Targeted therapy for non-small cell lung cancer: current standards and the promise of the future
Introduction
Lung cancer remains by far the single most common cause of cancer-related mortality with nearly 1.6 million deaths worldwide in 2012 or nearly 20% of cancer mortality as a whole (1). Over the last decade, molecular translational research advances have heralded major breakthroughs in the understanding, diagnosis and management of lung cancer, particularly for the more common (~80%) non-small cell lung cancer (NSCLC). Conversely, treatment for small cell lung cancer remains chemotherapy-based and whilst there are promising results with novel cytotoxics, its platinum-etoposide backbone holds strong (2).
The term ‘Theranostics’ whereby therapeutics and diagnostics have been meaningfully combined to achieve personalised pharmacotherapy has now become commonplace in oncology. Sequencing of the human genome has permitted more efficient identification of epigenetic mutations, tumour-suppressor-gene inactivation as well as oncogene driver mutations that are potential targets for therapy (3-8). Such examples include trastuzumab for HER-2 over-expressing breast cancer and vemurafenib for BRAF-mutant melanoma (9,10).
It is now accepted that NSCLC is not a singular entity but is in fact multiple pathologies with unique molecular signatures that we are only beginning to unravel and understand (11-13). Broadly speaking, the main subtypes are pulmonary adenocarcinoma, squamous cell carcinoma (SCC) and large cell carcinoma. This distinction alone allows for a more tailored selection of cytotoxic chemotherapy in advanced NSCLC without a driver mutation, as seen with enhanced efficacy with pemetrexed in adenocarcinoma (14,15) or the toxicity concerns of bevacizumab in patients with squamous histology (16).
Optimal management of NSCLC now requires that tumours be screened for a range of predictive and prognostic biomarkers that help to predict sensitivity to targeted therapy and estimate prognosis respectively (17). For NSCLC, much of the work in the last decade has been focussed on mutations of the epidermal growth factor receptor (EGFR) and on the abnormal fusion of the anaplastic lymphoma kinase (ALK) being inhibited successfully with EGFR tyrosine kinase inhibitors (TKI) and crizotinib respectively. Targeted agents are now being rationally designed to inhibit particular mutations leading to a more streamlined clinical trial process. In this review, we will examine the major subtypes of driver mutations that have been identified in NSCLC and relevant targeted therapies available both now, and in the foreseeable future.
Signalling pathway targets in NSCLC
The traditional and now over-simplified histological distinctions within NSCLC include adenocarcinoma, SCC and large cell carcinoma (Figure 1). Up to 60% of lung adenocarcinoma and up to 50-80% of SCC have a known oncogenic driver mutation (Figure 1) (18,19). These mutations in receptors or protein kinases can stimulate a complex cascade of cross signalling pathways such as the RAS-RAF-MEK-ERK or MAPK, PI3K-AKT-mTOR or JAK-STAT pathways (Figure 2) (3,4,7,18,20). Ultimately these lead to uncontrolled growth, proliferation and survival. Successful targeted therapy involves the identification and inhibition of these up-regulated pathways by either small molecule inhibitors or receptor monoclonal antibodies (mAb). The best studied in NSCLC is the interaction between EGFR and its downstream pathways.

Epidermal growth factor receptor (EGFR)
The epidermal growth factor receptor (EGFR or ErbB1 or HER1) belongs to a family of receptor tyrosine kinases that can trigger a vast array of signalling pathways leading to cell growth, proliferation and survival (20,21). Such flow-on pathways include the RAS-RAF-MEK-ERK or MAPK pathway and the PI3K-AKT-mTOR pathways.
There are three main mechanisms leading to EGFR activation: increased expression of EGFR on malignant cells; enhanced ligand production by malignant cells; and activating mutations of EGFR within malignant cells. EGFR is overexpressed in up to 40-80% of NSCLC and was a promising translational therapeutic target however it was subsequently discovered that activating mutations rather than overexpression of EGFR was the prime therapeutic target. The two most common mutations are exon 19 deletions (60%) and L858R missense substitutions at position 858 (35%) where leucine is replaced by arginine resulting in constitutive activation of the receptor without ligand binding (21-23). Mutant EGFR can be inhibited either by small molecule TKI (such as gefitinib and erlotinib) or mAb (such as cetuximab).
Gefitinib and erlotinib were the first EGFR TKIs to be developed. Both are reversible competitive inhibitors of ATP for the tyrosine kinase domain of EGFR resulting in blockade of downstream pathways. Early trials used EGFR TKIs in an unselected population as these predated the now known clinical and molecular predictive biomarkers (24-28). As trials matured, subgroup analyses identified characteristics that correlated with response such as adenocarcinoma histology, Asian ethnicity and minimal smoking history (24-26,29-34). Molecular testing of tissue samples from those who had responded to TKIs revealed that somatic activating mutations in EGFR underpinned the responses seen (29,30,35-37). The incidence of EGFR mutation varies with ethnicity, with Asian populations having up to 50% of adenocarcinomas driven by activating EGFR mutations compared to only 10% to 15% in Caucasians (37). Unfortunately, there are no reliable clinical phenotypes or characteristics that allow for accurate prediction of an EGFR mutation, thus all tumours must undergo specific mutational testing (38).
EGFR-mutant NSCLC
The most significant paradigm change in the last 10 years for NSCLC management was heralded by the use of EGFR TKIs as first-line therapy for patients with a targetable EGFR driver mutation. The landmark Iressa Pan-Asia Study (IPASS) randomised 1,217 patients from several East Asian countries with untreated stage IIIB or IV adenocarcinoma to gefitinib or carboplatin and paclitaxel chemotherapy (Table 1) (39). Subjects were clinically selected with no or minimal smoking history and EGFR was explored as a potential biomarker. IPASS met its primary endpoint with a 12-month progression-free survival (PFS) of 24.9% with gefitinib versus 6.7% with chemotherapy (39). EGFR status was known in approximately a third of patients, and of these, 60% harboured an activating mutation. For these patients, PFS was significantly prolonged with gefitinib compared to chemotherapy [HR 0.48 (95% CI, 0.36-0.64); P<0.001]. Conversely, patients with wild-type EGFR did better with chemotherapy [HR 2.85; (95% CI, 2.05-3.98); P<0.001]. The First-SIGNAL study (41) verified these findings by clinically selecting never smokers with adenocarcinoma then comparing chemotherapy to gefitinib first-line (Table 1). Overall PFS was not significantly different but upon review of patients treated with gefitinib, an activating EGFR mutation did predict for superior overall response rate (ORR) (84.6% vs. 25.9%, P<0.001) and significantly longer PFS (HR 0.377; 95% CI, 0.21-0.67; P<0.001) (41).
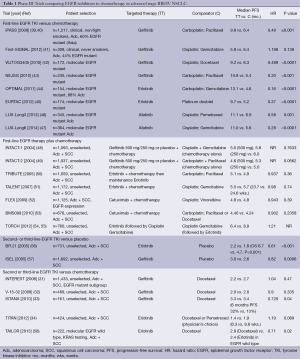
Full table
Further confirmatory trials (Table 1) compared gefitinib (42,43), erlotinib (44,45) or afatinib (46,47) to chemotherapy specifically in EGFR-mutated NSCLC rather than simply by the clinical enrichment criteria of earlier studies. All found that first-line EGFR TKIs afforded superior ORR, PFS and quality of life compared to chemotherapy. Thus upfront tumour interrogation for predictive biomarkers has now become standard and if EGFR demonstrates an activating mutation, then EGFR TKIs should be given as first-line therapy. However, despite mature follow up data for IPASS (40) and other studies, no EGFR TKI in first-line has demonstrated an overall survival benefit most likely due to extensive crossover after progression (59).
Currently, there are no published head to head trials directly comparing the efficacy of first-line EGFR TKIs. In general, these agents all demonstrate similar efficacy so the choice of agent depends on toxicity or clinician preference at the present time (60). Results of the phase IIb LUX-Lung 7 study directly comparing afatinib to gefitinib as first-line treatment for EGFR-mutant adenocarcinoma are eagerly anticipated and may address this (ClinicalTrials.gov Identifier: NCT01466660).
The role of adjuvant EGFR TKIs for resected stage I to III NSCLC remains uncertain (Table 2). Adjuvant erlotinib after surgery, specifically in EGFR-mutants, is currently being investigated in the RADIANT trial, with or without chemotherapy and is expected to complete in 2016 (ClinicalTrials.gov identifier: NCT00373425). Data from this study will be particularly interesting as a previous trial, NCIC BR19 (66), in an unselected patient population using adjuvant gefitinib, proved negative.
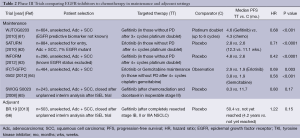
Full table
EGFR wild type and EGFR-unknown advanced NSCLC
Most tumours do not harbour an activating EGFR mutation (known as EGFR wild-type) and the role of TKIs in this specific population is contentious. With regards to first-line therapy, guidelines discourage the use of first-line TKIs based on the IPASS (39,40) and TORCH (54,55) trials which both demonstrated inferior survival compared to up-front chemotherapy (67,68). For second-line therapy (Table 1), the TAILOR trial (58) compared erlotinib to docetaxel specifically in EGFR wild-type tumours. All endpoints of ORR, PFS and overall survival (OS), were significantly better with docetaxel compared to erlotinib (58). This supports the continuing role for cytotoxic chemotherapy as the preferred therapeutic option in NSCLC without targetable driver mutations (69).
