Implementing lung cancer screening in the real world: opportunity, challenges and solutions
Lung cancer is a devastating disease, with the majority of patients diagnosed in advanced stages, resulting in an overall 5-year survival of only 18% (1). National Cancer Institute (NCI) data for non-small cell lung cancer in the United States (US) for the period from 2005 through 2011 showed that only 16% of lung cancers are diagnosed at a localized stage, and the majority, 57%, is diagnosed with distant disease (2). The goal of screening for lung cancer is to reduce lung cancer mortality by increasing the number of cases diagnosed at a localized stage, thereby allowing a curative approach to treatment. In 2011, the largest randomized controlled trial of CT screening for lung cancer to date, the National Lung Screening Trial (NLST), changed the face of early detection of lung cancer when it reported a 20% reduction in lung-cancer specific mortality in a high- risk cohort of patients screened in the US with CT in comparison with the control arm of high-risk individuals screened with annual chest radiographs (CXR) for 3 years (3). In contrast to symptom-detected lung cancers, the majority (63%) of CT-screen-detected lung cancers was Stage I, and only 12.8% with a positive screen had distant disease. The optimistic approach to these results is that CT screening could shift the lung cancer population from one dominated by advanced, incurable disease to a population with a high percentage of early stage, resectable disease. On the other hand, it is yet to be shown if the NLST results can be generalized either to the community setting within the US or to countries outside the US (4). In this article, we will explore the issues driving the lung cancer screening debate.
Prior attempts at lung cancer screening
In the 1970s, there was a large scale effort, funded by the NCI, to examine the role of sputum cytology in addition to chest radiography in screening for lung cancer. Three institutions, the Mayo Clinic in Rochester, Minnesota, the Johns Hopkins Medical Institution in Baltimore, Maryland, and the Memorial Sloan-Kettering Cancer Center (MSKCC) in New York City accrued approximately 30,000 adult male smokers (5-8). The trial designs at Johns Hopkins and MSKCC were identical—patients were randomized to either a single screen (CXR annually) or a dual screen (CXR annually, sputum cytology every 4 months). This trial design specifically addressed the role of sputum cytology as all participants underwent annual CXR screening, and eventually determined that there was no mortality benefit from the addition of sputum cytology. In the Mayo Lung Project (MLP), participants were randomized to an experimental arm in which participants were offered (and encouraged) free CXR and free sputum cytology to be obtained every 4 months, or to a control arm in which participants were simply advised to undergo these tests annually (9). The early results were encouraging. After 6 years, there were more lung cancers diagnosed in the screened group, and the screen-detected lung cancers were more likely to be resectable, and in Stage I or II, in comparison with a group of clinical cases of lung cancer seen at the Mayo Clinic (7). There were, however, slightly more lung cancer deaths in the experimental arm than in the control arm, and consequently no reduction in lung cancer mortality with CXR screening (10).
In 1993, the NCI initiated the Prostate, Lung, Colon and Ovarian (PLCO) Cancer Screening trial to determine if screening could reduce mortality from these four cancers (11). The design of the lung component of the PLCO differed from the earlier NCI trials in several areas: (i) it included women and never-smokers; (ii) the control arm did not include a CXR; and (iii) the sample size was much larger. A total of 154,934 participants were enrolled between 1993 and 2001. After 13 years of follow-up, it was reported that screening with CXR did not reduce lung-cancer specific mortality (12). There were 1,213 lung cancer deaths in the CXR-screened group and 1,230 lung cancer deaths in the control group, for a relative risk of lung cancer mortality of 0.99 (95% CI, 0.87-1.22). In a subset of participants who also met NLST eligibility criteria, the relative risk of lung cancer mortality in the CXR arm over the same 6-year follow-up period was 0.94 (95% CI, 0.81-1.10).
The introduction of CT screening
As CT technology evolved from single slice scanners to multi-slice scanners, the thorax could now be imaged with high spatial resolution in a single breath-hold (13). This technological advance spurred a renewed interest in imaging-based lung cancer screening. In the early 1990s, single-arm trials of low-dose CT screening for lung cancer began in Japan and in the US. Masahiro Kaneko and his colleagues at the National Cancer Center Hospital in Japan performed low-dose spiral CT for the purposes of lung cancer screening in 1,369 individuals, between September 1993 and April 1995 (14). They identified 15 peripheral lung cancers, 14 of which were Stage I, and 11 of which were missed on CXR. Simultaneously, Claudia Henschke and her colleagues at Cornell and New York University initiated the Early Lung Cancer Action Project (ELCAP), scanning 1,000 individuals who were ages 60 and older, with at least a 10 pack-year smoking history (15). In the nodules identified at CT, 27 proved to be malignant, and 23 of those were Stage I disease. They also diagnosed malignancy in four additional participants, two with endobronchial disease and two with mediastinal disease. As in Japan, many (20 of 27) nodules representing lung cancer were not detected by CXR.
These single-arm trials suggested that low-dose CT screening for lung cancer might achieve what CXR screening had not, not only a stage shift towards earlier, resectable disease, but also a reduction in lung cancer mortality. Proving that would require randomized controlled trials.
