Potential toxicities of prophylactic cranial irradiation
Abstract: Prophylactic cranial irradiation (PCI) with total doses of 20-30 Gy reduces the incidence of brain metastasis (BM) and increases survival of patients with limited and extensive-disease small-cell lung cancer (SCLC) that showed any response to chemotherapy. PCI is currently not applied in non-small-cell lung cancer (NSCLC) since it has not proven to significantly improve OS rates in stage IIIA/B, although novel data suggest that subgroups that could benefit may exist.
Here we briefly review potential toxicities of PCI which have to be considered before prescribing PCI. They are mostly difficult to delineate from pre-existing risk factors which include preceding chemotherapy, patient age, paraneoplasia, as well as smoking or atherosclerosis. On the long run, this will force radiation oncologists to evaluate each patient separately and to estimate the individual risk. Where PCI is then considered to be of benefit, novel concepts, such as intensity-modulated radiotherapy and/or neuroprotective drugs with potential to lower the rates of side effects will eventually be superior to conventional therapy. This in turn will lead to a re-evaluation whether benefits might then outweigh the (lowered) risks.
Key words: Prophylactic cranial irradiation (PCI); potential toxicities; brain metastasis (BM); small-cell lung cancer (SCLC); non-small-cell lung cancer (NSCLC)
Introduction
Since many cytotoxic or cytostatic drugs that were used in the treatment of acute lymphoblastic leukemia (ALL) did not adequately cross the blood-brain barrier, prophylactic cranial irradiation (PCI) was introduced in the 1960s to target the subclinical leukemic residuals in the central nervous system (1). PCI led to a substantial improvement of disease-free survival (DFS) and overall survival (OS), yet has on the long run become nearly fully replaced by (long-term) intrathecal chemotherapy (ICT) without affecting DFS and OS (2). The rationale behind the change of modalities was not established quickly, but has rather evolved on the basis of long-term follow-ups, in which late radiation-induced toxicities including neurological and neurocognitive impairments, hormone deregulation, and secondary cancers appeared in high frequencies especially in pediatric patients (3-7).
To date, only few indications for PCI in adult and childhood leukemia exist. It is still applied in childhood AML (8) as well as in childhood ALL if the risk for relapse is high or if patients do not adequately respond to chemotherapy (9). In adults, PCI is performed in highly aggressive (Philadelphia-chromosome positive) ALL, for example in the GMALL PH-01 study as part of the induction therapy (EudraCT 2010-022854-18).
For small-cell lung cancer (SCLC), PCI was included in standard regimens for limited disease (LD) patients in the early 1980s, where it was clearly shown that it efficiently reduced the 2-year occurrence rate of brain metastases (BM) from 58% to 11% (10) . PCI also showed to improve OS of patients in complete remission (CR), as impressively demonstrated in a meta-analysis of seven individual trials from 1977-1994 by Aupérin and colleagues (11). Furthermore, the EORTC multicentre randomized phase III trial showed that even patients with extensive disease (ED)-SCLC will benefit from PCI with respect to DFS and OS if they showed any response to chemotherapy (12).
In contrast to the beneficiary effects in SCLC, PCI has failed to improve OS rates in non-small-cell lung cancer (NSCLC) in multiple randomized clinical studies (13-16). Additionally, since the relapse rates with brain as first site have not changed after application of PCI (17,18), it is currently omitted in NSCLC treatment regimens.
There is controversy about how precisely one may delineate PCI toxicity from disease- or treatment specific toxicities that are present a priori or that will (co-)elicit side effects of the irradiation. We will briefly introduce current dose regimens and review toxicities that, from our clinical experience, are the commonly experienced ones during and after PCI.
Technical implementation and dosess
To date, PCI is commonly initiated within 4-6 weeks after chemotherapy using bilateral, parallel opposing fields with high energy (megavoltage) photons (doses are commonly specified to the midline), whereas each field is treated daily on a 4-5 times per week schedule. Depending on the underlying disease, the total doses range from 12 to 36 Gy.
As a part of the treatment for childhood ALL, early PCI regimens consisted of doses of 12-24 Gy and long-term studies found that the dose can be safely reduced without any loss of effectivity (19). The DFCI Consortium protocol includes PCI with 12 Gy in two daily fractions of 0.9 Gy concomitant with double ICT for standard-risk patients (20). For patients with high risk ALL, the AALL0232 study protocol foresees PCI with 12 Gy in 8 fractions only for slow early responders without manifest CNS involvement (9).
