PD-L1 expression and immune cells infiltration in primary tracheobronchial neoplasm
Introduction
The incidence of primary tracheobronchial neoplasm comprises only 0.2% of respiratory tract neoplasm (1,2), and within this minority, squamous cell carcinoma (SCC) is the predominant pathological pattern, followed by the adenoid cystic carcinoma (ACC). Together, SCC and ACC represent more than 2/3 of primary tracheobronchial neoplasms (3,4). Other histological subtypes, including mucoepidermoid carcinoma, sarcoma, plasmacytoma, lymphoepithelioma etc. are much rare (5). Surgical resection is the most effective treatment for primary tracheobronchial neoplasm (6). The 5-year overall survival (OS) for SCC and ACC has been shown to range from 20% to 39% and 52% to 85%, respectively, in resected patients (7-9). However, due to the limitation of tracheal length or other surgical contraindications, approximately 30% of patients with tracheobronchial neoplasm are unsuitable for surgery (7,10). The 5-year survival rate is 7.3–26% and 33–63.7% in unresectable patients with SCC and ACC, respectively (7-9). Conservative treatments, including endoscopic resection by various techniques, radiotherapy, and chemotherapy may be a favorable choice (11,12). However, the efficacy is still not very satisfactory. Therefore, finding new alternative treatments is of great significance for those patients with unresectable lesions or second relapse.
In recent years, immunotherapy has received increasing attention, which inhibits tumor progression by reactivating the immune system (13,14). Programmed cell death 1 (PD-1) and programmed cell death ligand 1 (PD-L1) are key targets in immunotherapy (15). Currently, the application of PD-1 or PD-L1 inhibitors has been approved in multiple tumors (16), including non-small cell lung cancer (17), head and neck cancer (18), melanoma (19), and breast cancer (20). In recent years, several case reports have shown that patients with tracheobronchial neoplasm can benefit from PD-1 inhibitors, nivolumab and pembrolizumab (21-23). And SCC of primary tracheobronchial neoplasm was found to have significant PD-L1 expression, even 95% of tumor cells (22). This indicated that immunotherapy may be a potential alternative treatment for patients with unresectable tracheobronchial neoplasms.
The immune cell infiltration of tumors is a critical component of the anti-tumor immune response. For example, compared with the absence of CD8+ cells in the tumor immune microenvironment (TIME), a patient with a high level of CD8+ cell infiltration is more likely to benefit from immunotherapy (24). Among several different types of immune cells, the infiltrations of CD8+, FOXP3+, CD16+, and CD68+ cells have been seen as potential biomarkers of clinical response to immunotherapy (25). Further, immune cells in the TIME are also regarded as new prognostic factors in various cancers (26-30). Therefore, understanding the infiltration phenotype of immune cells in the TIME is important for treatment selection and prognosis assessment of patients. However, due to the low incidence of primary tracheobronchial neoplasms, the characterization of infiltration of immune cells phenotype of such tumors is still unclear. Moreover, the relationship between the expression of PD-L1 and immune cells requires further exploration.
In this study, we evaluated the density of immune cells expressing CD8 (a marker of cytotoxic T cells), FOXP3 [a marker of regulatory T cells (Treg)], CD16 (a marker of NK cells), CD68 (a marker of macrophages), and CD163 (a marker of M2 polarized macrophages), and expression of PD-L1 positive tumor cells in primary tracheobronchial neoplasms. The TIME was assessed based on the expression of PD-L1 positive tumor cells and the presence of tumor-infiltrating lymphocytes. The relationship between the immune cells and clinical features of primary tracheobronchial neoplasms was also examined to identify potential candidates who may benefit from immunotherapy. We present the following article in accordance with the REMARK reporting checklist (available at https://dx.doi.org/10.21037/tlcr-21-958).
Methods
Patients
From January 2016 to July 2021, a total of 28 patients underwent surgical resection of tracheobronchial neoplasm at the Tangdu Hospital of Air Force Military Medical University. Due to sample acquisition, 21 patients were included in this study. Baseline characteristics including age, gender, smoking status, size and location of lesions, histopathological types, margin status (R0: no residual tumor; R1: microscopic residual tumor), lymph node metastasis, extension of tumor (E0, tumor confined to the tracheobronchial area or spread outside, but not to adjacent structures; E1, neoplasm had invaded the adjacent structures) (8), postoperative adjuvant therapy, and complications were evaluated. Participants were reexamined every 3 months in the first 2 years after surgery and every subsequent 6 months. This study was conducted in accordance with the ethical guidelines of Declaration of Helsinki (as revised in 2013) and approved by the Ethics Committee of Tangdu Hospital (No. K202111-04). Individual consent for this retrospective analysis was waived.
Immunohistochemistry and image analysis
Consecutive tissue sections (3 µm thick) were obtained from formalin-fixed and paraffin-embedded primary tracheobronchial neoplasm samples stored in the pathology department. And the automated staining system (Ventana, Bench Mark XT, America) was used for immunohistochemical staining (IHC). Deparaffinization and rehydration were conducted under xylene and gradient ethanol, respectively. We used 3% H2O2 to block endogenous peroxidases at room temperature, and heat treatment was performed for antigen retrieval. After being washed in phosphate-buffered saline (PBS), the tissue sections were incubated with CD8 (Abcam, Cambridge, UK; Cat# ab178089, RRID: AB_2756374), CD16 [rabbit monoclonal antibody, Abcam (ab183354)], FOXP3 (Abcam Cat# ab215206, RRID: AB_2860568), CD68 (Abcam Cat# ab213363, RRID: AB_2801637), CD163 (Abcam Cat# ab182422, RRID: AB_2753196) for 1.5 h at room temperature. The tissue sections were incubated with the peroxidase-conjugated secondary antibody for 18 min after washing in PBS for 15 min. Diaminobenzidine was used for chromogen and hematoxylin was used for counterstaining nuclei. Human placental tissue was used as positive controls for PD-L1.