The National Lung Screening Trial (NLST)
The NLST opened in the US in 2002, enrolling 53,454 participants between September 2002 and April 2004. Eligibility criteria included ages 55 to 74, and current or former (quit within the last 15 years) smoker with at least 30 pack-year smoking history. Exclusion criteria included a history of lung cancer, treatment for any prior malignancy other than non-melanoma skin cancer within the last 5 years, present symptoms suggestive of lung cancer, requirement for home oxygen supplementation, and any medical condition that would pose a significant risk of mortality within the proposed 8-year trial period. The experimental arm received screening with low-dose CT annually for 3 years, and the control arm received screening with single view CXR. Although the control arm would have ideally been no screening (standard of care), including the CXR in the control arm facilitated accrual and retention, and the concurrently running PLCO trial would allow comparison between three arms—the CT arm, the CXR arm, and no screening.
In 2011, the NLST reported a 20% reduction in lung cancer mortality in heavy smokers screened with three annual low-dose computed tomography (LDCT) scans relative to those screened with three annual single view CXR (3). This was a milestone not only for lung cancer screening, as the first randomized controlled trial to demonstrate this benefit, but also for the diagnosis and treatment of lung cancer in general. The results have been met with cautious optimism, however, as it has become clear that the 20% mortality reduction was in a highly selected cohort, following a specific protocol, predominantly at large academic institutions.
European randomized lung cancer screening trials
In 2010, the principal investigators of the six on-going European randomized controlled trials of lung cancer screening and of the one trial in preparation in the United Kingdom began to pool information regarding the epidemiological, radiological, and nodule management aspects of their trials, creating the European Randomized Lung Cancer CT Screening Trials (EUCT) (16). These trials included the Nederlands-Leuvens Longkanker Screenings Onderzoek (NELSON) (17), the Danish Lung Cancer Screening Trial (DLCST) (18), the Multi-centric Italian Lung Detection Trial (MILD) (19), the Italian Lung cancer Computed Tomography screening trial (ITALUNG) (20), the Detection and screening of early lung cancer by Novel imaging TEchnology and molecular assays (DANTE) (21), the German Lung Cancer Screening Intervention Study (LUSI) (22), and the United Kingdom Lung Cancer Screening Trial (UKLS) (23).
Although there are slight variations in the individual study designs, all seven trials have a control arm of no screening, and inclusion criteria of heavy current or former smokers. The number of screening rounds varied, from one (UKLS) to ten (MILD); the screening interval ranged from 1 to 2.5 years; three-dimensional volume measurement was included in most trials, but two-dimensional nodule measurements only were utilized in two (DANTE, ITALUNG) (24). The lower age limit was 50 in most trials, with the exception of ITALUNG and DANTE, with lower age limits of 55 and 60, respectively.
The combined EUCT trials have a higher percentage of male participants than the NLST, in which 59% of the participants were male (25). In the largest EUCT trial, the NELSON trial, with 15,822 participants, 84% were male. Of the six EUCT trials with completed enrollment, 25,902 of 34,094 (76%) participants were male. The higher percentage of male participants in the EUCT has potential implications for the reduction of lung cancer mortality. In the NLST, Pinsky et al. calculated the overall lung cancer mortality risk ratio (RR), defined as the lung cancer death rate in the CT versus CXR arms, at 0.84 (95% CI, 0.75-0.95). There was a slight difference in the RR for women versus men, however, with a greater reduction in lung cancer mortality risk in women (RR of 0.73 vs. 0.92) (26). If this sex-linked difference in mortality is also seen in the EUCT, the overall mortality reduction would be impacted by a greater percentage of male participants.
The UKLS, with its planned enrollment of 32,000 individuals, could ultimately dominate the profile of the EUCT participants. The UKLS uses the Liverpool Lung Project (LLP) risk prediction model (27) to determine an individual’s lung cancer risk, and only those with at least a 5% risk of developing lung cancer within the next 5 years are invited to participate in the trial (23,27). In the first recruitment phase of the trial, men responding to the recruitment questionnaire were more than twice as likely as women to be considered high risk by the LLP model; 2,016 (17%) of male and 832(7%) of female positive responders were deemed high risk (28).
Potential impact of screening on stage distribution and mortality
When a patient first begins a CT screening program for lung cancer, a diagnosed lung cancer may be in advanced stages even though the patient is asymptomatic. A better estimate of the potential for CT screening to generate a stage shift in lung cancer is the percentage of lung cancers in early stages at subsequent annual screens. In the CT arm of the NLST, 87/183 (47.5%) of lung cancers were stage IA and 57/183 (31.1%) were stage III or IV in the low-dose CT group at the second annual (T1) screen, among lung cancers of known stage (29). In comparison with the initial T0 screen the percentage of stage IA lung cancers was slightly higher, and the percentage of Stage III lung cancers was slightly lower (30) (Table 1). This was also observed in the NELSON trial, with 74.1% of lung cancers in stage IA in the second screening round and 64.9% in stage IA at the third screening round, as compared with 59.5% of lung cancers in stage IA in the first screening round (31). Although a stage shift suggests that screening will identify more surgically resectable cancers, it is not a substitute for the true measure of screening efficacy, which is a reduction in lung cancer mortality in comparison with a control arm.

