Functional CT imaging techniques for the assessment of angiogenesis in lung cancer
Introduction
Lung cancer remains the leading cause of cancer mortality worldwide. Only 7% of patients will survive five years (1). At the time of diagnosis most patients already will have advanced disease and may only be treated by palliation. However, ongoing developments of chemotherapy and the availability of targeted therapies have improved the survival of patients with NSCLC.
Contrast-enhanced CT of the chest still remains the standard imaging test for the initial assessment of patients with suspected lung cancer. The broad clinical use of thin-section multi-detector CT inevitably leads to the detection of a large number of pulmonary nodules. Previous lung cancer screening trials have shown that the majority of individuals with a certain risk profile will have at least one non-calcified pulmonary nodule. At surgical biopsy approximately 50% of these indeterminate pulmonary nodules will turn out to be benign (2). Therefore, non-invasive characterization of pulmonary nodules by imaging is of major importance in the clinical work-up of incidentally detected pulmonary nodules. Using standard contrast-enhanced CT the characterization of pulmonary nodules is based on simple morphological criteria e.g., irregular or spiculated margins as a sign for malignancy or calcifications as a sign of benignity. However, in a clinical context these simple morphologic criteria are unreliable for an accurate differentiation between benign and malignant lung nodules.
In addition to nodule differentiation the assessment of therapy response by imaging is of major importance. The most widely accepted method for the assessment of response is based on measurements of the tumor size and subsequent classification of response using the REsponse Criteria In Solid Tumors (RECIST). Despite current improvements of the revised RECIST 1.1 classification there are well known limitations of RECIST (3). This includes major changes of the tumor morphology during treatment, such as cavitation, peritumoral inflammation, and atelectasis, which make a reliable assessment of tumor size impossible. Also improvements in systemic anticancer treatment such as the introduction of targeted agents have a major impact on the ability of imaging to assess treatment response. It has been shown, that response patterns are significantly different in patients undergoing targeted treatment. In particular less patients will show a decrease in tumor size as compared to conventional systemic chemotherapy. Moreover, in a study by Herbst et al. stable disease was the best criteria of response in 65% of all patients undergoing a combination therapy of bevacizumab and erlotinib (4).
As a consequence, non-invasive functional imaging techniques such as 18F-FDG PET-CT and dynamic contrast enhanced CT and MRI have gained major interest to improve the differentiation of lung nodules and response assessment of lung cancer during therapy.
Angiogenesis is a key factor in cancer growth and metastasis. In particular, angiogenesis promotes tumor growth by supplying the tumor with required nutrients. Furthermore, angiogenesis plays a major role in metastasis by limiting the integrity of tumor vessel walls. It is well known that the extent of vascularity differs between benign and malignant pulmonary nodules. This can be used for non-invasive tumor characterization by imaging techniques that allow a visualization of angiogenesis. Several imaging modalities can be used to assess differences in vascularity including conventional angiography (5), dynamic contrast-enhanced CT (6,7), and MRI (8,9). Likewise, these modalities may not only be used for nodule characterization but also to assess treatment-related changes in vascularity as a surrogate for response (10,11).
Functional CT imaging techniques
Dynamic contrast-enhanced CT
Due to tumor angiogenesis, regional blood flow and blood volume and consecutively contrast-enhancement is increased in malignant pulmonary lesions. Changes in perfusion and vascular permeability can be assessed by means of dynamic contrast-enhanced CT, which is based on the analysis of time-density curves acquired with consecutive scans after injection of a bolus of iodinated contrast material (2,7,12-16). The feasibility of dynamic contrast-enhanced CT for the characterization of pulmonary nodules has been demonstrated in a large number of studies using different scanner technology and protocols. A common finding in all studies was that malignant nodules show a higher contrast-enhancement than benign nodules. This was also proven by a prospective, multicenter trial evaluating dynamic contrast-enhanced CT for the differentiation of benign and malignant pulmonary nodules (7).
