Angiogenesis in cancer: Anti-VEGF escape mechanisms
Introduction
Angiogenesis, the growth of new blood vessels, is important in the pathogenesis of malignant, infectious, fibro-proliferative, and inflammatory diseases. Angiogenesis in tumors was first described 100 years ago (1) and 1971 J. Folkman proposed that tumor growth and metastasis are angiogenesis-dependent and blocking angiogenesis could be a strategy to block progress disease (2). Meanwhile, it is widely accepted that pre-cancerous tissues acquire angiogenic capacities on the way to become cancerous. Thereby, the net balance between pro-angiogenic and anti-angiogenic molecules is tipped to favor angiogenesis (the 'angiogenic switch'). In fact, tumors produce pro-angiogenic growth factors. Among them the vascular endothelial growth factor, VEGF, is thought to be the most important as VEGF acts pro-angiogenic by augmenting all steps of angiogenesis: vascular permeability, which allows exudation of plasma proteins that lay down a provisional scaffold for detached endothelial cells, endothelial cell proliferation, endothelial cell migration or invasion into the surrounding tissue and finally capillary-like tube formation. Thus, VEGF-A is the major regulator of physiological and pathological angiogenesis. While VEGF under physiologic conditions is a hypoxia-regulated gene via binding of HIF to its promoter (3), VEGF is highly over-expressed in a variety of tumors (4). VEGF-A specifically binds to endothelial cells either via VEGFR-1 (flt-1) or VEGFR-2 (flk-1), but was recently described also to bind to neuropilin-1, or-2, whereby the latter ones are thought to mediate VEGF signaling leading to enhanced endothelial cell migration (5). Thus, various inhibitors interfering with VEGF/VEGFR have received FDA approval and are currently in clinical use; beside the monoclonal antibody that blocks human VEGF bevacizumab (Avastin; Genentech) (6), also small-molecule inhibitors of the VEGFR-2 tyrosine kinase such as sorafenib, sunitinib, and pazopanib are approved for certain tumor types (7). The introduction of anti-angiogenic therapies have been demonstrated to prolong progression-free survival (PFS) and/or overall survival (OS), and improves objective tumor responses, in patients with advanced malignancies such as in renal cell cancer, non-small-cell lung cancer (NSCLC), ovary and colorectal cancer (8-10). However, not all patients benefit from such anti-angiogenic therapy, and those tumors that initially respond to treatment will finally become resistant to VEGF-based therapies and relapse (11). Therefore, the development of more durable anti-angiogenic therapies requires an improved understanding of the cellular and molecular mechanisms that mediate resistance to anti-angiogenic agents.
There is increasing evidence that blockade of the VEGF/VEGFR-2 signaling pathway leads to an upregulation of protein expression of secondary molecules in order to sustain the neo-vascularization response (12), whereby in particular components of the EGFR and FGFR pathways were shown to be upregulated in the tumor stroma (13). Further suggested mechanisms include genetic alterations of p53, which uncouples tumor dependency on a vascular blood supply (14). Other studies have shown that tumor cells may alter their pattern of growth when challenged with anti-angiogenic therapy by meeting their metabolic requirements via residing in close proximity to preexisting blood vessels (15). The key systems beyond VEGF/VEGFR-2 responsible for angiogenic endothelial cell behavior are discussed here, which will give an overview about most prominent mechanisms of escaping VEGF-targeting approaches in tumor angiogenesis.
Growth factors
FGF-2
The family of fibroblast growth factors (FGF) affects neurogenesis, organ development, branching morphogenesis, angiogenesis, but is also involved in the pathogenesis of cancer. Especially for tumor growth and angiogenesis, FGF-2 by binding to its receptor FGFR-1 (Figure 1), is thought to be the major family member as gene silencing by antisense targeting of FGF2 and FGFR-1 in models of human melanoma caused a dramatic reduction in the size of tumors (16). Other studies have given first evidence that FGF-1 and FGF-2 are able to affect angiogenesis in a VEGF independent manner, as both molecules were upregulated in tumors treated with anti-VEGFR-antibodies in a RIP-Tag model. Thus, together with an FGF-trap, anti-VEGF treatment was able to decrease tumor growth and lessen tumor angiogenesis (17,18). This was further supported by findings derived from clinical trials with FGF-2 affecting agents such as thalidomide or suramin, which demonstrated a therapeutic benefit in advanced prostate as well as renal cell cancer (16).
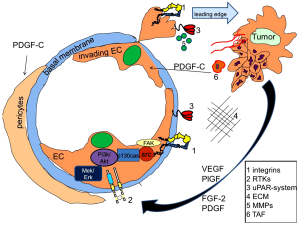
PDGF
The platelet derived growth factor (PDGF) is frequently upregulated in tumors and has recently been shown to synergistically with FGF-2 promote tumor angiogenesis and pulmonary metastasis. While overexpression of PDGF-BB alone in tumor cells resulted in dissociation of VSMCs from tumor vessels and decreased recruitment of pericytes, FGF-2 triggered PDGFR-α and-β expression at the transcriptional level in ECs, which acquire hyper-responsiveness to PDGF-BB (19).
However, increased expression of PDGF-BB has been demonstrated to reduce colorectal as well as pancreatic cancer growth via increasing the pericyte content of the tumor microenvironment, thus single-agent therapy targeting PDGF receptor might fail to show any benefit in cancer (20). This might explain the fact that tyrosine kinase inhibitors, which are characterized to be of broader substrate specificity, such as pazopanib (targeting PDGFR-a/b, but also the VEGFR-family and c-kit), is effective in some cancers.
Delta/Jagged family
Delta l-like ligand 4 (Dll4) is a member of the Delta/Jagged family of transmembrane ligands binding to Notch receptor. The delta-Notch pathway may not only have an effect on the regulation of artery-vein differentiation but is also able to stimulate blood vessel formation. Furthermore, it mediates cell-cell communication and controls cell determination, thus it is a major player in regulating proper vascular development (21). Recent studies have demonstrated that Dll4 knock-out mice have abnormal vessel sprouting, while blockade of Dll4-Notch signaling in tumors results in excessive, non-productive angiogenesis with resultant inhibitory effects on tumor growth, even in some tumors that are resistant to anti-VEGF therapies (22). Dll4 inhibitors are entering clinical trials and might provide novel therapeutic tools in VEGF/VEGFR inhibitor resistant tumors.
Angiopoietin system
The signaling system initiated by angiopoietin in endothelial cells, which express its receptor Tie2, advocates the PI3K-pathway resembling the survival signal. Ang2's role in angiogenesis generally is considered as an antagonist for Ang1, thereby inhibiting Ang1-promoted Tie2 signaling, which is critical for blood vessel maturation and stabilization. Ang2's role in tumor angiogenesis, however, is controversial. While upregulation of Ang2 correlates with malignancy of various types of human cancers, because Ang2 overexpression augmented tumor angiogenesis and growth in mice, other studies have reported that specific induction of Ang2 in gliomas, mammary carcinomas, and lung carcinomas inhibited tumor growth and metastasis (23). Pre-clinical trials have revealed controversial results: While treatment of mice bearing xenografts of human A431 epidermoid tumor and Colo205 colon cancer with neutralizing anti-Ang2 antibodies led to growth reduction and regression of large established tumors by suppressing cell proliferation and inducing apoptosis of tumor-derived ECs, overexpression of Ang2 attenuated VEGF expression, impaired pericyte coverage of the tumor vasculature, inhibited EC proliferation, and induced EC apoptosis, resulting in a massive regression of tumor vasculature and tumor growth (24). Thus, a better understanding of Ang2 functions in tumor angiogenesis and progression is mandatory for future advances in effective anti-angiogenic approaches.
Subcellular systems
The proteolytic system
Activated endothelial cells emigrate from their residential site, a process which requires polarization of endothelial cells and transmigration through the basal membrane into surrounding matrix. Especially for the latter step, proteolytic degradation of matrix proteins is essential, whereby the plasminogen system, the matrix-metalloproteinase (MMP) system, as well as the heparanase and chymase families are thought to be important. Thus, inhibition of MMP-2 binding to integrin αvβ3 by the non-catalytic MMP-2 fragment PEX was shown to inhibit tumor angiogenesis (25).
