Phosphatidylinositol 3-kinase-AKT-mammalian target of rapamycin (PI3K-Akt-mTOR) signaling pathway in non-small cell lung cancer
Introduction
Lung cancer is the most common cancer and leading cause of cancer death worldwide, with an incidence of 1.6 million new cases annually and 1.38 million deaths in 2008 (1). It is the fifth most common cancer and the leading cause of cancer death in Australia. There are approximately 9,700 new cases of lung cancer diagnosed each year. In 2007, there were 7,626 deaths from lung cancer in Australia, accounting for 19% of all cancer deaths (2,3).
For the past two decades, decisions regarding lung cancer treatment have been based largely on the histological distinction between non-small cell lung cancer (NSCLC) and small cell lung carcinoma. In recent years, more definitive histological classifications as well as identification of somatic mutations have become an essential component in determining the management of NSCLC. Molecular driven therapeutic targets such as epidermal growth factor receptor (EGFR) gene mutations (4) and abnormal fusion of echinoderm microtubule-associated protein-like 4 and anaplastic lymphoma kinase (EML4-ALK) genes (5) have resulted in a paradigm shift in the treatment of advanced lung adenocarcinoma. However, they only account for a small proportion of NSCLC. Therefore, there are ongoing efforts in identifying more molecular targets for potential targeted therapeutics. EGFR mutation and abnormal fusion of EML4-ALK activate two main downstream signaling pathways, RAS-RAF-MEK-ERK and PI3K-Akt-mTOR, resulting in uncontrolled growth and cell proliferation. PI3K-Akt-mTOR is one of the most commonly deregulated pathways (6), which has been implicated in the tumourigenesis of NSCLC. Therefore, there has been an increasing research interest in identifying novel therapies to target this signaling pathway.
This review is going to provide an overview of the biology of PI3K-Akt-mTOR signaling pathway in normal cells under physiological conditions, mechanisms of deregulation of the pathway in NSCLC, therapeutic implications and potential prognostic and predictive biomarkers of the PI3K-Akt-mTOR signaling pathway.
Biology of PI3K-Akt-mTOR pathway
Key components of PI3K-Akt-mTOR pathway
PI3Ks are a family of lipid kinases that phosphorylate the 3’-hydroxol group in phosphatidylinositol and phosphoinositides (7). There are three classes of PI3K (I-III), which are classified according to their structure and substrate specificity (8). They are heterodimeric proteins with catalytic and regulatory subunits. Class I divides into Class IA and IB based on the types of receptors that they are activated by and they consist of different catalytic and regulatory subunits, each has different isoforms. Class IA PI3Ks consist of a p110 catalytic subunit and a p85 regulatory subunit. They are activated by growth factor receptor tyrosine kinases (RTKs). The p110 catalytic subunit has three isoforms (p110α, p110β and p110δ) that are encoded by PIK3CA, PIK3CB and PIK3CD genes respectively. The p85 regulatory subunit has five isoforms (p50α, p55α, p85α, p85β and p55γ). The p85α, p50α and p55α are encoded by PIK3R1 gene; p85β encodes for PIK3R2 gene and p55γ encodes for the PIK3R3 gene. Class IB PI3Ks consist of a p110γ catalytic subunit and a p101 regulatory subunit. They are activated by G-protein coupled receptors (GPCRs) (7,9). The role of Class IA PI3Ks in carcinogenesis has been well demonstrated but Class IB PI3Ks are less clear(10). Class II PI3Ks has only a single p110-like catalytic subunit that catalyze the production of PtdIns[3]P and PtdIns[3,4]P2 and regulate clathrin mediated membrane trafficking (8,11). Class III consists of a single member, hVPS34 which produces PtdIns[3]P and is involved in the regulation of vesicle trafficking, activation of mTOR by amino acids and autophagy (12,13).
Akt, is a serine/threonine-specific protein kinase and also known as protein kinase B (PKB). It consists of an amino-terminal pleckstrin homology (PH) domain, a central catalytic domain and a short carboxy-terminal regulatory domain. There are three isoforms: Akt 1 (PKBα), Akt 2 (PKBβ) and Akt 3 (PKBγ) (10). The function of Akt is to phosphorylate and activate or inactivate numerous downstream cytoplasmic and nuclear substrates such as forkhead (FOXO) family of transcription factors, p-53 binding protein (MDM2), pro-apoptotic protein BCL2-antagonitst of cell death (BAD) and tuberous sclerosis 1 and 2 (TSC1 & 2) to regulate cell survival, proliferation and protein synthesis, and hence cell growth (7,10).
The mammalian target of rapamycin (mTOR) is a serine-threonine kinase that is a member of the phosphatidylinositol kinase-related kinase (Pikk) family of kinases (9). It presents in two multi-protein complexes (mTORC1 and mTORC2). The mTORC1 is an mTOR complex combining rgulatory-associated protein of mTOR (Raptor), PRAS40 (also known as Akt substrate 1) and mLST8 while mTORC2 combines with rapamycin-insensitive companion of mTOR (Rictor), mSIN1, Protor and mLST8. It carries out its function of control on cell growth and division as well as protein translation through pathways of ribosomal p70 S6 kinase (p70S6K) and the eukaryotic translation initiation factor 4E (eIF4E) binding proteins (4E-BPs) (14).
PI3K-Akt-mTOR signaling in normal cells under physiological condition
Physiologically, the PI3K-Akt-mTOR pathway is activated by the binding of the ligand to the tyrosine kinase receptors (RTKs) such as EGFR, ErbB3, MET, PDGFR, VEGFR, IGF-1R, HER2/neu resulting in recruitment of class IA PI3Ks to the cell membrane where they convert phosphatidylinositol-4,5-bisphosphate (PIP2) to phosphatidylinositol-3,4,5-trisphosphate (PIP3). Simultaneously, the Ras-Raf-MAPK pathway may also be activated leading to cross-talk with PI3K. The PIP3 generated at the cell membrane acts as a second messenger, which binds to the PH domain of Akt. Akt is then phosphorylated at Thr308 in the catalytic domain by the phosphoinositide dependent kinase 1 (PDK1) and at Ser473 in the C-terminal hydrophobic motif by the mammalian target of rapamycin complex 2 (mTORC2). This results in full activation of Akt that lead to phosphorylation and inactivation of the complex of tuberous sclerosis 2 and 1 (TSC2-TSC1), which is a GTPase-activating protein (GAP) for Ras homologue enriched in brain (RHEB). ERK-Rsk also inactivates TSC2. Both results in accumulation of GTP-bound RHEB, leading to activation of the mammalian target of rapamycin complex 1 (mTORC1), which phosphorylates p70 S6 kinase and 4E-binding protein 1 (4EBP1), 4EBP2 and 4EBP3 resulting increased protein translation, ribosome biogenesis and inhibition of autophagy (15-19) (Figure 1).