Several studies have correlated the results of dynamic contrast-enhanced CT to histological parameters of tumor angiogenesis such as microvessel density (MVD) or expression of vascular endothelial growth factor (VEGF). For example, Tateishi et al. analyzed the correlation of tumor enhancement with MVD and VEGF expression in 130 patients with histological proven lung cancer (17). They found a significantly higher peak enhancement of VEGF-positive tumors than in VEGF-negative tumors. There was also a significant positive correlation (r=0.65) between the peak-enhancement of VEGF-positive lung tumors with MVD. Lymph node positive lung cancers showed a higher peak enhancement, VEGF-expression and MVD when compared to patients with lymph node negative cancers.
Besides the differentiation of benign and malignant nodules dynamic contrast-enhanced CT may be used for the assessment of tumor response to targeted therapy or radiotherapy. It has been reported (18) that microvascular damage is a key mechanism in tumor response to radiation. Therefore, reduced volume of the vascular bed following radiation therapy would be reflected by reduced blood flow, blood volume, and permeability–surface area product. Ng et al. (19) found that hypo-fractionated radiotherapy increased tumor vascular blood volume and permeability of NSCLC. In a recently published study by Wang et al. it has been demonstrated that NSCLCs with higher perfusion is more sensitive to radio-chemo therapy than that with lower perfusion. Moreover, the study suggests that after radio-chemo therapy, findings at perfusion CT are a significant predictor of early tumor response and overall survival among patients with NSCLC. In another recent study by Lind et al. tumor blood flow measured by serial dynamic contrast-enhanced CT exams in 23 patients with advanced NSCLC was correlated with response to anti-angiogenic and anti-EGFR targeted therapy (i.e., sorafenib and erlotinib). CT exams were performed at baseline as well as 3 and 6 weeks after the initiation of treatment. Tumor perfusion was lower in responders than non-responders at week 3 and 6. Patients with a decrease of blood flow larger than the median at week 6 tended to have a longer (but non significant) PFS (20).
Despite these promising results, dynamic contrast-enhanced has not yet been established as a standard procedure in clinical routine. In addition to concerns regarding the applied radiation dose resulting from dynamic CT scans, as well as lack of standardized post-processing and quantification tools the method has been limited by the low temporal resolution and small detector coverage of CT scanner hardware. Consequently, in the majority of published studies the sample volume of dynamic contrast-enhanced CT has been limited to a single or a few slices, which is problematic for a reproducible quantitative assessment of angiogenesis in patients with larger and mobile tumors. Ng et al. demonstrated in 15 patients with histologically proven NSCLC that tumor blood volume varies up to 15% between adjacent slices using dynamic contrast-enhanced CT (21). With the most recent multidetector CT scanner systems with broad detector arrays or shuffle mode acquisitions, however, it became feasible to cover larger tissue volumes (19) and whole tumors (12). Using such scanner equipment it has recently been demonstrated that a substantial intra-observer agreement for lung cancer perfusion CT measurements can be achieved encouraging the use for tumor characterization and therapy response monitoring (22).
Also recently, researchers from Japan suggested that based on their study in 50 patients dynamic contrast-enhanced perfusion CT with a larger area 320 slice detector may even be more specific and accurate than 18F-FDG-PET/CT for differentiating malignant from benign pulmonary nodules (23).
Dual-energy CT
Dual Energy CT (DECT) is a new CT technique that allows the quantification of iodinated contrast material by applying different X-ray spectra and analyzing the differences in attenuation which allows differentiation of iodine from other materials due to its stronger photoelectric absorption (24). Therefore, DECT allows a selective visualization and quantification of iodinated contrast media in a single scan, and may be used as a surrogate for local blood volume at the time of the data acquisition. In addition to the functional component of DECT a standard CT scan with imaging properties equivalent to a standard single energy CT scan can be processed. DECT can further be used to process a virtual non-enhanced CT data set from a contrast-enhanced DECT data set that has similar imaging characteristics than a true non-enhanced CT data set (Figure 1).