Full table
Lung cancer screening has the potential to impact lung cancer mortality on a global level. The World Health Organization estimates that there were 1,824,701 new cases and 1,589,925 deaths from lung cancer worldwide in 2012 (32). If all those lung cancers could be identified through screening, a 20% reduction in lung cancer mortality could mean 317,985 fewer deaths from lung cancer world-wide each year. Many of those cancers occur, however, in individuals who do not meet current eligibility criteria for lung cancer screening, either because of age or smoking history. If the NLST eligibility criteria (age 55-74, at least 30 pack-year smoking history, former smokers quit within the last 15 years) were applied to the US population, only 26.7% of lung cancers would be identified (33). One of the reasons for this relatively low percentage of clinical cases of lung cancer is the age criterion: only 53.3% of cases of lung cancer in the US occur in patients 55 to 74; 27.6% of all lung cancers in the US occur in individuals 75 to 84 years old and an additional 8.4% are in the age group 45 to 54 (2). If the NLST eligibility criteria were revised to include age 50-79 and a 30+ pack-year history (any quit status), 46.3% of lung cancers would be included, but the percentage of the US population that would be eligible for screening would double, from 6.2% of the population over age 40 to 12.1%.
The NLST eligibility criterion of 30 pack-years, which reflects the total cigarette smoking exposure, does not distinguish between those who have smoked fewer cigarettes per day (smoking intensity) or a greater number of cigarettes over a shorter duration. It has been estimated that total exposure from smoking fewer cigarettes for a longer period of time is more deleterious than the equivalent exposure from smoking a greater number of cigarettes for a shorter period of time (34). Notably, the NCCN lung cancer screening guidelines currently include smokers with 20 pack-years and one additional risk factor as a second high-risk population for lung cancer (35).
Exclusion of former smokers who have quit for longer than 15 years contributes to a missed opportunity for early identification of lung cancer. We now know from PLCO data that, in individuals with a 30+ pack-year smoking history, the hazard ratio for lung cancer in former smokers relative to nonsmokers drops from 30.8 in individuals quit less than 5 years, to 14.8 in those quit 10 to 15 years (36). However the lung cancer risk remains high after 15 years of quitting, with hazard ratios of 13.5 and 9.9 in former smokers with 15 to 20 years, and 20 to 25 years quit time, respectively.
Who should be screened?
Optimizing the target population for lung cancer screening has tremendous significance, not only for the individual who weighs the risks and benefits of screening, but also for health care providers, health insurers and governments who must consider the fiscal impact of implementing lung cancer screening. With data from the NLST and the PLCO trials, de Koning et al. used computer modeling to estimate the benefits (lung cancer deaths averted, life-years gained) and harms (CT examinations, false-positive results, overdiagnosed cases, and radiation-related deaths) of lung cancer screening with varying eligibility criteria and screening intervals (37). They concluded that the most advantageous strategy was annual CT screening from ages 55 through 80 years for ever-smokers with a smoking history of at least 30 pack-years and ex-smokers with less than 15 years since quitting. The calculated benefits and harms of this screening strategy in a 100,000 person cohort are presented in Table 2.
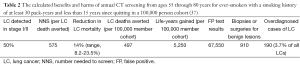
Full table
McMahon et al. reported similar eligibility criteria for lung cancer screening efficiency. They defined the most efficient screening strategy as the one with the greatest number of lung cancer deaths averted for each possible number of CT screens (38). Using five separate microsimulation models, they determined that the most efficient models included an average starting age of 55 years, a stopping age of 80 or 85 years, an average minimum pack-years of 27, and the maximum time since quitting of 20 years. Annual screening in a population of individuals 55 to 85 years old, with a ≥30 pack year smoking history, and fewer than 20 years since quitting yielded 593 lung cancer deaths avoided (per 100,000 population), with a number needed to screen of 44 per lung cancer death avoided, and 6,237 life-years saved. There was a consensus among models that, compared with the NLST eligibility criteria, continuing screening to older ages was more efficient than stopping at age 75, but initiating screening at younger ages (e.g., 45) was less efficient. Biennial screening and increasing the minimum pack-years (e.g., 40) provided fewer benefits.
Cost-effectiveness of lung cancer screening
For many years, estimates of the cost-effectiveness of CT screening for lung cancer were based on modeling, as limited data was available for real world analysis. These estimates varied widely, ranging from $2,500 (39) to $23,100 (40) per life-year saved for one-time screening, and $116,300 (current smokers) to $2,322,700 (former smokers) per quality-adjusted life-year (QALY) gained for a 20-year screening program (41). Current estimates continue to vary, especially in countries with differing models of health care delivery.
Using baseline results from ELCAP, Marshall et al. estimated that, in the US, a one-time screen was cost-effective in a very high-risk cohort of patients 60 to 74 years of age with a lung cancer prevalence of 2.7%, with a cost of US$5,940 per life year saved (based on 1999 reimbursement rates) (40). Even in a lower risk general population with a lung cancer prevalence of 0.7%, they determined that a one-time screen was cost-effective at US$23,100 per life year. They extended this analysis, in the same high-risk cohort, to annual screening for 5 years and determined that screening remained cost-effective, under optimal conditions, at approximately $19,000 per life year saved (42). Wisnivesky et al. also used ELCAP baseline screening data in a decision analysis model comparing low-dose CT scan screening of high-risk individuals (age >60 years, ≥10 pack-years of cigarette smoking) to observation without screening (39). They estimated that the incremental cost-effectiveness ratio (ICER) of a single screen was $2,500 per life year saved.