Phosphorylation of Akt not only activates the mTOR signaling but it also activates minute double minute 2 (MDM2) and inactivates targets for pro-apoptotic proteins such as forkhead box O (FOXO) belong to forkhead family of transcription factors and BAD, which are involved in cell survival (Figure 1). Akt phosphorylates FOXO resulting in activation of pro-apoptotic proteins such as BIM and FAS ligand and expression of p27Kip1 and retinobloastoma-like 2 (RBL2) causing cell cycle arrest (20). There are a number of review articles that have elegantly illustrated and described this complex network of downstream substrates of Akt (7,8,10,14).
Feedback and regulation mechanisms of PI3K-Akt-mTOR signaling
In order to maintain cellular homeostasis, there are a number of different mechanisms that regulate the PI3K-Akt-mTOR signal transduction pathway preventing abnormal growth and cell division. Phosphatase and tensin homolog (PTEN) is the key component involved in deactivation of PI3K signaling by converting PIP3 back to PIP2 at the cell membrane (21) while the phosphatase PHLPP inactivates Akt signaling by dephosphorylating Akt at Ser 473 (22) (Figure 1). When it comes to the regulation of mTOR signaling, it is directly influenced by the activity of TSC2-TSC1 complex, which itself is controlled by the activity of TSC2-TSC1 complex, which itself is controlled by the cellular energy level via LKB1 (liver kinase b1)/STK11-AMPK (5’adenosine monophosphate-activated protein kinase) pathway (23), cellular oxygenation via hypoxia-inducible factor-α (HIFα) and DNA damage response 1 (REDD1) (24) and amino acid availability mediated by class III PI3K (25,26). Furthermore, there is an inhibitor protein, PRAS40 within the mTORC1 that exerts control on mTOR signaling. Carracedo et al. (27) illustrated that the feedback and regulation mechanisms of PI3K-Akt-mTOR are far more complex.
Deregulation of PI3K-Akt-mTOR signal transduction in NSCLC
Hanahan and Weinberg (28) described that tumourigenesis is the result of the ability of cancer cells to sustain proliferative signaling, evade growth suppressors, activate invasion and metastasis, enable replicative immortality, induce angiogenesis and resist cell death. Many studies have demonstrated that there is 50-70% overexpression of phosphorylated Akt in NSCLC indicating that abnormal activation of PI3K-Akt-mTOR pathway is a frequent event (29-31). Constitutive activation of PI3K-Akt-mTOR signaling pathway could be the result of genetic aberrations in any components of PI3K-Akt-mTOR pathway, its negative regulators, interconnected pathways and RTK signaling resulting in abnormal growth and cell proliferation. EGFR mutation and increased copy numbers, MET mutation and amplification and EML4-ALK rearrangement are examples of genetic changes that could result in abnormal RTK signaling. EGFR mutations are more prevalent in East Asian (up to 60%) than Caucasian patients (15-20%) (32-36). EML4-ALK rearrangement is much less prevalent (2-7%) and does not have an ethnicity predilection (37,38). However, both driver mutations are more common in patients who are non-smokers than smokers. At the PI3K-Akt-mTOR pathway, PIK3CA mutation and amplification, PTEN loss, Akt1 and LKB1 mutation are examples of genetic abnormalities involved in deregulation of signal transduction (6-8). Furthermore, Ras-Raf-MEK-ERK-Rsk pathway cross talks with PI3K-Akt-mTOR, therefore, KRAS mutations also play a role (39). Some of these genetic changes are more prevalent in certain histological subtypes of NSCLC than others. EGFR, KRAS mutations and EML4-ALK rearrangement are more common in adenocarcinoma than squamous cell carcinoma (32,33,35-38,40,41) while PIK3CA amplification is more common in squamous cell carcinoma than non-squamous cell carcinoma (42-48). PTEN loss is equally common in both NSCLC with squamous and non-squamous histology (33,49,50). In adenocarcinoma, EGFR, KRAS mutations and EML4-ALK rearrangement are mutually exclusive while KRAS and PIK3CA co-mutations are common (51). LKB1 mutations are more common in NSCLC with non-squamous cell histology than squamous and often present with EGFR and/or KRAS mutations (52-56).
Therapeutic implications
From the RTKs signaling to PI3K-Akt-mTOR pathway, these are targets of major therapeutics development that are either already in clinical practice such as epidermal growth factor tyrosine kinase inhibitors, EGFR TKIs (gefitinib, erlotinib and afatinib) and mTOR inhibitors (everolimus and temsirolimus) or in development such as dual PI3K/mTOR, PI3K and Akt inhibitors.
Targeted therapy—approved for clinical use
EGFR TKIs are now the first line treatment for EGFR mutated advanced NSCLC of non-squamous histology. In 2009, Mok et al. (36) demonstrated in the Iressa Pan-Asia Study (IPASS) that patients treated with efitinib whose pulmonary adenocarcinoma harbored EGFR mutation have a longer progression-free survival than those who were treated with carboplatin and paclitaxel (HR 0.48; 95% CI: 0.36-0.64; P<0.001) in East Asia. Subsequent clinical trials with the same design using erlotinib or afatinib including Asian or western population reached the same conclusion (57-59). Crizotinib, an oral tyrosine kinase inhibitor targeting ALK (60), MET (61) and ROS1 (62) has also been approved in advanced non-squamous cell lung carcinoma with ALK translocation. Shaw et al. (63) demonstrated that crizotinib improved both progression-free survival (PFS) (median 7.7 vs. 3 months; HR 0.49; P<0.001) and overall response rate (65% vs. 20%; P<0.001) in patients with ALK positive non-squamous cell lung carcinoma who progressed after platinum-doublet chemotherapy. Similar benefit when use in the first line setting among patients with ALK positive non-squamous cell lung carcinoma (64). mTOR inhibitors have been approved for clinical use as monotherapy in advanced renal cell carcinoma, pancreatic neuroendocrine tumour and in combination with examestane in estrogen receptor positive, HER2-negative breast cancer but not in advanced NSCLC because of poor response rate with increased toxicities. Soria et al. (65) showed that the overall response rate of everolimus monotherapy was less than 5% in patients with advanced NSCLC who progressed from chemotherapy. Ramalingam et al. (66) demonstrated that only 2 out of 28 (7%) patients had a partial response with the combination of everolimus with docetaxel while Besse et al. (67) failed to meet the predefined clinically meaningful treatment benefit threshold of 15% or greater with the combination of everolimus with erlotinib over erlotinib alone in the 3-month disease control rate but increased toxicities.