Four trials investigated whether adding EGFR TKIs to standard platinum doublet chemotherapy could improve outcomes (Table 1). The INTACT 1 (48) and INTACT 2 (49) looked at gefitinib whereas the TRIBUTE (50) and TALENT (51) trials used erlotinib. All proved to be negative trials with no improvement in efficacy or survival compared to standard chemotherapy alone.
The prognosis for patients remains poor for those who progress after initial platinum doublet chemotherapy. Both docetaxel (70) and pemetrexed (71) are approved active agents in the second-line setting, but more therapeutic options were needed, especially for those unable to have further chemotherapy. The INTEREST study was a multinational phase III randomised trial that compared gefitinib to docetaxel in unselected second-line patients (Table 1) (31). Gefitinib was non-inferior with respect to median OS of 7.6 months with gefitinib and 8.0 months with docetaxel, HR 1.02 (95% CI, 0.905-1.150). Further trials with second-line gefitinib (32,33) and erlotinib (34) showed superior response rates, PFS and quality of life without significant differences in OS compared to chemotherapy.
For patients with unknown EGFR status who are unfit for chemotherapy, the phase III TOPICAL study (72) found a significant survival benefit with first-line erlotinib over placebo but only in those who developed a rash within 28 days. It should be noted that those who failed to develop a rash with erlotinib had inferior survival compared to placebo. Two early phase III trials investigated EGFR TKIs versus placebo in second- or third-line in unselected patients, prior to knowledge of predictive biomarkers (Table 1) (56,57). The BR.21 trial (56) was the first, and still the only phase III trial to show an overall survival benefit from an EGFR TKI (59). Survival with erlotinib was 6.7 months compared to 4.7 months with placebo (HR 0.70; 95% CI, 0.58-0.85; P<0.001) (56). In contrast, gefitinib failed to show a significant survival benefit in the ISEL trial (57). Icotinib, a novel EGFR TKI has also demonstrated non-inferiority in a head to head trial compared to gefitinib in previously treated, unselected advanced NSCLC (73). Therefore in patients with unknown or wild-type EGFR status, who have no further chemotherapy options, erlotinib may be beneficial as second- or third-line therapy after platinum-based chemotherapy.
Switch maintenance therapy to EGFR TKIs after initial induction chemotherapy has shown a modest but statistically significant benefit (Table 2). The WJTOG0203 (61) and INFORM (63) trials used gefitinib whereas SATURN (62) and IFCT-GFPC 0502 (64) showed similar benefits for erlotinib. However the SWOG S0023 study (65) demonstrated no benefit with gefitinib compared to placebo following definitive chemoradiation. In fact, there appeared to potentially be harm from gefitinib in this setting as placebo paradoxically demonstrated a superior PFS and OS.
Anti-EGFR monoclonal antibodies
Monoclonal antibodies represent an alternative way to inhibit EGFR activation and signalling. Apart from competitive inhibition of ligands binding to the extracellular domain, they can also form antibody-receptor complexes that are endocytosed and degraded. Available anti-EGFR mAbs now include cetuximab, necitumumab, panitumumab and matuzumab.Two phase III studies, FLEX (52) and BMS099 (53) have examined the combination of cetuximab with platinum doublet chemotherapy in advanced NSCLC (Table 1). Whilst the FLEX trial demonstrated a marginal improvement in median overall survival (11.3 months with cetuximab versus 10.1 months with chemotherapy alone), the smaller BMS099 trial was negative (52,53). Necitumumab is currently being investigated in two phase III studies. The ongoing INSPIRE study in non-squamous NSCLC (ClinicalTrials.gov identifier: NCT00982111) and the recently completed SQUIRE study for squamous NSCLC investigating cisplatin-gemcitabine with necitumumab. The SQUIRE study reportedly demonstrated an improved OS and formal publication of these results are eagerly anticipated (ClinicalTrials.gov identifier: NCT00981058). Other mAbs currently in phase II trials include panitumumab (ClinicalTrials.gov identifiers: NCT01038037 and NCT01088620) and matuzumab (ClinicalTrials.gov identifier: NCT00111839).
Resistance to EGFR targeted therapy
Although EGFR TKIs have revolutionised treatment of EGFR-mutant NSCLC, most responses have not proved to be durable with many patients progressing after 7-12 months. Resistance can occur primarily (that is, de novo) or develop after exposure to targeted agents, and can exist as resistant clones within a tumour or in different tumours within the same patient. Most will develop ‘acquired resistance’, either through secondary EGFR mutations or activation of EGFR-independent pathways. Clinicians should therefore consider re-biopsy at progression to assess contemporaneous tumour biology (74-77). The most frequent mechanism (~50%) is via concurrent acquisition of a mutation in exon 20 of EGFR, encoding for T790M (74-80). Threonine is replaced by methionine, altering the configuration of the kinase domain and enhancing its affinity (over wild-type) for ATP, with corresponding decreased affinity for first-generation reversible TKIs (81). The second most common mechanism (in 5-20%) involves amplification of MET to circumvent EGFR inhibition via PI3K-AKT-mTOR signalling (74-76). Other resistance mechanisms include mutations in PIK3CA (75), HER2 (79,82), BRAF (83), STAT3 (84), AXL kinase (85), CRKL amplification (86) and in 5%,the unexpected transformation into small cell lung cancer (75,76). Despite significant advances in our understanding of the mechanisms of acquired resistance, up to 30% of resistance is mediated via an unknown mechanism and hence empirical cytotoxic chemotherapy remains the treatment of choice (75).
In contrast to chemotherapy, resistance to targeted therapy can be approached rationally once aberrant pathways are identified. Second-generation irreversible ErbB-family TKIs such as afatinib, which covalently binds to EGFR/HER1 and HER2, can overcome the T790M mutation as seen in LUX-Lung1 with 7% ORR and PFS improved from 1.1 months with placebo to 3.3 months (HR 0.38; 95% CI, 0.31-0.48, P<0.0001) (87,88). Dual EGFR blockade with EGFR TKIs and cetuximab are now being tested after success in murine models (89-91). Combined inhibition of MET and T790M has also shown promise in murine models (92) and is now undergoing clinical trials in humans with a MET/ALK inhibitor (crizotinib) plus a pan-HER inhibitor (dacomitinib) (ClinicalTrials.gov identifier: NCT01121575). Third generation EGFR TKIs such as CO-1868 and AP26113 that specifically target T790M have preliminary evidence of efficacy in acquired resistance with reasonable toxicity (93,94). Although addressing resistance to targeted therapy appears possible, the challenge for the future will be rationally choosing combinations and whether upfront combination therapy will be more effective than first-line single-agents whilst balancing toxicity and costs.
EML4-ALK positive NSCLC
The ALK gene was first discovered in 1994 in the context of a subtype of Non-Hodgkin lymphoma where ALK was fused to nucleophosmin (NPM) as a result of a chromosomal translocation (95). In 2007, Soda et al. screened NSCLC tumours and found the same ALK gene but this time fused to Echinoderm Microtubule-associated protein-like protein 4 (EML4) as a result of a small inversion within chromosome 2p (96). The EML4-ALK fused oncogene is present in up to 3-7% of NSCLC and promotes malignant growth and proliferation (96). As with EGFR, ALK rearrangements are more likely to be seen in specific populations; younger patients who are light or never-smokers with adenocarcinoma and frequent signet ring cells seen on histology (97-101). Tumours carrying ALK rearrangements are mutually exclusive from those harbouring EGFR or KRAS mutations and represent the prototype for ‘oncogene addiction’ where a single gene product can result in malignancy (97,102,103).
Unlike the history of EGFR, lessons learnt since have allowed a more logical approach for ALK as a therapeutic target; from discovery, prospective tumour genotyping and specifically designed trials to test inhibitors and achieve positive patient outcomes. Crizotinib is an oral small molecule inhibitor of the ALK, MET and ROS tyrosine kinases (104). It was granted FDA approval in 2011 after only phase I/II studies showed impressive response rates (ORR 57%, including one complete response) in pre-treated patients (98). Final results revealed a PFS of 9.7 months (95% CI, 7.7-12.8 months) (105). Median OS data are awaited but a retrospective analysis of ALK-positive NSCLC suggests that crizotinib is associated with a survival advantage compared to those who did not have crizotinib available (106). Importantly, ALK-positivity itself is not a favourable prognostic factor as those without treatment have similar poor outcomes to the general population of NSCLC (106).
Crizotinib has also proved its superiority over second-line chemotherapy in those who had previously received a platinum doublet (101). Median PFS was 7.7 months with crizotinib versus 3.0 months with pemetrexed or docetaxel chemotherapy (HR 0.49; 95% CI, 0.37-0.64, P<0.001) (101). Overall survival was no different, likely due to extensive crossover and immature follow up for survival. This was all achieved with relatively few adverse effects, mainly mild visual disturbances (photopsia, blurred vision) and gastrointestinal side effects. Elevations in liver aminotransferases were severe in 16%, and one progressed to fatal hepatic failure. Interstitial lung disease was seen in 2% with two fatalities. Overall patients still reported superior reduction of symptoms and improvements in overall quality of life with crizotinib (101).
