Crocin inhibits cell proliferation and enhances cisplatin and pemetrexed chemosensitivity in lung cancer cells
Introduction
Lung cancer is the most common cancer and the leading cause of cancer death in both men and women worldwide. Great advances have been made in cancer therapy by a combination of surgery, chemotherapy, radiotherapy, and molecular-targeted therapy. However, due to increased drug resistance to common cancer drugs, the overall 5-year survival rate of cancer patients is still low. Although it is now widely accepted that the best method to terminate cancer is to prevent it, researchers have been also extensively studying the potential of using plants and medicinal herbs as safe and effective anti-tumor drugs (1).
Crocus sativus, also known as saffron, is a rare and valuable Chinese herbal medicine that has been shown to promote blood circulation and remove blood stasis (2). Saffron is a naturally derived product from the dried stigma of the Crocus sativus flower. Saffron extract and its biologically active compounds, including crocin, crocetin, carotene, and safranal possess anti-oxidative, anti-cancer, anti-inflammatory, and memory-improving properties (3,4). Crocin has shown the property of anti-oxidative (5,6), anti-hyperlipidemia (7), anti-atherosclerotic (8), cardio protective (9), hepatic protective (10), and neuro protective effects (11) in both in vitro and in vivo models. Crocetin has recently gained considerable interest in its capacity to inhibit the initiation, progression, and maintenance of cancer cells (12). Crocetin inhibits cell growth or induces cell death in several malignant cells, including human rhabdomyosarcoma cells (13), pancreatic cancer cells (14), and breast cancer cells (15). These data demonstrate that saffron has a variety of pharmacological effects such as anti-oxidation, immunity enhancement, and anti-tumor (16-20), but the molecular mechanism underlying the anti-tumor effect is largely unknown.
Crocin is a diester formed from the disaccharide gentiobiose and the dicarboxylic acid crocetin (21,22). Crocin is a water-soluble carotenoid (polyene dicarboxylic acid monosaccharide ester). This compound is the major constituent and the main dark red pigment of saffron. Crocin is well known as a potent anti-oxidant agent (23) and hence is being widely used for the treatment of oxidative stress-related disorders. Studies have shown that crocetin induced cancer cell death via p53-dependent and -independent mechanisms in colon cancer cells (24). Moreover, crocin was recently shown to inhibit the proliferation of human hepatocellular carcinoma cells by reducing the human telomerase reverse transcriptase (25). These studies promoted us to postulate that saffron’s components are potential agents for the treatment of lung cancer, the leading cause of cancer death in China.
In this study, we explored the effects of crocin on the proliferation and apoptosis of non-small cell lung cancer cells, e.g., lung adenocarcinoma A549 and SPC-A1 cell lines.
Materials and methods
Cell lines and reagents
Human lung adenocarcinoma A549 and SPC-A1 cells were purchased from American Type Culture Collection (Manassas, VA, USA) and cultured in RPMI 1640 medium (Hyclone, Cramlington, UK) containing 10% bovine calf serum, 100 U/mL penicillin, and 100 µg/mL streptomycin (Gibco, Carlsbad, CA, USA) in an incubator with 5% CO2 at 37 ºC. Crocin was purchased from Sigma-Aldrich (St. Louis, MO, USA) and stored at 4 ºC at a stock concentration of 50 mM in phosphate buffered saline (PBS) with 0.125 mg/mL ethylenediaminetetraacetic acid. Cisplatin and pemetrexed (PMX) were provided from Jiangsu Haosen Pharmaceutical (Jiangsu, China). Apoptosis and cell cycle analysis kits were purchased from Nanjing KeyGen Biotech (Nanjing, China). Reverse transcription- polymerase chain reaction (RT-PCR) kit was purchased from TaKaRa Biotechnology (Dalian, China). PCR primers were synthesized by Sangon Biotech (Shanghai, China). PCR Master Mix reagents were purchased from Promega (Beijing, China).