The costs per QALY gained were estimated to be much higher, in a computer-simulated model of 100,000 current, quitting, and former heavy smokers over a 20-year period, assuming a 50% stage shift and a 13% lung cancer mortality reduction (41). In this model, Mahadevia et al. estimated that the costs per QALY gained were $116,300 for current smokers, $558,600 for smokers who quit at the time of the screen, and $2,322,700 for smokers with a 5-year quit time. McMahon et al. implemented a patient-level microsimulation model (Lung Cancer Policy Model) to estimate the cost-effectiveness of lung cancer screening in six US cohorts (men and women, aged 50, 60 and 70 years), using survey data to attribute smoking histories to the cohorts (43). They determined that annual CT screening in individuals with ≥20 pack-years smoking history reduced lung cancer-specific mortality by approximately 18% to 25% at 10 years, at a cost of $126,000 to $169,000 per QALY gained.
Following the release of the NLST mortality data, cost-effectiveness analyses could now incorporate more specific data for lung cancer mortality reduction, diagnostic procedures and lung-cancer treatment. Within the American College of Radiology Imaging Network (ACRIN) arm of the NLST, detailed information was collected regarding health-related quality-of-life, diagnostic procedures performed, lung cancer staging and treatment. Black et al. calculated an incremental cost effectiveness ratio (ICER), which is the ratio of the incremental cost of screening to the incremental health benefit, of US$81,000 per QALY gained (95% CI, $52,000 to $186,000) in the CT-screened arm of the NLST, compared with no screening (44,45). There was a wide variation in ICERs in various subgroups, with lower ICERs in women ($46,000/QALY in women vs. $147,000/QALY in men), in current smokers ($43,000/QALY in current smokers vs. $615,000/QALY in former smokers), in the 60-69 years old age group ($48,000 to $52,000/QALY), and in individuals in the two highest risk quintiles ($32,000 and $52,000/QALY). These results highlight the opportunity to better refine selection criteria for screening, not only to maximize patient benefit and minimize patient risk, but to also provide a health service that is cost-effective. In this regard, extending eligibility criteria beyond age and smoking history may be of value.
Risk stratification
The NLST and NELSON trial designs incorporated the two most significant predictors of lung cancer risk: age and smoking history. Current recommendations for screening have followed suit, with slight variations in ages and pack-years for eligibility. It is possible, though, that with better risk prediction, screening could be more efficient, with more lung cancers diagnosed, and fewer individuals screened. The age and smoking history criteria that currently determine screening eligibility yield a population with widely varying risks of lung cancer. Kovalchik et al. stratified the NLST participants into five quintiles, according to the 5-year risk of lung cancer death, ranging from the lowest risk (0.15% to 0.55%) to the highest (more than 2.0%) (46). Within the CT arm, across all quintiles, there were 1,083 lung cancer cases, of which 530 (48.9%) were Stage I. When this data is broken down by risk quintile, it becomes apparent that there was only one lung cancer death prevented in the lowest risk quintile. The majority (88%) of screen-prevented lung cancer deaths were within the three highest risk quintiles. A screening program that selected only the 60% of patients in the higher risk groups would reduce the number needed to screen (NNS) to prevent one lung cancer death from 302 to 161, and would reduce the number of false positives per CT-prevented lung cancer death from 108 to 65. This would have a significant impact on the costs of lung cancer screening, not only in terms of financial costs, but in terms of patient risks. On the other hand, this approach would miss 16.2% (176/1,082) of lung cancer cases, and 12.5% (11/88) of preventable lung cancer deaths.
Tammemägi et al. developed a logistic regression lung cancer risk prediction model based on the 6-year incidence of lung cancer in smokers in the control arm of the PLCO trial (47). The model includes four smoking variables [smoking status (current/former), number of cigarettes smoked per day, duration of smoking (years), and quit time (years)] and seven non-smoking variables (age, education level, body mass index, history of COPD/emphysema/chronic bronchitis, personal history of cancer, family history of lung cancer, race and ethnicity). This model is the basis for an on-line calculator (48) that allows individuals to determine their likelihood of developing lung cancer within the next 6 years, after entering the personal data described above. Health care providers can use risk prediction calculations such as these, within a shared decision making session, to counsel patients on their individual lung cancer risks, and the advisability of lung cancer screening.
False positive screens
The NLST protocol defined a mean nodule diameter of 4 mm or greater as a positive screen. As a result, there was a high rate of false positive screens in both the LDCT and the CXR groups (96.4% and 94.5%). Further analysis of NLST and ELCAP data suggest that the number of false positive screens can be reduced without significantly impacting sensitivity by using larger nodule diameter thresholds (49,50). Currently, the NCCN and ACR guidelines define a positive screen as a nodule with a mean diameter of 6 mm or larger (35,51). If this threshold had been applied in the International ELCAP, there would have been a 36% decrease in diagnostic work-ups and no delayed diagnoses of lung cancer (49). Applying a 6 mm threshold to the NLST data avoids 37% of the false positives, but there would be a delay in the diagnosis of 3% of the lung cancer cases (50).
The NELSON trial design also reduces the number of screens considered false positive, by using three-dimensional volume measurements rather than the mean nodule diameters used in earlier trials. The NELSON trial added a third “indeterminate” category of scan results to the standard “positive” and “negative” categories. These three categories of screening results were based on nodule volume measurements, and defined as (I) positive: non-calcified nodule with a solid component more than 500 mm3 in volume (equivalent to >9.8 mm in diameter); (II) indeterminate: volume of the largest solid nodule or of the solid component of a part-solid nodule of 50 to 500 mm3 (4.6 to 9.8 mm in diameter) or the diameter of a nonsolid nodule greater than 8 mm and (III) negative. Patients with indeterminate screens underwent a 3-month follow-up CT. If there was no significant growth on the follow-up CT, the screen was considered to be negative and the patient was rescheduled for an annual repeat CT in 9 months. Nodule growth was measured as Volume Doubling Time (VDT), which was divided into three categories: >600 days, 400-600 days, and <400 days (52). This two-step approach to nodules 50 to 500 mm3 in volume significantly reduced the number of false positive screens, returning the majority of patients to an annual screening regimen after only a 3-month delay.