The phase III PROFILE 1014 study is currently investigating crizotinib as first-line therapy compared to platinum-pemetrexed chemotherapy in untreated patients and has now completed recruitment (ClinicalTrials.gov Identifier: NCT01154140). Results are expected shortly and if positive, will cement crizotinib as the gold standard treatment for all lines of therapy for ALK-positive NSCLC.
As with EGFR TKIs, resistance can also develop to crizotinib for ALK rearranged NSCLC. Unfortunately a wide variety of mechanisms are being discovered including; ALK amplification, EGFR/HER1, HER2 and HER3 up-regulation, cKIT amplification and various ALK mutations including L1196M (analogous to T790M for EGFR) (107-110). In those with acquired resistance to crizotinib, a phase I trial has just shown that a second-generation ALK inhibitor, ceritinib (LDK378), had an ORR of 56% (95% CI, 45-67%) (111). It is up to 20 times a more potent ALK inhibitor than crizotinib, explaining its potential to overcome the L1196M mutation (111-113). Particularly encouraging is that response rates were similar for patients with various known resistance mechanisms as well as those without an identifiable mutation (114). Other similar second generation ALK inhibitors such as alectinib are under investigation but, as is the case with EGFR, a rational approach to overcoming ALK-resistance holds promise for the future (115-117).
K-RAS mutation in NSCLC
K-RAS (Kirsten rat sarcoma 2 viral oncogene homolog) belongs to a family of GTPases that transduce growth signals from multiple tyrosine kinases including EGFR and MET (Figure 2) (18). Activating mutations in KRAS leading to constitutive signalling are present in about 30% of adenocarcinoma and 4% of SCC (118,119). KRAS mutations are more likely to be found in Caucasians, former or current smokers and are mutually exclusive from EGFR or ALK mutations (103,119-121). They have also been associated with a poorer prognosis as well as resistance to chemotherapy and EGFR TKIs (122-125). Despite KRAS being one of the earliest known oncogenic drivers in NSCLC (126), effective targeting remains a therapeutic challenge. Direct RAS inhibition with salirasib was unsuccessful (127), so novel approaches are currently attempting to inhibit downstream molecules in the RAS/RAF/MEK/ERK and PI3K/AKT/mTOR pathways (119). Other approaches include targeting the heat shock protein (HSP90) which KRAS mutant cells have increased dependence upon (92,119). Selumetinib (AZD6244; ARRY-142866) a MEK1/MEK2 inhibitor showed a PFS advantage when combined with docetaxel in a recent phase II trial in advanced KRAS-mutant NSCLC (128). It is now being investigated in a confirmatory phase III study, SELECT-1 (ClinicalTrials.gov Identifier: NCT01933932), in addition to preclinical combinations with AKT inhibitors (129).
MET amplification in NSCLC
Amplification of mesenchymal-epithelial transition (MET) factor is found in about 5% of lung adenocarcinoma and results in overexpression of its gene product—hepatocyte growth factor receptor (HGFR)—which is involved in cell proliferation, migration, invasion and metastasis (130). Various strategies to inhibit MET/HGFR mediated growth are in development including: HGF antagonists, anti-HGFR mAb, anti-MET mAb and MET TKIs such as tivantinib (ARQ197), cabozantinib (XL184) and of course crizotinib (131).
MET and EGFR appear to be synergistic for growth and MET amplification is also the second most common cause of acquired EGFR TKI resistance. Dual EGFR and MET inhibition, with erlotinib and tivantinib respectively, was tested in non-squamous NSCLC in the much anticipated global phase III trial MARQUEE (132), after phase II data (133) suggested improved PFS for KRAS-mutants. Onartuzumab, a monoclonal antibody against MET also showed promise in a phase II trial (134) so was brought to phase III in the MetLung study where it was combined with erlotinib for MET-positive NSCLC (ClinicalTrials.gov Identifier: NCT01456325) (135).
Despite these early promising results, confirmatory studies using MET TKIs and MET mAb have yielded disappointing results and early trial closures for both phase III trials. MARQUEE (132) was closed in late 2012 due to an interim analysis declaring futility in its primary outcome of overall survival (136). MetLung was also terminated early due to lack of efficacy (137). Interestingly, subset analyses from MARQUEE were presented at the European Cancer Conference 2013, which suggested that in tumours with strong MET immunostaining, there was a PFS and OS benefit (138). Only 40% of tumours in MARQUEE had tissue for MET expression analysis and it appears that the future progress with MET inhibition is likely to require a clear predictive biomarker to enhance appropriate patient selection moving into the future.
ROS1 rearrangements in NSCLC
ROS1 rearrangements were first seen in 2007 with around 1-2% of NSCLC harbouring different ROS1 fusion variants (139,140). Whilst its function in humans is yet unknown, its highest expression is seen in normal lung tissue (141). Like many other receptor tyrosine kinases, ROS1 feeds into multiple downstream pathways such as the RAS/RAF/MEK or MAPK, JAK/STAT3 and PI3K/AKT/mTOR pathways (Figure 2) (141,142). Both rearrangements share similar clinical phenotypes: younger, non-smokers with adenocarcinomas (141,143). There also appears to be ~50% sequence homology between ROS1 and ALK, and fortunately ALK inhibitors such as crizotinib can and do inhibit both kinases (139,141). Indeed crizotinib has shown some early activity in the phase I setting (144), but again, acquired resistance appears to limit the long-term efficacy of kinase inhibition (ClinicalTrials.gov Identifiers: NCT01449461, NCT01284192) and specific ROS1 inhibitors, such as foretinib are currently under investigation (145).
RET fusions in NSCLC
The RET (rearranged during transfection) is a novel fusion gene with various partners including KIF5B (kinesin family member 5B) and others such as CCDC6, NCOA4, and TRIM33 (146). It is found in around 1-2% of lung adenocarcinomas and predominantly in non-smokers (143,147). No specific RET inhibitors are currently available but multi-kinase inhibitors such as vandetanib (phase II) and cabozantinib (phase III) are being trialled in RET fusion-positive NSCLC (ClinicalTrials.gov Identifiers: NCT01823068 and NCT01639508).
HER2 overexpression and mutations in NSCLC
Human epidermal growth factor 2 (HER2/ErbB2/neu), like EGFR/HER1, is a member of the ErbB family of tyrosine kinase receptors that are activated by homo- or hetero-dimerisation with other ErbB receptors (21). HER2 overexpression is seen in up to 20% of NSCLC (148,149) but HER2 mutation rates occur less frequently in up to 3-4% (149,150). Rationale for blockade in NSCLC was borrowed from successes seen in HER2-positive breast cancer (9), however phase II trials combining trastuzumab with chemotherapy in NSCLC have so far been negative to date (148,149).
BRAF mutations in NSCLC
BRAF mutations in NSCLC are uncommon and seen in less than 5% of cases (151). As an important part of the RAS/RAF/MEK/ERK or MAPK pathway, BRAF inhibition seemed logical, especially since TKIs were already available for melanoma (10). However, only around half of those identified harbour the specific V600E mutation for which effective therapies exist (151). Currently a phase II trial is looking at the combination of a BRAF and MEK inhibitor, dabrafenib and trametinib respectively, in stage IV NSCLC (ClinicalTrials.gov Identifier: NCT01336634).
Squamous cell carcinomas (SCC)
Although many of the pathways and targeted agents described thus far apply primarily to adenocarcinoma, targeted therapy for SCC is now a focus of current research. Recent discoveries from the cancer genome atlas about the molecular pathology of SCC have identified several important signalling pathways (152). Although these pathways can be inhibited, clinically meaningful benefits are currently lacking but ongoing work should hopefully see the realisation of targeted agents for SCC in the near future.
The phosphatidylinositol 3-kinase (PIK3CA) pathway is one of the most commonly altered in SCC with PIK3CA mutation and amplification as well as loss of the PTEN tumour suppressor gene (4,153). Ongoing phase II trials of the PI3K inhibitor, buparlisib (BKM120) are underway in squamous NSCLC in combination with chemotherapy (ClinicalTrials.gov Identifiers: NCT01911325, NCT01820325).
The fibroblast growth factor receptor 1 (FGFR1) is another exploitable pathway with overexpression in up to 20% of SCC compared to only 3% of adenocarcinoma (154). FGFR inhibitors, such as brivanib (BMS-582664) and other multi-kinase inhibitors showed positive signals in vitro (154) and are now in early phase trials (ClinicalTrials.gov Identifier: NCT00633789) (155).
DDR2 (discoidin domain receptor 2) is a tyrosine kinase receptor seen in up to 4% of SCC (156). Again DDR2, with collagen as its ligand, is involved in cell migration, proliferation and survival (156). Early promise was seen in vitro and in murine models of DDR2 inhibition with dasatinib, a multi-TKI targeting BCR-Abl and the Src family of tyrosine kinases (156). The phase II trial was negative (157) but further research on DDR2 inhibition is ongoing.
Angiogenesis inhibition in NSCLC
Disrupting tumour blood supply and angiogenesis has been a enticing target for many years now (158) with some successes in other malignancies such as colorectal cancer (159), ovarian (160) and now cervical cancer (161). Complex signalling pathways with multiple growth factors and cytokines are thought to regulate angiogenesis (162,163). Two key growth factors include vascular endothelial growth factor (VEGF) and platelet derived growth factor (PDGF) (162,163).