Targeted therapy—under development
Inhibitors targeting the key components of PI3K-Akt-mTOR pathway involving NSCLC are all undergoing either phase I or II therapeutic development. All these novel agents are administered orally. Their toxicities are unique to the components of the pathway that they are blocking but the common side effects include fatigue, anorexia, rash, hyperglycaemia, nausea and diarrhea (68).
PI3K inhibitors
Wortmannin and its derivative LY294002 were the first generation pan-PI3K inhibitors but they have not been moved into clinical use because of their toxicities. Pan-PI3K and isoform specific PI3K inhibitors are the two types of PI3K inhibitors that are in clinical development. Pan-PI3K inhibitors inhibit all isoform of class IA PI3K while isoform-specific PI3K inhibitors inhibit the specific isoforms of catalytic subunit of class IA PI3K (p110α, p110β and p110δ). BKM120 (Buparlisib), GDC-0941 (Pictilisib), PX-866 and XL-147 (SAR 245408) are examples of pan-PI3K inhibitors. BYI719 is an example of isoform-specific PI3K inhibitor.
Buparlisib (BKM120) is an oral pyrimidine-derived pan-PI3K inhibitor against both wild-type and mutant class I PI3Ks isoforms. It does not inhibit the class III PI3K or Mtor (69). The maximal tolerated dose has been established in phase I clinical trials with most common adverse events including rash, hyperglycaemia, anorexia, nausea and diarrhea and there was no ethnic difference in pharmacokinetics properties (70-72). It has advanced the furthest in the therapeutic development among all PI3K inhibitors as it is being evaluated in a randomised placebo-controlled phase III clinical trial in receptor positive but HER2 negative breast cancer (BKM120 + placebo versus BKM120 + fulvestrant) (73). However, buparlisib is still undergoing early phases of development in NSCLC.
Akt inhibitors
Their mode of action is to block the serine/threonine kinase Akt but their disturbance to the metabolic homeostasis causing severe hyperglycaemia and other potential metabolic abnormalities could hamper the development of this group of agents (74-76). MK-2206 is an example of a pan-Akt kinase inhibitor, which has been shown to potentiate the pathway inhibition when combined with systemic chemotherapy or molecularly targeted agents in preclinical study (77). Therefore, it is being evaluated in a phase II study in combination with erlotinib for patients who have advanced NSCLC after progression from erlotinib (NCT01294306).
Dual PI3K-mTOR inhibitors
SAR245409 and BEZ235 are two examples of dual PI3K-mTOR inhibitors. SAR245409 is a selective inhibitor of Class I PI3Ks, TORC1 and TORC2 (78) and BEZ235 is an imidazo-quinoline derivative blocking the activity of both PI3K and mTOR simultaneously (69,79). Both have been demonstrated to have anti-tumour effect in preclinical studies (80,81) and are being evaluated in early phases of clinical trials in combination with another targeted agents (69,78).
Trials in progress
All the novel agents targeting the PI3K-Akt-mTOR pathway mentioned above are still undergoing early phases of development in NSCLC. Hypotheses generated from the preclinical models, suggested that PI3K pathway inhibitors have the ability to overcome RTK resistance (77,82,83) and they have synergistic effect when combined with cytotoxic chemotherapy (42) or other targeted agents of interconnected pathways such as MEK inhibitors (69,84). Therefore, combination therapeutics approach would yield more success than monotherapy (85). Lockwood et al. (86) demonstrated in their genomic study that the genetic pathways involved in squamous cell lung carcinoma are different from those in non-squamous. NOTCH3 and FOXM1 are overexpressed in squamous cell lung carcinoma and may be involved in cross-talk with PI3K pathway (86-88). This highlighted the importance of histology. Therefore, not only do we need to evaluate markers by genetic alternations but also by histology subtype. Clinical trials in progress that evaluate the efficacy of PI3K pathway inhibitors in advanced NSCLC below include biomarker analysis by histology subtype (squamous cell carcinoma versus non-squamous cell carcinoma) and/or enriched with PIK3CA, PTEN gene mutated tumours.
PI3K pathway inhibitors + EGFR TKI
Ultimately, all patients with EGFR mutated lung adenocarcinoma who are on first generation EGFR TKIs (gefitinib and erlotinib), will eventually develop resistance and cancer progression. The mechanisms of resistance include acquired resistance mutation T790M, MET amplification or PIK3CA mutation resulting in activation of PI3K signaling. PIK3CA mutation account for up to 5% of EGFR-mutated NSCLC acquired resistance to EGFR TKI (89). Studies have demonstrated that adding PI3K inhibitors to EGFR TKIs can overcome the EGFR TKI resistant NSCLC cell lines (82,83,90,91). Tan et al. (92) demonstrated in a phase Ib study of 15 patients with EGFR mutant NSCLC who progressed during or after gefitinib that it was safe to combine buparlisib 80 mg with gefitinib 250 mg and there was antitumour activity but significant toxicities with 40% of patients experiencing delayed grade 3 rash and diarrhea. The median progression free survival was 2.8 (range, 2.3-8.1) months. Four out of nine patients in the group that included patients who progressed while on gefitinib had clinical responses including slight tumor shrinkage and reduced pleural effusion. Molecular analysis showed 6 (50%) of 12 patients whose tumors harbored T790M mutation, 2 (40%) of 5 had MET amplification while no patients whose tumor had PIK3CA mutation or PTEN loss. The dose expansion phase and alternate dose schedule are ongoing. Another similar phase Ib/II trial but in combination with erlotinib is currently recruiting (NCT01487265).
