91. [Crossref] [PubMed]
- Nakamura N, Kusunoki Y, Akiyama M. Radiosensitivity of CD4 or CD8 positive human T-lymphocytes by an in vitro colony formation assay. Radiat Res 1990;123:224-7. [Crossref] [PubMed]
- Contreras JA, Lin AJ, Weiner A, et al. Cardiac dose is associated with immunosuppression and poor survival in locally advanced non-small cell lung cancer. Radiother Oncol 2018. [Epub ahead of print]. [Crossref] [PubMed]
- Yovino S, Kleinberg L, Grossman SA, et al. The etiology of treatment-related lymphopenia in patients with malignant gliomas: modeling radiation dose to circulating lymphocytes explains clinical observations and suggests methods of modifying the impact of radiation on immune cells. Cancer Invest 2013;31:140-4. [Crossref] [PubMed]
- Crocenzi T, Cottam B, Newell P, et al. A hypofractionated radiation regimen avoids the lymphopenia associated with neoadjuvant chemoradiation therapy of borderline resectable and locally advanced pancreatic adenocarcinoma. J Immunother Cancer 2016;4:45. [Crossref] [PubMed]
- Deng L, Liang H, Burnette B, et al. Irradiation and anti - PD-L1 treatment synergistically promote antitumor immunity in mice. J Clin Invest 2014;124:687-95. [Crossref] [PubMed]
- Brix N, Tiefenthaller A, Anders H, et al. Abscopal, immunological effects of radiotherapy: Narrowing the gap between clinical and preclinical experiences. Immunol Rev 2017;280:249-79. [Crossref] [PubMed]
- Reynders K, Illidge T, Siva S, et al. The abscopal effect of local radiotherapy: Using immunotherapy to make a rare event clinically relevant. Cancer Treat Rev 2015;41:503-10. [Crossref] [PubMed]
- Shaverdian N, Lisberg AE, Bornazyan K, et al. Previous radiotherapy and the clinical activity and toxicity of pembrolizumab in the treatment of non-small-cell lung cancer: a secondary analysis of the KEYNOTE-001 phase 1 trial. Lancet Oncol 2017;18:895-903. [Crossref] [PubMed]
- Hwang WL, Niemierko A, Hwang KL, et al. Clinical Outcomes in Patients With Metastatic Lung Cancer Treated With PD-1/PD-L1 Inhibitors and Thoracic Radiotherapy. JAMA Oncol 2018;4:253-5. [Crossref] [PubMed]
- Wang YS, Yang G, Wang YY, et al. Early efficacy of stereotactic body radiation therapy combined with adoptive immunotherapy for advanced malignancies. Mol Clin Oncol 2013;1:925-9. [Crossref] [PubMed]
- Tang C, Welsh JW, De Groot P, et al. Ipilimumab with stereotactic ablative radiation therapy: Phase i results and immunologic correlates from peripheral T cells. Clin Cancer Res 2017;23:1388-96. [Crossref] [PubMed]
- Welsh JW, Tang C, de Groot P, et al. Phase 2 5-Arm Trial of Ipilimumab Plus Lung or Liver Stereotactic Radiation for Patients with Advanced Malignancies. Int J Radiat Oncol 2017;99:1315. [Crossref]
- Luke JJ, Lemons JM, Karrison TG, et al. Safety and Clinical Activity of Pembrolizumab and Multisite Stereotactic Body Radiotherapy in Patients With Advanced Solid Tumors. J Clin Oncol 2018;36:1611-8. [Crossref] [PubMed]
- Verma V, Cushman TR, Selek U, et al. Safety of Combined Immunotherapy and Thoracic Radiation Therapy: Analysis of 3 Single-Institutional Phase I/II Trials. Int J Radiat Oncol Biol Phys 2018;101:1141-8. [Crossref] [PubMed]
- Goodman AM, Kato S, Bazhenova L, et al. Tumor Mutational Burden as an Independent Predictor of Response to Immunotherapy in Diverse Cancers. Mol Cancer Ther 2017;16:2598-608. [Crossref] [PubMed]
- Slovin SF, Higano CS, Hamid O, et al. Ipilimumab alone or in combination with radiotherapy in metastatic castration-resistant prostate cancer: Results from an open-label, multicenter phase i/ii study. Ann Oncol 2013;24:1813-21. [Crossref] [PubMed]
- Kwon ED, Drake CG, Scher HI, et al. Ipilimumab versus placebo after radiotherapy in patients with metastatic castration-resistant prostate cancer that had progressed after docetaxel chemotherapy (CA184-043): A multicentre, randomised, double-blind, phase 3 trial. Lancet Oncol 2014;15:700-12. [Crossref] [PubMed]
- Cancer Genome Atlas Research Network. The molecular taxonomy of primary prostate cancer. Cell 2015;163:1011-25. [Crossref] [PubMed]
- Chandra RA, Wilhite TJ, Balboni TA, et al. A systematic evaluation of abscopal responses following radiotherapy in patients with metastatic melanoma treated with ipilimumab. Oncoimmunology 2015;4:e1046028. [Crossref] [PubMed]
- Formenti SC. Optimizing Dose Per Fraction: A New Chapter in the Story of the Abscopal Effect? Int J Radiat Oncol Biol Phys 2017;99:677-9. [Crossref] [PubMed]
- Bradley JD, Paulus R, Komaki R, et al. Standard-dose versus high-dose conformal radiotherapy with concurrent and consolidation carboplatin plus paclitaxel with or without cetuximab for patients with stage IIIA or IIIB non-small-cell lung cancer (RTOG 0617): A randomised, two-by-two factorial p. Lancet Oncol 2015;16:187-99. [Crossref] [PubMed]
- Bradley JD, Hu C, Komaki RU, et al. Long-Term Results of RTOG 0617: A Randomized Phase 3 Comparison of Standard Dose Versus High Dose Conformal Chemoradiation Therapy +/- Cetuximab for Stage III NSCLC. Int J Radiat Oncol 2017;99:S105. [Crossref]
- Speirs CK, DeWees TA, Rehman S, et al. Heart Dose Is an Independent Dosimetric Predictor of Overall Survival in Locally Advanced Non-Small Cell Lung Cancer. J Thorac Oncol 2017;12:293-301. [Crossref] [PubMed]
- Chun SG, Hu C, Choy H, et al. Impact of Intensity-Modulated Radiation Therapy Technique for Locally Advanced Non-Small-Cell Lung Cancer: A Secondary Analysis of the NRG Oncology RTOG 0617 Randomized Clinical Trial. J Clin Oncol 2017;35:56-62. [Crossref] [PubMed]
- Kumar S, Feddock J, Li X, et al. Update of a Prospective Study of Stereotactic Body Radiation Therapy for Post-Chemoradiation Residual Disease in Stage II/III Non-Small Cell Lung Cancer. Int J Radiat Oncol Biol Phys 2017;99:652-9. [Crossref] [PubMed]