A large body of experimental evidence from in vitro and in vivo data as well as from the clinics indicates an important role of the urokinase (uPA)/plasminogen system in angiogenesis and cancer. E.g., it was demonstrated that inhibition of functional activity of the receptor of urokinase-type plasminogen activator, uPAR, significantly decreased the invasive potential of endothelial cells (26-28), and the absence of the host plasminogen activator inhibitor-1 (PAI-1) prevented cancer invasion and metastasis (29). Consistently, PAI-1 correlates with poor prognosis in cancer patients, probably by preventing excessive proteolysis or other not yet defined mechanisms (30). In this context we and others could recently reveal that this system is essential for degrading surrounding matrix proteins at the leading edge, but also coordinates the redistribution of proteolytic as well as adhesive proteins to newly formed focal adhesions. Especially migrating cells continuously form focal contacts at the leading edge by new integrin-matrix interactions. The cell matrix contacts persist until they reach the trailing end, where integrins have to release their ligands in order to allow cell locomotion (31,32). Thereby, integrins become internalized and recycle back to the leading edge during cell migration (33). Although it is still unclear how integrins are internalized, the involvement of clathrin-coated vesicles has been suggested (34). It was suspected that the NPXY motif in the cytoplasmic tail of beta subunits might be responsible for integrin signaling and internalization (35,36); however, the internalization process of integrins was not affected by point mutations of NPXY (37). We and others observed that uPAR, which interacts with the fibronectin receptors α3β1 and α5β1 integrins (38) or with integrin αvβ5 or αvβ3 (39) tracked integrins into the endocytotic compartment via clathrin coated pits (40). In detail, we revealed a mechanism by which in endothelial cells VEGF-A and VEGF-E rapidly induced pro-urokinase (pro-uPA) activation on the surface of endothelial cells (41). This involved a phosphatidylinositol 3-kinase (PI3-kinase)-dependent change in integrin affinity, leading to activation of proMMP-2 and pro-uPA, when pro-uPA is bound to its surface receptor uPAR. As a consequence, this VEGF-induced pro-uPA activation on endothelial cells was responsible for VEGF-dependent local fibrinolytic activity and might be one of the initial steps in matrix degradation during the angiogenic process. Furthermore, active uPA forms complexes with its inhibitor PAI-1, which-when bound to uPAR-can be internalized and degraded. Internalization is performed via a member of the LDL receptor family (28,42), involving clathrin-coated vesicles formation. Thereafter, uPAR itself can recycle back from the endocytotic compartment to the cell surface (43). In VEGF-stimulated endothelial cells we were able to show that pro-uPA activation not only led to extracellular matrix degradation, but-as a consequence-led to a coordinated internalization of uPAR by an LDL-receptor like molecule. Data obtained from PAI-1-/-cells indicated that uPAR internalization in response to VEGF is PAI-1-dependent, which is consistent with the prerequisite of an uPAR/uPA/PAI-1 complex formation. As a consequence we were able to show that uPAR recycles back to the cell surface via a coordinated process leading to focusing of uPAR to newly formed focal adhesions at the leading edge (28). Internalization and target oriented recycling of uPAR to the leading edge plays a role in growth factor-induced endothelial cell migration, because cleavage of the GPI-anchor of uPAR, via which uPAR is fixed to the cell surface, diminished the migratory response significantly. This mechanism is not limited to VEGF165, but is induced by a variety of different growth factors; however, it might also get bypassed such as by placental-like growth factor (PlGF), which did not induce pro-uPA activation on the endothelial cell surface and consequently led not to uPAR internalization and recycling to the leading edge (28).
That this system is not only required for endothelial cell migration, but also endothelial cell survival was shown in uPA (-/-) endothelial cells. Only when uPA was expressed, growth factor activated endothelial cells were protected against apoptosis, which was provoked via transcriptional up-regulation and partially by mRNA stabilization of inhibitor of apoptosis proteins, most prominently the X-linked inhibitor of apoptosis protein (XIAP). Thereby, the antiapoptotic activity of uPA was dependent on its protease activity, the presence of uPAR and LRP, but independent of the PI3kinase pathway, whereas VEGF-induced anti-apoptosis was PI3kinase dependent (44).
The uPAR-system itself is tightly regulated on a transcriptional level. Thus, Michael S. Pepper described first that pro-angiogenic growth factors led to a transcriptional upregulation of uPAR (45). In this context, we recently observed that uPAR expression on the surface of endothelial cells is only up-regulated when cells were in a sub-confluent state, but was down-regulated when cells reached confluence. Thereby, the receptor-like protein tyrosine phosphatase DEP-1 (density enhanced phosphatase-1/CD148), which is abundantly expressed in confluent endothelial cells, inhibited activation of MEK/ERK1/2 pathway, which is the major signaling pathway for transcriptional upregulation of uPAR expression. Consistently, overexpression of active ERK1 rescued the DEP-1 effect on uPAR. Hence, DEP-1 plays a biologic role in angiogenic endothelial cell behavior, such as in endothelial cell migration, proliferation, and capillary-like tube formation (46).
Integrin adhesion molecules and their role in angiogenesis
Integrin adhesion receptors are heterodimeric molecules, which mediate cell-matrix interaction, thereby affecting cellular behaviors such as cell migration, proliferation, differentiation and cell survival. Thus, the biological role of integrin over-expression and activation in human cancer was elucidated: Via induction of major signal transduction pathways, which lead to cancer cell invasion, metastasis as well as enhanced angiogenesis, integrins play a central role in all phases of tumorigenesis (47). Thus, a coordinated regulation of integrin expression and activation mediates the functional role adapted to specific conditions. During embryonic development the accurately patterned network of blood vessels in addition depends on the superior regulation of integrin activation, which is reduced by diverse chemo-attractants, for example growth factors that regulate angiogenesis. A disruption of this precision adjustment is expected to occur in cancer (48).
Blood vessel formation is decisively reliant on extracellular signaling as well as their connection with the extracellular matrix (ECM) proteins, such as fibronectin, fibrinogen, vitronectin, laminin as well as collagens. Consistently, endothelial cell migration as well as cell survival is regulated by integrins (49,50). The attachment to the ECM is adjusted by integrin adhesion receptors; integrins operate as bidirectional transducer molecules by matching signals from both, the outside to the inside (outside-in) of the cell, or from the inside to the outside (inside-out). Both signaling directions are tightly regulated in focal adhesion to support cell adhesion, spreading and motility (51).
Integrin interaction partners
As heteromeric adhesion receptors integrins consisting of an α and a β chain, and are expressed in most of human cell types. 24 different αβ combinations can be formed by 18 α subunits and eight identified β subunits and are paired to function as distinct receptors (52). These integrin subunits have a large extracellular domain, a single transmembrane domain and a short cytoplasmic domain (53). The α and β subunits of integrins are associated via non-covalent bonds. Integrins are also able to affect cell behavior by interfering with receptor tyrosine kinases (RTKs) such as fibroblast growth factor receptor (FGFR) (54). Many ligands of integrins are recognized via their Arg-Gly-Asp (RGD) sequence motif such as for αvβ3 and αvβ5, whereas α4β1 recognizes Glu-Ilu-Asp-Val (EILDV). They may also interact with cell surface receptors, like Vascular Cell Adhesion Molecule-1 (VCAM-1) (55). In the cytoplasmic tail of β-integrins interaction with the FERM domain of talin led to high-affinity conformation of integrins, which enables ligand binding and linking the ECM to the cytoskeleton (56).
Adhesion-dependent signal transduction via integrins
Integrins have to interact with several kinases as well as related adaptor proteins since they have no intrinsic kinase activity. Subsequently, the β tail is adjuvant as the major site in the arrangement of focal adhesions. By the recruitment of signaling components such as pp125 Focal Adhesion Kinase (FAK), integrins can activate "outside-in" signaling thereby creating and communicating signals that can induce cell migration or cell proliferation (57). Hereby the non receptor protein kinase FAK is phosporylated at tyrosine 397, leading to activation of src family kinases (SFK) which control not only the activity of Rho GTPases but also downstream kinases such as AKT, thereby affecting endothelial cell proliferation, migration and survival (58,59).