Due to the variety of different findings there is still uncertainty which dose might be optimal for SCLC. It is believed from dose-response analyses that at least 30-36 Gy, applied in 2 Gy-fractions are most likely needed to eliminate cranial metastasis (21) and that doses below 30 Gy have no effects (22). A meta-analysis in 1998 analyzed 7 different regimens for PCI and found the lowest hazard ratio for 36 Gy in 18 fractions (23). The younger RTOG 02 12 trial also addressed this question and randomly assigned LD-SCLC patients to PCI with the standard dose (arm 1) of 25 Gy in 10 fractions or in an arm using higher doses (arm 2) of 36 Gy in 18 or 24 fractions (24). The study showed no difference in the incidence of BM when comparing high to low dose PCI but a significant increase in mortality when higher doses were given. For ED-SCLC, in contrast, the 2007 EORTC trial showed comparable benefits and toxicities when using 20 Gy in 5-8 fractions, 24 Gy in 12 fractions, 25 Gy in 10 fractions and 30 Gy in 10 or 12 fractions (12).
A commonly applied dose for PCI in the past NSCLC studies was 30 Gy in 15 fractions (13,14,25). In the SWOG study, the first patients that were recruited were treated with 37.5 Gy in fractions of 2.5 Gy which led to an increased death rate, prompting the committee to reduce the dose 30 Gy in 2 Gy fractions for all further patients (26).
Toxicity
Prophylactic and, even more, therapeutic irradiation of the brain may be accompanied by early and late side effects. Early side effects may be reversible and appear during PCI or slightly delayed within few weeks after the end of radiotherapy. A first classification of the temporal occurrence of radiation-related toxicities was done by Sheline and colleagues in the 1980s (27), in which acute and subacute radiation reactions of the brain at around four months were distinguished from delayed irreversible impairments months or years after completion of cranial radiotherapy (Table 1). In a prospective study on 44 patients with and without BM our group evaluated very early effects (tests were performed before PCI, during PCI and 6-8 weeks after PCI) of PCI on neurocognition. Our analysis has shown that in contrast to therapeutic cranial irradiation (TCI), PCI does not considerably impair cognitive functions after 1-3 fractions of irradiation (28). In contrast, 6-8 weeks after PCI, patients scored significantly lower on verbal memory as compared to a control group receiving radiotherapy to the breast (28). Of note, 44% of patients that received PCI showed a decline of at least one verbal memory subtest and, at this time point, patients that received PCI scored similarly to patients that received TCI. However, there is still uncertainty about how this rate correlates to a much higher rate of CNS demyelination (60-100%) that can be seen months to years after PCI (Figure 1) (29).
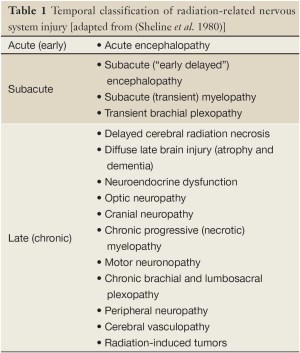
Full table
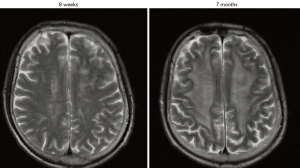
The incidence of toxicities are likely dependent on certain a priori risk factors, including age, preceding or concomitant chemotherapy and other putative risk factors that are to date not exactly defined as such (i.e. smoking and/or atherosclerosis). The most evident risk factor for developing side effects is age: since the developing brain is much more sensitive to irradiation than the adult brain, PCI in children leads to high rates of acute and late toxicities (30-32). An early effect after PCI in children has been observed in an ancient report of Freeman and colleagues, who noted abnormal behavioural patterns in patients with ALL. In detail, more than two thirds of the children showed somnolence, anorexia, and lethargy at around 6 weeks after PCI (33). Interestingly, all symptoms were transient and completely resolved spontaneously, fully consistent with later investigations which showed that a transient demyelination of CNS nerve fibres is the underlying cause (34). The complex mixture of symptoms with somnolence being the chief complaint lead the community to dub this early PCI toxicity “somnolence syndrome” (35). The syndrome does not seem to be dose-dependent and has to date appeared at fairly any dose between 18 and 24 Gy (36). It was even described to occur 6 weeks after total body irradiation (TBI) with 12 Gy before bone marrow transplantation (37). Although the somnolence syndrome was also described to transiently occur in adults (38), neurotoxicity after TBI remains extremely rare. In a prospective study by our group, we followed 58 patients that underwent hyperfractionated TBI (14.4 Gy in 12 fractions) before bone marrow transplantation and saw no impairment in any of the applied neuropsychological tests (the median follow up was 27 months) (39).
