Itraconazole can inhibit malignant pleural effusion by suppressing lymphangiogenesis in mice
Introduction
Non-small cell lung cancer (NSCLC) is still the leading cause of cancer mortality worldwide (1). When diagnosed, more than 15% of NSCLC patients present with malignant pleural effusion (MPE), and 50% of the patients will have MPE with the progression of the disease (2). As a poor prognostic marker for lung cancer patients, the causes and mechanisms involved in MPE has still been uncovered (3). Angiogenesis has been proved to be an important factor in promoting formation of MPE. However, anti-angiogenesis therapy in MPE still leaves much to be desired. Since lymphatic vessels have larger lumen and increased permeability compared to blood vessels, lymphangiogenesis is thought to play a crucial role in regional tumor lymph node metastasis and formation of MPE. Targeting MPE-associated lymphangiogenesis is considered to be an available therapy strategy for inhibiting MPE (4).
Itraconazole, an important antifungal agent, plays an important role in the conversion of lanosterol to ergosterol in fungi and lanosterol to cholesterol in humans through inhibiting lanosterol 14-α-demethylase. Existing evidence demonstrates that itraconazole has antiangiogenic potency and has been identified as a potent inhibitor of endothelial cell proliferation and can suppress matrigel-stimulated angiogensis in vitro (5,6). However, the inhibiting effect of itraconazole on the formation of MPE in NSCLC and whether itraconazole has anti-lymphangiogenesis potency or not has not yet been elucidated.
As proved in our previous study, angiogenesis and lymphangiogenesis both play an important role in formation of MPE, and MPE can be inhibited in vivo when angiogenesis and lymphangiogenesis were suppressed. Therefore, we give a hypothesis that itraconzole can act as a suppressor in MPE. The aim of our study was to investigate the effect of itraconazole on MPE in vivo and to explore whether the mechanism is associated with lymphangiogenesis.
Materials and methods
Animal experimental procedures were approved by Model Animal Research Centre of Jingling Hospital and conducted according to Institutional Animal Care and User guidelines.
Reagents and antibodies
Itraconazole was obtained from Janssen Company (Belgium), and hydroxypropyl-β-cyclodextrin (H-β-C) was obtained from Aoduonifu Company (Nanjing, China). The VEGF-C ELISA kit was obtained from R&D systems (USA). The mouse monoclonal D2-40 antibody and polyclonal goat anti-mouse VEGF-C antibody were both obtained from Abcam (USA).
Animals
BALB/c sex-matched nude mice (6-8 weeks old, weight of 18-24 g) were purchased from Model Animal Research Centre of Jingling Hospital. The animals were kept in rooms that were maintained at a constant temperature (22-25°) and humidity level (30-45%). Food, water and bedding were sterilized, and the animals were studied at the animal care facility for the MPE model.
Cell culture
The Lewis lung carcinoma (LLC) cell line was purchased from the Cell Bank of Chinese Academy of Sciences (Shanghai, China). The cells were cultured in Dulbecco’s Modified Eagle Medium (DMEM, Hyclone) with 10% fetal calf serum (Hyclone), 100 U/mL penicillin and 100 μg/mL streptomycin (Gibco). The cells were cultured at 37 °C in 5% CO2, grown to near confluence then harvested, washed with phosphate-buffered saline (PBS) and finally resuspended in DMEM to a concentration of 2×106/50 μL.
Pleural xenografts
According to our previous study, the mice were anesthetized with chloral hydrate, and a small vertical cut was done on the right chest wall near the manubrium sterni. The skin and subcutaneous fascia were carefully retracted, and 1×106 cells/50 μL DMEM were injected carefully into the pleural cavity at a depth of approximately 5 mm, ensuring minimal damage to the vasculature and other tissues. The procedure was conducted under a stereo microscope and no mortality was occurred (7).
Based on our previous experience, after the injection, a total 30 nu/nu mice were randomly divided into three groups with 10 mice in each group. H-β-C was used to dissolve itraconazole, so Animals in three groups were treated with H-β-C, low itraconazole (L-ITCZ) and high itraconazole (H-ITCZ), respectively. The mice were treated with itraconazole at a dose of 25 mg/kg as high dose and 8 mg/kg as low dose four times, with a 3-day interval between each treat, while the control group (H-β-C group) was similarly treated with 50 μL of H-β-C (130 mg/mL).
Computed tomography scanning
Fourteen days after injection of the LLC cells, the mice were scanned by computed tomography (CT, Siemens Somatom Sensation 16, 120 kVp, 93 µA) to observe the formation of pleural effusion. As described previously, the mice were anesthetized during the imaging session, and the real-time scanning images were transferred to a multi-functional image post-processing workstation (Syngo MMWP CT workplace VA30A) then downloaded as final images.