All tissue slides were digitized using Case Viewer 2.4 (3DHISTECH, Budapest, Hungary) after staining. Analysis was performed by 2 pathologists (Li Gong and Xue Wang) who were blind to the histopathological type and clinical dates. The expression of PD-L1 (22C3 clone) was evaluated according to the tumor proportion score (TPS) system based on the guidelines that have been established for non-small cell lung cancer (31). The expression of PD-L1 >1% was defined as positive. For CD8, FOXP3, CD16, CD68, and CD163 staining, the number of positive cells were counted automatically using Image-Pro Plus 6.0 (Media Cybernetics, Rockville, MD, USA), and among the positive cells labeled by Image-Pro Plus software, cells with false positive were manually removed. The intratumoral region (intra) was defined as tumor parenchyma and the peritumoral region (peri) was defined as the stroma surrounding nests of tumor cells (Figure S1) (32). We selected 5 high-powered representative fields (×400) of each region for respective analysis. The density of cells was calculated based on the average number of cells in the 5 fields divided by the area (0.07 mm2/field). The median density of immune cells positive for each maker was used as cut-off value, with a density of immune cells greater than the cut-off value defined as high level infiltration.
Statical analysis
The software SPSS 22.0 (IBM Corp., Armonk, NY, USA) was used for statistical computation. Chi-square and Fisher’s exact tests were used to compare groups on categorical variables. The Wilcoxon rank-sum test and Kruskal-Wallis test were used for evaluating the difference of the expression of immune markers among different groups. Kaplan-Meier method and log-rank test were used for survival analyzed. All statistics were 2-sided and P value less than 0.05 was considered statically significant.
Results
Clinical feature of 21 patients with primary tracheobronchial neoplasm
The baseline characteristics of participants are summarized in Table 1. In total, 21 patients diagnosed with primary tracheobronchial neoplasms who were treated with surgery were included in this cohort. The histopathology of 21 patients included ACC (16/21) and SCC (5/21). The median age of participants with ACC was 42 years (27–64 years), which was younger than that of patients with SCC. The gender ratio was about 2:1 (10 males vs. 6 females) in patients with ACC. Males comprised the majority of patients with SCC. Compared to those with ACC, most SCC participants were smokers, while the percentage of smokers in ACC was 31.25%. Cough was the most frequent symptom in these patients, following by dyspnea and hemoptysis. Among all 21 participants, none had a personal history of neoplasia, and only 1 participant with SCC had a family history of lung cancer (brother). Lymph node metastasis was observed in 3 participants (2 patients with ACC and 1 patient with SCC). The E1 extension was observed in 8 patients with ACC and 1 patient with SCC. Postoperative adjuvant therapy was performed in 10 patients.
Table 1
Variable | ACC (n=16) | SCC (n=5) |
---|---|---|
Age, years, median [range] | 42 [27–64] | 56 [37–73] |
Gender, n (%) | ||
Male | 10 (62.50) | 4 (80.00) |
Female | 6 (37.50) | 1 (20.00) |
Smoker, n (%) | ||
Yes | 5 (31.25) | 4 (80.00) |
No | 11 (68.75) | 1 (20.00) |
Presentations, n (%) | ||
Cough | 10 (62.50) | 3 (60.00) |
Dyspnea | 9 (56.25) | 2 (40.00) |
Hemoptysis | 4 (25) | 2 (40.00) |
Tumor size, n (%) | ||
≤3 cm | 9 (56.25) | 4 (80.00) |
>3 cm | 7 (43.75) | 1 (20.00) |
Location, n (%) | ||
Trachea | 12 (75.00) | 2 (40.00) |
Main bronchus | 4 (25.00) | 2 (40.00) |
Carinal | 0 | 1 (20.00) |
Lymph nodes metastasis, n (%) | ||
Negative | 14 (87.50) | 4 (80.00) |
Positive | 2 (12.50) | 1 (20.00) |
Extension, n (%) | ||
E0 | 8 (50.00) | 4 (80.00) |
E1 | 8 (50.00) | 1 (20.00) |
Margin, n (%) | ||
R0 | 12 (75.00) | 5 (100.00) |
R1 | 4 (25.00) | 0 |
Complications, n (%) | ||
Anastomotic fistula | 4 (25.00) | 0 |
Chylothorax | 1 (6.25) | 1 (20.00) |
None | 11 (68.75) | 4 (80.00) |
Adjuvant therapy, n (%) | ||
Radiotherapy | 1 (6.25) | 0 |
Chemotherapy | 6 (37.50) | 3 (60.00) |
None | 9 (56.25) | 2 (40.00) |
Distant metastasis, n (%) | 2 (12.50) | 2 (40.00) |
ACC, adenoid cystic carcinoma; SCC, squamous cell carcinoma
All patients had recovered from surgery and were discharged without deaths. The median follow-up was 24.8 (1.6–45.6) months and 35.8 (2.6–51.9) months for SCC and ACC participants, respectively. At the time of analysis, 4 participants were recorded with distant metastasis, including 2 participants with ACC and 2 participants with SCC. In ACC, both of the metastases were in the lung, at 12.6 and 18.7 months, respectively. The metastasis in mediastinal lymph nodes and lung had occurred in SCC at 3.1 and 23.4 months postoperatively, respectively.