An important aspect of PCI toxicity in children is temporary or permanent hormone dysfunction. It is to date not clear whether the pituitary gland, the hypothalamus or both are affected by irradiation. However, early studies showed that hypopituitarism is dose depended, whereas lower doses (in the range of 12-24 Gy) seem to solely induce growth hormone (GH) dysfunction (40,41). The GH axis is the most vulnerable axis and impairment represents a major concern when prescribing PCI for (pubertal) children. Of note, adult patients easily compensate GH deficiency (42). At higher doses (including those used for PCI, e.g., 30-36 Gy) TSH secretion is found impaired in nearly 30% and may remain disturbed long-term in up to 9 % of patients (43,44). It is well known that (in this case secondary) hypothyroidism will not only result in somatic disorders but also cause neuropsychiatric symptoms such as mood changes or depression. If left untreated (or undetected long time after PCI) cognitive functions will be affected and patients will show impaired executive functions and poor learning capabilities (45).
Caution is advised when diagnosing PCI toxicity in adults, as many of the “classical” PCI toxicity data is derived from studies on SCLC, where patients primarily undergo chemotherapy which is followed by PCI. The influence of chemotherapy on cognitive functions of many cancer patients, often referred to as “chemo-brain” or “chemo fog” remained underestimated for a long time (46). Largely similar to the side effects evoked by cranial irradiation, it is not surprising that the effects were rather attributed to the radiation than to chemotherapy (47). However, already more than a decade ago, it has been noted that patients receiving adjuvant (standard-dose) chemotherapy for breast cancer exhibit decreased cognitive functions (memory and language) as compared to matched control groups (48). In a prospective study by Komaki et al., SCLC patients that finished initial therapy were asked to perform neuropsychological tests before and after PCI (49). Although the authors saw a slightly significant decline of executive function and language after 1 year (which turned insignificant in later tests), they noted that roughly half of patients have neurocognitive deficits before PCI (49). Logically, highest toxicity rates were observed in studies where chemotherapy was concomitantly applied with PCI: a simultaneous low dose concurrent chemotherapy during PCI will result in abnormal neuropsychological tests in nearly one half of all treated patients with SCLC (median follow-up ~6 years) (50). Nowadays it is accepted that chemotherapy alone induces white matter changes especially in the frontal, parietal and occipital lobes, consistent with the notion of chemotherapy-related axonal degeneration and demyelination (51,52). Of note, there is evidence that associated cognitive deficits persist for more than 10 years, which can overlay most (if not all) PCI-induced toxicities (51).
Another confounder that has to be considered when diagnosing PCI toxicity is the broad variety of possible paraneoplastic syndromes, which may even be manifesting prior to the underlying lung cancer, or its recurrence, respectively (53). Especially patients with SCLC may present with CNS-involving paraneoplasias, such as subacute sensory neuropathy, mononeuritis multiplex, Lambert-Eaton myasthenic syndrome, encephalomyelitis or necrotizing myelopathy (54). Although the etiologies are currently not clear, it is discussed whether paraneoplasias may resemble a classical autoimmune disease with the presence of “onconeural” antibodies (55). CNS-involving paraneoplasia leads to impaired neurocognitive functions, including memory loss, distractibility, fatigue or mood disturbances (56), which are symptoms that are highly similar to those induced by PCI.
Beside residuals of the preceding chemotherapy and paraneoplasia, other factors have to be mentioned that may elicit PCI toxicities. “Baseline” pathologies such as undetected micrometastases, long-term smoking effects or other age-related abnormalities may flare up in patients during treatment. It is even possible that one condition or therapy may increase the toxicity of another: more than two decades ago Johnson et al. studied long-term survivors of SCLC and saw that CT-morphological (ventricular dilatation and cerebral atrophy) abnormalities and impaired neurocognitive effects are more frequent when chemotherapy was concurrently applied (50). Similar conclusions were drawn later by other groups (57-59). Another example might be that age per se can display a risk factor for late effects after PCI since the irradiated brain ages faster and is at risk for early onset dementia (60). In line with this, the RTOG 02-12 study (see above) detected higher age to be the most predictive risk factor for PCI toxicities (61). Of note, it is known since the early 70s that a pre-existing vascular damage is accelerated by radiation (62) and assuming that more than 95% of all SCLC/NSCLC patients are smokers, a possible underlying cause of this age-related PCI toxicity may be the higher incidence of hypertension and/or (cerebral) arthrosclerosis in these patients.