3-(4,5-dimethylthiazol-2-yl)-2,5-diphenyltetrazolium bromide (MTT) assay
A549 and SPC-A1 cells were seeded in 96-well plates at a density of 5×104 cells/mL in 100 µL medium. Twenty-four hours later, the culture medium was changed to fresh medium with different concentrations of crocin (0, 1, 2, 4, 8 and 16 mg/mL). The cells were synchronized by starvation in serum-free medium before treatment with crocin. Each concentration of crocin was added into 5 wells. After 24, 48, and 72 h, 20 µL MTT (5 mg/mL) was added into each well and then incubated for an additional 4 h at 37 ºC. Following removal of the supernatant, 100 µL crystalline in dimethyl sulfoxide was added to each well. Absorbance (OD) at 570 nm was measured in a microplate reader. The cell proliferation inhibitory rate was calculated according to the following formula: inhibition rate (%) =1− (OD value in treated group − OD value in the group with medium only)/ (OD value in the control group − OD value in the group with medium only) ×100. The 50% inhibitory concentration (IC50) after 48 h crocin treatment was calculated based on MTT assay, which was presented as CA for crocin, CB for cisplatin, and CC for PMX.
Determination of combinative effects
Exponentially growing A549 and SPC-A1 cells were seeded in 96-well plates and treated with drugs after 24 h. Seven groups were defined as follows: Group A, crocin with the concentration of CA (4.12 mg/mL for A549 and 5.28 mg/mL for SPC-A1); Group B, cisplatin with the concentration of CB (2.14 µg/mL for A549 and 3.37 µg/mL for SPC-A1); Group C, cisplatin and crocin with the concentration of CA and CB, respectively, Group D, PMX with the concentration of CC (2.63 µg/mL for A549 and 4.71 µg/mL for SPC-A1), Group E, crocin and PMX with the concentration of CA and CC, respectively, Group F, control group without any drugs, Group G, blank group containing only medium. The effect of drug combination on cell proliferation was expressed as the value of q according to the following formula: q=R(A+B)/(RA+RB-RA×RB), where R(A+B) was the inhibition rate of A and B drugs combined, R(A) was the inhibition rate of A drug, and R(B) was the inhibition rate of B drug. For interpreting the results, it was considered that 0.85< q <1.15, q >1.15, and q <0.85 indicated an additive, a synergistic, or an antagonistic effect of the two drugs, respectively.
Determination of apoptosis and cell cycle by flow cytometry
Human lung adenocarcinoma A549 and SPC-A1 cells were seeded in 6-well plates at a density of 1×105 cells/mL for 24 h. After removal of the supernatant, medium with drugs was added into cells and cultured for 48 h. For detection of apoptosis, cells were collected by trypsinization, washed twice with PBS, suspended in 500 µL binding buffer, and consecutively added 5 µL Annexin V-FITC and 5 µL propidium iodide. Following incubation for 10 min in the dark at room temperature, cells were subjected to flow cytometry analysis. For cell cycle analysis, cells were washed with PBS and fixed with cold 70% ethanol. After washing with PBS, 100 µL ribonuclease A was added to the cells, incubated at 37 ºC for 30 min, and subjected to flow cytometry analysis after adding 400 µL 1× Annexin V Binding Buffer. The red fluorescence was recorded at an excitation wavelength of 488 nm. Each experiment was performed in triplicate.