PD-L1 expression and immune cell infiltration in primary tracheobronchial neoplasm
The immunogenic features of ACC compared with SCC are showed in the Table 2 and representative images of the stained immune cells and PD-L1 are shown in Figure 1. A significant difference in the expression of PD-L1 was observed between ACC and SCC (P=0.001). All of 16 participants with ACC had no expression of PD-L1, whereas 4/5 (80%) of those with SCC were positive for PD-L1 expression, which was 6%, 10%, 20%, and 90%, respectively, based on the TPS. In the peritumoral region, the density of cells expressing CD16 was significantly higher in the SCC group than that in the ACC group (P<0.01) (Table 2). In both intratumoral and peritumoral regions, the density of cells expressing FOXP3 were significantly higher in the SCC group (P<0.01) (Table 2). In contrast, no significant difference was observed in the density of cells expressing CD8, CD68, and CD163 between SCC and ACC, neither in the intratumoral region nor peritumoral region (Table 2).
Table 2
Immunomarker | ACC (n=16) | SCC (n=5) | P value |
---|---|---|---|
Expression of PD-L1, n (%) | 0.001* | ||
≥1% | 0 | 4 (80.0) | |
<1%, or no expression | 16 (100.0) | 1 (20.0) | |
CD8+ cell density, /mm2 | |||
Intra | 168.5 (33–834) | 429 (51–720) | 0.368 |
Peri | 251 (86–1,385) | 897 (169–1,243) | 0.056 |
CD16+ cell density, /mm2 | |||
Intra | 161.5 (54–1,382) | 220 (89–991) | 0.093 |
Peri | 170 (54–1,100) | 1134 (254–1,477) | 0.003* |
CD68+ cell density, /mm2 | |||
Intra | 403 (137–1,129) | 469 (203–1,763) | 0.368 |
Peri | 351.5 (91–1,514) | 1209 (206–2,583) | 0.093 |
CD163+ cell density, /mm2 | |||
Intra | 187 (6–954) | 317 (71–1,011) | 0.263 |
Peri | 180 (20–797) | 460 (80–1,000) | 0.181 |
FOXP3+ cell density, /mm2 | |||
Intra | 54 (0–1,094) | 1,271 (871–2,763) | 0.002* |
Peri | 80 (0–357) | 477 (140–760) | 0.005* |
Cell density was showed as median (range). *, the difference was significant. ACC, adenoid cystic carcinoma; SCC, squamous cell carcinoma; Intra, intratumoral region; Peri, peritumoral region.
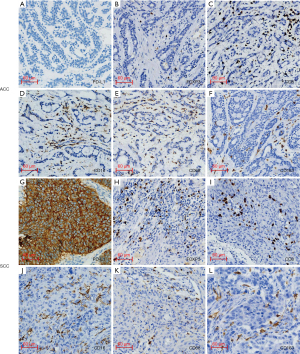
The comparison among different kinds of immune cells in primary tracheobronchial neoplasm is shown in Figure 2. In the ACC group, the density of CD68+ cells were significantly higher than that of CD163+ cells in the intratumoral region (P<0.01) and peritumoral region (P<0.05) and CD8+ cells in the intratumoral region (P<0.05) (Figure 2A). Similarly, the density of FOXP3+ cells were significantly lower than that of CD8+, CD16+, and CD68+ cells in the intratumoral region (P<0.01) and peritumoral region (P<0.01 and P<0.05) (Figure 2A). However, no significant differences were observed among the distribution of each kind of immune cell in the intratumoral or peritumoral region (Figure 2B). In the intratumoral region of the SCC group, the density of FOXP3+ cells were significantly higher than that of CD8+ cells, CD16+ cells, and CD163+ cells (P<0.01 and P<0.05) (Figure 2C). Further, the density of FOXP3+ cells located in the intratumoral region was higher than that in the peritumoral region (P<0.01) (Figure 2D).
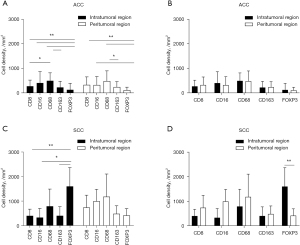
Relationship between the infiltration of immune cells and prognosis of tracheobronchial neoplasm
As shown in Figure 3A, the densities of cells expressing CD16 in the intratumoral region was higher in participants without metastasis than in those with metastasis in the ACC group (P<0.05). In contrast, no significant differences were observed between the density of CD16+ cells and metastasis or extratracheal invasion in the peritumoral region (Figure 3A,3B). The differences between the density of cells expressing CD8, CD68, CD163, or FOXP3 and patients with or without metastasis were not significant (P>0.05) in ACC (Figure 3A). As shown in Figure 3B, participants with or without extratracheal invasion were not associated with the infiltration of immune cells (P>0.05). No statistical analysis was performed on the relationship between the density of immune cells and metastasis or extratracheal invasion, given the small sample size of SCC. The Kaplan–Meier curves showed that the degree of immune cell infiltration had no significant effects on the disease-free survival (DFS) and the OS of tracheobronchial neoplasm (P>0.05) (Figures S2-S5).
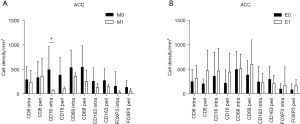
The immune status of primary tracheobronchial neoplasm
According to the location of the infiltration of CD8+ cells, (i.e., intratumoral or peritumoral region), the immune status was defined as immune inflamed, immune excluded, and immune desert (Figure 4A). The results demonstrated that there was no difference of immune status between ACC and SCC (P>0.05) (Figure 4B). In the SCC group, 2 of 5 cases were in the immune excluded, 2 cases were in immune inflamed, and immune desert was observed in 1 case (Figure 4B). And the distribution of immune status might be associated with smoking history, although the difference was not significantly (P=0.082). In the ACC group, the tumors under immune inflamed (n=6) and immune desert were equal (n=6), and 25% of participants (n=4) with ACC were immune excluded (Figure 4B).