Risk stratification can also occur at the nodule level. Just as risk stratification can determine an individual’s likelihood of malignancy, risk stratification can assist in determining the likelihood that a nodule identified on a screening CT is malignant. The use of these nodule-oriented risk prediction models can further minimize the harms of false positive findings by reducing the number of unnecessary diagnostic and invasive procedures. In 1997, Swensen et al. published a prediction model for indeterminate nodules that incorporated three clinical characteristics (patient age, smoking status, history of cancer) and three nodule characteristics (diameter, spiculation, upper lobe location) (53). More recent models have expanded this concept to include nodule volumes (54). Horeweg et al. reported that the NELSON nodule management protocols based on nodule volumes and volume doubling times described above (55) outperformed the American College of Chest Physicians (ACCP) nodule management guidelines (56), which are based on the diameter-specific Fleischner Society recommendations (57).
Establishing a clinical screening program
A successful screening program outside of a clinical trial requires robust resources and significant buy-in from a variety of stakeholders. The initial stage of program inception demands close collaboration between administrative and clinical staff. Preliminary meetings should include specialists in thoracic radiology, thoracic oncology, pulmonology, and thoracic surgery. If desired, interventional radiology and pulmonary pathology may participate as well. One or more of these specialists should be designated as a medical director or physician champion of the program. Other key participants in program construction include hospital administration, business management, nursing, and marketing personnel. Active participation from all of these stakeholders is required for initial startup and ultimate success.
Patient recruitment into the program is the fuel which feeds a sustained effort. An effective marketing strategy is required to reach health care providers and patients alike. For instance, educational resources can be offered to primary care providers and physician extenders who encounter significant numbers of potentially eligible patients. These resources should form the basis for a shared decision making process, and clearly delineate eligibility criteria, benefits and risks of screening. Educational resources may be in the form of pamphlets, e-mail brochures, one-on-one office visits, grand rounds, and after-hours seminars. Follow-up phone calls may address key questions from these providers. An effective marketing strategy may include direct marketing to at-risk patient populations. Print media, broadcast media, internet, office pamphlets and posters can all play a role in reaching middle-aged and older smokers. The program’s marketers should receive feedback from enrolled patients to determine the effectiveness of these marketing strategies, in order to tailor and refine these techniques.
Smooth operation of a clinical screening program will require a multifaceted effort. Prior to scheduling an exam, appropriate personnel must screen each patient to determine eligibility, and assure that shared decision making takes place with an appropriate clinical provider (58). Hospital administration and/or business personnel should oversee patient scheduling, registration, and payment. In the US, some programs offer services on a cash basis, but since the advent of the US Preventive Services Task Force (USPSTF) certification (59) and Centers for Medicare and Medicaid Services (CMS) approval (58), third party payment in the US has become more common. Precertification may or may not be necessary depending on the payer. Image acquisition requires a CT scanner capable of performing a single-breath-hold low-dose screening protocol, typically 16-channel or beyond. Although the scan protocols are straightforward, technologists with special registry in CT are necessary to provide consistent, high-quality scans with appropriate post-processing. Most programs utilize thoracic trained radiologists for image interpretation and reporting, although this function may also be performed by other radiologists with documented experience in chest CT (60). Images are transmitted to a picture archiving and communication system (PACS) which allow display for interpretation, archival, and accessibility to referring clinicians if desired.
Recent efforts by organized leadership in thoracic radiology have developed a structured reporting system analogous to commonly used mammography reporting (51). This “Lung-RADS™” system provides a common set of follow-up guidelines for nodules of certain levels of suspicion. It is critical that all members of the clinical team understand the meaning of the Lung-RADS™ categories so that appropriate follow-up care can be predictable and effective (Table 3) (51,61).
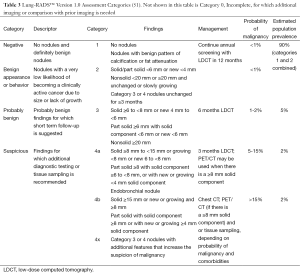
Full table
Successful centers typically enlist a core group of providers, often as part of a multi-disciplinary thoracic clinic, who manage data collected within the screening program. A nurse navigator is a crucial member of the clinic team. The navigator can be responsible for CMS-mandated patient risk counseling and shared/informed decision-making prior to the procedure, as well as smoking cessation counseling prior to and subsequent to the procedure (62). Weekly conferences can assist in discussing management of cases requiring intervention or close follow-up (e.g., Lung-RADS™ 3 & 4.) These management discussions are often part of a weekly multidisciplinary thoracic conference including members of thoracic surgery, pulmonary medicine, thoracic oncology, thoracic radiology, pathology, and radiation oncology.
Robust data collection is mandatory for a successful screening program. Local databases should include appropriate patient demographics (usually available through radiology/hospital information systems), exam results, follow-up recommendations, pathology results, and significant extrapulmonary findings. Structured reporting (e.g., Lung-RADS™) improves the meaningfulness of such data. From this data the team may analyze positive screen rates, false-positive rates, and outcomes. Data may also be used to provide timely reminders for follow-up. In addition, CMS rules mandate that screening programs participate in an approved lung cancer screening registry (58).