Two pivotal phase III trials provide evidence for targeting angiogenesis in NSCLC with both utilising the anti-VEGF monoclonal antibody, bevacizumab in combination with standard platinum chemotherapy doublets (164-166). The Eastern Cooperative Oncology Group ECOG 4599 study (164) reported a median OS advantage from 10.3 months with chemotherapy alone to 12.3 months with the addition of bevacizumab to chemotherapy and as maintenance (HR 0.79; 95% CI, 0.67-0.91; P=0.003). The AVAiL study (165) demonstrated an improved ORR and longer PFS although failed to demonstrate an improvement in overall survival. Toxicities with bevacizumab include bleeding, thromoboembolism, and hypertension (164,165). Major bleeding and haemoptysis was associated with squamous histology and cavitation, thus limiting its clinical use to non-squamous NSCLC after fatal pulmonary haemorrhagic events were noted in earlier phase II studies (164,167,168). A further phase III study (AVAPERL) in non-squamous NSCLC suggests that perhaps maintenance therapy with pemetrexed is improved by the addition of bevacizumab (169,170).
Small molecule TKI can also be utilised to inhibit the VEGF pathway. To date, several multi-TKIs have failed to demonstrate a clinically significant survival benefit in phase III trials (171-175). Nintedanib combined with second-line chemotherapy (LUME-Lung1) resulted in a very modest benefit in PFS without a benefit in OS, however, planned subgroup analyses suggest that patients with adenocarcinoma histology may benefit most (12.6 months with nintedanib plus docetaxel versus 10.3 months with docetaxel alone (HR 0.83; 95% CI, 0.70-0.99; P=0.0359) (176).
A novel class of anti-angiogenesis drugs known as tumour vascular disrupting agents did show some promise in pre-clinical trials. However vadimezan (ASA404) failed to show a benefit in phase III trials (177) and so further development has been abandoned. Further research is needed to elucidate appropriate predictive biomarkers for anti-angiogenic therapies in the future.
Conclusions
Within the last decade, significant advances in molecular pathology have afforded an improved understanding of the underlying pathology and significant heterogeneity of NSCLC. Multiple signalling pathways have now been identified as well as specific oncogenic driver mutations that lead to malignant transformations. Indeed in clinical practice, reflex molecular interrogation of tumour tissue for such driver mutations has now become commonplace. For the vast majority at present, no known drivers are detected and such patients are still empirically treated with standard cytotoxic chemotherapy. Whilst impressive clinical benefits have been observed for NSCLC with a known driver mutation, acquired resistance is frequently seen and presents us with the next challenge in the goal to deliver unique personalised medicine.
Building on past experience is helping to improve the approach to targeted therapy. For example, it took just over six years to progress from initiation of phase I to positive phase III trials of crizotinib in ALK-positive patients and just four years to achieve FDA approval with only phase II data—a truly remarkable achievement. The key to the future success of theranostics and truly personalised oncological management will be to ensure appropriate patient selection using predictive biomarkers to optimise limited resources and minimise harm. Addressing resistance, utilising the correct inhibitor, or combination of inhibitors, whilst minimising adverse effects will hopefully lead to the realisation of ongoing improvements in survival for patients in the future. Further to this, the real challenge will be bringing these agents into the management of patients with earlier stage disease with the hope of truly improving rates of cure for the devastating illness that is lung cancer.
Acknowledgements
Disclosure: Dr. Chan has no disclosures to declare. Dr. Hughes’ disclosures are Advisory Board for Roche and Boehringer Ingelheim.
References
- Ferlay J, Soerjomataram I, Ervik M, et al. GLOBOCAN 2012 v1.0, Cancer Incidence and Mortality Worldwide: IARC CancerBase No. 11 [Internet]. Lyon, France: International Agency for Research on Cancer; 2013 [cited 2014 March 21]. Available online: http://globocan.iarc.fr, accessed on 21/03/2014.
- Chan BA, Coward JI. Chemotherapy advances in small-cell lung cancer. J Thorac Dis 2013;5:S565-78. [PubMed]
- Weinstein IB, Begemann M, Zhou P, et al. Disorders in cell circuitry associated with multistage carcinogenesis: exploitable targets for cancer prevention and therapy. Clin Cancer Res 1997;3:2696-702. [PubMed]
- Hanahan D, Weinberg RA. Hallmarks of cancer: the next generation. Cell 2011;144:646-74. [PubMed]
- Ding L, Getz G, Wheeler DA, et al. Somatic mutations affect key pathways in lung adenocarcinoma. Nature 2008;455:1069-75. [PubMed]
- Pao W, Girard N. New driver mutations in non-small-cell lung cancer. Lancet Oncol 2011;12:175-80. [PubMed]
- Dearden S, Stevens J, Wu YL, et al. Mutation incidence and coincidence in non small-cell lung cancer: meta-analyses by ethnicity and histology (mutMap). Ann Oncol 2013;24:2371-6. [PubMed]
- Daniels MG, Bowman RV, Yang IA, et al. An emerging place for lung cancer genomics in 2013. J Thorac Dis 2013;5:S491-7. [PubMed]
- Moja L, Tagliabue L, Balduzzi S, et al. Trastuzumab containing regimens for early breast cancer. Cochrane Database Syst Rev 2012;4:CD006243. [PubMed]
- Chapman PB, Hauschild A, Robert C, et al. Improved survival with vemurafenib in melanoma with BRAF V600E mutation. N Engl J Med 2011;364:2507-16. [PubMed]
- Greulich H. The genomics of lung adenocarcinoma: opportunities for targeted therapies. Genes Cancer 2010;1:1200-10. [PubMed]
- West L, Vidwans SJ, Campbell NP, et al. A novel classification of lung cancer into molecular subtypes. PLoS One 2012;7:e31906. [PubMed]
- Dacic S, Nikiforova MN. Present and future molecular testing of lung carcinoma. Adv Anat Pathol 2014;21:94-9. [PubMed]
- Paz-Ares L, de Marinis F, Dediu M, et al. Maintenance therapy with pemetrexed plus best supportive care versus placebo plus best supportive care after induction therapy with pemetrexed plus cisplatin for advanced non-squamous non-small-cell lung cancer (PARAMOUNT): a double-blind, phase 3, randomised controlled trial. Lancet Oncol 2012;13:247-55. [PubMed]
- Paz-Ares LG, de Marinis F, Dediu M, et al. PARAMOUNT: Final overall survival results of the phase III study of maintenance pemetrexed versus placebo immediately after induction treatment with pemetrexed plus cisplatin for advanced nonsquamous non-small-cell lung cancer. Journal of clinical oncology: official journal of the American Society of Clinical Oncology 2013;31:2895-902. [PubMed]
- Johnson DH, Fehrenbacher L, Novotny WF, et al. Randomized phase II trial comparing bevacizumab plus carboplatin and paclitaxel with carboplatin and paclitaxel alone in previously untreated locally advanced or metastatic non-small-cell lung cancer. J Clin Oncol 2004;22:2184-91. [PubMed]
- Thunnissen E, van der Oord K, den Bakker M. Prognostic and predictive biomarkers in lung cancer. A review. Virchows Arch 2014;464:347-58. [PubMed]
- Alamgeer M, Ganju V, Watkins DN. Novel therapeutic targets in non-small cell lung cancer. Curr Opin Pharmacol 2013;13:394-401. [PubMed]
- Savas P, Hughes B, Solomon B. Targeted therapy in lung cancer: IPASS and beyond, keeping abreast of the explosion of targeted therapies for lung cancer. J Thorac Dis 2013;5:S579-92. [PubMed]
- Arteaga CL. The epidermal growth factor receptor: from mutant oncogene in nonhuman cancers to therapeutic target in human neoplasia. J Clin Oncol 2001;19:32S-40S. [PubMed]
- Yarden Y, Sliwkowski MX. Untangling the ErbB signalling network. Nat Rev Mol Cell Biol 2001;2:127-37. [PubMed]
- Jackman DM, Yeap BY, Sequist LV, et al. Exon 19 deletion mutations of epidermal growth factor receptor are associated with prolonged survival in non-small cell lung cancer patients treated with gefitinib or erlotinib. Clin Cancer Res 2006;12:3908-14. [PubMed]