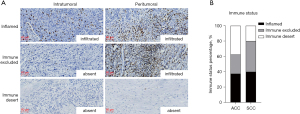
Furthermore, the relationship between the immune status and metastasis or extratracheal invasion were analyzed. All of the 5 participants (ACC, n=3; SCC, n=2) with metastasis (either lymph nodes metastasis or distant metastasis) were in the state of immune excluded or immune desert (Figure 5A,5B). Specifically, both participants with SCC and 2 of 3 participants with ACC were in the state of immune excluded, and the other 1 was in immune desert. When looking at extratracheal invasion, the results showed that the status of immune excluded accounted for 50% of participants with ACC (Figure 5C). In contrast, immune desert was the main status of the immune microenvironment in patients with extratracheal invasion in SCC (Figure 5D).
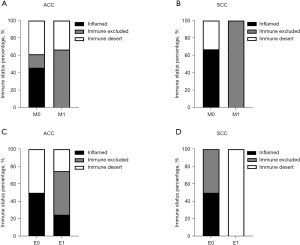
Immunotherapy candidates of primary tracheobronchial neoplasm
To develop a better immunotherapy strategy for patients with tracheobronchial neoplasm, the immune microenvironment was defined as 4 types based on the expression of PD-L1 and the CD8+ cell density intratumorally. The representative images of PD-L1 and CD8+ cell density in the 4 immunotypes are shown in Figure 6A. The expression of PD-L1 >1% and the median CD8+ cell density was used as the cutoff. As shown in Figure 6B, most of the SCC cases were in the immunotype I (PD-L1+/CD8+, adaptive immune resistance), accounting for 60%. On the contrary, immunotype I was not observed in ACC cases; immunotype II (PD-L1−/CD8−, immunologic ignorance) or immunotype IV (PD-L1−/CD8+, immune tolerance) were the main immunotypes in ACC cases. Immunotype III (PD-L1+/CD8−, intrinsic induction) was only observed in SCC case.
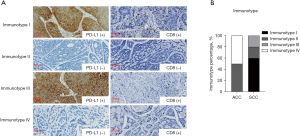
Discussion
Immune microenvironment plays an important role in the development and progression of tumors, in which the immune cells have been regarded as treatment response markers and prognostic factors (33-37). In this study, based on current literature, several specific types of immune cells were analyzed, including CD8+ T cells, CD16+ cells, FOXP3+ Treg cells, CD68+ macrophages, and CD163+, indicating M2 macrophages. Currently, microsatellite instability (MSI), PD-L1 expression and tumor mutational burden (TMB) play important roles as predictive biomarkers for immunotherapy. However, the relationship between MSI, PD-L1 expression and TMB is different in different tumor types (38). Different tumor types should select different biomarkers (even combination) for evaluating immunotherapy response. In NSCLC, the PD-L1 expression is approved as biomarker for determining usage of immunotherapy. Therefore, in this study, the PD-L1 expression in primary tracheobronchial was analyzed. To our knowledge, this is the first study to systemically describe the immune cells infiltration in primary tracheobronchial neoplasm.
The expression of PD-L1 varies widely in different solid tumors, ranging from 0% to 100% (39). A previous study demonstrated that the expression of PD-L1 >1% in primary tracheal tumors with a predominant squamous cell component was 50%, and salivary-type tracheal tumors without any expression of PD-L1 (40). In this study, the expression of PD-L1 accounted for 80% of the SCC group, whereas ACC did not show any degree of PD-L1 expression. Overexpression of PD-L1 indicated poor clinical outcome in some studies (39,41,42). In this study, PD-L1 expression was positive in 2/2 SCC with distant metastases that the expression levels of PD-L1 was 10% and 90% and in 1/1 patient with lymph node metastasis that the expression levels of PD-L1 was 10%. Based on the staging system proposed by Bhattacharyya N (43), 3/3 of patients in stage I–II and 1/2 of patients in stage IV have high expression of PD-L1 (>1%). And in this study, no significant relationship is observed between the expression of PD-L1 and the staging of SCC of primary tracheobronchial neoplasm. And PD-L1 overexpression also means that the patient may be sensitive to PD-L1 inhibitor. There have been 2 case reports showing the successful use of immune therapy in tracheal SCC with significant PD-L1 expression (21,22). Specifically, the patients were treated with nivolumab and pembrolizumab respectively, and both of them obtained completed tumor response after several months. These results indicated that the expression of PD-L1 should be examined in SCC. And due to the heterogeneity of tumor, the immune escape of tumor can occur through different immune checkpoints. Therefore, patients with low expression of PD-L1 can combine other immune checkpoint inhibitors. For example, in melanoma, combining anti-cytotoxic T lymphocyte associated antigen 4 (anti-CTLA) inhibitor and anti-PD-1 inhibitor showed better therapeutic effects than that of single immune checkpoint inhibitor (44). Although more evidence is needed to support the actual effect of immune checkpoint inhibitor, patients with primary tracheobronchial squamous cell neoplasm who have failed to other therapies could try to use immune checkpoint inhibitor for treatment.
However, the responses to the anti-PD-L1/PD-1 immune checkpoint inhibitors not only depended on the expression of PD-L1, but on a variety of factors, such as the infiltration of CD8+ T cells. The TIME has been divided into 4 categories based on the expression of PD-L1 and the infiltration of CD8+ T cells (24). The main immunotype in the SCC group was immunotype I (PD-L1+/CD8+), which was considered the group that was most likely to benefit from single-agent checkpoint blockade. The high level of pre-existing CD8+ T cells intratumorally was the main contributor to response to anti-PD-L1/PD-1 therapy. Immunotype III (PD-L1+/CD8−) is another immune status with PD-L1 overexpression; however, different from immunotype I, a PD-L1/PD-1 inhibitor alone would not elicit response to treatment. In this situation, immunotherapy combined with stimulating CD8+ cell infiltration is a good option. Radiation has been reported to enhance the number of CD8+ cell infiltration in tissue (45). Therefore, anti-PD-1/PD-L1 combined with radiotherapy may be an optional treatment strategy for patients with immunotype III. In this study, 25% of SCC group participants were in immunotype III, which means that evaluating the infiltration of CD8+ T cells, rather than the expression of PD-L1, is necessary for primary tracheobronchial squamous cell neoplasm before conducting immunotherapy.