Two cytokine-related pathways affect integrin-dependent angiogenesis. One is either induced by fibroblast growth factor (FGF) or tumor necrosis factor α (TNF-α) and needs the function of integrin αvβ3, whereas the other one requires αvβ5 and is initiated by vascular endothelial growth factor (VEGF) or transforming growth factor α (TGF-α) (60). The distinction is given by the fact that β3 and β5 can affect the activation of Ras/Raf/MEK/Erk pathways in the vasculature in different manners. Thus, the αvβ5 related pathway downstream from VEGF activates focal adhesion kinases (FAK) and Src kinases, however αvβ3 integrins is able to activate p21 kinases (61). In addition αvβ3 induces activation of Raf at tyrosine 340/341 and Mek dependent protection from extrinsic mediated apoptosis, which is initiated by pro-apoptotic ligand binding to receptors, including TNF-α and Fas (62). On the other hand, αvβ5 affected pathways activate Raf on serine 338/ 339, which leads to Raf-1 mitochondrial translocation and shelter from the intrinsic pathway of apoptosis (62).
Interfering with angiogenesis via integrin family members
A range of integrins control endothelial cell functions such as cell proliferation, survival, and migration and invasion during angiogenesis, whereby various integrins have been functionally related, such as α1β1, α2β1, α4β1, α5β1, α6β1, α6β4, α9β1, αvβ3 and αvβ5 (63). A major regulator for developmental angiogenesis and blood vessel formation is resembled by fibronectin (64). Its receptor, the β1 integrin is an essential mediator in angiogenesis, as the genetically knock out of β1 integrins led to embryonic lethality or blocked blood vessel development in teratomas (65,66). Another fibronectin binding partner, α5 is also crucial, because its loss led to developmental deviations and vascular deformation (67). α5β1 integrins have been described to be overexpressed in endothelial cells of malignant tumors, suggesting their functional role in tumor angiogenesis (68,69), which was supported by the fact that targeting α5β1 was able to block tumor-angiogenesis (68). Even for normal vasculature development α5 is thought to be essential as its genetical knock out was embryonic lethal at day E10-E11 with abnormalities in the vascular development (70). In contrast, patients suffering from the Glanzmann thrombasthenia, which is characterized by null mutation in integrin β3 subunit, do not have any aberrations in angiogenesis and vascular development. However, knockout studies of αv, including αvβ3, αvβ5 and all other αv combinations, revealed a deficiency in vascular integrity (71). It was hypothesized that αvβ8 is the key αv integrin as vasculature abnormalities aroused only in β8 integrin knockouts, but not in β5 or β3 knockout mice. β3 knockout as well as β3/ β5 double knockout mice revealed improved tumor angiogenesis (72), probably via upregulation of receptor tyrosine kinases.
Antagonists for α5β1 are already under investigation for cancer therapies (73). αvβ3 integrins are mainly expressed by proliferating and activated vascular endothelial cells and is consequently a main molecule for the arrangement of vasculature, supporting migration and survival of endothelial cells (74). The activation can either be induced by cytokines or within the influence of a malignant tumor whereas blocking αvβ3 integrins restrains tumor angiogenesis in addition to blood vessel formation of in vivo models (60,75,76). Subsequently, αvβ3 might represent a potential target in anti-angiogenic therapy. Integrins α1β1 and α2β1, which bind to laminin and collagen, seemed to be indispensable for angiogenesis (77), because functional blocking α1β1 and α2β1 integrins inhibited angiogenesis in vitro, as well as it reduced tumor growth and angiogenesis in vivo (78-80). Current concepts of anti-integrin therapeutics are focused on targeting directly or indirectly the receptor binding sites, although new functional insights propose alternative approaches such as targeting most upstream integrin signaling (81).
Reduced toxicity has been recognized for various antagonists of αvβ3 integrins as they have been seen to be only expressed on activated or remodeling tissues such as tumors. Among these antagonists, abergrin (vitaxin, Medi-522) is a humanized antibody against αvβ3 integrins, and was the first anti-integrin approach introduced into clinical trials for malignant tumors (82). This antibody blocks binding to vitronectin and fibrinogen, prevents cell adhesion, migration, proliferation and integrin mediated cell signaling in a dose dependent manner (83). Tumors of patients with a high expression of αvβ3 had a poorer prognosis than patients with lower expression levels. Consistently, high expression of αvβ3 in tumors was accompanied with an aggressive phenotype and augmented metastatic potential and invasive traits. Furthermore, association of αvβ3 with Src promoted attachment-independent autonomous cell growth while αvβ3 integrin mediated FAK signaling fostered the survival of cancer stem cells (84).
α5β1 integrin antagonists are under development for treatment of malignant tumors. Volociximab is a chimeric human-mouse monoclonal antibody binding to human α5β1 integrins (85). Volociximab is able to induce cell death and prevents capillary tube formation in vitro, thereby inhibiting signaling cascades. Because there is no effect on cell proliferation when combined with a VEGF blocking antibody, it was suggested that volociximab induced blocking of α5β1 is downstream of the VEGF induced pathway. In vivo volociximab showed also anti-tumor as well as anti-angiogenic capacities (86). In a Phase II study, volociximab showed promising potential efficacy in prolonging disease progression and overall survival. In clear cell renal cell carcinoma, volociximab delayed disease progression up to 22 months. Additional Phase II and III trials, where volociximab is used as single agent as well as in combination regiments, are currently ongoing to test its effect in advanced melanoma, non-small cell lung cancer and peritoneal cancer (85).
Because integrin αvβ3 and αvβ5 both regulate angiogenesis a human monoclonal antibody against both integrins was developed, called CNTO 95. It showed preclinical activity by reducing angiogenesis and tumor growth in human melanoma xenografts in nude mice and rats (87). CNTO 95 was recently tested in a clinical Phase I/II trial for the treatment of melanoma (88). αv integrin targeting is able to inhibit both, tumor cell invasion and metastasis as well as tumor angiogenesis (89,90).
During adulthood the majority of blood vessels remain quiescent, and angiogenesis occurs only during embryonic development. Whereas in many diseases angiogenesis processes are activated, resembling tumor growth and metastasis as well as ocular and inflammatory diseases. Huge advances have been made over the last two decades in finding and engineering integrin targeting therapeutics, as integrin expression or functions are altered in many disorders. Progress in the structural characterization of the integrin-ligand complex led to the development and design of powerful and selective blocking agents for a variety of integrins, thereby targeting angiogenesis, metastasis and tumor growth.
The tumor microenvironment
Tumor cells have long been thought to be the main source of angiogenic factors (4,91). However, growing evidence now indicate that the tumor microenvironment, which consist of fibroblasts, pericytes, mesenchymal-stem cells and inflammatory-immune cells also contributes to angiogenesis via secretion of angiogenic molecules as well as via modulation of the immune system (92). In this context Bv8, also known as prokineticin 2, has recently been shown to be a mediator of myeloid cell-dependent tumor angiogenesis. In detail, myeloid cells defined by the expression of CD11b+ and Gr1+ have been associated with refractoriness to anti-VEGF therapy (93,94). Thus, the bone marrow derived CD11b+ and Gr1+ cells are frequently increased in the tumors and the peripheral blood of tumor bearing animals as well as in humans (92), thereby promoting angiogenesis (95). In several xenograft models as well as transgenic cancer models, it was demonstrated that CD11b+ and Gr1+ cells produce Bv8, which led to an enhanced peripheral mobilization of myeloid cells and local stimulation of angiogenesis (96,97). Thereby G-CSF, which is a well known inducer of granulocytic-progenitor cell differentiation and mobilization, was identified to be a strong inducer of Bv8 expression. Although discussed controversial, administration of G-CSF has been correlated to enhanced tumor angiogenesis and growth (98,99). Consistently, whenever G-CSF or Bv8 were targeted by administration of blocking inhibiting antibodies, reduced tumor angiogenesis and growth was observed. Notably, the effect was additive to anti-VEGF mAb in refractory tumors and treatment with G-CSF resulted in increased CD11b+ and Gr1+ cell numbers and reduced responsiveness to anti-VEGF therapy (94).