PI3K pathway inhibitors + chemotherapy
BASALT-1 (NCT01297491) is a two-stage phase II trial that included patients with metastatic NSCLC and PI3K pathway activation defined by PIK3CA mutation, PTEN mutation or loss of PTEN expression by immunohistochemistry. All patients in the first stage of the trial received buparlisib as a single agent and were stratified into squamous and non-squamous NSCLC subgroups. In the second stage, it was planned to randomise patients with squamous NSCLC to either buparlisib (100 mg/day) or docetaxel (75 mg/m2 every 3 weeks) while patients with non-squamous NSCLC randomise to either buparlisib (100 mg/day) or docetaxel (75 mg/m2) or pemetrexed (500 mg/m2) every three weeks. However, Soria et al. (93) demonstrated the progression free survival rate at 12 weeks was less than 50% for both the squamous and non-squamous NSCLC groups. Therefore, the second stage was not initiated because of futility. BASALT-2 (NCT01820325) is a phase Ib/II trial to determine the safety and efficacy of buparlisib in combination with carboplatin (area under the curve, AUC 6) and paclitaxel (200 mg/m2) every 3 weeks as first line treatment for patients with squamous NSCLC. NCT01911325 is another phase Ib/II trial that includes only squamous NSCLC.
There are other phase I or II trials using different chemotherapy regimens with buparlisib. Carboplatin with pemetrexed (NCT01723800 is recruiting) and cisplatin with gemcitabine are the two regimens used in two phase I trials and one has not opened yet (NCT01971489). Besse et al. (94) demonstrated in a phase Ib study that included patients with stage IIIB/IV NSCLC who failed first or second line chemotherapy that the overall response rate for squamous NSCLC patients was 75% (one complete responder and 2 partial responders) while non-squamous NSCLC eligible for bevacizumab was 66% (6 partial responders). There were no dose-limiting toxicities at 250 mg and the maximum dose for evaluation was at 330 mg. In the study, patients were stratified into two groups based on histology. For patients with squamous histology and the lesions centrally located or those with non-squamous histology and had contraindications to bevacizumab such as hypertension, haemoptysis or haematuria, pictilisib and carboplatin with paclitaxel were given for six cycles followed by maintenance pictilisib until progression (Arm A). For patients with non-squamous histology or squamous histology of peripherally locating lesions and no contraindications to bevacizumab, six cycles of carboplatin with paclitaxel and bevacizumab and pictilisib was given followed by maintenance bevacizumab with pictilisib (Arm B).
Prognostic and predictive biomarkers in NSCLC
Prognostic factor refers to patient or cancer characteristics that identify patients who are going to have a better or poorer survival regardless of treatment. They are validated in retrospective studies and are more important in early stage NSCLC because patients identified as high-risk may benefit from additional adjuvant therapies. Predictive factor refers to patient or cancer characteristics that identify patients who are more likely to respond to a particular treatment. They are validated in randomized controlled trials that can examine the predictive effect of the marker in both the placebo and treatment groups. They are more important in the advanced stage NSCLC because this allows delivery of personalised medicine to patients by maximizing benefit while minimizing potential harm. Genes, proteins, mRNAs and miRNAs are potential biomarkers, which can be discovered and evaluated using many techniques including immunohistochemistry, multi-gene profiling or next generations sequencing. With the advancement of molecular technology, we are increasingly more likely to classify and treat NSCLC according to their molecular genetic aberrations such as using EGFR TKIs and crizotinib in EGFR and ALK mutant NSCLC respectively. However, they only account for and benefit a small proportion of patients with advanced NSCLC. Furthermore, the clinical activity of novel targeted inhibitors targeting the PI3K-Akt-mTOR pathway, have been modest. Therefore, it would likely be cost ineffective to treat all patients with them. It is important to identify predictive biomarkers that could select patients who are more likely to respond to the novel targeted therapies than those who do not. To date, EGFR and ALK are the only predictive biomarkers in advanced NSCLC. There have been no predictive markers identified that correlate with clinical activity of novel targeted PI3K, Akt or dual PI3K/mTOR agents yet despite the effort of evaluating tumour molecular status in early phases of therapeutic clinical trials. In a preliminary analysis of a phase I buparlisib dose-escalation trial of 35 patients, over 70% of patients had adequate tumor to evaluate for PIK3CA status, PTEN expression and KRAS status (70). The study included only 2 patients (5.7%) with lung cancer and the majority of patients had colorectal (43%) or breast cancers (26%). There was one patient with triple-negative breast cancer who showed partial response while seven patients (20%) had stable disease of eight months or more. Five of them had tumors with PI3K pathway dependence. However, there were no correlation between tumor molecular alterations and clinical activity. Rodon et al. (71) reached the same conclusion at the final analysis of the full cohort of 83 patients and also among the subgroup of patients with breast or colorectal cancers. There were 43 patients in the expansion arm of the trial whose tumors harbored PIK3CA and/or PTEN mutations.
PIK3CA mutations or amplification and loss of PTEN are potential predictive markers of response because a preclinical study has demonstrated that squamous cell lung cancer cells lines with PIK3CA mutations or amplifications and loss of PTEN protein expression are more sensitive to pictilisib (42).
As for prognostic biomarkers, there have been many evaluated but none have been used in daily clinical practice. The overexpression of phosphorylated Akt (pAkt) using immunohistochemistry is a promising potential prognostic biomarker, which is expressed in both squamous and non-squamous cell lung carcinoma (95). Earlier studies (29,46,95-98) demonstrated that pAkt has no prognostic value while later studies suggested the contrary. David et al. (99) was the first to demonstrate that high level of pAkt expression correlated with shortened survival and was an independent prognostic factor. Subsequently, more studies further confirmed that overexpression of pAkt (Ser 473) assessed by immunohistochemistry is a negative prognostic factor in NSCLC (31,100-103) (Table 1). Interestingly, Shah et al. (105) demonstrated that expression of pAkt assessed by immunohistochemistry did not correlate with patient prognosis. However, high levels of pAkt protein assessed by Western blotting and semiquantitative densitometry correlated with a good prognosis in both univariate and multivariate analysis. This highlighted the difficulties in identifying validated prognostic markers that are applicable to clinical use because studies included patients with heterogeneous stages of disease, used different immunohistochemical protocols and pAkt antibodies against different phosphorylation sites (Ser 473 vs. Thr 308), while others had small sample sizes, which were underpowered to demonstrate prognostic significance.