Detection of the mRNA expression of p53, B-cell lymphoma 2-associated X protein (Bax), and B-cell lymphoma 2 (Bcl-2) by RT-PCR
After cells were treated with drug for 48 h in 6-well plates, total RNA was extracted by one-step method according to the instruction of the TRIzol Plus RNA Kit (Life Technologies, Carlsbad, CA, USA). The concentration and purity of RNA was determined by a DNA/RNA analyzer. Complementary DNA was synthesized by TaKaRa Biotechnology (Dalian, P. R. China) reverse transcriptase. PCR was performed as follows: predenaturation at 94 ºC for 2 min followed by 35 cycles of denaturation at 94 ºC for 1 min, annealing at 57 ºC for 30 s, and extension at 72 ºC for 30 s; And last extension at 72 ºC for 10 min. The primers used for Bax (188 bp), Bcl-2 (356 bp), p53 (455 bp), and β-actin (251 bp) are listed in Table 1. PCR products were run with a 1% agarose gel electrophoresis and imaged by the gel imaging system. The experiment was performed in triplicate.
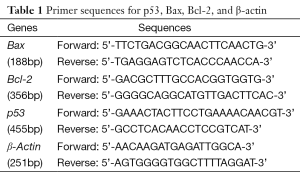
Full table
Statistical analysis
Data were analyzed using SPSS v17.0 software package (SPSS Inc., Chicago, IL, USA) and expressed as mean ± standard deviation. Single factor analysis of variance and multiple comparisons were analyzed by the LDS method. A P-value <0.05 was considered to be statistically significant.
Results
Treatment of A549 and SPC-A1 cells with crocin leads to morphological changes
The shape of A549 cells were either round or oval, and the refractivity under the microscope was weak under normal growth condition. In contrast, after crocin treatment, the cell size was changed from large to small, cells became irregularly contoured, and cell refractivity under the microscope was increased. Changes of these morphologies were more obvious with increasing concentrations of crocin. SPC-A1 cells were spindle-shaped under normal growth condition. After crocin treatment the cell morphology changed significantly with increasing concentrations of crocin, cells were smaller and irregular, and displayed typical apoptotic characteristics. Cell growth was significantly reduced simultaneously with morphological changes. In particular, cells treated with 16 mg/mL of crocin showed a round shape, shrinkage, and decreased refractivity.
Crocin inhibits the proliferation of A549 and SPC-A1 cells
Our results showed that crocin not only changed the morphology but also decreased the cell number of both A549 and SPC-A1 cells. To further confirm these observations, cell proliferation was determined by MTT assay. Crocin significantly inhibited the proliferation of A549 cells in a concentration-dependent manner (Figure 1A). Similar inhibitory effects of crocin were observed in SPC-A1 cells (Figure 1B).
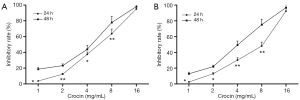
Crocin induces apoptosis in A549 and SPC-A1 cells
To test if crocin reduced cell viability via increased apoptosis, cell apoptosis in A549 and SPC-A1 cells treated with crocin were determined using Annexin V-FITC staining followed by flow cytometry analysis (Figure 2). Interestingly, crocin significantly induced apoptosis in SPC-A1 cells in a dose-dependent manner (Figure 2B,C), but not A549 cells (Figure 2A,C), indicating that the compound induced cell apoptosis in a cell type-dependent manner. These results showed that one of the mechanisms by which crocin inhibits SPC-A1 lung cancer cell proliferation is via the induction of cell apoptosis.
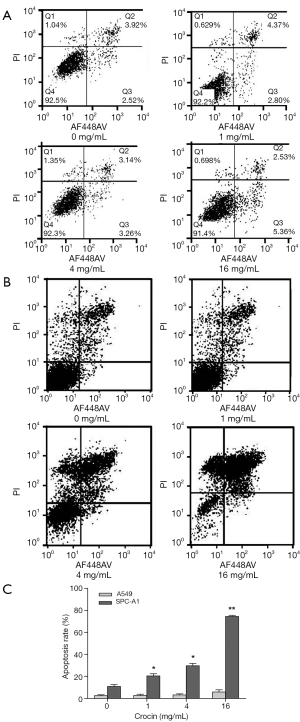
Crocin induces cell cycle arrest at G0/G1 phase
To further study the mechanism by which crocin inhibits the proliferation of A549 and SPC-A1 cells, we determined the cell cycle distribution by flow cytometry. Treatment of A549 cells with crocin resulted in cell cycle arrest at G0/G1 phase (Figure 3A). Similar results were found in SPC-A1 cells (Figure 3B).