As many of the patients receiving PCI have a more or less reduced life expectancy, the remaining quality of life (QoL) probably displays one of the most important measures for clinical decision making. For SCLC, QoL after PCI has been assessed in three randomized controlled trials, whereas two of which showed no significant differences between patients receiving PCI and controls (22,61). In the EORTC trial, Slotman and colleagues defined six QoL end points: global health status, hair loss, fatigue, and role, cognitive and emotional functioning. Although baseline scores were similar in both arms, a significant decline of QoL due to hair loss and fatigue (at 6 weeks and 3 months) was observed in patients receiving PCI (63). It has to be noted for completeness that there was no evidence for reduced QoL after PCI in patients with advanced-stage NSCLC (16).
Novel concepts avoiding PCI toxicities and putatively new indications
More work will be necessary to further delineate the influencing factors that may contribute to PCI toxicities. To start with, the optimal dose and fractionation schedule for PCI should soon be clearly defined as this question is a part of ongoing studies (e.g., for SCLC in RTOG 02-12). Novel techniques to avoid known adverse effects at any dose will also be required as part of a new concept aiming at improving the therapeutic ratio of PCI. With the advent of highly-conformal intensity-modulated radiotherapy (IMRT), organs at risk such as the hippocampus and scalp may be better spared.
The RTOG 0933 trial aims to find out whether sparing of the hippocampus during cranial irradiation may lower the incidence of radiation-induced neurocognitive toxicity. The rationale behind this trial is that the hippocampus is rarely affected by BM but displays the major site of learning, memory and spatial information (64). In one of the initial feasibility studies exploring IMRT for PCI, the mean doses to the hippocampus could be reduced by 81-87% to doses of 0.49-0.73 Gy with preserved target volume coverage and homogeneity (65).
Given that IMRT may also be used to prevent permanent hair loss, which significantly decreases QoL of both female and male cancer patients (66-69) and (as stated above) also decreases QoL after PCI (63). Roberge et al. were among the first to show the possibility to reduce doses on the scalp. They validated their alopecia-avoiding whole brain radiation therapy plans with thermoluminescent dosimetry and showed that the median dose to the scalp can be reduced by nearly 40% without affecting target volume doses (70). Although this does not prevent transient alopecia in most of the treated patients, it may be of benefit for those with a priori risk factor for increased alopecia (e.g., previous or concomitant chemotherapy).
However, assuming that IMRT might not be available to every radiation oncologist, the use of neuroprotective drugs before and/or during PCI might be an option. Several drugs are currently studied that may protect the CNS from irradiation damage, whereas the most promising data were obtained for peroxisome proliferator-activated agonists, angiotensin-converting enzyme inhibitors and angiotensin type-1 receptor blockers, which have shown to ameliorate radiation-induced injuries in vivo (71).
In the light of potentially reduced toxicities of future PCIs, one may now have to re-evaluate whether there are patient collectives that are at risk for BM but currently do not receive PCI due to toxicity. The key studies that evaluated PCI toxicity analyzed patients of stage IIIA/B, that resemble a collective of lymph node positive, non-metastatic patients with diverse non-small-cell entities (13-16). It is known from early studies in the late 1980s that especially nonsquamous-cell (NSq) carcinomas strongly tend to metastasize to the brain (72) and retrospective analyses and necropsies have revealed that roughly 50% of NSq patients will experience BM (73-75). On the way to find a subgroup in the NSq group that might benefit from PCI, it is important to mention that tumor size and lymph node status are the key determinants for assessing the risk of BM in NSCLC (76,77). In this regard, a very recent analysis by Ding and colleagues revealed that nearly 60% of patients with NSCLC stage IIIA-N2 developed BM within 5 years if more than 30% of all excised lymph nodes were affected (78). If less than 30 % were affected, they saw that roughly 30% of patients had BM. Their data suggest that patients with NSq-NSCLC with N≥2 and >30% affected nodes might benefit from PCI. Consequently, it should now be tested if the (putatively) low toxicities of novel PCI-treatment modalities (such as IMRT) could provide a benefit to this subgroup.