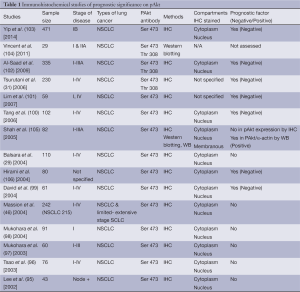
Full table
Conclusions
PI3K-Akt-mTOR signaling pathway is the target of many novel inhibitors. In order for patients to enjoy the maximal benefits and minimize side effects of these targeted therapies, identification of predictive markers are paramount. Incorporating biomarker analysis and patient enrichment strategies in clinical trials are essential in translational lung cancer research.
Acknowledgements
Disclosure: The author declares no conflict of interest.
References
- Cancer Incidence and Mortality Worldwide. International Agency for Research on Cancer. World Health Organization GLOBOCAN, 2013. Available online: http://globocan.iarc.fr
- Lung cancer-nonsmall cell. Cancer Council Australia, 2011. Available online: http://www.cancer.org.au/aboutcancer/cancertypes/lungcancernonsmallcell.htm
- Australian Institute of Health and Welfare & Australasian Association of Cancer Registries. Cancer in Australia: an overview 2012. Cancer series no. 74. Cat. no. CAN 70. AIHW, 2012.
- Lynch TJ, Bell DW, Sordella R, et al. Activating mutations in the epidermal growth factor receptor underlying responsiveness of non-small-cell lung cancer to gefitinib. N Engl J Med 2004;350:2129-39. [PubMed]
- Shaw AT, Yeap BY, Mino-Kenudson M, et al. Clinical features and outcome of patients with non-small-cell lung cancer who harbor EML4-ALK. J Clin Oncol 2009;27:4247-53. [PubMed]
- Papadimitrakopoulou V. Development of PI3K/AKT/mTOR pathway inhibitors and their application in personalized therapy for non-small-cell lung cancer. J Thorac Oncol 2012;7:1315-26. [PubMed]
- Engelman JA, Luo J, Cantley LC. The evolution of phosphatidylinositol 3-kinases as regulators of growth and metabolism. Nat Rev Genet 2006;7:606-19. [PubMed]
- Solomon B, Pearson RB. Class IA phosphatidylinositol 3-kinase signaling in non-small cell lung cancer. J Thorac Oncol 2009;4:787-91. [PubMed]
- Engelman JA. Targeting PI3K signalling in cancer: opportunities, challenges and limitations. Nat Rev Cancer 2009;9:550-62. [PubMed]
- Vivanco I, Sawyers CL. The phosphatidylinositol 3-Kinase AKT pathway in human cancer. Nat Rev Cancer 2002;2:489-501. [PubMed]
- Katso R, Okkenhaug K, Ahmadi K, et al. Cellular function of phosphoinositide 3-kinases: implications for development, homeostasis, and cancer. Annu Rev Cell Dev Biol 2001;17:615-75. [PubMed]
- Nobukuni T, Kozma SC, Thomas G. hvps34, an ancient player, enters a growing game: mTOR Complex1/S6K1 signaling. Curr Opin Cell Biol 2007;19:135-41. [PubMed]
- Gulati P, Gaspers LD, Dann SG, et al. Amino acids activate mTOR complex 1 via Ca2+/CaM signaling to hVps34. Cell Metab 2008;7:456-65. [PubMed]
- Papadimitrakopoulou V, Adjei AA. The Akt/mTOR and mitogen-activated protein kinase pathways in lung cancer therapy. J Thorac Oncol 2006;1:749-51. [PubMed]
- Sarbassov DD, Guertin DA, Ali SM, et al. Phosphorylation and regulation of Akt/PKB by the rictor-mTOR complex. Science 2005;307:1098-101. [PubMed]
- Cantley LC. The phosphoinositide 3-kinase pathway. Science 2002;296:1655-7. [PubMed]
- Shaw RJ, Cantley LC. Ras, PI(3)K and mTOR signalling controls tumour cell growth. Nature 2006;441:424-30. [PubMed]
- Bader AG, Kang S, Zhao L, et al. Oncogenic PI3K deregulates transcription and translation. Nat Rev Cancer 2005;5:921-9. [PubMed]
- Manning BD, Cantley LC. United at last: the tuberous sclerosis complex gene products connect the phosphoinositide 3-kinase/Akt pathway to mammalian target of rapamycin (mTOR) signalling. Biochem Soc Trans 2003;31:573-8. [PubMed]
- Accili D, Arden KC. FoxOs at the crossroads of cellular metabolism, differentiation, and transformation. Cell 2004;117:421-6. [PubMed]
- Keniry M, Parsons R. The role of PTEN signaling perturbations in cancer and in targeted therapy. Oncogene 2008;27:5477-85. [PubMed]
- Gao T, Furnari F, Newton AC. PHLPP: a phosphatase that directly dephosphorylates Akt, promotes apoptosis, and suppresses tumor growth. Mol Cell 2005;18:13-24. [PubMed]
- Shaw RJ, Bardeesy N, Manning BD, et al. The LKB1 tumor suppressor negatively regulates mTOR signaling. Cancer Cell 2004;6:91-9. [PubMed]
- Sofer A, Lei K, Johannessen CM, et al. Regulation of mTOR and cell growth in response to energy stress by REDD1. Mol Cell Biol 2005;25:5834-45. [PubMed]
- Byfield MP, Murray JT, Backer JM. hVps34 is a nutrient-regulated lipid kinase required for activation of p70 S6 kinase. J Biol Chem 2005;280:33076-82. [PubMed]
- Nobukuni T, Joaquin M, Roccio M, et al. Amino acids mediate mTOR/raptor signaling through activation of class 3 phosphatidylinositol 3OH-kinase. Proc Natl Acad Sci USA 2005;102:14238-43. [PubMed]