- Rosell R, Moran T, Queralt C, et al. Screening for epidermal growth factor receptor mutations in lung cancer. N Engl J Med 2009;361:958-67. [PubMed]
- Fukuoka M, Yano S, Giaccone G, et al. Multi-institutional randomized phase II trial of gefitinib for previously treated patients with advanced non-small-cell lung cancer (The IDEAL 1 Trial) J Clin Oncol 2003;21:2237-46. [PubMed]
- Kris MG, Natale RB, Herbst RS, et al. Efficacy of gefitinib, an inhibitor of the epidermal growth factor receptor tyrosine kinase, in symptomatic patients with non-small cell lung cancer: a randomized trial. JAMA 2003;290:2149-58. [PubMed]
- Pérez-Soler R, Chachoua A, Hammond LA, et al. Determinants of tumor response and survival with erlotinib in patients with non--small-cell lung cancer. J Clin Oncol 2004;22:3238-47. [PubMed]
- Perez-Soler R. Phase II clinical trial data with the epidermal growth factor receptor tyrosine kinase inhibitor erlotinib (OSI-774) in non-small-cell lung cancer. Clin Lung Cancer 2004;6 Suppl 1:S20-3. [PubMed]
- Perez-Soler R. The role of erlotinib (Tarceva, OSI 774) in the treatment of non-small cell lung cancer. Clin Cancer Res 2004;10:4238s-40s. [PubMed]
- Miller VA, Kris MG, Shah N, et al. Bronchioloalveolar pathologic subtype and smoking history predict sensitivity to gefitinib in advanced non-small-cell lung cancer. J Clin Oncol 2004;22:1103-9. [PubMed]
- Giaccone G. Epidermal growth factor receptor inhibitors in the treatment of non-small-cell lung cancer. J Clin Oncol 2005;23:3235-42. [PubMed]
- Kim ES, Hirsh V, Mok T, et al. Gefitinib versus docetaxel in previously treated non-small-cell lung cancer (INTEREST): a randomised phase III trial. Lancet 2008;372:1809-18. [PubMed]
- Maruyama R, Nishiwaki Y, Tamura T, et al. Phase III study, V-15-32, of gefitinib versus docetaxel in previously treated Japanese patients with non-small-cell lung cancer. J Clin Oncol 2008;26:4244-52. [PubMed]
- Lee DH, Park K, Kim JH, et al. Randomized Phase III trial of gefitinib versus docetaxel in non-small cell lung cancer patients who have previously received platinum-based chemotherapy. Clin Cancer Res 2010;16:1307-14. [PubMed]
- Ciuleanu T, Stelmakh L, Cicenas S, et al. Efficacy and safety of erlotinib versus chemotherapy in second-line treatment of patients with advanced, non-small-cell lung cancer with poor prognosis (TITAN): a randomised multicentre, open-label, phase 3 study. Lancet Oncol 2012;13:300-8. [PubMed]
- Lynch TJ, Bell DW, Sordella R, et al. Activating mutations in the epidermal growth factor receptor underlying responsiveness of non-small-cell lung cancer to gefitinib. N Engl J Med 2004;350:2129-39. [PubMed]
- Paez JG, Janne PA, Lee JC, et al. EGFR mutations in lung cancer: correlation with clinical response to gefitinib therapy. Science 2004;304:1497-500. [PubMed]
- Pao W, Miller V, Zakowski M, et al. EGF receptor gene mutations are common in lung cancers from “never smokers” and are associated with sensitivity of tumors to gefitinib and erlotinib. Proc Natl Acad Sci U S A 2004;101:13306-11. [PubMed]
- Keedy VL, Temin S, Somerfield MR, et al. American Society of Clinical Oncology provisional clinical opinion: epidermal growth factor receptor (EGFR) Mutation testing for patients with advanced non-small-cell lung cancer considering first-line EGFR tyrosine kinase inhibitor therapy. J Clin Oncol 2011;29:2121-7. [PubMed]
- Mok TS, Wu YL, Thongprasert S, et al. Gefitinib or carboplatin-paclitaxel in pulmonary adenocarcinoma. N Engl J Med 2009;361:947-57. [PubMed]
- Fukuoka M, Wu YL, Thongprasert S, et al. Biomarker analyses and final overall survival results from a phase III, randomized, open-label, first-line study of gefitinib versus carboplatin/paclitaxel in clinically selected patients with advanced non-small-cell lung cancer in Asia (IPASS). J Clin Oncol 2011;29:2866-74. [PubMed]
- Han JY, Park K, Kim SW, et al. First-SIGNAL: first-line single-agent iressa versus gemcitabine and cisplatin trial in never-smokers with adenocarcinoma of the lung. J Clin Oncol 2012;30:1122-8. [PubMed]
- Mitsudomi T, Morita S, Yatabe Y, et al. Gefitinib versus cisplatin plus docetaxel in patients with non-small-cell lung cancer harbouring mutations of the epidermal growth factor receptor (WJTOG3405): an open label, randomised phase 3 trial. Lancet Oncol 2010;11:121-8. [PubMed]
- Maemondo M, Inoue A, Kobayashi K, et al. Gefitinib or chemotherapy for non-small-cell lung cancer with mutated EGFR. N Engl J Med 2010;362:2380-8. [PubMed]
- Zhou C, Wu YL, Chen G, et al. Erlotinib versus chemotherapy as first-line treatment for patients with advanced EGFR mutation-positive non-small-cell lung cancer (OPTIMAL, CTONG-0802): a multicentre, open-label, randomised, phase 3 study. Lancet Oncol 2011;12:735-42. [PubMed]
- Rosell R, Carcereny E, Gervais R, et al. Erlotinib versus standard chemotherapy as first-line treatment for European patients with advanced EGFR mutation-positive non-small-cell lung cancer (EURTAC): a multicentre, open-label, randomised phase 3 trial. Lancet Oncol 2012;13:239-46. [PubMed]
- Sequist LV, Yang JC, Yamamoto N, et al. Phase III study of afatinib or cisplatin plus pemetrexed in patients with metastatic lung adenocarcinoma with EGFR mutations. J Clin Oncol 2013;31:3327-34. [PubMed]
- Wu YL, Zhou C, Hu CP, et al. Afatinib versus cisplatin plus gemcitabine for first-line treatment of Asian patients with advanced non-small-cell lung cancer harbouring EGFR mutations (LUX-Lung 6): an open-label, randomised phase 3 trial. Lancet Oncol 2014;15:213-22. [PubMed]
- Giaccone G, Herbst RS, Manegold C, et al. Gefitinib in combination with gemcitabine and cisplatin in advanced non-small-cell lung cancer: a phase III trial--INTACT 1. J Clin Oncol 2004;22:777-84. [PubMed]
- Herbst RS, Giaccone G, Schiller JH, et al. Gefitinib in combination with paclitaxel and carboplatin in advanced non-small-cell lung cancer: a phase III trial--INTACT 2. J Clin Oncol 2004;22:785-94. [PubMed]
- Herbst RS, Prager D, Hermann R, et al. TRIBUTE: a phase III trial of erlotinib hydrochloride (OSI-774) combined with carboplatin and paclitaxel chemotherapy in advanced non-small-cell lung cancer. J Clin Oncol 2005;23:5892-9. [PubMed]
- Gatzemeier U, Pluzanska A, Szczesna A, et al. Phase III study of erlotinib in combination with cisplatin and gemcitabine in advanced non-small-cell lung cancer: the Tarceva Lung Cancer Investigation Trial. J Clin Oncol 2007;25:1545-52. [PubMed]
- Pirker R, Pereira JR, Szczesna A, et al. Cetuximab plus chemotherapy in patients with advanced non-small-cell lung cancer (FLEX): an open-label randomised phase III trial. Lancet 2009;373:1525-31. [PubMed]
- Lynch TJ, Patel T, Dreisbach L, et al. Cetuximab and first-line taxane/carboplatin chemotherapy in advanced non-small-cell lung cancer: results of the randomized multicenter phase III trial BMS099. J Clin Oncol 2010;28:911-7. [PubMed]
- Gridelli C, Ciardiello F, Gallo C, et al. First-line erlotinib followed by second-line cisplatin-gemcitabine chemotherapy in advanced non-small-cell lung cancer: the TORCH randomized trial. J Clin Oncol 2012;30:3002-11. [PubMed]
- Gridelli C, Butts C, Ciardiello F, et al. An international, multicenter, randomized phase III study of first-line erlotinib followed by second-line cisplatin/gemcitabine versus first-line cisplatin/gemcitabine followed by second-line erlotinib in advanced non-small-cell lung cancer: treatment rationale and protocol dynamics of the TORCH trial. Clin Lung Cancer 2008;9:235-8. [PubMed]
- Shepherd FA, Rodrigues Pereira J, Ciuleanu T, et al. Erlotinib in previously treated non-small-cell lung cancer. N Engl J Med 2005;353:123-32. [PubMed]
- Thatcher N, Chang A, Parikh P, et al. Gefitinib plus best supportive care in previously treated patients with refractory advanced non-small-cell lung cancer: results from a randomised, placebo-controlled, multicentre study (Iressa Survival Evaluation in Lung Cancer). Lancet 2005;366:1527-37. [PubMed]