The financial resources required to establish a lung cancer screening program are not small. Some of the key expenses involved include clinic space, salaries of administrative personnel, professional services, marketing teams, and employees. In the US, typical direct reimbursements for lung cancer screening include Medicare Part B (approved in February 2015), third party commercial insurance (mandated by the Affordable Care Act due to USPSTF recommendations), donations, grants, and occasionally direct cash payments from screened patients. Many screening programs will find that these direct reimbursement sources do not cover the entire cost of the program, and that reimbursement for “downstream” events such as follow-up diagnostic testing or surgical procedures may make up for such shortfalls.
Despite excellent planning and execution of a lung cancer screening program, multiple challenges remain. Primary care clinicians may feel that their patients are candidates for screening, and wish to refer them, but fear relinquishing control of the patient’s care to the multidisciplinary team. While it is key that the multidisciplinary team supervise and control the screening process and data collection, primary care providers should be welcomed to participate with the multidisciplinary team when their patients are discussed.
The CMS-mandated shared decision making process can be time intensive for the nurse coordinator or referring primary care physician. Not uncommonly, patients will present for screening but do not meet accepted eligibility criteria. Program leaders should have established policies on whether they wish to screen self-paying patients not meeting eligibility criteria, as this can present an ethical challenge. These patients should still be offered counseling regarding smoking cessation.
Fail-safe mechanisms need to be in place to assure that timely results are communicated to the patient and referring provider. Additionally, similar mechanisms must assure that appropriate follow-up exams or procedures are scheduled in a timely manner. Accurate patient contact data (e.g., phone number, home address, email address) are crucial to prevent patients from “slipping through the cracks”. Hospital or clinic information systems may have methods to provide automated patient reminders for follow-up screening, and can be helpful. During all patient contacts, the nurse coordinator must often coach patients on the importance of adherence to follow-up recommendations.
As has been the case with other types of screening over the past several decades, the growth and effectiveness of a population screening strategy is often heavily reliant on appropriately adequate reimbursements to allow the healthy sustenance of a screening program. Not surprisingly, third party payers often look to cost-effectiveness models to justify inclusion of a screening procedure in their covered benefits. Early analyses of lung cancer screening indeed seem to support its cost-effectiveness (44,63).
Future directions for lung cancer screening
As we move forward from the research domain into clinical application, guidelines for screening for lung cancer should continue to incorporate emerging data. Risk stratification calculations can refine patient eligibility criteria so that we optimize the diagnostic yield of CT screening. This will hopefully help identify lung cancers in patients who do not meet current eligibility criteria, as well as limit screening in patients who are currently eligible but at lower risk. Techniques that offer even lower radiation doses could be mandated. The risks of screening can be further reduced by using nodule risk predictors, including measuring nodule volumes and growth rates, to reduce the number of follow-up examinations and invasive procedures. Structured reporting systems such as Lung-RADS™ should undergo continual evaluation and modification. Finally, we must collect the data from clinical screening programs to ensure that CT screening for lung cancer continues to provide a cost-effective mortality benefit in specific populations, regions, or age groups.
Acknowledgements
None.
Footnote
Conflicts of Interest: The authors have no conflicts of interest to declare.
References
- Siegel RL, Miller KD, Jemal A. Cancer statistics, 2015. CA Cancer J Clin 2015;65:5-29. [PubMed]
- Available online: http://seer.cancer.gov/statfacts/, accessed May 22, 2015.
- National Lung Screening Trial Research Team, Aberle DR, Adams AM, et al. Reduced lung-cancer mortality with low-dose computed tomographic screening. N Engl J Med 2011;365:395-409. [PubMed]
- Heuvers ME, Wisnivesky J, Stricker BH, et al. Generalizability of results from the National Lung Screening Trial. Eur J Epidemiol 2012;27:669-72. [PubMed]
- Berlin NI, Buncher CR, Fontana RS, et al. The National Cancer Institute Cooperative Early Lung Cancer Detection Program. Results of the initial screen (prevalence). Early lung cancer detection: Introduction. Am Rev Respir Dis 1984;130:545-9. [PubMed]