Fibroblasts in the tumor-microenvironment
Tumor associated fibroblasts (TAFs) have been shown to promote angiogenesis mainly via stromal cell-derived factor 1 (SDF-1) expression. Thereby, SDF-1 recruits endothelial progenitor cells (EPCs) into carcinomas and contributes to tumor promotion (100). That TAFs derived from anti-VEGF treatment resistant tumors can induce angiogenesis independent of VEGF was shown by Crawford, Y., et al. (101), who demonstrated that platelet-derived growth factor C (PDGF-C) was upregulated in TAFs from resistant tumors, and PDGF-C-neutralizing antibodies blocked the angiogenesis induced by such TAFs in vivo. This demonstrated that PDGF-C does not require VEGF activity to fulfill its angiogenic function. A combination therapy using both anti-PDGF-C and anti-VEGF antibodies was more effective in inhibiting tumor angiogenesis than using anti-VEGF treatment alone. Notably, PDGF-C has direct effects on all three types of vascular cells, endothelial cells, pericytes and smooth muscle cells, by promoting their proliferation, survival and migration, but might also affect fibroblasts themselves as well as inflammatory cells (102). In this context, it is notably that tumors, which highly express PDGF-C, such as pancreatic cancer, do not respond towards VEGF targeting treatment regiments (4,103,104), thus, it is tempting to speculate whether targeting PDGF-C would lead to a therapeutic response. However, neither sorafenib nor imatinib has so far been demonstrated to be effective in pancreatic cancer (105,106). This might be explained by the observation that interfering with PDGF-C induced pericyte recruitment leads to pro-motion of metastasis (107).
Clinical aspects
Inhibitors against the VEGF / VEGFR-system are already in clinical use. As only a certain number of patients suffering from cancer respond to such therapy, efforts were attempted to targeted angiogenesis by novel strategies. Thus, a variety of compounds, which revealed promising pre-clinical results, were tested in clinical phase I to III studies, whereby the most important drugs, if not mentioned above, are discussed here:
Aflibercept
Aflibercept is a fusion protein with sequence homologies with native VEGFR-1 and VEGFR-2 and was specifically designed to bind several variants of VEGF (108). The soluble VEGF receptor fragment was tested in Phase II trials described minor single agent activity in platinum-and erlotinib-resistant adenocarcinoma of the lung and metastatic urothelial cancer, respectively (109,110). However, a recent Phase III trial (VELOUR trial) evaluated effects of aflibercept with irinotecan/5-FU as second line chemotherapy for metastatic colorectal cancer and experienced extended progression free survival and overall survival suggesting it for clinical approval (111). A subgroup analysis revealed that aflibercept was also effective in patients pretreated with the anti-VEGF antibody bevacizumab. This interesting finding might be explained by the fact that aflibercept does not only bind to VEGF165, but also to PlGF, and other splicing variants of VEGF-A.
Vandetanib
Vandetanib, a once-daily oral inhibitor of RET kinase (an abbreviation for "rearranged during transfection"), EGFR as well as VEGFR signaling, has previously shown antitumor activity in a Phase II study of patients with advanced hereditary medullary thyroid carcinoma (MTC) (112). Furthermore, in a double-blind Phase III trial 231 patients suffering from advanced MTC who received vandetanib showed significant progression free survival (PFS) rates when compared with the placebo control group. The role of vandetanib was also investigated in the second-line treatment for advanced non-small-cell-lung cancer patients: A meta-analysis by Wei-Xiang Qi et al. (113) of randomized controlled Phase III trials, showed significant improvement of PFS and overall response rate (ORR) but no enhancement of overall survival. The superior response towards vandetanib may underlie the importance of the combined blockade of RET kinase and growth factor receptors, all involved in MAPK and PI3K/Akt signaling, which is not precisely disclosed yet, but gives hope for beneficial effects in other cancers. Furthermore, in patients suffering from advanced breast cancer, vandetanib in combination with docetaxel was already tested in a Phase II trial showing promising results (114).
Cilengitide
Another αvβ3 antagonist is cilengitide (EMD 121974), a cyclic RGD-peptide inhibitor of αvβ3 and αvβ5 integrins. Significantly enhanced progression free survival was observed in a Phase I/IIa clinical trial for glioblastomas (115). Cilengitide is now in clinical Phase III studies for glioblastomas and in Phase II studies for several other tumor identities including breast cancer, squamous cell cancer, non-small cell lung cancer and melanoma (116-119). Cilengitide is able to detach cells that have attached to the matrix at concentrations nearly equal to concentrations that inhibit cell attachment. Cilengitide decreases cell growth, cell proliferation and cell survival in vitro. Moreover, it blocks FAK-Src-Akt and Erk signaling pathways (120,121). Cilengetide is therefore able to influence embryonic as well as established tumor vasculature. As the communication of tumor cell with endothelial cells is still unclear, additional experimental advances have to be done to get more insights into this complex interplay (81). Other integrin inhibitors are discussed above.
Pazopanib
Since Phase I and II trials have shown partial responses and well tolerability, a randomized, double-blind, placebo-controlled Phase III study was assessed to investigate efficacy and safety of pazopanib monotherapy in patients with advanced renal cell carcinoma (RCC) (122,123). Results indicated significant prolonged PFS with pazopanib compared with placebo in the overall study population (median, PFS 9.2 vs. 4.2 months; hazard ratio [HR], 0.46; 95% CI, 0.34 to 0.62; P<0.0001)(124). Therefore in 2009, the Food and Drug Administration (FDA) granted approval to pazopanib for the treatment of patients with advanced renal cell carcinoma.
Because of its potent and selective multi-targeted receptor tyrosine kinase inhibitor characteristics for VEGFR-1, VEGFR-2, VEGFR-3, PDGFR-a/β, and c-kit (125), pazopanib might also effect the outcome of other tumors and was consequently tested in ovarian-and breast cancer as well as advanced soft tissue sarcoma in several trials suggesting further investigations and rising hope for new treatment indications (126-128).
Thalidomid and thalidomide-derived immunomodulatory drugs (IMiDs)
Initially used because of its sedative effects, thalidomid has long been known for its adverse properties in development such as phocomelia. Intense investigations, however, have revealed many immunomodulatory functions (129).
Additionally, in hematologic malignancies, thalidomid is an upcoming drug showing inhibition of endothelial cell activation via VEGF, FGF-2 and others (130). Especially in myeloma patients, thalidomide was shown to suppress VEGF secretion in bone marrow endothelial cells (129). In a Phase II clinical trial, 169 refractory myeloma patients were treated with thalidomide monotherapy (131). 30% of patients showed a partial response, reflected by 50% paraprotein reduction, and 14% achieved a nearly complete remission with two-year event-free and overall survival rates around 48%.
These findings resulted in many clinical trials of thalidomide, finally leading to FDA approval in 2006 for the treatment of newly diagnosed multiple myeloma in combination with dexamethasone. In a respective Phase III trial, the response rate in patients receiving thalidomide plus dexamethasone was 63% compared to 41% with dexamethasone alone (P=0.0017) (132).
Besides suppressing VEGF secretion, thalidomide is also known to inhibit NFkB signaling, an important pathway in prostate cancer. A randomized Phase II trial found remarkable PSA decline (53% vs. 37%)-and an overall median survival rates (68.2% vs. 42.9%) in 74 patients with metastatic androgen-independent prostate cancer when treatment with docetaxel plus thalidomide vs. docetaxel alone (133).
Angiostatin/Endostatin
Potent endogenous angiogenesis inhibitors like angiostatin, a plasmin fragment, or endostatin, derived from type XVIII collagen, are currently under investigations for clinical usage. Recent promising results in mice have shown that angiostatin is not only reducing angiogenesis in combination with bevacizumab but also decreases a possible bevacizumab-induced invasion of U87 glioma cells (134). Angiostatin shows multiple ways of inhibiting angiogenesis like diminishing of ERK activation by basic fibroblast growth factor and vascular endothelial growth factor but there is still uncertainty on its mechanism of action (135).