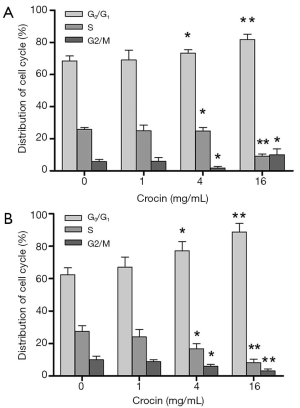
Crocin increases the mRNA expression of p53 and Bax while reduces the mRNA expression of Bcl-2 in A549 and SPC-A1 cells
Many drugs induce cell death by increasing p53 levels and the Bax/Bcl-2 ratio. We therefore tested the mRNA expression of p53, Bax, and Bcl-2 by RT-PCR. In A549 cells, crocin did not alter Bax mRNA levels, but significantly increased p53 mRNA levels, while decreasing Bcl-2 mRNA levels at a higher concentration (Figure 4A,B). In SPC-A1 cells, crocin significantly and gradually increased the mRNA levels of both p53 and Bax, while decreasing Bcl-2 (Figure 4C,D). In addition, the Bax/Bcl-2 ratios were 1.74, 1.81, 2.10, and 2.73 in A549 cells, and 0.62, 0.88, 2.33 and 3.60 in SPC-A1 treated with 0, 1, 4, and 16 mg/mL crocin, respectively. The Bax/Bcl-2 ratio showed a significant dose-dependent increase in both A549 and SPC-A1 cells (P<0.05).
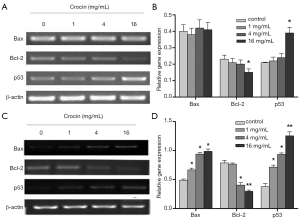
Crocin sensitizes A549 cells to cisplatin and PMX
MTT analysis showed that the IC50 values of crocin, cisplatin, and PMX were 4.12 mg/mL, 2.14 µg/mL, and 2.63 µg/mL in A549 cells, respectively. In SPC-A1 cells, IC50 values of crocin, cisplatin, and PMX were 5.28 mg/mL, 3.37 µg/mL, and 4.71 µg/mL, respectively. Using the concentrations of these IC50s, we treated A549 and SPC-A1 cells with these three drugs alone and in combination for 48 h and then detected cell proliferation by MTT assay. Crocin combined with cisplatin had a stronger inhibitory effect on A549 cells than the single-agent cisplatin (P<0.05, Figure 5). In accordance with the effective formula, the q-value of the two-drug combination was 1.20, indicating that the two drugs had a synergistic effect. Moreover, flow cytometry analysis showed that crocin + cisplatin increased the proportion of cells in G0/G1 phase as compared to cisplatin alone (P<0.05, data not shown). Similarly, crocin in combination with PMX demonstrated an additive inhibitory effect than that with PMX alone (P<0.05, Figure 5), which the q-value of the two-drug combination was 1.14. In addition, crocin + cisplatin or PMX additively inhibited the proliferation of SPC-A1 cells with q-values of 0.97 and 0.90, respectively (Figure 5).