- Carracedo A, Ma L, Teruya-Feldstein J, et al. Inhibition of mTORC1 leads to MAPK pathway activation through a PI3K-dependent feedback loop in human cancer. J Clin Invest 2008;118:3065-74. [PubMed]
- Hanahan D, Weinberg RA. The hallmarks of cancer. Cell 2000;100:57-70. [PubMed]
- Balsara BR, Pei J, Mitsuuchi Y, et al. Frequent activation of AKT in non-small cell lung carcinomas and preneoplastic bronchial lesions. Carcinogenesis 2004;25:2053-9. [PubMed]
- Cappuzzo F, Ligorio C, Janne PA, et al. Prospective study of gefitinib in epidermal growth factor receptor fluorescence in situ hybridization positive/phospho-Akt positive or never smoker patients with advanced non-small-cell lung cancer: The ONCOBELL trial. J Clin Oncol 2007;25:2248-55. [PubMed]
- Tsurutani J, Fukuoka J, Tsurutani H, et al. Evaluation of Two Phosphorylation Sites Improves the Prognostic Significance of Akt Activation in Non-Small-Cell Lung Cancer Tumors. J Clin Oncol 2006;24:306-14. [PubMed]
- Eberhard DA, Johnson BE, Amler LC, et al. Mutations in the epidermal growth factor receptor and in KRAS are predictive and prognostic indicators in patients with non-small-cell lung cancer treated with chemotherapy alone and in combination with erlotinib. J Clin Oncol 2005;23:5900-9. [PubMed]
- O'Byrne KJ, Gatzemeier U, Bondarenko I, et al. Molecular biomarkers in non-small-cell lung cancer: a retrospective analysis of data from the phase 3 FLEX study. Lancet Oncol 2011;12:795-805. [PubMed]
- Shigematsu H, Lin L, Takahashi T, et al. Clinical and biological features associated with epidermal growth factor receptor gene mutations in lung cancers. J Natl Cancer Inst 2005;97:339-46. [PubMed]
- Zhu CQ, da Cunha Santos G, Ding K, et al. Role of KRAS and EGFR as biomarkers of response to erlotinib in National Cancer Institute of Canada Clinical Trials Group Study BR.21. J Clin Oncol 2008;26:4268-75. [PubMed]
- Mok TS, Wu YL, Thongprasert S, et al. Gefitinib or carboplatin-paclitaxel in pulmonary adenocarcinoma. N Engl J Med 2009;361:947-57. [PubMed]
- Soda M, Choi YL, Enomoto M, et al. Identification of the transforming EML4-ALK fusion gene in non-small-cell lung cancer. Nature 2007;448:561-6. [PubMed]
- Choi YL, Takeuchi K, Soda M, et al. Identification of novel isoforms of the EML4-ALK transforming gene in non-small cell lung cancer. Cancer Res 2008;68:4971-6. [PubMed]
- Carracedo A, Pandolfi PP. The PTEN-PI3K pathway: of feedbacks and cross-talks. Oncogene 2008;27:5527-41. [PubMed]
- Inamura K, Takeuchi K, Togashi Y, et al. EML4-ALK fusion is linked to histological characteristics in a subset of lung cancers. J Thorac Oncol 2008;3:13-7. [PubMed]
- Wong DW, Leung EL, So KK, et al. The EML4-ALK fusion gene is involved in various histologic types of lung cancers from nonsmokers with wild-type EGFR and KRAS. Cancer 2009;115:1723-33. [PubMed]
- Spoerke JM, O'Brien C, Huw L, et al. Phosphoinositide 3-kinase (PI3K) pathway alterations are associated with histologic subtypes and are predictive of sensitivity to PI3K inhibitors in lung cancer preclinical models. Clin Cancer Res 2012;18:6771-83. [PubMed]
- Okudela K, Suzuki M, Kageyama S, et al. PIK3CA mutation and amplification in human lung cancer. Pathol Int 2007;57:664-71. [PubMed]
- Yamamoto H, Shigematsu H, Nomura M, et al. PIK3CA mutations and copy number gains in human lung cancers. Cancer Res 2008;68:6913-21. [PubMed]
- Kawano O, Sasaki H, Okuda K, et al. PIK3CA gene amplification in Japanese non-small cell lung cancer. Lung Cancer 2007;58:159-60. [PubMed]
- Massion PP, Taflan PM, Shyr Y, et al. Early involvement of the phosphatidylinositol 3-kinase/Akt pathway in lung cancer progression. American journal of respiratory and critical care medicine 2004;170:1088-94. [PubMed]
- Angulo B, Suarez-Gauthier A, Lopez-Rios F, et al. Expression signatures in lung cancer reveal a profile for EGFR-mutant tumours and identify selective PIK3CA overexpression by gene amplification. J Pathol 2008;214:347-56. [PubMed]
- Massion PP, Kuo WL, Stokoe D, et al. Genomic copy number analysis of non-small cell lung cancer using array comparative genomic hybridization: implications of the phosphatidylinositol 3-kinase pathway. Cancer Res 2002;62:3636-40. [PubMed]
- Marsit CJ, Zheng S, Aldape K, et al. PTEN expression in non-small-cell lung cancer: evaluating its relation to tumor characteristics, allelic loss, and epigenetic alteration. Hum Pathol 2005;36:768-76. [PubMed]
- Soria JC, Lee HY, Lee JI, et al. Lack of PTEN expression in non-small cell lung cancer could be related to promoter methylation. Clin Cancer Res 2002;8:1178-84. [PubMed]
- Chaft JE, Arcila ME, Paik PK, et al. Coexistence of PIK3CA and other oncogene mutations in lung adenocarcinoma-rationale for comprehensive mutation profiling. Mol Cancer Ther 2012;11:485-91. [PubMed]