Conclusions and outlook
It is well documented that PCI reduces the incidence of BM and improves overall survival of patients with LD-SCLCL and of patients that showed any response to chemotherapy in ED-SCLC. With the advancement of novel anticancer-therapies, more and more patients that require PCI will survive long term, which will require new approaches to prevent toxicities. Concurrent or preceding chemotherapy as well as higher age are co-impairing cognition in patients receiving PCI, which should be considered in clinical decision making. In the future, novel concepts of prophylactic and therapeutic whole-brain irradiation have to be established for these patients. The techniques will include hippocampus-sparing and scalp-sparing highly conformal prophylactic cranial intensity-modulated radiotherapy (IMRT). Prospective studies will then have to prove similar efficiency as conventional PCI. Finally, assuming reduced rates of future PCI, new studies will have to be set up to re-evaluate whether patients with NSq-NSCLC that are at high risk for BM may eventually benefit of PCI.
Acknowledgements
Disclosure: The authors declare no conflict of interest.
References
- Aur RJ, Verzosa MS, Hustu HO, et al. Response to combination therapy after relapse in childhood acute lymphocytic leukemia. Cancer 1972;30:334-8.
- Clarke M, Gaynon P, Hann I, et al. CNS-directed therapy for childhood acute lymphoblastic leukemia: Childhood ALL Collaborative Group overview of 43 randomized trials. J Clin Oncol 2003;21:1798-809.
- Bleyer WA, Poplack DG. Prophylaxis and treatment of leukemia in the central nervous system and other sanctuaries. Semin Oncol 1985;12:131-48.
- Ochs J, Mulhern R. Long-term sequelae of therapy for childhood acute lymphoblastic leukaemia. Baillieres Clin Haematol 1994;7:365-76.
- Pui CH, Evans WE. Acute lymphoblastic leukemia. N Engl J Med 1998;339:605-15.
- Pui CH, Cheng C, Leung W, et al. Extended follow-up of long-term survivors of childhood acute lymphoblastic leukemia. N Engl J Med 2003;349:640-9.
- Walter AW, Hancock ML, Pui CH, et al. Secondary brain tumors in children treated for acute lymphoblastic leukemia at St Jude Children’s Research Hospital. J Clin Oncol 1998;16:3761-7.
- Creutzig U, Ritter J, Zimmermann M, et al. Does cranial irradiation reduce the risk for bone marrow relapse in acute myelogenous leukemia? Unexpected results of the Childhood Acute Myelogenous Leukemia Study BFM-87. J Clin Oncol 1993;11:279-86.
- Larsen E, Raetz EA, Winick NJ, et al. Outcome in adolescent and young adult (AYA) patients compared with younger patients treated for high-risk B-precursor acute lymphoblastic leukemia (HR-ALL): A report from the Children’s Oncology Group study AALL0232. J Clin Oncol 2012;30:abstr CRA9508.
- Komaki R, Cox JD, Whitson W. Risk of brain metastasis from small cell carcinoma of the lung related to length of survival and prophylactic irradiation. Cancer Treat Rep 1981;65:811-4.
- Aupérin A, Arriagada R, Pignon JP, et al. Prophylactic cranial irradiation for patients with small-cell lung cancer in complete remission. Prophylactic Cranial Irradiation Overview Collaborative Group. N Engl J Med 1999;341:476-84.
- Slotman B, Faivre-Finn C, Kramer G, et al. Prophylactic cranial irradiation in extensive small-cell lung cancer. N Engl J Med 2007;357:664-72.
- Gore EM, Bae K, Wong SJ, et al. Phase III comparison of prophylactic cranial irradiation versus observation in patients with locally advanced non-small-cell lung cancer: primary analysis of radiation therapy oncology group study RTOG 0214. J Clin Oncol 2011;29:272-8.
- Pöttgen C, Eberhardt W, Grannass A, et al. Prophylactic cranial irradiation in operable stage IIIA non small-cell lung cancer treated with neoadjuvant chemoradiotherapy: results from a German multicenter randomized trial. J Clin Oncol 2007;25:4987-92.
- Russell AH, Pajak TE, Selim HM, et al. Prophylactic cranial irradiation for lung cancer patients at high risk for development of cerebral metastasis: results of a prospective randomized trial conducted by the Radiation Therapy Oncology Group. Int J Radiat Oncol Biol Phys 1991;21:637-43.
- un A, Bae K, Gore EM, et al. Phase III trial of prophylactic cranial irradiation compared with observation in patients with locally advanced non-small-cell lung cancer: neurocognitive and quality-of-life analysis. J Clin Oncol 2011;29:279-86.