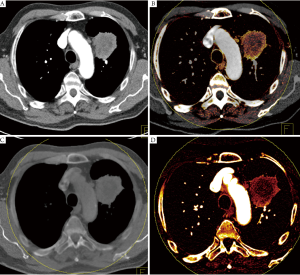
Several studies have applied DECT for the differentiation between benign and malignant lesions, to improve tumor conspicuity, and to assess treatment response in renal cancer, hepatic cancer, or gastrointestinal stromal tumors (25-27).
So far, only one study has evaluated DECT for the characterization of pulmonary nodules (28). It was shown that DECT allows a clear visualization of calcifications (as an indicator of benignity) in enhancing lung nodules by calculating VNE datasets. Due to this DECT can obviate the routine need for true unenhanced acquisitions and thus reduce the radiation exposure to patients (28).
Due to the selective visualization of iodine by DECT the degree of enhancement can be analyzed by DECT without the need of a separate unenhanced scan. It may be assumed that if the scan delay is chosen in an appropriate way the iodine map of DECT may be used as a surrogate for the regional blood volume.
Comparison of the CT number on non-enhanced weighted average and virtual non-enhanced images showed good agreement in this study. The CT numbers of pulmonary nodules on iodine-enhanced image and the degree of enhancement as determined by the difference of CT numbers of the contrast-enhanced and non-enhanced weighted average CT images also showed good agreement. The sum of the CT numbers of pulmonary nodules on iodine-enhanced image and virtual non-enhanced image showed a strong agreement with that on enhanced weighted average images. A total of 45 of 49 nodules were confirmed as benign or malignant on the basis of percutaneous needle aspiration, whereas four nodules could not confirmatively diagnosed. The rate of malignancy was 55.6% (25 of 45 nodules). The results of both the CT number on iodine-enhanced images and the degree of enhancement showed that malignant nodules showed a significantly higher enhancement than benign nodules (P=0.001). By comparing the diagnostic accuracy with a cutoff value of 20 HU for malignant nodules, which was selected as a cutoff value based on previous studies on dynamic contrast enhanced CT (7,13-15,29), iodine-enhanced images had a higher sensitivity and accuracy than the degree of enhancement.
Besides the differentiation of pulmonary nodules another potential application of DECT could be the evaluation of therapy response by analyzing changes in the tumor iodine distribution, especially in patients undergoing novel targeted therapy.
In a retrospective study by our own group we investigated the correlation between maximum standardized uptake value (SUVmax) of 18F-FDG-PET-CT and iodine-related attenuation of DECT of primary tumors and thoracic lymph nodes in 37 patients with lung cancer (30) (Figure 2). The mean and maximum IRA of DECT as well as of virtual unenhanced and virtual 120 kV images of DECT was analyzed and correlated to the SUVmax. A moderate correlation was found between SUVmax and maximum IRA in all tumors (n=37; r=0.507; P=0.025) whereas a strong correlation was found in patients with study intervals ≤21 days (n=17; r=0.768; P=0.017). Thoracic lymph nodes showed a moderate correlation between SUVmax and maximum IRA in patients with study intervals ≤21 days (r=0.654; P=0.010) whereas a weak correlation was found between SUVmax and maximum IRA in patients with study intervals >21 days (r=0.299; P=0.035). We concluded that DECT could serve as a valuable functional imaging test for patients with NSCLC as the IRA of DECT seems to correlate with the SUVmax of 18F-FDG-PET-CT.
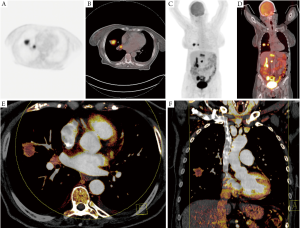
In a recently published study, Kawai et al. investigated the potential of DECT to demonstrate contrast enhancement of pulmonary lesions with ground-glass attenuation (GGA). This is of major importance, as especially in the context of screening trials more lesions with GGA have to be differentiated between benign (e.g., pneumonia, hemorrhage or localized fibrosis) and malignant etiologies (adeoncarcinoma). In phantom experiments they found a good correlation between DECT measured iodine values and iodine concentration. In a clinical study contrast enhancement could be demonstrated in 22 adenocarcinomas but not in a pulmonary hemorrhage as well as in inflammatory lesions.