Pleural effusion and tumor foci measurement
All mice were sacrificed three days after the fourth time treatment. Before the pleural cavity opened, pleural effusion was gently aspirated and measured using a 1 mL syringe. Then the thoracic cavity of the mice was opened to further observe the distribution of tumor lesions on pleura. Tumors on both visceral and parietal pleural surfaces, as well as on pulmonary hilar and mediastinal lymph nodes were together counted. The counting process was independently done by two investigators (Wang and Yao) and discrepancies were resolved by consensus to get the final numbers.
Hematoxylin Eosin staining and cytological testing
Tumor loci on parietal pleura were obtained and fixed in 4% paraformaldehyde (PFA) for 16-24 hours and then incubated in 70% ethanol for another 3 days. The samples were embedded in paraffin and finally stained with hematoxylin-eosin.
For cytological testing, the pleural effusion from control group was centrifuged, the cells from the effusion were then smeared, fixed in methanol and stained with modified Wright’s Giemsa.
ELISA analysis
After centrifuged, the cell-free supernatants from pleural effusion were collected and stored at –80 °C. An ELISA was performed on the MPE supernatant, the concentration of VEGF-C was measured using commercial ELISA kits (R&D, USA) according to the manufactures protocol. The absorbance at 450 nm was determined using a microplate reader (Bio-Rad, Hercules, USA).
Immunohistochemistry (IHC) staining
Lymphangiogenesis was assessed by IHC staining. Tumors on parietal pleura were fixed in 4% PFA for 16-24 hours, dehydrated and then embedded in paraffin.
Consecutive paraffin slides were respectively stained for lymphatic micro vessel density (LMVD) by mouse monoclonal D2-40 antibody (1:200, Abcam) and VEGF-C expression by a polyclonal goat anti-mouse VEGF-C antibody (1:200, Abcam). The slides were incubated with primary antibody at 4 °C overnight. The slides were then stained using 3,3-diamino-benzidine (DAB, Vector Laboratories) and positive reaction was defined as a brown staining, following by hematoxylin staining of the nucleus.
LMVD was evaluated using D2-40 staining. The positive stained lymphatic micro vessels were quantitated at a 200× magnification, and the mean number of vessels in five different visions was defined as final LMVD. According to the previous studies (8), the staining intensity of VEGF-C expression was classified into four grades of scores as none [0], weak [1], moderate [2] and strong [3]. The percentage of positive cells in a vision at a 200× magnification was also stratified into four grades: 0% as 0, 1-10% as 1, 11-49% as 2, and 50-100% as 3. The final result was assessed as a combination of the intensity score and the percentage score. The scores from two independent investigators were compared, and inconsistencies were resolved by consensus. The independent scores from two investigators (Wang and Yao) were compared and discrepancies were resolved by consensus to get the final numbers.
Statistical analysis
SPSS 18.0 software was used to perform the statistical analyses. Data were expressed as the mean ± standard deviation and analyzed by a one-way analysis of variance (ANOVA). Dunn’s test was used to analyze the differences between two or multiple groups. Graphs were completed using GraphPad Prism version 5. All P values are two-tailed and P value <0.05 is considered to be statistically significant.
Results
Itraconazole can inhibit MPE and tumor formation
At 14 days following the intra-pleural tumor cell injection, CT scan demonstrated that pleural effusion in mice treated with H-β-C was more than that in the L-ITCZ group (Figure 1A,B). Compared to control group and L-ITCZ group, rare pleural effusion was observed in the H-ITCZ group, as shown in Figure 1C.
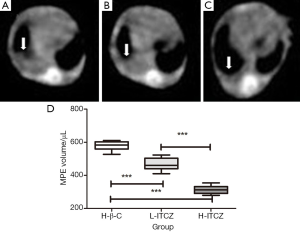
Pleural effusions from all three groups were confirmed to be hemorrhagic and not coagulated. As shown in Figure 1D, the mean volume of pleural effusion was 575.76±32.61 μL in the H-β-C control group, 445.64±27.75 μL in L-ITCZ group, and 311.19±27.20 μL in H-ITCZ group, respectively. The volume of pleural effusion in the L-ITCZ group was significantly different with that in control group (P<0.01). Similarly, the volume in the H-ITCZ group was significantly reduced when compared to both control group (P<0.01) and the L-ITCZ group (P<0.01).