- Andre F, Grunenwald D, Pujol JL, et al. Patterns of relapse of N2 nonsmall-cell lung carcinoma patients treated with preoperative chemotherapy: should prophylactic cranial irradiation be reconsidered? Cancer 2001;91:2394-400.
- Gaspar LE, Chansky K, Albain KS, et al. Time from treatment to subsequent diagnosis of brain metastases in stage III non-small-cell lung cancer: a retrospective review by the Southwest Oncology Group. J Clin Oncol 2005;23:2955-61.
- Schrappe M, Reiter A, Zimmermann M, et al. Long-term results of four consecutive trials in childhood ALL performed by the ALL-BFM study group from 1981 to 1995. Berlin-Frankfurt-Münster. Leukemia 2000;14:2205-22.
- Waber DP, Turek J, Catania L, et al. Neuropsychological outcomes from a randomized trial of triple intrathecal chemotherapy compared with 18 Gy cranial radiation as CNS treatment in acute lymphoblastic leukemia: findings from Dana-Farber Cancer Institute ALL Consortium Protocol 95-01. J Clin Oncol 2007;25:4914-21.
- Suwinski R, Lee SP, Withers HR. Dose-response relationship for prophylactic cranial irradiation in small cell lung cancer. Int J Radiat Oncol Biol Phys 1998;40:797-806.
- Gregor A, Cull A, Stephens RJ, et al. Prophylactic cranial irradiation is indicated following complete response to induction therapy in small cell lung cancer: results of a multicentre randomised trial. United Kingdom Coordinating Committee for Cancer Research (UKCCCR) and the European Organization for Research and Treatment of Cancer (EORTC). Eur J Cancer 1997;33:1752-8.
- Gregor A. Prophylactic cranial irradiation in small-cell lung cancer: is it ever indicated? Oncology (Williston Park) 1998;12:19-24.
- Le Péchoux C, Dunant A, Senan S, et al. Standard-dose versus higher-dose prophylactic cranial irradiation (PCI) in patients with limited-stage small-cell lung cancer in complete remission after chemotherapy and thoracic radiotherapy (PCI 99-01, EORTC 22003-08004, RTOG 0212, and IFCT 99-01): a randomised clinical trial. Lancet Oncol 2009;10:467-74.
- Stuschke M, Eberhardt W, Pöttgen C, et al. Prophylactic cranial irradiation in locally advanced non-small-cell lung cancer after multimodality treatment: long-term follow-up and investigations of late neuropsychologic effects. J Clin Oncol 1999;17:2700-9.
- Miller TP, Crowley JJ, Mira J, et al. A randomized trial of chemotherapy and radiotherapy for stage III Non-small cell lung cancer. Cancer Therapeutics 1998;1:229-36.
- Sheline GE, Wara WM, Smith V. Therapeutic irradiation and brain injury. Int J Radiat Oncol Biol Phys 1980;6:1215-28.
- Welzel G, Fleckenstein K, Schaefer J, et al. Memory function before and after whole brain radiotherapy in patients with and without brain metastases. Int J Radiat Oncol Biol Phys 2008;72:1311-8.
- Penitzka S, Steinvorth S, Sehlleier S, et al. Assessment of cognitive function after preventive and therapeutic whole brain irradiation using neuropsychological testing. Strahlenther Onkol 2002;178:252-8.
- Merchant TE, Kiehna EN, Li C, et al. Modeling radiation dosimetry to predict cognitive outcomes in pediatric patients with CNS embryonal tumors including medulloblastoma. Int J Radiat Oncol Biol Phys 2006;65:210-21.
- Moore BD 3rd. Neurocognitive outcomes in survivors of childhood cancer. J Pediatr Psychol 2005;30:51-63.
- Mulhern RK, Palmer SL. Neurocognitive late effects in pediatric cancer. Curr Probl Cancer 2003;27:177-97.
- Freeman JE, Johnston PG, Voke JM. Somnolence after prophylactic cranial irradiation in children with acute lymphoblastic leukaemia. Br Med J 1973;4:523-5.
- Kelsey CR, Marks LB. Somnolence syndrome after focal radiation therapy to the pineal region: case report and review of the literature. J Neurooncol 2006;78:153-6.
- Littman P, Rosenstock J, Gale G, et al. The somnolence syndrome in leukemic children following reduced daily dose fractions of cranial radiation. Int J Radiat Oncol Biol Phys 1984;10:1851-3.