- Flehinger BJ, Melamed MR, Zaman MB, et al. Early lung cancer detection: results of the initial (prevalence) radiologic and cytologic screening in the Memorial Sloan-Kettering study. Am Rev Respir Dis 1984;130:555-60. [PubMed]
- Fontana RS, Sanderson DR, Taylor WF, et al. Early lung cancer detection: results of the initial (prevalence) radiologic and cytologic screening in the Mayo Clinic study. Am Rev Respir Dis 1984;130:561-5. [PubMed]
- Frost JK, Ball WC Jr, Levin ML, et al. Early lung cancer detection: results of the initial (prevalence) radiologic and cytologic screening in the Johns Hopkins study. Am Rev Respir Dis 1984;130:549-54. [PubMed]
- Fontana RS. The Mayo Lung Project: a perspective. Cancer 2000;89:2352-5. [PubMed]
- Strauss GM, Gleason RE, Sugarbaker DJ. Screening for lung cancer re-examined. A reinterpretation of the Mayo Lung Project randomized trial on lung cancer screening. Chest 1993;103:337S-341S. [PubMed]
- Hocking WG, Hu P, Oken MM, et al. Lung cancer screening in the randomized Prostate, Lung, Colorectal, and Ovarian (PLCO) Cancer Screening Trial. J Natl Cancer Inst 2010;102:722-31. [PubMed]
- Oken MM, Hocking WG, Kvale PA, et al. Screening by chest radiograph and lung cancer mortality: the Prostate, Lung, Colorectal, and Ovarian (PLCO) randomized trial. JAMA 2011;306:1865-73. [PubMed]
- Goldman LW. Principles of CT: multislice CT. J Nucl Med Technol 2008;36:57-68. [PubMed]
- Kaneko M, Eguchi K, Ohmatsu H, et al. Peripheral lung cancer: screening and detection with low-dose spiral CT versus radiography. Radiology 1996;201:798-802. [PubMed]
- Henschke CI, McCauley DI, Yankelevitz DF, et al. Early Lung Cancer Action Project: overall design and findings from baseline screening. Lancet 1999;354:99-105. [PubMed]
- Field JK, van Klaveren R, Pedersen JH, et al. European randomized lung cancer screening trials: Post NLST. J Surg Oncol 2013;108:280-6. [PubMed]
- van Iersel CA, de Koning HJ, Draisma G, et al. Risk-based selection from the general population in a screening trial: selection criteria, recruitment and power for the Dutch-Belgian randomised lung cancer multi-slice CT screening trial (NELSON). Int J Cancer 2007;120:868-74. [PubMed]
- Pedersen JH, Ashraf H, Dirksen A, et al. The Danish randomized lung cancer CT screening trial--overall design and results of the prevalence round. J Thorac Oncol 2009;4:608-14. [PubMed]
- Pastorino U, Rossi M, Rosato V, et al. Annual or biennial CT screening versus observation in heavy smokers: 5-year results of the MILD trial. Eur J Cancer Prev 2012;21:308-15. [PubMed]
- Design, recruitment and baseline results of the ITALUNG trial for lung cancer screening with low-dose CT. Lung Cancer 2009;64:34-40. [PubMed]
- Infante M, Lutman FR, Cavuto S, et al. Lung cancer screening with spiral CT: baseline results of the randomized DANTE trial. Lung Cancer 2008;59:355-63. [PubMed]
- Becker N, Motsch E, Gross ML, et al. Randomized study on early detection of lung cancer with MSCT in Germany: study design and results of the first screening round. J Cancer Res Clin Oncol 2012;138:1475-86. [PubMed]
- Baldwin DR, Duffy SW, Wald NJ, et al. UK Lung Screen (UKLS) nodule management protocol: modelling of a single screen randomised controlled trial of low-dose CT screening for lung cancer. Thorax 2011;66:308-13. [PubMed]
- Heuvelmans MA, Vliegenthart R, Oudkerk M. Contributions of the European trials (European randomized screening group) in computed tomography lung cancer screening. J Thorac Imaging 2015;30:101-7. [PubMed]
- National Lung Screening Trial Research Team, Aberle DR, Adams AM, et al. Baseline characteristics of participants in the randomized national lung screening trial. J Natl Cancer Inst 2010;102:1771-9. [PubMed]
- Pinsky PF, Church TR, Izmirlian G, et al. The National Lung Screening Trial: results stratified by demographics, smoking history, and lung cancer histology. Cancer 2013;119:3976-83. [PubMed]
- Cassidy A, Myles JP, van Tongeren M, et al. The LLP risk model: an individual risk prediction model for lung cancer. Br J Cancer 2008;98:270-6. [PubMed]
- McRonald FE, Yadegarfar G, Baldwin DR, et al. The UK Lung Screen (UKLS): demographic profile of first 88,897 approaches provides recommendations for population screening. Cancer Prev Res (Phila) 2014;7:362-71. [PubMed]
- Aberle DR, DeMello S, Berg CD, et al. Results of the two incidence screenings in the National Lung Screening Trial. N Engl J Med 2013;369:920-31. [PubMed]
- National Lung Screening Trial Research Team, Church TR, Black WC, et al. Results of initial low-dose computed tomographic screening for lung cancer. N Engl J Med 2013;368:1980-91. [PubMed]
- Horeweg N, van der Aalst CM, Thunnissen E, et al. Characteristics of lung cancers detected by computer tomography screening in the randomized NELSON trial. Am J Respir Crit Care Med 2013;187:848-54. [PubMed]
- Available online: http://globocan.iarc.fr/Pages/fact_sheets_population.aspx, accessed May 22, 2015.