Future validation studies are required to analyse if the iodine related attenuation of DECT also correlated to histopathological parameters of angiogenesis. If so, DECT might be used similarly as dynamic contrast-enhanced CT. The main clinical benefit of a single acquisition DECT scan compared to a dynamic contrast enhanced CT would be that the whole tumor is covered within a single scan and that the method is not as susceptible for respiratory motion as the data is acquired within a single scan during a single breathhold (Figure 3). Moreover, radiation dose and patient room time could be significantly reduced.
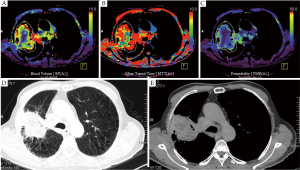
Conclusions
Dual Energy CT and Dynamic contrast-enhanced perfusion CT are important imaging tools for a non-invasive assessment of regional blood volume and angiogenesis. In clinical practice this will be of importance for non-invasive characterization of pulmonary nodules and response assessment in lung cancer.
Acknowledgements
Disclosure: The authors declare no conflict of interest.
References
- Rami-Porta R, Crowley JJ, Goldstraw P. The revised TNM staging system for lung cancer. Ann Thorac Cardiovasc Surg 2009;15:4-9. [PubMed]
- Swensen SJ. Functional CT: lung nodule evaluation. Radiographics 2000;20:1178-81. [PubMed]
- Eisenhauer EA, Therasse P, Bogaerts J, et al. New response evaluation criteria in solid tumours: revised RECIST guideline (version 1.1). Eur J Cancer 2009;45:228-47. [PubMed]
- Herbst RS, Johnson DH, Mininberg E, et al. Phase I/II trial evaluating the anti-vascular endothelial growth factor monoclonal antibody bevacizumab in combination with the HER-1/epidermal growth factor receptor tyrosine kinase inhibitor erlotinib for patients with recurrent non-small-cell lung cancer. J Clin Oncol 2005;23:2544-55. [PubMed]
- Viamonte M Jr. Angiographic evaluation of lung neoplasms. Radiol Clin North Am 1965;3:529-42. [PubMed]
- Littleton JT, Durizch ML, Moeller G, et al. Pulmonary masses: contrast enhancement. Radiology 1990;177:861-71. [PubMed]
- Swensen SJ, Viggiano RW, Midthun DE, et al. Lung nodule enhancement at CT: multicenter study. Radiology 2000;214:73-80. [PubMed]
- Kono M, Adachi S, Kusumoto M, et al. Clinical utility of Gd-DTPA-enhanced magnetic resonance imaging in lung cancer. J Thorac Imaging 1993;8:18-26. [PubMed]
- Gückel C, Schnabel K, Deimling M, et al. Solitary pulmonary nodules: MR evaluation of enhancement patterns with contrast-enhanced dynamic snapshot gradient-echo imaging. Radiology 1996;200:681-6. [PubMed]
- de Langen AJ, van den Boogaart V, Lubberink M, et al. Monitoring response to antiangiogenic therapy in non-small cell lung cancer using imaging markers derived from PET and dynamic contrast-enhanced MRI. J Nucl Med 2011;52:48-55. [PubMed]
- Lind JS, Postmus PE, Smit EF. Osteoblastic bone lesions developing during treatment with erlotinib indicate major response in patients with non-small cell lung cancer: a brief report. J Thorac Oncol 2010;5:554-7. [PubMed]
- Li Y, Yang ZG, Chen TW, et al. Whole tumour perfusion of peripheral lung carcinoma: evaluation with first-pass CT perfusion imaging at 64-detector row CT. Clin Radiol 2008;63:629-35. [PubMed]
- Swensen SJ, Brown LR, Colby TV, et al. Pulmonary nodules: CT evaluation of enhancement with iodinated contrast material. Radiology 1995;194:393-8. [PubMed]