- Ochs J, Mulhern R, Fairclough D, et al. Comparison of neuropsychologic functioning and clinical indicators of neurotoxicity in long-term survivors of childhood leukemia given cranial radiation or parenteral methotrexate: a prospective study. J Clin Oncol 1991;9:145-51.
- Miyahara M, Azuma E, Hirayama M, et al. Somnolence syndrome in a child following 1200-cGy total body irradiation in an unrelated bone marrow transplantation. Pediatr Hematol Oncol 2000;17:489-95.
- Soussain C, Ricard D, Fike JR, et al. CNS complications of radiotherapy and chemotherapy. Lancet 2009;374:1639-51.
- Wenz F, Steinvorth S, Lohr F, et al. Prospective evaluation of delayed central nervous system (CNS) toxicity of hyperfractionated total body irradiation (TBI). Int J Radiat Oncol Biol Phys 2000;48:1497-501.
- Clayton PE, Shalet SM. Dose dependency of time of onset of radiation-induced growth hormone deficiency. J Pediatr 1991;118:226-8.
- Clayton PE, Shalet SM. The evolution of spinal growth after irradiation. Clin Oncol (R Coll Radiol) 1991;3:220-2.
- Schmiegelow M, Lassen S, Poulsen HS, et al. Growth hormone response to a growth hormone-releasing hormone stimulation test in a population-based study following cranial irradiation of childhood brain tumors. Horm Res 2000;54:53-9.
- Agha A, Sherlock M, Brennan S, et al. Hypothalamic-pituitary dysfunction after irradiation of nonpituitary brain tumors in adults. J Clin Endocrinol Metab 2005;90:6355-60.
- Constine LS, Woolf PD, Cann D, et al. Hypothalamic-pituitary dysfunction after radiation for brain tumors. N Engl J Med 1993;328:87-94.
- Davis JD, Tremont G. Neuropsychiatric aspects of hypothyroidism and treatment reversibility. Minerva Endocrinol 2007;32:49-65.
- Wefel JS, Lenzi R, Theriault RL, et al. The cognitive sequelae of standard-dose adjuvant chemotherapy in women with breast carcinoma: results of a prospective, randomized, longitudinal trial. Cancer 2004;100:2292-9.
- Welzel G, Steinvorth S, Wenz F. Cognitive effects of chemotherapy and/or cranial irradiation in adults. Strahlenther Onkol 2005;181:141-56.
- Brezden CB, Phillips KA, Abdolell M, et al. Cognitive function in breast cancer patients receiving adjuvant chemotherapy. J Clin Oncol 2000;18:2695-701.
- Grosshans DR, Meyers CA, Allen PK, et al. Neurocognitive function in patients with small cell lung cancer: effect of prophylactic cranial irradiation. Cancer 2008;112:589-95.
- Johnson BE, Becker B, Goff WB 2nd, et al. Neurologic, neuropsychologic, and computed cranial tomography scan abnormalities in 2- to 10-year survivors of small-cell lung cancer. J Clin Oncol 1985;3:1659-67.
- de Ruiter MB, Reneman L, Boogerd W, et al. Cerebral hyporesponsiveness and cognitive impairment 10 years after chemotherapy for breast cancer. Hum Brain Mapp 2011;32:1206-19.
- Deprez S, Amant F, Smeets A, et al. Longitudinal assessment of chemotherapy-induced structural changes in cerebral white matter and its correlation with impaired cognitive functioning. J Clin Oncol 2012;30:274-81.
- Bunn PA, Ridgway EC. Paraneoplastic syndromes. In: DeVita VT, Hellman S, Rosenberg SA, eds. Cancer: Principles and Practice of Oncology, 4 edn. Philadelphia: Lippincott Co. 1993:2026-71.
- Heinemann S, Zabel P, Hauber HP. Paraneoplastic syndromes in lung cancer. Cancer Therapy 2008;6:87-98.
- Graus F, Delattre JY, Antoine JC, et al. Recommended diagnostic criteria for paraneoplastic neurological syndromes. J Neurol Neurosurg Psychiatry 2004;75:1135-40.
- Meyers CA. Neurocognitive dysfunction in cancer patients. Oncology (Williston Park) 2000;14:75-9; discussion 79, 81-2, 85.
- Lee JS, Umsawasdi T, Dhingra HM, et al. Effects of brain irradiation and chemotherapy on myelosuppression in small-cell lung cancer. J Clin Oncol 1986;4:1615-9.