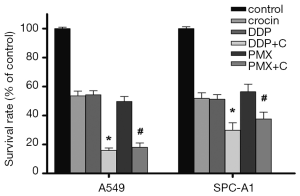
Discussion
In the present study, we found that crocin inhibited cell proliferation and induced cell cycle arrest in G0/G1 phase in both A549 and SPC-A1 cells in a dose-dependent manner. In addition, crocin treatment induced remarkable apoptosis in SPC-A1 cells. Moreover, our study showed that crocin significantly increased p53 mRNA levels and decreased Bcl-2 mRNA levels, while it did not affect the expression of Bax in A549 cells. However, in SPC-A1 cells, levels of p53 and Bax mRNA were gradually increased, while the expression of Bcl-2 mRNA was gradually decreased with the increase of crocin concentration. These findings suggest that crocin could act as a potential anti-cancer agent for lung cancer treatment. More importantly, we found that crocin combined with either cisplatin or PMX showed synergistic or additive effects on A549 or SPC-A1 cells, indicating that crocin sensitizes cancer cells to cisplatin and PMX, both of which are at the forefront of drugs in lung cancer clinical therapy.
Saffron is a natural herb that is commonly used in clinics to promote blood circulation and remove blood stasis, and recent studies have shown that this compound has anti-cancer activity (26). Crocin, the extract of saffron, can inhibit effectively the growth of cancer cells, and prolonged treatment can kill cancer cells (26). García-Olmo et al. found that crocin could prolong the survival of female mice suffering from colon adenocarcinoma and inhibit tumor growth without side effects (27). Escribano et al. found that crocin-treated HeLa cells displayed swelling, plasma membrane rupture, nuclear condensation, and acceleration of cell apoptosis (28). Crocin also demonstrated greater inhibitory effect on cancer cell growth than the crude extract of saffron, indicating that crocin is a promising anti-cancer pharmacological compound of the various components of saffron (28). All together, these findings show that crocin is a potential chemotherapeutic agent (29,30).
The underlying anticancer mechanisms of crocin remain to be determined. The chemotherapeutic effects of crocin may be related to its strong cytotoxicity in tumor cells (12). Our results in the present study further showed that crocin can although inhibit the growth of both A549 and SPC-A1 cells, A549 seems to be more sensitive to crocin than SPC-A1. Our data suggest that necrosis instead of apoptosis might involve in this process due to the fact that the rate of apoptosis in A549 cells after crocin treatment was remarkably less than that in SPC-A1 cells. In addition, the apoptotic genes including p53, Bax, and Bcl-2, especially the Bax/Bcl-2 ratio, distinctly changed in SPC-A1 cells after crocin treatment, but not in A549 cells, indicated that crocin induced significant apoptosis in SPC-A1 cells. A previous study has also shown the consistent findings, demonstrating that crocin triggers the apoptosis through increasing the Bax/Bcl-2 ratio and caspase activation in human gastric adenocarcinoma cells (31). Nevertheless, the exact molecular mechanisms for crocin-induced cell cytotoxicity in lung cancer are not clear and warrant further investigations.
Chemotherapy and molecular target therapy are now the main therapeutic regimen for the treatment of lung cancer patients but currently facing a bottleneck. The combination therapy using existing drugs is difficult due to the challenge of multi-drug toxicity. However, incorporating of anticancer therapy using traditional medicine has provided great value in increasing not only the efficacy, but reducing the adverse effects (32). Our study has first evaluated whether synergistic or additive antitumor effects on lung cancer cells could be achieved when crocin is combined with chemotherapy (cisplatin) or molecularly targeted drug (PMX). We found that crocin and cisplatin had a synergistic effect while crocin and PMX had an additive effect on A549 cells. Moreover, crocin showed additive effects with cisplatin or PMX in SPC-A1 cells. These data suggest that crocin could enhance the efficacy of lung cancer treatment. These evident effects could be related to signaling pathways in cell survival, apoptosis, or necrosis, and etc, and the potential molecular mechanisms underlining these phenomena require further study.
Conclusions
In summary, we discovered that crocin significantly suppressed the proliferation of human lung adenocarcinoma cells and enhanced the chemosensitivity of these cells to cisplatin or PMX. The molecular mechanism may be related to the induction of cell cycle arrest and apoptosis through the regulation of p53 and Bax/Bcl-2. The future studies are needed to evaluate the molecular mechanisms of the anti- lung cancer effects of crocin alone or in combination with chemotherapy or molecularly targeted drugs in both in vitro but mouse lung tumor model(s).