As shown in Figure 2A-C, tumor foci on pleural surfaces and lymph nodes were decreased in L-ITCZ and H-ITCZ groups. The number of tumor foci in the control, L-ITCZ and H-ITCZ groups was 27.40±3.92, 21.40±3.37 and 11.55±3.28, respectively, and the number in the H-ITCZ group were significantly reduced than those in the control group (P<0.001) and the L-ITCZ (P<0.001) group (Figure 2D).
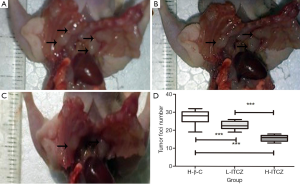
The presence of MPEs was revealed during the anatomy of the thoracic cavity (Figure 3A). Tumors on the parietal pleura of the H-β-C-treated mice were obtained and followed by histological staining for observation as control. The results of the staining confirmed the presence of adenocarcinoma cells in the tumors (Figure 3B). The cytological staining of the pleural effusion in the control group also demonstrated to be the tumor cells with large nuclei and nucleoli (Figure 3C).
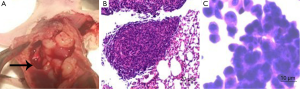
Itraconazole can inhibit VEFG-C expression in MPE
The expression level of VEGF-C in the MPE was evaluated by ELISA assay. As shown in Figure 4, compared to the control group, the expressions of VEGF-C were reduced in the supernatants of pleural effusions from L-ITCZ group and H-ITCZ group and the expression in MPE from the H-ITCZ group was significantly lower than those from both two other groups (P<0.001).
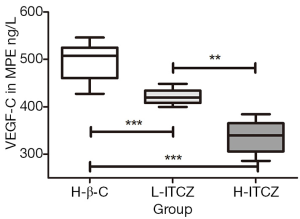
Itraconazole can suppress lymphangiogenesis in MPE
Since the positive staining was observed to be restricted to the cytoplasm without nuclear staining, LMVD and VEGF-C were detected in the stroma and the expression was mainly in the cancerous regions rather than normal tumor tissue.
The mean value of LMVD in the control, L-ITCZ and H-ITCZ groups was 24.1±2.51, 20.5±1.58 and 14.1±1.45, respectively (Figure 5A-C). LMVD was significantly decreased in the H-ITCZ-treated group than that in the control group (P<0.001) and the L-ITCZ group (P<0.001). Additionally, a significant difference of LMVD was revealed between the two other groups (P<0.01) (Figure 5D).
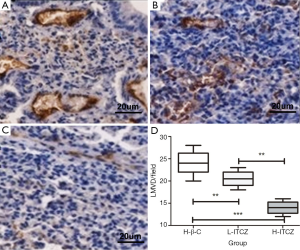
The percentage of VEGF-C expression was 65% in control group, 47% in L-ITCZ group and 36% in H-ITCZ group, respectively. The IHC staining for VEGF-C expression in all three groups is shown in Figure 6A-C. A significant decrease was observed in the H-ITCZ group compared to the control group (P<0.001) and L-ITCZ group (P<0.01), and the VEGF-C expressions in the two other groups were also significantly different (P<0.05) (Figure 6D).
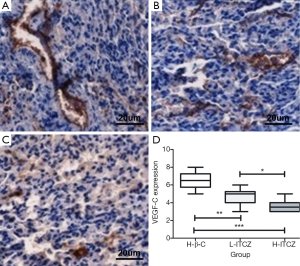
Discussion
Currently, MPE, a common complication in lung cancer patients, is an imminent problem for both patients and physicians (9). The patients with MPE often undergo disease-associated severe dyspnea and other complications which sharply decrease the quality of life and reduce survival time. The life expectancy may be improved only when the formation of MPE well controlled (3,10,11). A better understanding of the associated mechanisms involved in MPE is urgently needed for a curative treatment to present the possibility of prevention or inhibition of MPE (12).
Angiogenesis and lymphangiogenesis, the characteristics of cancer, are essential for the progression of neoplasm (13-16). Recent studies have reported that angiogenesis and lymphangiogenesis both participate in the development of MPE (17). Since lymphatic vessels have a larger lumen and increased permeability than blood vessels, lymphangiogenesis is thought to play a more crucial role in regional tumor lymph node metastasis and MPE (18-20). Therefore, as it provides approach for practical therapeutic intervention for MPE, the identification of the cytokines and cells that involved in the process of lymphangiogenesis is important (21,22). Vascular endothelial growth factors (VEGFs) and their receptors (VEGFRs) are at the center of the lymphangiogenesis signaling network (23), and increasing research demonstrated that VEGF-C acts as an important lymphatic-specific growth factor (24,25).