- Swensen SJ, Morin RL, Schueler BA, et al. Solitary pulmonary nodule: CT evaluation of enhancement with iodinated contrast material--a preliminary report. Radiology 1992;182:343-7. [PubMed]
- Yamashita K, Matsunobe S, Tsuda T, et al. Solitary pulmonary nodule: preliminary study of evaluation with incremental dynamic CT. Radiology 1995;194:399-405. [PubMed]
- Zhang M, Kono M. Solitary pulmonary nodules: evaluation of blood flow patterns with dynamic CT. Radiology 1997;205:471-8. [PubMed]
- Tateishi U, Kusumoto M, Nishihara H, et al. Contrast-enhanced dynamic computed tomography for the evaluation of tumor angiogenesis in patients with lung carcinoma. Cancer 2002;95:835-42. [PubMed]
- Garcia-Barros M, Paris F, Cordon-Cardo C, et al. Tumor response to radiotherapy regulated by endothelial cell apoptosis. Science 2003;300:1155-9. [PubMed]
- Ng QS, Goh V, Milner J, et al. Acute tumor vascular effects following fractionated radiotherapy in human lung cancer: In vivo whole tumor assessment using volumetric perfusion computed tomography. Int J Radiat Oncol Biol Phys 2007;67:417-24. [PubMed]
- Lind JS, Meijerink MR, Dingemans AM, et al. Dynamic contrast-enhanced CT in patients treated with sorafenib and erlotinib for non-small cell lung cancer: a new method of monitoring treatment? Eur Radiol 2010;20:2890-8. [PubMed]
- Ng QS, Goh V, Milner J, et al. Quantitative helical dynamic contrast enhanced computed tomography assessment of the spatial variation in whole tumour blood volume with radiotherapy in lung cancer. Lung Cancer 2010;69:71-6. [PubMed]
- Sauter AW, Merkle A, Schulze M, et al. Intraobserver and interobserver agreement of volume perfusion CT (VPCT) measurements in patients with lung lesions. Eur J Radiol 2012;81:2853-9. [PubMed]
- Ohno Y, Koyama H, Matsumoto K, et al. Differentiation of malignant and benign pulmonary nodules with quantitative first-pass 320-detector row perfusion CT versus FDG PET/CT. Radiology 2011;258:599-609. [PubMed]
- Johnson TR, Krauss B, Sedlmair M, et al. Material differentiation by dual energy CT: initial experience. Eur Radiol 2007;17:1510-7. [PubMed]
- Altenbernd J, Heusner TA, Ringelstein A, et al. Dual-energy-CT of hypervascular liver lesions in patients with HCC: investigation of image quality and sensitivity. Eur Radiol 2011;21:738-43. [PubMed]
- Graser A, Becker CR, Staehler M, et al. Single-phase dual-energy CT allows for characterization of renal masses as benign or malignant. Invest Radiol 2010;45:399-405. [PubMed]
- Apfaltrer P, Meyer M, Meier C, et al. Contrast-Enhanced Dual-Energy CT of Gastrointestinal Stromal Tumors: Is Iodine-Related Attenuation a Potential Indicator of Tumor Response? Invest Radiol 2012;47:65-70. [PubMed]
- Chae EJ, Song JW, Seo JB, et al. Clinical utility of dual-energy CT in the evaluation of solitary pulmonary nodules: initial experience. Radiology 2008;249:671-81. [PubMed]
- Swensen SJ, Morin RL, Aughenbaugh GL, et al. CT reconstruction algorithm selection in the evaluation of solitary pulmonary nodules. J Comput Assist Tomogr 1995;19:932-5. [PubMed]
- Schmid-Bindert G, Henzler T, Chu TQ, et al. Functional imaging of lung cancer using dual energy CT: how does iodine related attenuation correlate with standardized uptake value of 18FDG-PET-CT? Eur Radiol 2012;22:93-103. [PubMed]