- Lishner M, Feld R, Payne DG, et al. Late neurological complications after prophylactic cranial irradiation in patients with small-cell lung cancer: the Toronto experience. J Clin Oncol 1990;8:215-21.
- Turrisi AT. Brain irradiation and systemic chemotherapy for small-cell lung cancer: dangerous liaisons? J Clin Oncol 1990;8:196-9.
- Daams M, Schuitema I, van Dijk BW, et al. Long-term effects of cranial irradiation and intrathecal chemotherapy in treatment of childhood leukemia: a MEG study of power spectrum and correlated cognitive dysfunction. BMC Neurol 2012;12:84.
- Wolfson AH, Bae K, Komaki R, et al. Primary analysis of a phase II randomized trial Radiation Therapy Oncology Group (RTOG) 0212: impact of different total doses and schedules of prophylactic cranial irradiation on chronic neurotoxicity and quality of life for patients with limited-disease small-cell lung cancer. Int J Radiat Oncol Biol Phys 2011;81:77-84.
- Hopewell JW, Wright EA. The nature of latent cerebral irradiation damage and its modification by hypertension. Br J Radiol 1970;43:161-7.
- Slotman BJ, Mauer ME, Bottomley A, et al. Prophylactic cranial irradiation in extensive disease small-cell lung cancer: short-term health-related quality of life and patient reported symptoms: results of an international Phase III randomized controlled trial by the EORTC Radiation Oncology and Lung Cancer Groups. J Clin Oncol 2009;27:78-84.
- Ghia A, Tomé WA, Thomas S, et al. Distribution of brain metastases in relation to the hippocampus: implications for neurocognitive functional preservation. Int J Radiat Oncol Biol Phys 2007;68:971-7.
- Gondi V, Tolakanahalli R, Mehta MP, et al. Hippocampal-sparing whole-brain radiotherapy: a “how-to” technique using helical tomotherapy and linear accelerator-based intensity-modulated radiotherapy. Int J Radiat Oncol Biol Phys 2010;78:1244-52.
- Budd D, Himmelberger D, Rhodes T, et al. The effects of hair loss in European men: a survey in four countries. Eur J Dermatol 2000;10:122-7.
- Hilton S, Hunt K, Emslie C, et al. Have men been overlooked? A comparison of young men and women’s experiences of chemotherapy-induced alopecia. Psychooncology 2008;17:577-83.
- Lemieux J, Maunsell E, Provencher L. Chemotherapy-induced alopecia and effects on quality of life among women with breast cancer: a literature review. Psychooncology 2008;17:317-28.
- Münstedt K, Manthey N, Sachsse S, et al. Changes in self-concept and body image during alopecia induced cancer chemotherapy. Support Care Cancer 1997;5:139-43.
- Roberge D, Parker W, Niazi TM, et al. Treating the contents and not the container: dosimetric study of hair-sparing whole brain intensity modulated radiation therapy. Technol Cancer Res Treat 2005;4:567-70.
- Greene-Schloesser D, Robbins ME, Peiffer AM, et al. Radiation-induced brain injury: A review. Front Oncol 2012;2:73.
- Figlin RA, Piantadosi S, Feld R. Intracranial recurrence of carcinoma after complete surgical resection of stage I, II, and III non-small-cell lung cancer. N Engl J Med 1988;318:1300-5.
- Bajard A, Westeel V, Dubiez A, et al. Multivariate analysis of factors predictive of brain metastases in localised non-small cell lung carcinoma. Lung Cancer 2004;45:317-23.
- Cox JD, Yesner RA. Adenocarcinoma of the lung: recent results from the Veterans Administration Lung Group. Am Rev Respir Dis 1979;120:1025-9.
- Strauss B, Weller CV. Bronchogenic carcinoma; a statistical analysis of two hundred ninety-six cases with necropsy as to relationships between cell types and age, sex, and metastasis. AMA Arch Pathol 1957;63:602-11.
- Hubbs JL, Boyd JA, Hollis D, et al. Factors associated with the development of brain metastases: analysis of 975 patients with early stage nonsmall cell lung cancer. Cancer 2010;116:5038-46.
- Mujoomdar A, Austin JH, Malhotra R, et al. Clinical predictors of metastatic disease to the brain from non-small cell lung carcinoma: primary tumor size, cell type, and lymph node metastases. Radiology 2007;242:882-8.
- Ding X, Dai H, Hui Z, et al. Risk factors of brain metastases in completely resected pathological stage IIIA-N2 non-small cell lung cancer. Radiat Oncol 2012;7:119.