Acknowledgements
None.
Footnote
Conflicts of Interest: The authors have no conflicts of interest to declare.
References
- Abdullaev FI, Espinosa-Aguirre JJ. Biomedical properties of saffron and its potential use in cancer therapy and chemoprevention trials. Cancer Detect Prev 2004;28:426-32. [PubMed]
- Kang C, Lee H, Jung ES, et al. Saffron (Crocus sativus L.) increases glucose uptake and insulin sensitivity in muscle cells via multipathway mechanisms. Food Chem 2012;135:2350-8. [PubMed]
- Abdullaev FI. Cancer chemopreventive and tumoricidal properties of saffron (Crocus sativus L.). Exp Biol Med (Maywood) 2002;227:20-5. [PubMed]
- Das I, Das S, Saha T. Saffron suppresses oxidative stress in DMBA-induced skin carcinoma: A histopathological study. Acta Histochem 2010;112:317-27. [PubMed]
- Kanakis CD, Tarantilis PA, Pappas C, et al. An overview of structural features of DNA and RNA complexes with saffron compounds: Models and antioxidant activity. J Photochem Photobiol B 2009;95:204-12. [PubMed]
- Ordoudi SA, Befani CD, Nenadis N, et al. Further examination of antiradical properties of Crocus sativus stigmas extract rich in crocins. J Agric Food Chem 2009;57:3080-6. [PubMed]
- Lee IA, Lee JH, Baek NI, et al. Antihyperlipidemic effect of crocin isolated from the fructus of Gardenia jasminoides and its metabolite Crocetin. Biol Pharm Bull 2005;28:2106-10. [PubMed]
- Zheng S, Qian Z, Tang F, et al. Suppression of vascular cell adhesion molecule-1 expression by crocetin contributes to attenuation of atherosclerosis in hypercholesterolemic rabbits. Biochem Pharmacol 2005;70:1192-9. [PubMed]
- Goyal SN, Arora S, Sharma AK, et al. Preventive effect of crocin of Crocus sativus on hemodynamic, biochemical, histopathological and ultrastuctural alterations in isoproterenol-induced cardiotoxicity in rats. Phytomedicine 2010;17:227-32. [PubMed]
- Lari P, Abnous K, Imenshahidi M, et al. Evaluation of diazinon-induced hepatotoxicity and protective effects of crocin. Toxicol Ind Health 2015;31:367-76. [PubMed]
- Mehri S, Abnous K, Mousavi SH, et al. Neuroprotective effect of crocin on acrylamide-induced cytotoxicity in PC12 cells. Cell Mol Neurobiol 2012;32:227-35. [PubMed]
- Abdullaev Jafarova F, Caballero-Ortega H, Riverón-Negrete L, et al. In vitro evaluation of the chemopreventive potential of saffron. Rev Invest Clin 2002;54:430-6. [PubMed]
- Jagadeeswaran R, Thirunavukkarasu C, Gunasekaran P, et al. In vitro studies on the selective cytotoxic effect of crocetin and quercetin. Fitoterapia 2000;71:395-9. [PubMed]
- Dhar A, Mehta S, Dhar G, et al. Crocetin inhibits pancreatic cancer cell proliferation and tumor progression in a xenograft mouse model. Mol Cancer Ther 2009;8:315-23. [PubMed]
- Chryssanthi DG, Dedes PG, Karamanos NK, et al. Crocetin inhibits invasiveness of MDA-MB-231 breast cancer cells via downregulation of matrix metalloproteinases. Planta Med 2011;77:146-51. [PubMed]
- Deng Y, Guo ZG, Zeng ZL, et al. Studies on the pharmacological effects of saffron(Crocus sativus L.)--a review. Zhongguo Zhong Yao Za Zhi 2002;27:565-8. [PubMed]