In the ACC group, the distribution of immunotypes was equal (immunotype II 50% vs. immunotype IV 50%). Besides anti-PD-1/PD-L1 or anti-CTL4 therapy, inducing CD8+ cell infiltration was a critical part in the therapeutic strategy for immunotype II (PD-L1−/CD8−). In contrast, instead of anti-PD1/PD-L1, patients in immunotype IV (PD-L1−/CD8+) should consider other immunosuppressive pathways, including vaccines and metabolites. Therefore, the treatment for primary tracheobronchial ACC may be focused on stimulating CD8+ cells infiltration and some novel immunosuppressants. Based on the cancer immunity cycle, the immune cells was activated and migrated from immune organ to tumor bed through various factors, including stimulatory factors, such as IL-1, TNF-α, CD28/B7-1, OX40/OX40L, CX3CL1, CXCL9, LFA1/ICAM1, etc., and inhibitory factors, such as IL-10, IL-4, CTLA4/B7-1, PD-L1/PD-1, LAG-3, IDO, TGF-β, etc. (46). And CD8+ cell can be recruited by the secretion of CXCL11, which may be a direction for stimulating CD8+ cells infiltration (47).
Whether CD8+ T cells can play an anti-tumor role depends on whether they can effectively infiltrate into the tumor parenchyma. Therefore, based on spatial distribution of CD8+ cells, the tumor microenvironment was defined as 3 immune statuses, including inflamed tumor, immune-excluded tumor, and immune-desert tumor (48). The result showed that 4 of 5 patients with metastasis were of immune excluded status, which means that CD8+ cells had accumulated in stroma rather than infiltrating into tumor parenchyma. These results indicated that patients with immune excluded status were more like to experience metastasis. Therefore, both the density and location of CD8+ cells infiltration should be considered when evaluating the TIME of primary tracheobronchial neoplasm.
The cluster of differentiation molecule CD16 (Fcγ receptor III), expressing on natural killer (NK) cells, macrophages, and neutrophils, plays an important role in antibody-dependent cell-mediated cytotoxicity (ADCC) (49). When the antibodies secreted by plasma cells bind specifically to the target cells, NK cells can bind to the Fc segment of the antibody through CD16, and then release perforin to kill the target cells. Furtherly, the migratory pattern of NK cell is associated with a downregulation of CX3C chemokine receptor 1 (CX3CR1) and sphingosine-1-phosphate receptor 1 (S1PR1) and overexpression of C-X-C chemokine receptor type 6 (CXCR6) and C-X-C chemokine receptor type 5 (CXCR5) (50). And a study showed that the depletion of regulatory T cells mediated by ADCC has been regarded a possible mechanism of action of anti-CTLA-4 antibody therapy (51). Further, CD16+ cells have been significantly associated with response to ipilimumab treatment in patients with metastatic melanoma (25). In this study, in the intratumoral region, the density of CD16+ cell was significantly lower in participants with metastasis than those without metastasis (P<0.05). These results demonstrated that CD16+ cell infiltration represented a favorable prognostic factor. Furthermore, high level of CD16+ cell infiltration correlates with CD8+ cell infiltration in colorectal cancer, indicating that CD16+ cells may be able to trigger T-cell response (52). Therefore, stimulating the infiltration of CD16+ cells could be an important treatment for improving the curative effect and prognosis of primary tracheobronchial neoplasm.
Tumor-associated macrophages (TAM) are important components of the TIME, among which CD68 is a characteristic generic macrophage maker and CD163 is the marker for M2 macrophages (53). And colony-stimulating factor-1 receptor (CSF1R)-mediated signaling is critical for the survival and differentiation of TAM (54). Some studies have reported that higher density of TAM infiltration is associated with poor prognosis. For example, in laryngeal SCC, weak CD68+ and CD163+ cell infiltration presented with early tumor stage compared with advance tumor stage (55). Furthermore, strong CD68+ cell infiltration was observed in the majority of patients who relapsed (55). However, in contrast, ACC patients with metastasis exhibited lower-level density of CD68+ cells and CD163+ cells infiltration, although the difference was not significant. This was similar to the results of a study of duodenal cancers, which showed that CD68+ TAMs were a positive prognostic factor (56). The positive prognostic function of macrophages has also been observed in human colorectal cancer (57). Therefore, larger participant cohorts are necessary to further explore the function of TAM in primary tracheobronchial neoplasm.
Overexpression of FOXP3+ cells has been found to indicate an immunosuppressive microenvironment, and FOXP3 is regarded as the most accurate maker of Tregs (41). And FOXP3+ Treg cell will be recruited by the high expression of chemokine CCL22 and CCL17, and TGF-β activation can further promote Treg differentiation and inhibit T cell activation (58). Toss et al. (41) and Li et al. (59) showed that higher infiltration of FOXP3+ cells was significantly associated with decreased recurrence-free time and OS in breast cancer. Previous studies have also demonstrated that Tregs are associated with worse OS in uveal melanoma (60) In this study, the density of FOPX3+ cells in ACC were significantly lower than that of FOPX3+ cells in SCC. Furthermore, the density of cells expressing FOXP3 was significantly lower than that of cells expressing CD8, CD16, and CD68 in ACC. However, the density of cells expressing FOXP3 was significantly higher than that of cells expressing CD8, CD16, and CD163 in SCC. These results indicated that the TIME between ACC and SCC of primary tracheobronchial neoplasm was significantly different.