In a randomized, open-label, Phase II study, two different doses (15 vs. 60 mg) of rh-angiostatin were subcutaneously administered in combination with paclitaxel and carboplatin to patients with advanced NSCLC (136). Partial response showed favorable results for higher dosage of angiostatin (54.5% when administered 60 mg and 25% with 15 mg). 1-year survival data of 45.8% are higher than in treatments with caboplatin and paclitaxel alone, which is additionally suggesting further studies for angiostatin.
Endostatin has long been known for its inhibitory effects on normal-and lymphangiogenesis (137,138). Inhibiting the expression of VEGF-A, VEGF-C and VEGF-D besides others, opens a wide array of therapeutic potential (139). A randomized, double-blind Phase III trial of rh-endostatin in the treatment of advanced non-small cell lung cancer patients showed significantly improved results that the drug was consequently approved by the State Food and Drug Administration of China (SFDA) in 2005 (140). The 486 patients in this multicenter trial were treated either with vinorelbine and cisplatin plus endostatin or vinorelbine and cisplatin plus placebo. Patients included in the endostatin group had increased overall response rates (35.4% vs. 19.5%; P=0.0003) and also showed enhanced median time to progression (TTP) of around 3 months.
The promising results rose hope also to be beneficial in other tumors affected by lymphangiogenesis and metastasis. However, results could not be confirmed in a Phase II study of advanced neuroendocrine tumor patients as treatment with rh-endostatin did not lead to a significant tumor regression (141). Recently, endostatin was shown to suppress growth and metastasis in a mouse xenograft model of colon cancer (139) opening the door for further studies.
Conclusions
Accumulated studies on VEGF-independent angiogenesis and its therapeutic implications using models of cell culture, tumor xenografts, as well as clinical investigations, have depicted several candidates as potential targets to interfere with tumor growth and metastasis formation. As not every cancer patient benefits from an anti-VEGF treatment or finally becomes resistant to such therapeutic approaches, there is an urgent need for a better understanding of VEGF-independent mechanisms leading to angiogenesis in cancer.
Acknowledgements
The study was supported by the Initiative Krebsforschung to G.W.P, as well as the Austrian Science Foundation projects (FWF P21301 and FWF P23199) of G.W.P Contribution: G.W.P., M.P., M.U. and C.C.Z. wrote the manuscript.
Disclosure: Gerald W. Prager, Marina Poettler and Matthias Unseld declare no conflicts of interest. Christoph C. Zielinski declores: Consultancy, Roche, Merck-Serono; payment for lectures, Roche.
References
- Goldmann E. The Growth of Malignant Disease in Man and the Lower Animals, with special reference to the Vascular System. Proc R Soc Med 1908;1:1-13. [PubMed]
- Folkman J. Tumor angiogenesis: therapeutic implications. N Engl J Med 1971;285:1182-6. [PubMed]
- Wang GL, Semenza GL. Purification and characterization of hypoxia-inducible factor 1. J Biol Chem 1995;270:1230-7. [PubMed]
- Ellis LM, Hicklin DJ. VEGF-targeted therapy: mechanisms of anti-tumour activity. Nat Rev Cancer 2008;8:579-91. [PubMed]
- Schwarz Q, Ruhrberg C. Neuropilin, you gotta let me know: should I stay or should I go? Cell Adh Migr 2010;4:61-6. [PubMed]
- Dvorak HF. Vascular permeability factor/vascular endothelial growth factor: a critical cytokine in tumor angiogenesis and a potential target for diagnosis and therapy. J Clin Oncol 2002;20:4368-80. [PubMed]
- Chung AS, Lee J, Ferrara N. Targeting the tumour vasculature: insights from physiological angiogenesis. Nat Rev Cancer 2010;10:505-14. [PubMed]
- Sandler A, Gray R, Perry MC, et al. Paclitaxel-carboplatin alone or with bevacizumab for non-small-cell lung cancer. N Engl J Med 2006;355:2542-50. [PubMed]
- Hurwitz H, Fehrenbacher L, Novotny W, et al. Bevacizumab plus irinotecan, fluorouracil, and leucovorin for metastatic colorectal cancer. N Engl J Med 2004;350:2335-42. [PubMed]
- Rini BI, Halabi S, Rosenberg JE, et al. Bevacizumab plus interferon alfa compared with interferon alfa monotherapy in patients with metastatic renal cell carcinoma: CALGB 90206. J Clin Oncol 2008;26:5422-8. [PubMed]
- Fischer C, Mazzone M, Jonckx B, et al. FLT1 and its ligands VEGFB and PlGF: drug targets for anti-angiogenic therapy? Nat Rev Cancer 2008;8:942-56. [PubMed]
- Bergers G, Hanahan D. Modes of resistance to anti-angiogenic therapy. Nat Rev Cancer 2008;8:592-603. [PubMed]
- Cascone T, Herynk MH, Xu L, et al. Upregulated stromal EGFR and vascular remodeling in mouse xenograft models of angiogenesis inhibitor-resistant human lung adenocarcinoma. J Clin Invest 2011;121:1313-28. [PubMed]
- Yu JL, Rak JW, Coomber BL, et al. Effect of p53 status on tumor response to antiangiogenic therapy. Science 2002;295:1526-8. [PubMed]
- Leenders WP, Küsters B, de Waal RM. Vessel co-option: how tumors obtain blood supply in the absence of sprouting angiogenesis. Endothelium 2002;9:83-7. [PubMed]
- Beenken A, Mohammadi M. The FGF family: biology, pathophysiology and therapy. Nat Rev Drug Discov 2009;8:235-53. [PubMed]
- Casanovas O, Hicklin DJ, Bergers G, et al. Drug resistance by evasion of antiangiogenic targeting of VEGF signaling in late-stage pancreatic islet tumors. Cancer Cell 2005;8:299-309. [PubMed]
- Cao Y, Cao R, Hedlund EM. R Regulation of tumor angiogenesis and metastasis by FGF and PDGF signaling pathways. J Mol Med (Berl) 2008;86:785-9. [PubMed]
- Nissen LJ, Cao R, Hedlund EM, et al. Angiogenic factors FGF2 and PDGF-BB synergistically promote murine tumor neovascularization and metastasis. J Clin Invest 2007;117:2766-77. [PubMed]
- McCarty MF, Somcio RJ, Stoeltzing O, et al. Overexpression of PDGF-BB decreases colorectal and pancreatic cancer growth by increasing tumor pericyte content. J Clin Invest 2007;117:2114-22. [PubMed]
- Dufraine J, Funahashi Y, Kitajewski J. Notch signaling regulates tumor angiogenesis by diverse mechanisms. Oncogene 2008;27:5132-7. [PubMed]
- Kuhnert F, Kirshner JR, Thurston G. Dll4-Notch signaling as a therapeutic target in tumor angiogenesis. Vasc Cell 2011;3:20. [PubMed]