- Harrington M. Saffron offers protection from liver cancer. Lab Anim (NY) 2011;40:289. [PubMed]
- Kianbakht S, Ghazavi A. Immunomodulatory effects of saffron: a randomized double-blind placebo-controlled clinical trial. Phytother Res 2011;25:1801-5. [PubMed]
- Tokgun O, Akca H, Mammadov R, et al. Convolvulus galaticus, Crocus antalyensis, and Lilium candidum extracts show their antitumor activity through induction of p53-mediated apoptosis on human breast cancer cell line MCF-7 cells. J Med Food 2012;15:1000-5. [PubMed]
- Yamauchi M, Tsuruma K, Imai S, et al. Crocetin prevents retinal degeneration induced by oxidative and endoplasmic reticulum stresses via inhibition of caspase activity. Eur J Pharmacol 2011;650:110-9. [PubMed]
- Mohajeri SA, Hosseinzadeh H, Keyhanfar F, et al. Extraction of crocin from saffron (Crocus sativus) using molecularly imprinted polymer solid-phase extraction. J Sep Sci 2010;33:2302-9. [PubMed]
- Umigai N, Tanaka J, Tsuruma K, et al. Crocetin, a carotenoid derivative, inhibits VEGF-induced angiogenesis via suppression of p38 phosphorylation. Curr Neurovasc Res 2012;9:102-9. [PubMed]
- Jnaneshwari S, Hemshekhar M, Santhosh MS, et al. Crocin, a dietary colorant, mitigates cyclophosphamide-induced organ toxicity by modulating antioxidant status and inflammatory cytokines. J Pharm Pharmacol 2013;65:604-14. [PubMed]
- Li CY, Huang WF, Wang QL, et al. Crocetin induces cytotoxicity in colon cancer cells via p53-independent mechanisms. Asian Pac J Cancer Prev 2012;13:3757-61. [PubMed]
- Noureini SK, Wink M. Antiproliferative effects of crocin in HepG2 cells by telomerase inhibition and hTERT down-regulation. Asian Pac J Cancer Prev 2012;13:2305-9. [PubMed]
- Bolhassani A, Khavari A, Bathaie SZ. Saffron and natural carotenoids: Biochemical activities and anti-tumor effects. Biochim Biophys Acta 2014;1845:20-30.
- García-Olmo DC, Riese HH, Escribano J, et al. Effects of long-term treatment of colon adenocarcinoma with crocin, a carotenoid from saffron (Crocus sativus L.): an experimental study in the rat. Nutr Cancer 1999;35:120-6. [PubMed]
- Escribano J, Alonso GL, Coca-Prados M, et al. Crocin, safranal and picrocrocin from saffron (Crocus sativus L.) inhibit the growth of human cancer cells in vitro. Cancer Lett 1996;100:23-30. [PubMed]
- Magesh V, Singh JP, Selvendiran K, et al. Antitumour activity of crocetin in accordance to tumor incidence, antioxidant status, drug metabolizing enzymes and histopathological studies. Mol Cell Biochem 2006;287:127-35. [PubMed]
- Mousavi SH, Moallem SA, Mehri S, et al. Improvement of cytotoxic and apoptogenic properties of crocin in cancer cell lines by its nanoliposomal form. Pharm Biol 2011;49:1039-45. [PubMed]
- Hoshyar R, Bathaie SZ, Sadeghizadeh M. Crocin triggers the apoptosis through increasing the Bax/Bcl-2 ratio and caspase activation in human gastric adenocarcinoma, AGS, cells. DNA Cell Biol 2013;32:50-7. [PubMed]
- Li SG, Chen HY, Ou-Yang CS, et al. The efficacy of Chinese herbal medicine as an adjunctive therapy for advanced non-small cell lung cancer: a systematic review and meta-analysis. PLoS One 2013;8:e57604. [PubMed]