It should be noted that there were several limitations to this study. Firstly, due to the low incidence of tracheobronchial neoplasm, the sample size was small. Larger cohorts are needed to further confirm our results. Secondly, only ACC and SCC of primary tracheobronchial neoplasm were included in this study. Recently, a study showed that patients with primary tracheal lymphoepithelioma-like neoplasm and primary tracheal large-cell neuroendocrine neoplasm can also benefit from immunotherapy (23). Therefore, other than ACC and SCC, more pathological types of primary tracheobronchial neoplasm should be included in subsequent studies.
Conclusions
In primary tracheobronchial neoplasm, the expression of PD-L1 was mainly concentrated among patients with SCC, whereas patients with ACC did not show any expression of PD-L1. The infiltrations of immune cells in primary tracheobronchial neoplasm were significantly different between ACC and SCC. Compared with other immune cells, the density of FOXP3+ cells were the highest in SCC, while the infiltration degree of FOPX3+ cells were the lowest in ACC. The CD68+ cells were the main immune cells in the TIME of ACC. Notably, the location of CD8+ cells infiltration is an important factor in tumor progression, in which immune excluded and immune desert were more common in primary tracheobronchial neoplasm with metastasis. Therefore, immunotherapy should be realized as a potential component of treatment strategies for patients with primary tracheobronchial neoplasm. The infiltration of immune cells should be considered for immunotherapy and prognosis.
Acknowledgments
The authors appreciate the academic support from the AME Thoracic Surgery Collaborative Group. And the authors also appreciate the pathologist (Xue Wang) for IHC analysis.
Funding: This work was supported by the National Natural Science Foundation of China (82070101, 82002421); the Everest Project Fund of The Air Force Medical University (2020); The Science and Technology Innovation Fund (2019QYTS004); and the Top Talent Fund of Tangdu Hospital (2018).
Footnote
Reporting Checklist: The authors have completed the REMARK reporting checklist. Available at https://dx.doi.org/10.21037/tlcr-21-958
Data Sharing Statement: Available at https://dx.doi.org/10.21037/tlcr-21-958
Conflicts of Interest: All authors have completed the ICMJE uniform disclosure form (available at https://dx.doi.org/10.21037/tlcr-21-958). PH serves as an unpaid editorial board member of Translational Lung Cancer Research from March 2021 to February 2023. GM serves as an unpaid editorial board member of Translational Lung Cancer Research from August 2021 to July 2023. Dr. MT reports personal fees from MSD, Ono Pharmaceutical, Bristol-Myers Squibb Co. Ltd., Chugai Pharmaceutical, and AstraZeneca KK. The other authors have no conflicts of interest to declare.
Ethical Statement: The authors are accountable for all aspects of the work in ensuring that questions related to the accuracy or integrity of any part of the work are appropriately investigated and resolved. This study was conducted in accordance with the ethical guidelines of Declaration of Helsinki (as revised in 2013). The study was approved by the Ethics Committee of Tangdu Hospital (No. K202111-04). Individual consent for this retrospective analysis was waived.
Open Access Statement: This is an Open Access article distributed in accordance with the Creative Commons Attribution-NonCommercial-NoDerivs 4.0 International License (CC BY-NC-ND 4.0), which permits the non-commercial replication and distribution of the article with the strict proviso that no changes or edits are made and the original work is properly cited (including links to both the formal publication through the relevant DOI and the license). See: https://creativecommons.org/licenses/by-nc-nd/4.0/.
References
- Webb BD, Walsh GL, Roberts DB, et al. Primary tracheal malignant neoplasms: the University of Texas MD Anderson Cancer Center experience. J Am Coll Surg 2006;202:237-46. [Crossref] [PubMed]
- Junker K. Pathology of tracheal tumors. Thorac Surg Clin 2014;24:7-11. [Crossref] [PubMed]
- Zhengjaiang L, Pingzhang T, Dechao Z, et al. Primary tracheal tumours: 21 years of experience at Peking Union Medical College, Beijing, China. J Laryngol Otol 2008;122:1235-40. [Crossref] [PubMed]
- Moores D, Mane P. Pathology of Primary Tracheobronchial Malignancies Other than Adenoid Cystic Carcinomas. Thorac Surg Clin 2018;28:149-54. [Crossref] [PubMed]
- Mallick S, Benson R, Giridhar P, et al. Demography, patterns of care and survival outcomes in patients with malignant tumors of trachea: A systematic review and individual patient data analysis of 733 patients. Lung Cancer 2019;132:87-93. [Crossref] [PubMed]
- Grillo HC. Tracheal tumors: surgical management. Ann Thorac Surg 1978;26:112-25. [Crossref] [PubMed]
- Gaissert HA, Grillo HC, Shadmehr MB, et al. Long-term survival after resection of primary adenoid cystic and squamous cell carcinoma of the trachea and carina. Ann Thorac Surg 2004;78:1889-96; discussion 1896-7. [Crossref] [PubMed]