- Hu B, Cheng SY. Angiopoietin-2: development of inhibitors for cancer therapy. Curr Oncol Rep 2009;11:111-6. [PubMed]
- Cao Y, Sonveaux P, Liu S, et al. Systemic overexpression of angiopoietin-2 promotes tumor microvessel regression and inhibits angiogenesis and tumor growth. Cancer Res 2007;67:3835-44. [PubMed]
- Brooks PC, Silletti S, von Schalscha TL, et al. Disruption of angiogenesis by PEX, a noncatalytic metalloproteinase fragment with integrin binding activity. Cell 1998;92:391-400. [PubMed]
- Min HY, Doyle LV, Vitt CR, et al. Urokinase receptor antagonists inhibit angiogenesis and primary tumor growth in syngeneic mice. Cancer Res 1996;56:2428-33. [PubMed]
- Ossowski L. Effect of antisense inhibition of Urokinase receptor on malignancy. Curr Top Microbiol Immunol 1996;213:101-12. [PubMed]
- Prager GW, Breuss JM, Steurer S, et al. Vascular endothelial growth factor receptor-2-induced initial endothelial cell migration depends on the presence of the urokinase receptor. Circ Res 2004;94:1562-70. [PubMed]
- Bajou K, Noël A, Gerard RD, et al. Absence of host plasminogen activator inhibitor 1 prevents cancer invasion and vascularization. Nat Med 1998;4:923-8. [PubMed]
- Jänicke F, Schmitt M, Pache L, et al. Urokinase (uPA) and its inhibitor PAI-1 are strong and independent prognostic factors in node-negative breast cancer. Breast Cancer Res Treat 1993;24:195-208. [PubMed]
- Felsenfeld DP, Choquet D, Sheetz MP. Ligand binding regulates the directed movement of beta1 integrins on fibroblasts. Nature 1996;383:438-40. [PubMed]
- Lauffenburger DA, Horwitz AF. Cell migration: a physically integrated molecular process. Cell 1996;84:359-69. [PubMed]
- Lawson MA, Maxfield FR. Ca(2+)-and calcineurin-dependent recycling of an integrin to the front of migrating neutrophils. Nature 1995;377:75-9. [PubMed]
- Zoellner H, Höfler M, Beckmann R, et al. Fibrinolytic proteins in apoptotic human umbilical vein endothelial cells. Thromb Res 1998;91:209-19. [PubMed]
- Filardo EJ, Brooks PC, Deming SL, et al. Requirement of the NPXY motif in the integrin beta 3 subunit cytoplasmic tail for melanoma cell migration in vitro and in vivo. J Cell Biol 1995;130:441-50. [PubMed]
- Li Y, Marzolo MP, van Kerkhof P, et al. The YXXL motif, but not the two NPXY motifs, serves as the dominant endocytosis signal for low density lipoprotein receptor-related protein. J Biol Chem 2000;275:17187-94. [PubMed]
- Fabbri M, Fumagalli L, Bossi G, et al. A tyrosine-based sorting signal in the beta2 integrin cytoplasmic domain mediates its recycling to the plasma membrane and is required for ligand-supported migration. EMBO J 1999;18:4915-25. [PubMed]
- Chaurasia P, Aguirre-Ghiso JA, Liang OD, et al. A region in urokinase plasminogen receptor domain III controlling a functional association with alpha5beta1 integrin and tumor growth. J Biol Chem 2006;281:14852-63. [PubMed]
- Carriero MV, Del Vecchio S, Capozzoli M, et al. Urokinase receptor interacts with alpha(v)beta5 vitronectin receptor, promoting urokinase-dependent cell migration in breast cancer. Cancer Res 1999;59:5307-14. [PubMed]
- Czekay RP, Aertgeerts K, Curriden SA, et al. Plasminogen activator inhibitor-1 detaches cells from extracellular matrices by inactivating integrins. J Cell Biol 2003;160:781-91. [PubMed]
- Prager GW, Breuss JM, Steurer S, et al. Vascular endothelial growth factor (VEGF) induces rapid prourokinase (pro-uPA) activation on the surface of endothelial cells. Blood 2004;103:955-62. [PubMed]
- Herz J, Clouthier DE, Hammer RE. LDL receptor-related protein internalizes and degrades uPA-PAI-1 complexes and is essential for embryo implantation. Cell 1992;71:411-21. [PubMed]
- Nykjaer A, Conese M, Christensen EI, et al. Recycling of the urokinase receptor upon internalization of the uPA:serpin complexes. EMBO J 1997;16:2610-20. [PubMed]
- Prager GW, Mihaly J, Brunner PM, et al. Urokinase mediates endothelial cell survival via induction of the X-linked inhibitor of apoptosis protein. Blood 2009;113:1383-90. [PubMed]
- Mandriota SJ, Seghezzi G, Vassalli JD, et al. Vascular endothelial growth factor increases urokinase receptor expression in vascular endothelial cells. J Biol Chem 1995;270:9709-16. [PubMed]
- Brunner PM, Heier PC, Mihaly-Bison J, et al. Density enhanced phosphatase-1 down-regulates urokinase receptor surface expression in confluent endothelial cells. Blood 2011;117:4154-61. [PubMed]
- Stupack DG, Cheresh DA. ECM remodeling regulates angiogenesis: endothelial integrins look for new ligands. Sci STKE 2002;2002:pe7.
- Alghisi GC, Rüegg C. Vascular integrins in tumor angiogenesis: mediators and therapeutic targets. Endothelium 2006;13:113-35. [PubMed]
- Hynes RO. Integrins: versatility, modulation, and signaling in cell adhesion. Cell 1992;69:11-25. [PubMed]
- Adams RH, Alitalo K. Molecular regulation of angiogenesis and lymphangiogenesis. Nat Rev Mol Cell Biol 2007;8:464-78. [PubMed]
- Hynes RO. Integrins: bidirectional, allosteric signaling machines. Cell 2002;110:673-87. [PubMed]
- van der Flier A, Sonnenberg A. Function and interactions of integrins. Cell Tissue Res 2001;305:285-98. [PubMed]
- Colombatti A, Bonaldo P, Doliana R. Type A modules: interacting domains found in several non-fibrillar collagens and in other extracellular matrix proteins. Matrix 1993;13:297-306. [PubMed]
- Walker JL, Fournier AK, Assoian RK. Regulation of growth factor signaling and cell cycle progression by cell adhesion and adhesion-dependent changes in cellular tension. Cytokine Growth Factor Rev 2005;16:395-405. [PubMed]
- Gamble LJ, Borovjagin AV, Matthews QL. Role of RGD-containing ligands in targeting cellular integrins: Applications for ovarian cancer virotherapy Exp Ther Med 2010;1:233-40. [PubMed]
- Critchley DR. Cytoskeletal proteins talin and vinculin in integrin-mediated adhesion. Biochem Soc Trans 2004;32:831-6. [PubMed]
- Schwartz MA. Integrin signaling revisited. Trends Cell Biol 2001;11:466-70. [PubMed]
- Bolós V, Gasent JM, López-Tarruella S, et al. The dual kinase complex FAK-Src as a promising therapeutic target in cancer. Onco Targets Ther 2010;3:83-97. [PubMed]
- Hehlgans S, Haase M, Cordes N. Signalling via integrins: implications for cell survival and anticancer strategies. Biochim Biophys Acta 2007;1775:163-80.