- Gao B, Sun Y, Zhang J, et al. Spectrum of LKB1, EGFR, and KRAS mutations in chinese lung adenocarcinomas. J Thorac Oncol 2010;5:1130-5. [PubMed]
- Onozato R, Kosaka T, Achiwa H, et al. LKB1 gene mutations in Japanese lung cancer patients. Cancer Sci 2007;98:1747-51. [PubMed]
- Sanchez-Cespedes M, Parrella P, Esteller M, et al. Inactivation of LKB1/STK11 is a common event in adenocarcinomas of the lung. Cancer Res 2002;62:3659-62. [PubMed]
- Matsumoto S, Iwakawa R, Takahashi K, et al. Prevalence and specificity of LKB1 genetic alterations in lung cancers. Oncogene 2007;26:5911-8. [PubMed]
- Koivunen JP, Kim J, Lee J, et al. Mutations in the LKB1 tumour suppressor are frequently detected in tumours from Caucasian but not Asian lung cancer patients. Br J Cancer 2008;99:245-52. [PubMed]
- Rosell R, Carcereny E, Gervais R, et al. Erlotinib versus standard chemotherapy as first-line treatment for European patients with advanced EGFR mutation-positive non-small-cell lung cancer (EURTAC): a multicentre, open-label, randomised phase 3 trial. Lancet Oncol 2012;13:239-46. [PubMed]
- Zhou C, Wu YL, Chen G, et al. Erlotinib versus chemotherapy as first-line treatment for patients with advanced EGFR mutation-positive non-small-cell lung cancer (OPTIMAL, CTONG-0802): a multicentre, open-label, randomised, phase 3 study. Lancet Oncol 2011;12:735-42. [PubMed]
- Sequist LV, Yang JC, Yamamoto N, et al. Phase III study of afatinib or cisplatin plus pemetrexed in patients with metastatic lung adenocarcinoma with EGFR mutations. J Clin Oncol 2013;31:3327-34. [PubMed]
- Kwak EL, Bang YJ, Camidge DR, et al. Anaplastic lymphoma kinase inhibition in non-small-cell lung cancer. N Engl J Med 2010;363:1693-703. [PubMed]
- Ou SH, Bazhenova L, Camidge DR, et al. Rapid and dramatic radiographic and clinical response to an ALK inhibitor (crizotinib, PF02341066) in an ALK translocation-positive patient with non-small cell lung cancer. J Thorac Oncol 2010;5:2044-6. [PubMed]
- Bergethon K, Shaw AT, Ou SH, et al. ROS1 rearrangements define a unique molecular class of lung cancers. J Clin Oncol 2012;30:863-70. [PubMed]
- Shaw AT, Kim DW, Nakagawa K, et al. Crizotinib versus chemotherapy in advanced ALK-positive lung cancer. N Engl J Med 2013;368:2385-94. [PubMed]
- Mok T, Kim D, Wu Y, et al. First-line crizotinib versus pemetrexed-cisplatin or pemetrexed-carboplatin in patients (pts) with advanced ALK-positive non-squamous non-small cell lung cancer (NSCLC): results of a phase III study (PROFILE 1014). J Clin Oncol 2014;32:5s(suppl; abstr 8002).
- Soria JC, Shepherd FA, Douillard JY, et al. Efficacy of everolimus (RAD001) in patients with advanced NSCLC previously treated with chemotherapy alone or with chemotherapy and EGFR inhibitors. Ann Oncol 2009;20:1674-81. [PubMed]
- Ramalingam SS, Owonikoko TK, Behera M, et al. Phase II study of docetaxel in combination with everolimus for second- or third-line therapy of advanced non-small-cell lung cancer. J Thorac Oncol 2013;8:369-72. [PubMed]
- Besse B, Leighl N, Bennouna J, et al. Phase II study of everolimus-erlotinib in previously treated patients with advanced non-small-cell lung cancer. Ann Oncol 2014;25:409-15. [PubMed]
- Beck JT, Ismail A, Tolomeo C. Targeting the phosphatidylinositol 3-kinase (PI3K)/AKT/mammalian target of rapamycin (mTOR) pathway: an emerging treatment strategy for squamous cell lung carcinoma. Cancer Treat Rev 2014;40:980-9. [PubMed]
- Maira SM, Pecchi S, Huang A, et al. Identification and characterization of NVP-BKM120, an orally available pan-class I PI3-kinase inhibitor. Mol Cancer Ther 2012;11:317-28. [PubMed]
- Bendell JC, Rodon J, Burris HA, et al. Phase I, dose-escalation study of BKM120, an oral pan-Class I PI3K inhibitor, in patients with advanced solid tumors. J Clin Oncol 2012;30:282-90. [PubMed]
- Rodon J, Brana I, Siu LL, et al. Phase I dose-escalation and -expansion study of buparlisib (BKM120), an oral pan-Class I PI3K inhibitor, in patients with advanced solid tumors. Invest New Drugs 2014;32:670-81. [PubMed]
- Ando Y, Inada-Inoue M, Mitsuma A, et al. Phase I dose-escalation study of buparlisib (BKM120), an oral pan-class I PI3K inhibitor, in Japanese patients with advanced solid tumors. Cancer Sci 2014;105:347-53. [PubMed]
- Iwata H, Baselga J, Campone M, et al. Ph III randomized studies of the oral pan-PI3K inhibitor buparlisib (BKM120) with fulvestrant in postmenopausal women with HR+/HER2– locally advanced or metastatic breast cancer (BC) after aromatase inhibitor (AI; BELLE-2) or AI and mTOR inhibitor (BELLE-3) treatment. J Clin Oncol 2013;31(suppl; abstr TPS650).