Itraconazole, an azole family member of antifungal drugs, inhibits the enzyme lanosterol 14α-demethylase which plays an important role in the conversion of lanosterol to ergosterol in fungi and lanosterol to cholesterol in humans (26). Research and clinical trials have proven that itraconazole suppresses cancer progression largely through inhibiting endothelial cell proliferation and angiogenesis (5,6). However, the mechanism of how Itraconazole suppressing endothelial cell proliferation has not been elucidated. Some research indicated that Itraconazole might inhibit cholesterol trafficking via antagonizing the hedgehog signaling pathway. There still is no systematic study on itraconazole in lymphangiogenesis and MPE (26-29).
To define the role of itraconazole in lymphangiogenesis, we established a mouse model of MPE and treated the mice with MPE using either itraconazole or placebo in the pleural cavity. The result suggested that the pleural effusion were notably decreased in the H-ITCZ group than that in the control group and L-ITCZ group, and MPE in the L-ITCZ group were less than that in control group. Tumor lesions on the pleura were also significantly reduced in the H-ITCZ group compared to the placebo and the L-ITCZ group, suggesting that itraconazole suppresses the generation of MPE in vivo. IHC staining for LMVD showed that lymphangiogenesis was decreased in the H-ITCZ group than that in other two groups, and VEGF-C expressions in both MPE and tumors were accordingly decreased in the H-ITCZ group. The results suggested that itraconazole had inhibited the expression of VEGF-C, which is proved to play an important part in lymphangiogenesis, and further suppressed the generation of MPE. Based on the above results, Itraconazole is identified in our study to block tumor lymphangiogenesis by inhibiting tumor VEGF-C expression in a dose-dependent manner, resulting in reduced MPE in vivo.
Besides the result on itraconazole inhibiting MPE, the MPE mouse model is also another advantage in our study. Other MPE mouse models often damage the skin, fascia, muscles or intercostal artery or present other problems (17). Based on previous experience, MPE mouse model in our present study was established by using a stereo microscope as a guide for the injections, reducing the complications to the lowest (8). In our study, lung cancer cells were visually injected into the pleural cavity, and other tissues were not easily injured. We also found that the skin wound resulting from injecting the LLC cells in the right chest wall of mice healed better without sutures (7,8).
Our study did have its shortcomings. As a treatment for MPE, drugs were injected into the pleural cavity in clinic after MPE was extracted. However, the extraction of the effusion was difficult in mice, making some differences between our experimental and clinical treatment. Additionally, there was certain difficulty to identify all the primary tumor lesions from the metastatic lymph nodes, therefore the tumor loci may be both tumors lesions and lymph nodes. However, taken these together, the reduced tumor loci still revealed the inhibitory effect of itraconazole on MPE formation.
Taken together, the results of our study substantiate the therapeutic effect of itraconazole on MPE in vivo, largely through inhibiting lymphangiogenesis in the generation and progression of MPE. The results of our study provide a theoretical basis for the effectiveness of itraconazole on MPE treatment, and further translational clinical studies are required for confirming these results.
Acknowledgements
We appreciate Chang-Sheng Zhou (Department of Radiology, Jinling Hospital, Nanjing 210002, China) for providing generous help with the CT scans. This study was supported by the Natural Science Fund of Jiangsu Province (BK2011658) to Yong Song.
Funding: This work was supported by grants from the National Natural Scientific Foundation of China (No. 81370172, No. 81302032, No. 81170064 and No. 81401903) and Clinical Science and Technology Project of Jiangsu Province (NO. BL2013026).
Disclosure: The authors declare no conflict of interest.
References
- Siegel R, Naishadham D, Jemal A. Cancer statistics, 2012. CA Cancer J Clin 2012;62:10-29. [PubMed]
- Hsu IL, Su WC, Yan JJ, et al. Angiogenetic biomarkers in non-small cell lung cancer with malignant pleural effusion: correlations with patient survival and pleural effusion control. Lung Cancer 2009;65:371-6. [PubMed]
- Basso SM, Mazza F, Marzano B, et al. Improved quality of life in patients with malignant pleural effusion following videoassisted thoracoscopic talc pleurodesis. Preliminary results. Anticancer Res 2012;32:5131-4. [PubMed]
- Aelony Y. Best current therapy for patients with malignant pleural effusion. Respiration 2013;85:13-4. [PubMed]
- Aftab BT, Dobromilskaya I, Liu JO, et al. Itraconazole inhibits angiogenesis and tumor growth in non-small cell lung cancer. Cancer Res 2011;71:6764-72. [PubMed]
- Chong CR, Xu J, Lu J, et al. Inhibition of angiogenesis by the antifungal drug itraconazole. ACS Chem Biol 2007;2:263-70. [PubMed]
- Ma X, Sun Y, Wang S, et al. Establishment of a malignant pleural effusion mouse model with Lewis lung carcinoma cell lines expressing enhanced green fluorescent protein. Zhongguo Fei Ai Za Zhi 2012;15:317-23. [PubMed]
- Ma X, Yao Y, Yuan D, et al. Recombinant human endostatin endostar suppresses angiogenesis and lymphangiogenesis of malignant pleural effusion in mice. PLoS One 2012;7:e53449. [PubMed]
- Lee YC, Light RW. Management of malignant pleural effusions. Respirology 2004;9:148-56. [PubMed]
- Fysh ET, Waterer GW, Kendall PA, et al. Indwelling pleural catheters reduce inpatient days over pleurodesis for malignant pleural effusion. Chest 2012;142:394-400. [PubMed]
- Maldonado F, Astoul P. Management of recurrent malignant pleural effusions: an ever-recurring issue? Chest 2012;142:1696; author reply 1696-7.