- Friedlander M, Brooks PC, Shaffer RW, et al. Definition of two angiogenic pathways by distinct alpha v integrins. Science 1995;270:1500-2. [PubMed]
- Hood JD, Frausto R, Kiosses WB, et al. Differential alphav integrin-mediated Ras-ERK signaling during two pathways of angiogenesis. J Cell Biol 2003;162:933-43. [PubMed]
- Alavi A, Hood JD, Frausto R, et al. Role of Raf in vascular protection from distinct apoptotic stimuli. Science 2003;301:94-6. [PubMed]
- Avraamides CJ, Garmy-Susini B, Varner JA. Integrins in angiogenesis and lymphangiogenesis. Nat Rev Cancer 2008;8:604-17. [PubMed]
- George EL, Georges-Labouesse EN, Patel-King RS, et al. Defects in mesoderm, neural tube and vascular development in mouse embryos lacking fibronectin. Development 1993;119:1079-91. [PubMed]
- Fässler R, Meyer M. Consequences of lack of beta 1 integrin gene expression in mice. Genes Dev 1995;9:1896-908. [PubMed]
- Fässler R, Pfaff M, Murphy J, et al. Lack of beta 1 integrin gene in embryonic stem cells affects morphology, adhesion, and migration but not integration into the inner cell mass of blastocysts. J Cell Biol 1995;128:979-88. [PubMed]
- Goh KL, Yang JT, Hynes RO. Mesodermal defects and cranial neural crest apoptosis in alpha5 integrin-null embryos. Development 1997;124:4309-19. [PubMed]
- Kim S, Bell K, Mousa SA, et al. Regulation of angiogenesis in vivo by ligation of integrin alpha5beta1 with the central cell-binding domain of fibronectin. Am J Pathol 2000;156:1345-62. [PubMed]
- Boudreau NJ, Varner JA. The homeobox transcription factor Hox D3 promotes integrin alpha5beta1 expression and function during angiogenesis. J Biol Chem 2004;279:4862-8. [PubMed]
- Yang JT, Rayburn H, Hynes RO. Embryonic mesodermal defects in alpha 5 integrin-deficient mice. Development 1993;119:1093-105. [PubMed]
- Bader BL, Rayburn H, Crowley D, et al. Extensive vasculogenesis, angiogenesis, and organogenesis precede lethality in mice lacking all alpha v integrins. Cell 1998;95:507-19. [PubMed]
- Reynolds LE, Wyder L, Lively JC, et al. Enhanced pathological angiogenesis in mice lacking beta3 integrin or beta3 and beta5 integrins. Nat Med 2002;8:27-34. [PubMed]
- Bell-McGuinn KM, Matthews CM, Ho SN, et al. A phase II, single-arm study of the anti-α5β1 integrin antibody volociximab as monotherapy in patients with platinum-resistant advanced epithelial ovarian or primary peritoneal cancer. Gynecol Oncol 2011;121:273-9. [PubMed]
- Brooks PC, Clark RA, Cheresh DA. Requirement of vascular integrin alpha v beta 3 for angiogenesis. Science 1994;264:569-71. [PubMed]
- Tonnesen MG, Feng X, Clark RA. Angiogenesis in wound healing. J Investig Dermatol Symp Proc 2000;5:40-6. [PubMed]
- Drake CJ, Cheresh DA, Little CD. An antagonist of integrin alpha v beta 3 prevents maturation of blood vessels during embryonic neovascularization. J Cell Sci 1995;108:2655-61. [PubMed]
- Senger DR, Claffey KP, Benes JE, et al. Angiogenesis promoted by vascular endothelial growth factor: regulation through alpha1beta1 and alpha2beta1 integrins. Proc Natl Acad Sci U S A 1997;94:13612-7. [PubMed]
- Whelan MC, Senger DR. Collagen I initiates endothelial cell morphogenesis by inducing actin polymerization through suppression of cyclic AMP and protein kinase A. J Biol Chem 2003;278:327-34. [PubMed]
- Sweeney SM, DiLullo G, Slater SJ, et al. Angiogenesis in collagen I requires alpha2beta1 ligation of a GFP*GER sequence and possibly p38 MAPK activation and focal adhesion disassembly. J Biol Chem 2003;278:30516-24. [PubMed]
- Senger DR, Perruzzi CA, Streit M, et al. The alpha(1)beta(1) and alpha(2)beta(1) integrins provide critical support for vascular endothelial growth factor signaling, endothelial cell migration, and tumor angiogenesis. Am J Pathol 2002;160:195-204. [PubMed]
- Millard M, Odde S, Neamati N. Integrin targeted therapeutics. Theranostics 2011;1:154-88. [PubMed]
- Zhang D, Pier T, McNeel DG, et al. Effects of a monoclonal anti-alphavbeta3 integrin antibody on blood vessels-a pharmacodynamic study. Invest New Drugs 2007;25:49-55. [PubMed]
- Cai W, Wu Y, Chen K, et al. In vitro and in vivo characterization of 64Cu-labeled Abegrin, a humanized monoclonal antibody against integrin alpha v beta 3. Cancer Res 2006;66:9673-81. [PubMed]
- Desgrosellier JS, Cheresh DA. Integrins in cancer: biological implications and therapeutic opportunities. Nat Rev Cancer 2010;10:9-22. [PubMed]
- Kuwada SK. Drug evaluation: Volociximab, an angiogenesis-inhibiting chimeric monoclonal antibody. Curr Opin Mol Ther 2007;9:92-8. [PubMed]
- Ricart AD, Tolcher AW, Liu G, et al. Volociximab, a chimeric monoclonal antibody that specifically binds alpha5beta1 integrin: a phase I, pharmacokinetic, and biological correlative study. Clin Cancer Res 2008;14:7924-9. [PubMed]
- Trikha M, Zhou Z, Nemeth JA, et al. CNTO 95, a fully human monoclonal antibody that inhibits alphav integrins, has antitumor and antiangiogenic activity in vivo. Int J Cancer 2004;110:326-35. [PubMed]
- O'Day SJ, Pavlick AC, Albertini MR, et al. Clinical and pharmacologic evaluation of two dose levels of intetumumab (CNTO 95) in patients with melanoma or angiosarcoma. Invest New Drugs 2011. [Epub ahead of print]
- Mullamitha SA, Ton NC, Parker GJ, et al. Phase I evaluation of a fully human anti-alphav integrin monoclonal antibody (CNTO 95) in patients with advanced solid tumors. Clin Cancer Res 2007;13:2128-35. [PubMed]
- Brooks PC, Klemke RL, Schon S, et al. Insulin-like growth factor receptor cooperates with integrin alpha v beta 5 to promote tumor cell dissemination in vivo. J Clin Invest 1997;99:1390-8. [PubMed]
- Ferrara N. VEGF as a therapeutic target in cancer. Oncology 2005;69:11-6. [PubMed]
- Zhong C, Qu X, Tan M, et al. Characterization and regulation of bv8 in human blood cells. Clin Cancer Res 2009;15:2675-84. [PubMed]
- Shojaei F, Zhong C, Wu X, et al. Role of myeloid cells in tumor angiogenesis and growth. Trends Cell Biol 2008;18:372-8. [PubMed]
- Shojaei F, Wu X, Qu X, et al. G-CSF-initiated myeloid cell mobilization and angiogenesis mediate tumor refractoriness to anti-VEGF therapy in mouse models. Proc Natl Acad Sci U S A 2009;106:6742-7. [PubMed]
- Yang L, DeBusk LM, Fukuda K, et al. Expansion of myeloid immune suppressor Gr+CD11b+ cells in tumor-bearing host directly promotes tumor angiogenesis. Cancer Cell 2004;6:409-21. [PubMed]
- Shojaei F, Wu X, Zhong C, et al. Bv8 regulates myeloid-cell-dependent tumour angiogenesis. Nature 2007;450:825-31. [PubMed]
- Shojaei F, Singh M, Thompson JD, et al. Role of Bv8 in neutrophil-dependent angiogenesis in a transgenic model of cancer progression. Proc Natl Acad Sci U S A 2008;105:2640-5. [PubMed]
- Okazaki T, Ebihara S, Asada M, et al. Granulocyte colony-stimulating factor promotes tumor angiogenesis via increasing circulating endothelial progenitor cells and Gr1+CD11b+ cells in cancer animal models. Int Immunol 2006;18:1-9. [PubMed]
- Natori T, Sata M, Washida M, et al. G-CSF stimulates angiogenesis and promotes tumor growth: potential contribution of bone marrow-derived endothelial progenitor cells. Biochem Biophys Res Commun 2002;297:1058-61. [PubMed]
- Orimo A, Gupta PB, Sgroi DC, et al. Stromal fibroblasts present in invasive human breast carcinomas promote tumor growth and angiogenesis through elevated SDF-1/CXCL12 secretion. Cell 2005;121:335-48. [PubMed]
- Crawford Y, Kasman I, Yu L, et al. PDGF-C mediates the angiogenic and tumorigenic properties of fibroblasts associated with tumors refractory to anti-VEGF treatment. Cancer Cell 2009;15:21-34. [PubMed]
- Li X, Kumar A, Zhang F, et al. VEGF-independent angiogenic pathways induced by PDGF-C. Oncotarget 2010;1:309-14. [PubMed]
- Kindler HL, Niedzwiecki D, Hollis D, et al. Gemcitabine plus bevacizumab compared with gemcitabine plus placebo in patients with advanced pancreatic cancer: phase III trial of the Cancer and Leukemia Group B (CALGB 80303). J Clin Oncol 2010;28:3617-22. [PubMed]
- Kerbel RS. Tumor angiogenesis. N Engl J Med 2008;358:2039-49. [PubMed]
- Kindler HL, Wroblewski K, Wallace JA, et al. Gemcitabine plus sorafenib in patients with advanced pancreatic cancer: a phase II trial of the University of Chicago Phase II Consortium. Invest New Drugs 2012;30:382-6. [PubMed]
- Gharibo M, Patrick-Miller L, Zheng L, et al. A phase II trial of imatinib mesylate in patients with metastatic pancreatic cancer. Pancreas 2008;36:341-5. [PubMed]
- Xian X, Håkansson J, Ståhlberg A, et al. Pericytes limit tumor cell metastasis. J Clin Invest 2006;116:642-51. [PubMed]
- Stewart MW. Aflibercept (VEGF-TRAP): The Next Anti-VEGF Drug. Inflamm Allergy Drug Targets 2011. [Epub ahead of print]
- Leighl NB, Raez LE, Besse B, et al. A multicenter, phase 2 study of vascular endothelial growth factor trap (Aflibercept) in platinum-and erlotinib-resistant adenocarcinoma of the lung. J Thorac Oncol 2010;5:1054-9. [PubMed]
- Twardowski P, Stadler WM, Frankel P, et al. Phase II study of Aflibercept (VEGF-Trap) in patients with recurrent or metastatic urothelial cancer, a California Cancer Consortium Trial. Urology 2010;76:923-6. [PubMed]
- Tabernero J VCELRea. Results from VELOUR, a phase 3 study of aflibercept versus placebo in combination with FOLFIRI for the treatment of patients with previously treated metastatic colorectal cancer. 2011 European Multidisciplinary Congress Abstract 6LBA.