- Garassino MC, Martelli O, Broggini M, et al. Erlotinib versus docetaxel as second-line treatment of patients with advanced non-small-cell lung cancer and wild-type EGFR tumours (TAILOR): a randomised controlled trial. Lancet Oncol 2013;14:981-8. [PubMed]
- Lee CK, Brown C, Gralla RJ, et al. Impact of EGFR inhibitor in non-small cell lung cancer on progression-free and overall survival: a meta-analysis. J Natl Cancer Inst 2013;105:595-605. [PubMed]
- Liang W, Wu X, Fang W, et al. Network meta-analysis of erlotinib, gefitinib, afatinib and icotinib in patients with advanced non-small-cell lung cancer harboring EGFR mutations. PLoS One 2014;9:e85245. [PubMed]
- Takeda K, Hida T, Sato T, et al. Randomized phase III trial of platinum-doublet chemotherapy followed by gefitinib compared with continued platinum-doublet chemotherapy in Japanese patients with advanced non-small-cell lung cancer: results of a west Japan thoracic oncology group trial (WJTOG0203). J Clin Oncol 2010;28:753-60. [PubMed]
- Cappuzzo F, Ciuleanu T, Stelmakh L, et al. Erlotinib as maintenance treatment in advanced non-small-cell lung cancer: a multicentre, randomised, placebo-controlled phase 3 study. Lancet Oncol 2010;11:521-9. [PubMed]
- Zhang L, Ma S, Song X, et al. Gefitinib versus placebo as maintenance therapy in patients with locally advanced or metastatic non-small-cell lung cancer (INFORM; C-TONG 0804): a multicentre, double-blind randomised phase 3 trial. Lancet Oncol 2012;13:466-75. [PubMed]
- Pérol M, Chouaid C, Pérol D, et al. Randomized, phase III study of gemcitabine or erlotinib maintenance therapy versus observation, with predefined second-line treatment, after cisplatin-gemcitabine induction chemotherapy in advanced non-small-cell lung cancer. J Clin Oncol 2012;30:3516-24. [PubMed]
- Kelly K, Chansky K, Gaspar LE, et al. Phase III trial of maintenance gefitinib or placebo after concurrent chemoradiotherapy and docetaxel consolidation in inoperable stage III non-small-cell lung cancer: SWOG S0023. J Clin Oncol 2008;26:2450-6. [PubMed]
- Goss GD, O’Callaghan C, Lorimer I, et al. Gefitinib versus placebo in completely resected non-small-cell lung cancer: results of the NCIC CTG BR19 study. J Clin Oncol 2013;31:3320-6. [PubMed]
- Peters S, Adjei AA, Gridelli C, et al. Metastatic non-small-cell lung cancer (NSCLC): ESMO Clinical Practice Guidelines for diagnosis, treatment and follow-up. Ann Oncol 2012;23 Suppl 7:vii56-64. [PubMed]
- Ettinger DS, Akerley W, Borghaei H, et al. Non-small cell lung cancer. J Natl Compr Canc Netw 2012;10:1236-71. [PubMed]
- Lee JK, Hahn S, Kim DW, et al. Epidermal growth factor receptor tyrosine kinase inhibitors vs conventional chemotherapy in non-small cell lung cancer harboring wild-type epidermal growth factor receptor: a meta-analysis. JAMA 2014;311:1430-7. [PubMed]
- Shepherd FA, Dancey J, Ramlau R, et al. Prospective randomized trial of docetaxel versus best supportive care in patients with non-small-cell lung cancer previously treated with platinum-based chemotherapy. J Clin Oncol 2000;18:2095-103. [PubMed]
- Hanna N, Shepherd FA, Fossella FV, et al. Randomized phase III trial of pemetrexed versus docetaxel in patients with non-small-cell lung cancer previously treated with chemotherapy. J Clin Oncol 2004;22:1589-97. [PubMed]
- Lee SM, Khan I, Upadhyay S, et al. First-line erlotinib in patients with advanced non-small-cell lung cancer unsuitable for chemotherapy (TOPICAL): a double-blind, placebo-controlled, phase 3 trial. Lancet Oncol 2012;13:1161-70. [PubMed]
- Shi Y, Zhang L, Liu X, et al. Icotinib versus gefitinib in previously treated advanced non-small-cell lung cancer (ICOGEN): a randomised, double-blind phase 3 non-inferiority trial. Lancet Oncol 2013;14:953-61. [PubMed]
- Bean J, Brennan C, Shih JY, et al. MET amplification occurs with or without T790M mutations in EGFR mutant lung tumors with acquired resistance to gefitinib or erlotinib. Proc Natl Acad Sci U S A 2007;104:20932-7. [PubMed]
- Sequist LV, Waltman BA, Dias-Santagata D, et al. Genotypic and histological evolution of lung cancers acquiring resistance to EGFR inhibitors. Sci Transl Med 2011;3:75ra26. [PubMed]
- Arcila ME, Oxnard GR, Nafa K, et al. Rebiopsy of lung cancer patients with acquired resistance to EGFR inhibitors and enhanced detection of the T790M mutation using a locked nucleic acid-based assay. Clin Cancer Res 2011;17:1169-80. [PubMed]
- Kobayashi S, Boggon TJ, Dayaram T, et al. EGFR mutation and resistance of non-small-cell lung cancer to gefitinib. N Engl J Med 2005;352:786-92. [PubMed]
- Oxnard GR, Arcila ME, Sima CS, et al. Acquired resistance to EGFR tyrosine kinase inhibitors in EGFR-mutant lung cancer: distinct natural history of patients with tumors harboring the T790M mutation. Clin Cancer Res 2011;17:1616-22. [PubMed]
- Yu HA, Arcila ME, Rekhtman N, et al. Analysis of tumor specimens at the time of acquired resistance to EGFR-TKI therapy in 155 patients with EGFR-mutant lung cancers. Clin Cancer Res 2013;19:2240-7. [PubMed]
- Sun JM, Ahn MJ, Choi YL, et al. Clinical implications of T790M mutation in patients with acquired resistance to EGFR tyrosine kinase inhibitors. Lung Cancer 2013;82:294-8. [PubMed]
- Yun CH, Mengwasser KE, Toms AV, et al. The T790M mutation in EGFR kinase causes drug resistance by increasing the affinity for ATP. Proc Natl Acad Sci U S A 2008;105:2070-5. [PubMed]
- Takezawa K, Pirazzoli V, Arcila ME, et al. HER2 amplification: a potential mechanism of acquired resistance to EGFR inhibition in EGFR-mutant lung cancers that lack the second-site EGFRT790M mutation. Cancer Discov 2012;2:922-33. [PubMed]
- Ohashi K, Sequist LV, Arcila ME, et al. Lung cancers with acquired resistance to EGFR inhibitors occasionally harbor BRAF gene mutations but lack mutations in KRAS, NRAS, or MEK1. Proc Natl Acad Sci U S A 2012;109:E2127-33. [PubMed]
- Wu K, Chang Q, Lu Y, et al. Gefitinib resistance resulted from STAT3-mediated Akt activation in lung cancer cells. Oncotarget 2013;4:2430-8. [PubMed]
- Zhang Z, Lee JC, Lin L, et al. Activation of the AXL kinase causes resistance to EGFR-targeted therapy in lung cancer. Nat Genet 2012;44:852-60. [PubMed]
- Serizawa M, Takahashi T, Yamamoto N, et al. Genomic aberrations associated with erlotinib resistance in non-small cell lung cancer cells. Anticancer Res 2013;33:5223-33. [PubMed]
- Li D, Ambrogio L, Shimamura T, et al. BIBW2992, an irreversible EGFR/HER2 inhibitor highly effective in preclinical lung cancer models. Oncogene 2008;27:4702-11. [PubMed]
- Miller VA, Hirsh V, Cadranel J, et al. Afatinib versus placebo for patients with advanced, metastatic non-small-cell lung cancer after failure of erlotinib, gefitinib, or both, and one or two lines of chemotherapy (LUX-Lung 1): a phase 2b/3 randomised trial. Lancet Oncol 2012;13:528-38. [PubMed]
- Regales L, Gong Y, Shen R, et al. Dual targeting of EGFR can overcome a major drug resistance mutation in mouse models of EGFR mutant lung cancer. J Clin Invest 2009;119:3000-10. [PubMed]
- Janjigian YY, Groen HJ, Horn L, et al. Activity and tolerability of afatinib (BIBW 2992) and cetuximab in NSCLC patients with acquired resistance to erlotinib or gefitinib. J Clin Oncol 2011;29 (suppl; abstr 7525).
- Janjigian YY, Azzoli CG, Krug LM, et al. Phase I/II trial of cetuximab and erlotinib in patients with lung adenocarcinoma and acquired resistance to erlotinib. Clin Cancer Res 2011;17:2521-7. [PubMed]
- Xu L, Kikuchi E, Xu C, et al. Combined EGFR/MET or EGFR/HSP90 inhibition is effective in the treatment of lung cancers codriven by mutant EGFR containing T790M and MET. Cancer Res 2012;72:3302-11. [PubMed]
- Sequist LV, Soria JC, Gadgeel SM, et al. First-in-human evaluation of CO-1686, an irreversible, selective, and potent tyrosine kinase inhibitor of EGFR T790M. J Clin Oncol 2013;31:abstr 2524.
- Camidge DR, Bazhenova L, Salgia R, et al. First-in-human dose-finding study of the ALK/EGFR inhibitor AP26113 in patients with advanced malignancies: Updated results. J Clin Oncol 2013;31.