- Heffner JE. Management of the patient with a malignant pleural effusion. Semin Respir Crit Care Med 2010;31:723-33. [PubMed]
- Gomes FG, Nedel F, Alves AM, et al. Tumor angiogenesis and lymphangiogenesis: tumor/endothelial crosstalk and cellular/microenvironmental signaling mechanisms. Life Sci 2013;92:101-7. [PubMed]
- Dréanic J, Coriat R, Mir O, et al. Cervical extravasation of bevacizumab. Anticancer Drugs 2013;24:426-8. [PubMed]
- Evans T. Utility of hypertension as a surrogate marker for efficacy of antiangiogenic therapy in NSCLC. Anticancer Res 2012;32:4629-38. [PubMed]
- Gelibter AJ, Di Segni S, Zeuli M, et al. Bevacizumab: one treatment for all the seasons? J Clin Oncol 2008;26:511-author reply 511-2. [PubMed]
- Stathopoulos GT, Kalomenidis I. Malignant pleural effusion: tumor-host interactions unleashed. Am J Respir Crit Care Med 2012;186:487-92. [PubMed]
- Hirakawa S. From tumor lymphangiogenesis to lymphvascular niche. Cancer Sci 2009;100:983-9. [PubMed]
- Lv M, Mou Y, Wang P, et al. Diagnostic and predictive role of cell-free midkine in malignant pleural effusions. J Cancer Res Clin Oncol 2013;139:543-9. [PubMed]
- Santos GT, Prolla JC, Camillo ND, et al. Clinical and pathological factors influencing the survival of breast cancer patients with malignant pleural effusion. J Bras Pneumol 2012;38:487-93. [PubMed]
- Li T, Yang J, Zhou Q, et al. Molecular regulation of lymphangiogenesis in development and tumor microenvironment. Cancer Microenviron 2012;5:249-60. [PubMed]
- Tammela T, Alitalo K. Lymphangiogenesis: Molecular mechanisms and future promise. Cell 2010;140:460-76. [PubMed]
- Lohela M, Bry M, Tammela T, et al. VEGFs and receptors involved in angiogenesis versus lymphangiogenesis. Curr Opin Cell Biol 2009;21:154-65. [PubMed]
- Hirakawa S, Brown LF, Kodama S, et al. VEGF-C-induced lymphangiogenesis in sentinel lymph nodes promotes tumor metastasis to distant sites. Blood 2007;109:1010-7. [PubMed]
- Shin JW, Huggenberger R, Detmar M. Transcriptional profiling of VEGF-A and VEGF-C target genes in lymphatic endothelium reveals endothelial-specific molecule-1 as a novel mediator of lymphangiogenesis. Blood 2008;112:2318-26. [PubMed]
- Kim J, Tang JY, Gong R, et al. Itraconazole, a commonly used antifungal that inhibits Hedgehog pathway activity and cancer growth. Cancer Cell 2010;17:388-99. [PubMed]
- Kobayashi R, Suzuki D, Yasuda K, et al. Itraconazole for invasive fungal infection with pediatric malignancies. Pediatr Int 2010;52:707-10. [PubMed]
- Simon A, Besuden M, Vezmar S, et al. Itraconazole prophylaxis in pediatric cancer patients receiving conventional chemotherapy or autologous stem cell transplants. Support Care Cancer 2007;15:213-20. [PubMed]
- Takahashi N, Kameoka Y, Yamanaka Y, et al. Itraconazole oral solution enhanced vincristine neurotoxicity in five patients with malignant lymphoma. Intern Med 2008;47:651-3. [PubMed]