- Cherrin C, Haskell K, Howell B, et al. An allosteric Akt inhibitor effectively blocks Akt signaling and tumor growth with only transient effects on glucose and insulin levels in vivo. Cancer Biol Ther 2010;9:493-503. [PubMed]
- Crouthamel MC, Kahana JA, Korenchuk S, et al. Mechanism and management of AKT inhibitor-induced hyperglycemia. Clin Cancer Res 2009;15:217-25. [PubMed]
- Luo Y, Shoemaker AR, Liu X, et al. Potent and selective inhibitors of Akt kinases slow the progress of tumors in vivo. Mol Cancer Ther 2005;4:977-86. [PubMed]
- Hirai H, Sootome H, Nakatsuru Y, et al. MK-2206, an allosteric Akt inhibitor, enhances antitumor efficacy by standard chemotherapeutic agents or molecular targeted drugs in vitro and in vivo. Mol Cancer Ther 2010;9:1956-67. [PubMed]
- Jänne PA, Cohen RB, Laird AD, et al. Phase I safety and pharmacokinetic study of the PI3K/mTOR inhibitor SAR245409 (XL765) in combination with erlotinib in patients with advanced solid tumors. J Thorac Oncol 2014;9:316-23. [PubMed]
- Stauffer F, Maira SM, Furet P, Garcia-Echeverria C. Imidazo[4,5-c]quinolines as inhibitors of the PI3K/PKB-pathway. Bioorg Med Chem Lett 2008;18:1027-30. [PubMed]
- Fokas E, Im JH, Hill S, et al. Dual inhibition of the PI3K/mTOR pathway increases tumor radiosensitivity by normalizing tumor vasculature. Cancer Res 2012;72:239-48. [PubMed]
- Yu P, Laird AD, Du X, et al. Characterization of the activity of the PI3K/mTOR inhibitor XL765 (SAR245409) in tumor models with diverse genetic alterations affecting the PI3K pathway. Mol Cancer Ther 2014;13:1078-91. [PubMed]
- Ihle NT, Paine-Murrieta G, Berggren MI, et al. The phosphatidylinositol-3-kinase inhibitor PX-866 overcomes resistance to the epidermal growth factor receptor inhibitor gefitinib in A-549 human non-small cell lung cancer xenografts. Mol Cancer Ther 2005;4:1349-57. [PubMed]
- Janmaat ML, Rodriguez JA, Gallegos-Ruiz M, Kruyt FA, Giaccone G. Enhanced cytotoxicity induced by gefitinib and specific inhibitors of the Ras or phosphatidyl inositol-3 kinase pathways in non-small cell lung cancer cells. Int J Cancer 2006;118:209-14. [PubMed]
- Miller TW, Balko JM, Fox EM, et al. ERalpha-dependent E2F transcription can mediate resistance to estrogen deprivation in human breast cancer. Cancer Discov 2011;1:338-51. [PubMed]
- Belani CP, Goss G, Blumenschein G Jr. Recent clinical developments and rationale for combining targeted agents in non-small cell lung cancer (NSCLC). Cancer Treat Rev 2012;38:173-84. [PubMed]
- Lockwood WW, Wilson IM, Coe BP, et al. Divergent genomic and epigenomic landscapes of lung cancer subtypes underscore the selection of different oncogenic pathways during tumor development. PLoS One 2012;7:e37775. [PubMed]
- Gutierrez A, Look AT. NOTCH and PI3K-AKT pathways intertwined. Cancer Cell 2007;12:411-3. [PubMed]
- Park HJ, Carr JR, Wang Z, et al. FoxM1, a critical regulator of oxidative stress during oncogenesis. EMBO J 2009;28:2908-18. [PubMed]
- Kawano O, Sasaki H, Endo K, et al. PIK3CA mutation status in Japanese lung cancer patients. Lung Cancer 2006;54:209-15. [PubMed]
- Kim SM, Kim JS, Kim JH, et al. Acquired resistance to cetuximab is mediated by increased PTEN instability and leads cross-resistance to gefitinib in HCC827 NSCLC cells. Cancer Lett 2010;296:150-9. [PubMed]
- Donev IS, Wang W, Yamada T, et al. Transient PI3K inhibition induces apoptosis and overcomes HGF-mediated resistance to EGFR-TKIs in EGFR mutant lung cancer. Clin Cancer Res 2011;17:2260-9. [PubMed]
- Tan DS, Lim KH, Tai WM, et al. A phase Ib safety and tolerability study of a pan class I PI3K inhibitor buparlisib (BKM120) and gefitinib (gef) in EGFR TKI-resistant NSCLC. J Clin Oncol 2013;31:(suppl; abstr 8107).
- Soria JC, Vansteenkiste JF, Canon J, et al. Buparlisib (BKM120) in patients with PI3K pathway-activated, metastatic non-small cell lung cancer (NSCLC): results from the BASALT-1 study. Ann Oncol 2014;25:suppl 4.
- Besse B, Soria J, Gomez-Roca C, et al. A phase Ib study to evaluate the PI3-kinase inhibitor GDC-0941 with paclitaxel (P) and carboplatin (C), with and without bevacizumab (BEV), in patients with advanced non-small cell lung cancer (NSCLC). J Clin Oncol 2011;29:(suppl; abstr 3044).
- Lee SH, Kim H, Park W, et al. Non-small cell lung cancers frequently express phosphorylated Akt; an immunohistochemical study. Apmis 2002;110:587-92. [PubMed]
- Tsao AS, McDonnell T, Lam S, et al. Increased Phospho-AKT (Ser473) Expression in Bronchial Dysplasia Implications for Lung Cancer Prevention Studies. Cancer Epidemiol Biomarkers & Prev 2003;12:660-4. [PubMed]
- Mukohara T, Kudoh S, Yamauchi S, et al. Expression of epidermal growth factor receptor (EGFR) and downstream-activated peptides in surgically excised non-small-cell lung cancer (NSCLC). Lung Cancer 2003;41:123-30. [PubMed]
- Mukohara T, Kudoh S, Matsuura K, et al. Activated Akt expression has significant correlation with EGFR and TGF-alpha expressions in stage I NSCLC. Anticancer Res 2004;24:11-7. [PubMed]
- David O, Jett J, LeBeau H, et al. Phospho-Akt overexpression in non‚small cell lung cancer confers significant stage-independent survival disadvantage. Clin Cancer Res 2004;10:6865-71. [PubMed]
- Tang JM, He QY, Guo RX, et al. Phosphorylated Akt overexpression and loss of PTEN expression in non-small cell lung cancer confers poor prognosis. Lung cancer 2006;51:181-91. [PubMed]
- Lim WT, Zhang W, Miller C, et al. PTEN and phosphorylated AKT expression and prognosis in early-and late-stage non-small cell lung cancer. Oncol Rep 2007;17:853. [PubMed]
- Al-Saad S, Donnem T, Al-Shibli K, et al. Diverse prognostic roles of Akt isoforms, PTEN and PI3K in tumor epithelial cells and stromal compartment in non-small cell lung cancer. Anticancer Res 2009;29:4175-83. [PubMed]
- Yip PY, Cooper WA, Kohonen-Corish MR, et al. Phosphorylated Akt expression is a prognostic marker in early-stage non-small cell lung cancer. J Clin Pathol 2014;67:333-40. [PubMed]
- Vincent EE, Elder D, Thomas E, et al. Akt phosphorylation on Thr308 but not on Ser473 correlates with Akt protein kinase activity in human non-small cell lung cancer. Br J Cancer 2011;104:1755-61. [PubMed]
- Shah A, Swain WA, Richardson D, et al. Phospho-Akt Expression Is Associated with a Favorable Outcome in Non Small Cell Lung Cancer. Clin Cancer Res 2005;11:2930-6. [PubMed]
- Hirami Y, Aoe M, Tsukuda K, et al. Relation of epidermal growth factor receptor, phosphorylated-Akt, and hypoxia-inducible factor-1alpha in non-small cell lung cancers. Cancer Lett 2004;214:157-64. [PubMed]