- Morris SW, Kirstein MN, Valentine MB, et al. Fusion of a kinase gene, ALK, to a nucleolar protein gene, NPM, in non-Hodgkin’s lymphoma. Science 1995;267:316-7. [PubMed]
- Soda M, Choi YL, Enomoto M, et al. Identification of the transforming EML4-ALK fusion gene in non-small-cell lung cancer. Nature 2007;448:561-6. [PubMed]
- Wong DW, Leung EL, So KK, et al. The EML4-ALK fusion gene is involved in various histologic types of lung cancers from nonsmokers with wild-type EGFR and KRAS. Cancer 2009;115:1723-33. [PubMed]
- Kwak EL, Bang YJ, Camidge DR, et al. Anaplastic lymphoma kinase inhibition in non-small-cell lung cancer. N Engl J Med 2010;363:1693-703. [PubMed]
- Shaw AT, Solomon B, Kenudson MM. Crizotinib and testing for ALK. J Natl Compr Canc Netw 2011;9:1335-41. [PubMed]
- Paik JH, Choe G, Kim H, et al. Screening of anaplastic lymphoma kinase rearrangement by immunohistochemistry in non-small cell lung cancer: correlation with fluorescence in situ hybridization. J Thorac Oncol 2011;6:466-72. [PubMed]
- Shaw AT, Kim DW, Nakagawa K, et al. Crizotinib versus chemotherapy in advanced ALK-positive lung cancer. N Engl J Med 2013;368:2385-94. [PubMed]
- Chen Z, Sasaki T, Tan X, et al. Inhibition of ALK, PI3K/MEK, and HSP90 in murine lung adenocarcinoma induced by EML4-ALK fusion oncogene. Cancer Res 2010;70:9827-36. [PubMed]
- Gainor JF, Varghese AM, Ou SH, et al. ALK rearrangements are mutually exclusive with mutations in EGFR or KRAS: an analysis of 1,683 patients with non-small cell lung cancer. Clin Cancer Res 2013;19:4273-81. [PubMed]
- Cui JJ, Tran-Dubé M, Shen H, et al. Structure based drug design of crizotinib (PF-02341066), a potent and selective dual inhibitor of mesenchymal-epithelial transition factor (c-MET) kinase and anaplastic lymphoma kinase (ALK). J Med Chem 2011;54:6342-63. [PubMed]
- Camidge DR, Bang YJ, Kwak EL, et al. Activity and safety of crizotinib in patients with ALK-positive non-small-cell lung cancer: updated results from a phase 1 study. Lancet Oncol 2012;13:1011-9. [PubMed]
- Shaw AT, Yeap BY, Solomon BJ, et al. Effect of crizotinib on overall survival in patients with advanced non-small-cell lung cancer harbouring ALK gene rearrangement: a retrospective analysis. Lancet Oncol 2011;12:1004-12. [PubMed]
- Doebele RC, Pilling AB, Aisner DL, et al. Mechanisms of resistance to crizotinib in patients with ALK gene rearranged non-small cell lung cancer. Clin Cancer Res 2012;18:1472-82. [PubMed]
- Katayama R1, Shaw AT, Khan TM, et al. Mechanisms of acquired crizotinib resistance in ALK-rearranged lung Cancers. Sci Transl Med 2012;4:120ra17.
- Kim S, Kim TM, Kim DW, et al. Heterogeneity of genetic changes associated with acquired crizotinib resistance in ALK-rearranged lung cancer. J Thorac Oncol 2013;8:415-22. [PubMed]
- Tanizaki J, Okamoto I, Okabe T, et al. Activation of HER family signaling as a mechanism of acquired resistance to ALK inhibitors in EML4-ALK-positive non-small cell lung cancer. Clin Cancer Res 2012;18:6219-26. [PubMed]
- Shaw AT, Kim DW, Mehra R, et al. Ceritinib in ALK-rearranged non-small-cell lung cancer. N Engl J Med 2014;370:1189-97. [PubMed]
- Marsilje TH, Pei W, Chen B, et al. Synthesis, structure-activity relationships, and in vivo efficacy of the novel potent and selective anaplastic lymphoma kinase (ALK) inhibitor 5-chloro-N2-(2-isopropoxy-5-methyl-4-(piperidin-4-yl)phenyl)-N4-(2-(isopropylsulfonyl)phenyl)pyrimidine-2,4-diamine (LDK378) currently in phase 1 and phase 2 clinical trials. J Med Chem 2013;56:5675-90. [PubMed]
- Chen J, Jiang C, Wang S. LDK378: a promising anaplastic lymphoma kinase (ALK) inhibitor. J Med Chem 2013;56:5673-4. [PubMed]
- Friboulet L, Li N, Katayama R, et al. The ALK inhibitor ceritinib overcomes crizotinib resistance in non-small cell lung cancer. Cancer Discov 2014;4:662-73. [PubMed]
- Seto T, Kiura K, Nishio M, et al. CH5424802 (RO5424802) for patients with ALK-rearranged advanced non-small-cell lung cancer (AF-001JP study): a single-arm, open-label, phase 1-2 study. Lancet Oncol 2013;14:590-8. [PubMed]
- Iwama E, Okamoto I, Harada T, et al. Development of anaplastic lymphoma kinase (ALK) inhibitors and molecular diagnosis in ALK rearrangement-positive lung cancer. Onco Targets Ther 2014;7:375-85. [PubMed]
- Perez CA, Velez M, Raez LE, et al. Overcoming the resistance to Crizotinib in patients with Non-Small Cell Lung Cancer harboring EML4/ALK translocation. Lung Cancer 2014;84:110-5. [PubMed]
- Guin S, Ru Y, Wynes MW, et al. Contributions of KRAS and RAL in non-small-cell lung cancer growth and progression. J Thorac Oncol 2013;8:1492-501. [PubMed]
- Suda K, Tomizawa K, Mitsudomi T. Biological and clinical significance of KRAS mutations in lung cancer: an oncogenic driver that contrasts with EGFR mutation. Cancer Metastasis Rev 2010;29:49-60. [PubMed]
- Riely GJ, Kris MG, Rosenbaum D, et al. Frequency and distinctive spectrum of KRAS mutations in never smokers with lung adenocarcinoma. Clin Cancer Res 2008;14:5731-4. [PubMed]
- Johnson ML, Sima CS, Chaft J, et al. Association of KRAS and EGFR mutations with survival in patients with advanced lung adenocarcinomas. Cancer 2013;119:356-62. [PubMed]
- Mascaux C, Iannino N, Martin B, et al. The role of RAS oncogene in survival of patients with lung cancer: a systematic review of the literature with meta-analysis. Br J Cancer 2005;92:131-9. [PubMed]
- Macerelli M, Caramella C, Faivre L, et al. Does KRAS mutational status predict chemoresistance in advanced non-small cell lung cancer (NSCLC)? Lung Cancer 2014;83:383-8. [PubMed]
- Pao W, Wang TY, Riely GJ, et al. KRAS mutations and primary resistance of lung adenocarcinomas to gefitinib or erlotinib. PLoS Med 2005;2:e17. [PubMed]
- Massarelli E, Varella-Garcia M, Tang X, et al. KRAS mutation is an important predictor of resistance to therapy with epidermal growth factor receptor tyrosine kinase inhibitors in non-small-cell lung cancer. Clin Cancer Res 2007;13:2890-6. [PubMed]
- Santos E, Martin-Zanca D, Reddy EP, et al. Malignant activation of a K-ras oncogene in lung carcinoma but not in normal tissue of the same patient. Science 1984;223:661-4. [PubMed]
- Riely GJ, Johnson ML, Medina C, et al. A phase II trial of Salirasib in patients with lung adenocarcinomas with KRAS mutations. J Thorac Oncol 2011;6:1435-7. [PubMed]
- Jänne PA, Shaw AT, Pereira JR, et al. Selumetinib plus docetaxel for KRAS-mutant advanced non-small-cell lung cancer: a randomised, multicentre, placebo-controlled, phase 2 study. Lancet Oncol 2013;14:38-47. [PubMed]
- Meng J, Dai B, Fang B, et al. Combination treatment with MEK and AKT inhibitors is more effective than each drug alone in human non-small cell lung cancer in vitro and in vivo. PLoS One 2010;5:e14124. [PubMed]
- Sattler M, Reddy MM, Hasina R, et al. The role of the c-Met pathway in lung cancer and the potential for targeted therapy. Ther Adv Med Oncol 2011;3:171-84. [PubMed]
- Gelsomino F, Facchinetti F, Haspinger ER, et al. Targeting the MET gene for the treatment of non-small-cell lung cancer. Crit Rev Oncol Hematol 2014;89:284-99. [PubMed]
- Scagliotti GV, Novello S, Schiller JH, et al. Rationale and design of MARQUEE: a phase III, randomized, double-blind study of tivantinib plus erlotinib versus placebo plus erlotinib in previously treated patients with locally advanced or metastatic, nonsquamous, non-small-cell lung cancer. Clin Lung Cancer 2012;13:391-5. [PubMed]
- Sequist LV, von Pawel J, Garmey EG, et al. Randomized phase II study of erlotinib plus tivantinib versus erlotinib plus placebo in previously treated non-small-cell lung cancer. J Clin Oncol 2011;29:3307-15. [PubMed]
- Spigel DR, Ervin TJ, Ramlau RA, et al. Randomized phase II trial of Onartuzumab in combination with erlotinib in patients with advanced non-small-cell lung cancer. J Clin Oncol 2013;31:4105-14. [PubMed]
- Spigel DR, Edelman MJ, Mok T, et al. Treatment Rationale Study Design for the MetLung Trial: A Randomized, Double-Blind Phase III Study of Onartuzumab (MetMAb) in Combination With Erlotinib Versus Erlotinib Alone in Patients Who Have Received Standard Chemotherapy for Stage IIIB or IV Met-Positive Non-Small-Cell Lung Cancer. Clin Lung Cancer 2012;13:500-4. [PubMed]
- Arqule and Daiichi Sankyo announce discontinuation of Phase 3 MARQUEE clinical trial in non-small cell lung cancer Oct 2, 2012 [April 27, 2014]. Available online: http://www.daiichisankyo.com/media_investors/media_relations/press_releases/detail/005871.html
- Genentech Provides Update On Phase III Study Of Onartuzumab in People With Specific Type of Lung Cancer Mar 2, 2014 [27th April 2014]. Available online: http://www.gene.com/media/press-releases/14562/2014-03-02/genentech-provides-update-on-phase-iii-s
- Scagliotti G. eds. MARQUEE: A randomized, double-blind, placebo-controlled, phase 3 trial of tivantinib (ARQ 197) plus erlotinib versus placebo plus erlotinib in previously treated patients with locally advanced or metastatic, non-squamous, non-small-cell lung cancer (NSCLC) #3410. European Cancer Conference 2013; 2013; Brussels, Belgium.