- Wang Y, Cai S, Gao S, et al. Tracheobronchial Adenoid Cystic Carcinoma: 50-Year Experience at the National Cancer Center, China. Ann Thorac Surg 2019;108:873-82. [Crossref] [PubMed]
- Abbate G, Lancella A, Contini R, et al. A primary squamous cell carcinoma of the trachea: case report and review of the literature. Acta Otorhinolaryngol Ital 2010;30:209. [PubMed]
- Grillo HC, Mathisen DJ. Primary tracheal tumors: treatment and results. Ann Thorac Surg 1990;49:69-77. [Crossref] [PubMed]
- Macchiarini P. Primary tracheal tumours. Lancet Oncol 2006;7:83-91. [Crossref] [PubMed]
- Sherani K, Vakil A, Dodhia C, et al. Malignant tracheal tumors: a review of current diagnostic and management strategies. Curr Opin Pulm Med 2015;21:322-6. [Crossref] [PubMed]
- Steven A, Fisher SA, Robinson BW. Immunotherapy for lung cancer. Respirology 2016;21:821-33. [Crossref] [PubMed]
- Kennedy LB, Salama AKS. A review of cancer immunotherapy toxicity. CA Cancer J Clin 2020;70:86-104. [Crossref] [PubMed]
- O'Donnell JS, Teng MWL, Smyth MJ. Cancer immunoediting and resistance to T cell-based immunotherapy. Nat Rev Clin Oncol 2019;16:151-67. [Crossref] [PubMed]
- Thompson JA. New NCCN Guidelines: Recognition and Management of Immunotherapy-Related Toxicity. J Natl Compr Canc Netw 2018;16:594-6. [Crossref] [PubMed]
- Doroshow DB, Sanmamed MF, Hastings K, et al. Immunotherapy in Non-Small Cell Lung Cancer: Facts and Hopes. Clin Cancer Res 2019;25:4592-602. [Crossref] [PubMed]
- Gavrielatou N, Doumas S, Economopoulou P, et al. Biomarkers for immunotherapy response in head and neck cancer. Cancer Treat Rev 2020;84:101977. [Crossref] [PubMed]
- Blank CU, Rozeman EA, Fanchi LF, et al. Neoadjuvant versus adjuvant ipilimumab plus nivolumab in macroscopic stage III melanoma. Nat Med 2018;24:1655-61. [Crossref] [PubMed]
- Emens LA. Breast Cancer Immunotherapy: Facts and Hopes. Clin Cancer Res 2018;24:511-20. [Crossref] [PubMed]
- Osho AA, Azzoli CJ, Pai S, et al. Successful Treatment of an Aggressive Tracheal Malignancy With Immunotherapy. Ann Thorac Surg 2017;103:e123-5. [Crossref] [PubMed]
- Maller B, Kaszuba F, Tanvetyanon T. Complete tumor response of tracheal squamous cell carcinoma after treatment with pembrolizumab. Ann Thorac Surg 2019;107:e273-4. [Crossref] [PubMed]
- Jiang BY, Zhang JT, Yan LX, et al. Neoadjuvant immune checkpoint inhibitor plus chemotherapy in rare tracheal tumors. Cancer Commun (Lond) 2021;41:1243-5. [Crossref] [PubMed]
- Teng MW, Ngiow SF, Ribas A, et al. Classifying Cancers Based on T-cell Infiltration and PD-L1. Cancer Res 2015;75:2139-45. [Crossref] [PubMed]
- Balatoni T, Mohos A, Papp E, et al. Tumor-infiltrating immune cells as potential biomarkers predicting response to treatment and survival in patients with metastatic melanoma receiving ipilimumab therapy. Cancer Immunol Immunother 2018;67:141-51. [Crossref] [PubMed]
- Yagi T, Baba Y, Ishimoto T, et al. PD-L1 Expression, Tumor-infiltrating Lymphocytes, and Clinical Outcome in Patients With Surgically Resected Esophageal Cancer. Ann Surg 2019;269:471-8. [Crossref] [PubMed]
- Stasikowska-Kanicka O, Wągrowska-Danilewicz M, Danilewicz M. Immunohistochemical Analysis of Foxp3+, CD4+, CD8+ Cell Infiltrates and PD-L1 in Oral Squamous Cell Carcinoma. Pathol Oncol Res 2018;24:497-505. [Crossref] [PubMed]
- Zhou Z, Mu D, Zhang D, et al. PD-L1 in Combination with CD8+TIL and HIF-1α are Promising Prognosis Predictors of Head and Neck Squamous Cell Carcinoma. Cancer Manag Res 2020;12:13233-9. [Crossref] [PubMed]
- Kesar N, Winkelmann R, Oppermann J, et al. Prognostic impact of CD8-positive tumour-infiltrating lymphocytes and PD-L1 expression in salivary gland cancer. Oral Oncol 2020;111:104931. [Crossref] [PubMed]
- Salmi S, Siiskonen H, Sironen R, et al. The number and localization of CD68+ and CD163+ macrophages in different stages of cutaneous melanoma. Melanoma Res 2019;29:237-47. [Crossref] [PubMed]
- Ferrata M, Schad A, Zimmer S, et al. PD-L1 Expression and Immune Cell Infiltration in Gastroenteropancreatic (GEP) and Non-GEP Neuroendocrine Neoplasms With High Proliferative Activity. Front Oncol 2019;9:343. [Crossref] [PubMed]
- Chen DS, Mellman I. Elements of cancer immunity and the cancer-immune set point. Nature 2017;541:321-30. [Crossref] [PubMed]
- Gonzalez-Ericsson PI, Stovgaard ES, Sua LF, et al. The path to a better biomarker: application of a risk management framework for the implementation of PD-L1 and TILs as immuno-oncology biomarkers in breast cancer clinical trials and daily practice. J Pathol 2020;250:667-84. [Crossref] [PubMed]
- Lei X, Lei Y, Li JK, et al. Immune cells within the tumor microenvironment: Biological functions and roles in cancer immunotherapy. Cancer Lett 2020;470:126-33. [Crossref] [PubMed]
- Pan H, Yu T, Sun L, et al. LncRNA FENDRR-mediated tumor suppression and tumor-immune microenvironment changes in non-small cell lung cancer. Transl Cancer Res 2020;9:3946-59. [Crossref]