- Wells SA Jr, Gosnell JE, Gagel RF, et al. Vandetanib for the treatment of patients with locally advanced or metastatic hereditary medullary thyroid cancer. J Clin Oncol 2010;28:767-72. [PubMed]
- Qi WX, Tang LN, He AN, et al. The role of vandetanib in the second-line treatment for advanced non-small-cell-lung cancer: a meta-analysis of four randomized controlled trials. Lung 2011;189:437-43. [PubMed]
- Oh SY, Kim WS, Lee DH, et al. Phase II study of gemcitabine for treatment of patients with advanced stage marginal zone B-cell lymphoma: Consortium for Improving Survival of Lymphoma (CISL) trial. Invest New Drugs 2010;28:171-7. [PubMed]
- Nabors LB, Mikkelsen T, Rosenfeld SS, et al. Phase I and correlative biology study of cilengitide in patients with recurrent malignant glioma. J Clin Oncol 2007;25:1651-7. [PubMed]
- Weller M. Novel diagnostic and therapeutic approaches to malignant glioma. Swiss Med Wkly 2011;141:w13210. [PubMed]
- Vermorken JB, Guigay J, Mesia R, et al. Phase I/II trial of cilengitide with cetuximab, cisplatin and 5-fluorouracil in recurrent and/or metastatic squamous cell cancer of the head and neck: findings of the phase I part. Br J Cancer 2011;104:1691-6. [PubMed]
- Cheng C, Komljenovic D, Pan L, et al. Evaluation of treatment response of cilengitide in an experimental model of breast cancer bone metastasis using dynamic PET with 18F-FDG. Hell J Nucl Med 2011;14:15-20. [PubMed]
- Reardon DA, Neyns B, Weller M, et al. Cilengitide: an RGD pentapeptide ανβ3 and ανβ5 integrin inhibitor in development for glioblastoma and other malignancies. Future Oncol 2011;7:339-54. [PubMed]
- Oliveira-Ferrer L, Hauschild J, Fiedler W, et al. Cilengitide induces cellular detachment and apoptosis in endothelial and glioma cells mediated by inhibition of FAK/src/AKT pathway. J Exp Clin Cancer Res 2008;27:86. [PubMed]
- Nisato RE, Tille JC, Jonczyk A, et al. alphav beta 3 and alphav beta 5 integrin antagonists inhibit angiogenesis in vitro. Angiogenesis 2003;6:105-19. [PubMed]
- Hurwitz HI, Dowlati A, Saini S, et al. Phase I trial of pazopanib in patients with advanced cancer. Clin Cancer Res 2009;15:4220-7. [PubMed]
- Hutson TE, Davis ID, Machiels JP, et al. Efficacy and safety of pazopanib in patients with metastatic renal cell carcinoma. J Clin Oncol 2010;28:475-80. [PubMed]
- Sternberg CN, Davis ID, Mardiak J, et al. Pazopanib in locally advanced or metastatic renal cell carcinoma: results of a randomized phase III trial. J Clin Oncol 2010;28:1061-8. [PubMed]
- Sonpavde G, Hutson TE. Pazopanib: a novel multitargeted tyrosine kinase inhibitor. Curr Oncol Rep 2007;9:115-9. [PubMed]
- Friedlander M, Hancock KC, Rischin D, et al. A Phase II, open-label study evaluating pazopanib in patients with recurrent ovarian cancer. Gynecol Oncol 2010;119:32-7. [PubMed]
- Taylor SK, Chia S, Dent S, et al. A phase II study of pazopanib in patients with recurrent or metastatic invasive breast carcinoma: a trial of the Princess Margaret Hospital phase II consortium. Oncologist 2010;15:810-8. [PubMed]
- Sleijfer S, Ray-Coquard I, Papai Z, et al. Pazopanib, a multikinase angiogenesis inhibitor, in patients with relapsed or refractory advanced soft tissue sarcoma: a phase II study from the European organisation for research and treatment of cancer-soft tissue and bone sarcoma group (EORTC study 62043). J Clin Oncol 2009;27:3126-32. [PubMed]
- Melchert M, List A. The thalidomide saga. Int J Biochem Cell Biol 2007;39:1489-99. [PubMed]
- D'Amato RJ, Loughnan MS, Flynn E, et al. Thalidomide is an inhibitor of angiogenesis. Proc Natl Acad Sci U S A 1994;91:4082-5. [PubMed]
- Barlogie B, Desikan R, Eddlemon P, et al. Extended survival in advanced and refractory multiple myeloma after single-agent thalidomide: identification of prognostic factors in a phase 2 study of 169 patients. Blood 2001;98:492-4. [PubMed]
- Rajkumar SV, Blood E, Vesole D, et al. Phase III clinical trial of thalidomide plus dexamethasone compared with dexamethasone alone in newly diagnosed multiple myeloma: a clinical trial coordinated by the Eastern Cooperative Oncology Group. J Clin Oncol 2006;24:431-6. [PubMed]
- Dahut WL, Gulley JL, Arlen PM, et al. Randomized phase II trial of docetaxel plus thalidomide in androgen-independent prostate cancer. J Clin Oncol 2004;22:2532-9. [PubMed]
- Zhang W, Fulci G, Buhrman JS, et al. Bevacizumab With Angiostatin-armed oHSV Increases Antiangiogenesis and Decreases Bevacizumab-induced Invasion in U87 Glioma. Mol Ther 201. [Epub ahead of print]
- Redlitz A, Daum G, Sage EH. Angiostatin diminishes activation of the mitogen-activated protein kinases ERK-1 and ERK-2 in human dermal microvascular endothelial cells. J Vasc Res 1999;36:28-34. [PubMed]
- Kurup A, Lin CW, Murry DJ, et al. Recombinant human angiostatin (rhAngiostatin) in combination with paclitaxel and carboplatin in patients with advanced non-small-cell lung cancer: a phase II study from Indiana University. Ann Oncol 2006;17:97-103. [PubMed]
- Kim YM, Hwang S, Kim YM, et al. Endostatin blocks vascular endothelial growth factor-mediated signaling via direct interaction with KDR/Flk-1. J Biol Chem 2002;277:27872-9. [PubMed]
- Fukumoto S, Morifuji M, Katakura Y, et al. Endostatin inhibits lymph node metastasis by a down-regulation of the vascular endothelial growth factor C expression in tumor cells. Clin Exp Metastasis 2005;22:31-8. [PubMed]
- Jia Y, Liu M, Huang W, et al. Recombinant Human Endostatin Endostar Inhibits Tumor Growth and Metastasis in a Mouse Xenograft Model of Colon Cancer. Pathol Oncol Res 2012;18:315-23. [PubMed]
- Wang J, Sun Y, Liu Y, et al. Zhongguo Fei Ai Za Zhi 2005;8:283-90. [PubMed]
- Kulke MH, Bergsland EK, Ryan DP, et al. Phase II study of recombinant human endostatin in patients with advanced neuroendocrine tumors. J Clin Oncol 2006;24:3555-61. [PubMed]