- Bergethon K, Shaw AT, Ou SH, et al. ROS1 rearrangements define a unique molecular class of lung cancers. J Clin Oncol 2012;30:863-70. [PubMed]
- Davies KD, Doebele RC. Molecular pathways: ROS1 fusion proteins in cancer. Clin Cancer Res 2013;19:4040-5. [PubMed]
- Ou SH, Tan J, Yen Y, et al. ROS1 as a ‘druggable’ receptor tyrosine kinase: lessons learned from inhibiting the ALK pathway. Expert Rev Anticancer Ther 2012;12:447-56. [PubMed]
- Davies KD, Le AT, Theodoro MF, et al. Identifying and targeting ROS1 gene fusions in non-small cell lung cancer. Clin Cancer Res 2012;18:4570-9. [PubMed]
- Gainor JF, Shaw AT. Novel targets in non-small cell lung cancer: ROS1 and RET fusions. Oncologist 2013;18:865-75. [PubMed]
- Ou SI, Camidge DR, Engelman J, et al. Clinical activity of Crizotinib in patients with advanced non-small cell lung cancer (NSCLC) harboring ROS1 gene rearrangement (1191PD). Ann Oncol 2012;23:ix389-ix399.
- Davare MA, Saborowski A, Eide CA, et al. Foretinib is a potent inhibitor of oncogenic ROS1 fusion proteins. Proc Natl Acad Sci U S A 2013;110:19519-24. [PubMed]
- Kohno T, Ichikawa H, Totoki Y, et al. KIF5B-RET fusions in lung adenocarcinoma. Nat Med 2012;18:375-7. [PubMed]
- Takeuchi K, Soda M, Togashi Y, et al. RET, ROS1 and ALK fusions in lung cancer. Nat Med 2012;18:378-81. [PubMed]
- Gatzemeier U, Groth G, Butts C, et al. Randomized phase II trial of gemcitabine-cisplatin with or without trastuzumab in HER2-positive non-small-cell lung cancer. Ann Oncol 2004;15:19-27. [PubMed]
- Krug LM, Miller VA, Patel J, et al. Randomized phase II study of weekly docetaxel plus trastuzumab versus weekly paclitaxel plus trastuzumab in patients with previously untreated advanced nonsmall cell lung carcinoma. Cancer 2005;104:2149-55. [PubMed]
- Hsieh AC, Moasser MM. Targeting HER proteins in cancer therapy and the role of the non-target HER3. Br J Cancer 2007;97:453-7. [PubMed]
- Marchetti A, Felicioni L, Malatesta S, et al. Clinical features and outcome of patients with non-small-cell lung cancer harboring BRAF mutations. J Clin Oncol 2011;29:3574-9. [PubMed]
- Rooney M, Devarakonda S, Govindan R. Genomics of squamous cell lung cancer. Oncologist 2013;18:707-16. [PubMed]
- Engelman JA. Targeting PI3K signalling in cancer: opportunities, challenges and limitations. Nat Rev Cancer 2009;9:550-62. [PubMed]
- Dutt A, Ramos AH, Hammerman PS, et al. Inhibitor-sensitive FGFR1 amplification in human non-small cell lung cancer. PLoS One 2011;6:e20351. [PubMed]
- Jonker DJ, Rosen LS, Sawyer MB, et al. A phase I study to determine the safety, pharmacokinetics and pharmacodynamics of a dual VEGFR and FGFR inhibitor, brivanib, in patients with advanced or metastatic solid tumors. Ann Oncol 2011;22:1413-9. [PubMed]
- Hammerman PS, Sos ML, Ramos AH, et al. Mutations in the DDR2 kinase gene identify a novel therapeutic target in squamous cell lung cancer. Cancer Discov 2011;1:78-89. [PubMed]
- Johnson FM, Bekele BN, Feng L, et al. Phase II study of dasatinib in patients with advanced non-small-cell lung cancer. J Clin Oncol 2010;28:4609-15. [PubMed]
- Folkman J. Tumor angiogenesis: therapeutic implications. N Engl J Med 1971;285:1182-6. [PubMed]
- Giantonio BJ, Catalano PJ, Meropol NJ, et al. Bevacizumab in combination with oxaliplatin, fluorouracil, and leucovorin (FOLFOX4) for previously treated metastatic colorectal cancer: results from the Eastern Cooperative Oncology Group Study E3200. J Clin Oncol 2007;25:1539-44. [PubMed]
- Perren TJ, Swart AM, Pfisterer J, et al. A phase 3 trial of bevacizumab in ovarian cancer. N Engl J Med 2011;365:2484-96. [PubMed]
- Tewari KS, Sill MW, Long HJ, et al. Improved survival with bevacizumab in advanced cervical cancer. N Engl J Med 2014;370:734-43. [PubMed]
- Bergers G, Benjamin LE. Tumorigenesis and the angiogenic switch. Nat Rev Cancer 2003;3:401-10. [PubMed]
- Shikada Y, Yonemitsu Y, Koga T, et al. Platelet-derived growth factor-AA is an essential and autocrine regulator of vascular endothelial growth factor expression in non-small cell lung carcinomas. Cancer Res 2005;65:7241-8. [PubMed]
- Sandler A, Gray R, Perry MC, et al. Paclitaxel-carboplatin alone or with bevacizumab for non-small-cell lung cancer. N Engl J Med 2006;355:2542-50. [PubMed]
- Reck M, von Pawel J, Zatloukal P, et al. Overall survival with cisplatin-gemcitabine and bevacizumab or placebo as first-line therapy for nonsquamous non-small-cell lung cancer: results from a randomised phase III trial (AVAiL). Ann Oncol 2010;21:1804-9. [PubMed]
- Sandler A. Bevacizumab in non small cell lung cancer. Clin Cancer Res 2007;13:s4613-6. [PubMed]
- Sandler A, Yi J, Dahlberg S, et al. Treatment outcomes by tumor histology in Eastern Cooperative Group Study E4599 of bevacizumab with paclitaxel/carboplatin for advanced non-small cell lung cancer. J Thorac Oncol 2010;5:1416-23. [PubMed]
- Sandler AB, Schiller JH, Gray R, et al. Retrospective evaluation of the clinical and radiographic risk factors associated with severe pulmonary hemorrhage in first-line advanced, unresectable non-small-cell lung cancer treated with Carboplatin and Paclitaxel plus bevacizumab. J Clin Oncol 2009;27:1405-12. [PubMed]
- Barlesi F, Scherpereel A, Gorbunova V, et al. Maintenance bevacizumab-pemetrexed after first-line cisplatin-pemetrexed-bevacizumab for advanced nonsquamous nonsmall-cell lung cancer: updated survival analysis of the AVAPERL (MO22089) randomized phase III trial. Ann Oncol 2014;25:1044-52. [PubMed]
- Barlesi F, Scherpereel A, Rittmeyer A, et al. Randomized phase III trial of maintenance bevacizumab with or without pemetrexed after first-line induction with bevacizumab, cisplatin, and pemetrexed in advanced nonsquamous non-small-cell lung cancer: AVAPERL (MO22089). J Clin Oncol 2013;31:3004-11. [PubMed]
- Paz-Ares LG, Biesma B, Heigener D, et al. Phase III, randomized, double-blind, placebo-controlled trial of gemcitabine/cisplatin alone or with sorafenib for the first-line treatment of advanced, nonsquamous non-small-cell lung cancer. J Clin Oncol. 2012;30:3084-92. [PubMed]
- Scagliotti G, Novello S, von Pawel J, et al. Phase III study of carboplatin and paclitaxel alone or with sorafenib in advanced non-small-cell lung cancer. J Clin Oncol 2010;28:1835-42. [PubMed]
- Herbst RS, Sun Y, Eberhardt WE, et al. Vandetanib plus docetaxel versus docetaxel as second-line treatment for patients with advanced non-small-cell lung cancer (ZODIAC): a double-blind, randomised, phase 3 trial. Lancet Oncol 2010;11:619-26. [PubMed]
- Lee JS, Hirsh V, Park K, et al. Vandetanib Versus placebo in patients with advanced non-small-cell lung cancer after prior therapy with an epidermal growth factor receptor tyrosine kinase inhibitor: a randomized, double-blind phase III trial (ZEPHYR). J Clin Oncol 2012;30:1114-21. [PubMed]
- de Boer RH, Arrieta Ó, Yang CH, et al. Vandetanib plus pemetrexed for the second-line treatment of advanced non-small-cell lung cancer: a randomized, double-blind phase III trial. J Clin Oncol 2011;29:1067-74. [PubMed]
- Reck M, Kaiser R, Mellemgaard A, et al. Docetaxel plus nintedanib versus docetaxel plus placebo in patients with previously treated non-small-cell lung cancer (LUME-Lung 1): a phase 3, double-blind, randomised controlled trial. Lancet Oncol 2014;15:143-55. [PubMed]
- Lara PN, Douillard JY, Nakagawa K, et al. Randomized phase III placebo-controlled trial of carboplatin and paclitaxel with or without the vascular disrupting agent vadimezan (ASA404) in advanced non-small-cell lung cancer. J Clin Oncol 2011;29:2965-71. [PubMed]