- Datar I, Sanmamed MF, Wang J, et al. Expression analysis and Significance of PD-1, LAG-3, and TIM-3 in human non-small cell lung cancer using spatially resolved and multiparametric single-cell analysis. Clin Cancer Res 2019;25:4663-73. [Crossref] [PubMed]
- Peng L, Wang Y, Fei S, et al. The effect of combining Endostar with radiotherapy on blood vessels, tumor-associated macrophages, and T cells in brain metastases of Lewis lung cancer. Transl Lung Cancer Res 2020;9:745-60. [Crossref] [PubMed]
- Luchini C, Bibeau F, Ligtenberg MJL, et al. ESMO recommendations on microsatellite instability testing for immunotherapy in cancer, and its relationship with PD-1/PD-L1 expression and tumour mutational burden: a systematic review-based approach. Ann Oncol 2019;30:1232-43. [Crossref] [PubMed]
- Silva-Figueroa A, Villalobos P, Williams MD, et al. Characterizing parathyroid carcinomas and atypical neoplasms based on the expression of programmed death-ligand 1 expression and the presence of tumor-infiltrating lymphocytes and macrophages. Surgery 2018;164:960-4. [Crossref] [PubMed]
- Tapias LF, Shih A, Mino-Kenudson M, et al. Programmed death ligand 1 and CD8+ immune cell infiltrates in resected primary tracheal malignant neoplasms. Eur J Cardiothorac Surg 2019;55:691-8. [Crossref] [PubMed]
- Toss MS, Abidi A, Lesche D, et al. The prognostic significance of immune microenvironment in breast ductal carcinoma in situ. Br J Cancer 2020;122:1496-506. [Crossref] [PubMed]
- Matsutani S, Shibutani M, Maeda K, et al. Significance of tumor-infiltrating lymphocytes before and after neoadjuvant therapy for rectal cancer. Cancer Sci 2018;109:966-79. [Crossref] [PubMed]
- Bhattacharyya N. Contemporary staging and prognosis for primary tracheal malignancies: a population-based analysis. Otolaryngol Head Neck Surg 2004;131:639-42. [Crossref] [PubMed]
- Topalian SL, Taube JM, Anders RA, et al. Mechanism-driven biomarkers to guide immune checkpoint blockade in cancer therapy. Nat Rev Cancer 2016;16:275-87. [Crossref] [PubMed]
- Du S, Zhou L, Alexander GS, et al. PD-1 Modulates Radiation-Induced Cardiac Toxicity through Cytotoxic T Lymphocytes. J Thorac Oncol 2018;13:510-20. [Crossref] [PubMed]
- Chen DS, Mellman I. Oncology meets immunology: the cancer-immunity cycle. Immunity 2013;39:1-10. [Crossref] [PubMed]
- Gao Q, Wang S, Chen X, et al. Cancer-cell-secreted CXCL11 promoted CD8+ T cells infiltration through docetaxel-induced-release of HMGB1 in NSCLC. J Immunother Cancer 2019;7:42. [Crossref] [PubMed]
- Hegde PS, Chen DS. Top 10 Challenges in Cancer Immunotherapy. Immunity 2020;52:17-35. [Crossref] [PubMed]
- Björkström NK, Gonzalez VD, Malmberg KJ, et al. Elevated numbers of Fc gamma RIIIA+ (CD16+) effector CD8 T cells with NK cell-like function in chronic hepatitis C virus infection. J Immunol 2008;181:4219-28. [Crossref] [PubMed]
- Russick J, Joubert PE, Gillard-Bocquet M, et al. Natural killer cells in the human lung tumor microenvironment display immune inhibitory functions. J Immunother Cancer 2020;8:e001054. [Crossref] [PubMed]
- Simpson TR, Li F, Montalvo-Ortiz W, et al. Fc-dependent depletion of tumor-infiltrating regulatory T cells co-defines the efficacy of anti-CTLA-4 therapy against melanoma. J Exp Med 2013;210:1695-710. [Crossref] [PubMed]
- Sconocchia G, Zlobec I, Lugli A, et al. Tumor infiltration by FcγRIII (CD16)+ myeloid cells is associated with improved survival in patients with colorectal carcinoma. Int J Cancer 2011;128:2663-72. [Crossref] [PubMed]
- Yomoda T, Sudo T, Kawahara A, et al. The Immunoscore is a Superior Prognostic Tool in Stages II and III Colorectal Cancer and is Significantly Correlated with Programmed Death-Ligand 1 (PD-L1) Expression on Tumor-Infiltrating Mononuclear Cells. Ann Surg Oncol 2019;26:415-24. [Crossref] [PubMed]
- Cannarile MA, Weisser M, Jacob W, et al. Colony-stimulating factor 1 receptor (CSF1R) inhibitors in cancer therapy. J Immunother Cancer 2017;5:53. [Crossref] [PubMed]
- Zhou L, Li Y, Gao W, et al. Assessment of tumor-associated immune cells in laryngeal squamous cell carcinoma. J Cancer Res Clin Oncol 2019;145:1761-72. [Crossref] [PubMed]
- Donisi G, Capretti G, Cortese N, et al. Immune infiltrating cells in duodenal cancers. J Transl Med 2020;18:340. [Crossref] [PubMed]
- Malesci A, Bianchi P, Celesti G, et al. Tumor-associated macrophages and response to 5-fluorouracil adjuvant therapy in stage III colorectal cancer. Oncoimmunology 2017;6:e1342918. [Crossref] [PubMed]
- Liu W, Wei X, Li L, et al. CCR4 mediated chemotaxis of regulatory T cells suppress the activation of T cells and NK cells via TGF-β pathway in human non-small cell lung cancer. Biochem Biophys Res Commun 2017;488:196-203. [Crossref] [PubMed]
- Li F, Zhao Y, Wei L, et al. Tumor-infiltrating Treg, MDSC, and IDO expression associated with outcomes of neoadjuvant chemotherapy of breast cancer. Cancer Biol Ther 2018;19:695-705. [Crossref] [PubMed]
- Mougiakakos D, Johansson CC, Trocme E, et al. Intratumoral forkhead box P3-positive regulatory T cells predict poor survival in cyclooxygenase-2-positive uveal melanoma. Cancer 2010;116:2224-33. [Crossref] [PubMed]
(English Language Editor: J. Jones)