- Pinsky PF, Berg CD. Applying the National Lung Screening Trial eligibility criteria to the US population: what percent of the population and of incident lung cancers would be covered? J Med Screen 2012;19:154-6. [PubMed]
- Lubin JH, Caporaso NE. Cigarette smoking and lung cancer: modeling total exposure and intensity. Cancer Epidemiol Biomarkers Prev 2006;15:517-23. [PubMed]
- NationalComprehensiveCancerNetwork. Lung cancer screening, Version 2.2015 [accessed June 16, 2015]. Available online: http://www.nccn.org/professionals/physician_gls/f_guidelines.asp#lung_screening
- Pinsky PF, Zhu CS, Kramer BS. Lung cancer risk by years since quitting in 30+ pack year smokers. J Med Screen 2015. [Epub ahead of print]. [PubMed]
- de Koning HJ, Meza R, Plevritis SK, et al. Benefits and harms of computed tomography lung cancer screening strategies: a comparative modeling study for the U.S. Preventive Services Task Force. Ann Intern Med 2014;160:311-20. [PubMed]
- McMahon PM, Meza R, Plevritis SK, et al. Comparing benefits from many possible computed tomography lung cancer screening programs: extrapolating from the National Lung Screening Trial using comparative modeling. PLoS One 2014;9:e99978. [PubMed]
- Wisnivesky JP, Mushlin AI, Sicherman N, et al. The cost-effectiveness of low-dose CT screening for lung cancer: preliminary results of baseline screening. Chest 2003;124:614-21. [PubMed]
- Marshall D, Simpson KN, Earle CC, et al. Potential cost-effectiveness of one-time screening for lung cancer (LC) in a high risk cohort. Lung Cancer 2001;32:227-36. [PubMed]
- Mahadevia PJ, Fleisher LA, Frick KD, et al. Lung cancer screening with helical computed tomography in older adult smokers: a decision and cost-effectiveness analysis. JAMA 2003;289:313-22. [PubMed]
- Marshall D, Simpson KN, Earle CC, et al. Economic decision analysis model of screening for lung cancer. Eur J Cancer 2001;37:1759-67. [PubMed]
- McMahon PM, Kong CY, Bouzan C, et al. Cost-effectiveness of computed tomography screening for lung cancer in the United States. J Thorac Oncol 2011;6:1841-8. [PubMed]
- Black WC, Gareen IF, Soneji SS, et al. Cost-effectiveness of CT screening in the National Lung Screening Trial. N Engl J Med 2014;371:1793-802. [PubMed]
- Black WC. Computed tomography screening for lung cancer in the National Lung Screening Trial: a cost-effectiveness analysis. J Thorac Imaging 2015;30:79-87. [PubMed]
- Kovalchik SA, Tammemagi M, Berg CD, et al. Targeting of low-dose CT screening according to the risk of lung-cancer death. N Engl J Med 2013;369:245-54. [PubMed]
- Tammemägi MC, Katki HA, Hocking WG, et al. Selection criteria for lung-cancer screening. N Engl J Med 2013;368:728-36. [PubMed]
- Available online: http://www.brocku.ca/lung-cancer-risk-calculator, accessed June 1, 2015.
- Henschke CI, Yip R, Yankelevitz DF, et al. Definition of a positive test result in computed tomography screening for lung cancer: a cohort study. Ann Intern Med 2013;158:246-52. [PubMed]
- Gierada DS, Pinsky P, Nath H, et al. Projected outcomes using different nodule sizes to define a positive CT lung cancer screening examination. J Natl Cancer Inst 2014.106. [PubMed]
- Available online: http://www.acr.org/~/media/ACR/Documents/PDF/QualitySafety/Resources/LungRADS/AssessmentCategories, accessed June 1, 2015.
- Xu DM, Gietema H, de Koning H, et al. Nodule management protocol of the NELSON randomised lung cancer screening trial. Lung Cancer 2006;54:177-84. [PubMed]
- Swensen SJ, Silverstein MD, Ilstrup DM, et al. The probability of malignancy in solitary pulmonary nodules. Application to small radiologically indeterminate nodules. Arch Intern Med 1997;157:849-55. [PubMed]
- Mehta HJ, Ravenel JG, Shaftman SR, et al. The utility of nodule volume in the context of malignancy prediction for small pulmonary nodules. Chest 2014;145:464-72. [PubMed]
- Horeweg N, van Rosmalen J, Heuvelmans MA, et al. Lung cancer probability in patients with CT-detected pulmonary nodules: a prespecified analysis of data from the NELSON trial of low-dose CT screening. Lancet Oncol 2014;15:1332-41. [PubMed]
- Gould MK, Fletcher J, Iannettoni MD, et al. Evaluation of patients with pulmonary nodules: when is it lung cancer?: ACCP evidence-based clinical practice guidelines (2nd edition). Chest 2007;132:108S-30S.
- MacMahon H, Austin JH, Gamsu G, et al. Guidelines for management of small pulmonary nodules detected on CT scans: a statement from the Fleischner Society. Radiology 2005;237:395-400. [PubMed]
- Available online: http://www.cms.gov/medicare-coverage-database/details/nca-proposed-decision-memo.aspx?NCAId=274, accessed June 1, 2015.
- Moyer VA, U.S. Preventive Services Task Force. Screening for lung cancer: U.S. Preventive Services Task Force recommendation statement. Ann Intern Med 2014;160:330-8. [PubMed]
- Available online: http://www.acr.org/~/media/ACR/Documents/PGTS/guidelines/LungScreening.pdf, accessed June 1, 2015.
- McKee BJ, McKee AB, Kitts AB, et al. Low-dose computed tomography screening for lung cancer in a clinical setting: essential elements of a screening program. J Thorac Imaging 2015;30:115-29. [PubMed]
- Park ER, Gareen IF, Japuntich S, et al. Primary Care Provider-Delivered Smoking Cessation Interventions and Smoking Cessation Among Participants in the National Lung Screening Trial. JAMA Intern Med 2015. [Epub ahead of print]. [PubMed]
- Pyenson BS, Henschke CI, Yankelevitz DF, et al. Offering lung cancer screening to high-risk medicare beneficiaries saves lives and is cost-effective: an actuarial analysis. Am Health Drug Benefits 2014;7:272-82. [PubMed]