Correlation of tumor microenvironment-related markers with clinical outcomes in patients with squamous cell carcinoma of the lung
Introduction
Lung cancer (LC) is a leading cause of cancer-related death worldwide (1-3). LC is histologically classified into two types: non-small cell lung cancer (NSCLC) and small cell LC (1). NSCLC is the most common histological type (1). Among LCs, small cell carcinoma is associated with poor prognosis; however, outcomes in patients with NSCLC are also poor, with a 10-year survival rate of only 5% (4,5). Moreover, NSCLC can be subdivided into squamous cell carcinoma (SCC) and adenocarcinoma (1). Lung SCC may be different from adenocarcinoma of the lung in terms of carcinogenesis (tobacco-related versus not tobacco-related) and patient survival (6-8).
The tumor microenvironment (TME) plays crucial roles in neoplastic progression and cancer metastasis (9-11). The TME is a complex region comprising cancer cells, extracellular matrix (ECM), and various types of stromal cells, including macrophages, inflammatory cells, cancer-associated fibroblasts (CAFs), and mesenchymal stem cells (6). Together, these TME-related components, particularly CAFs, contribute to tumor growth and metastasis via secretion of growth factors and cytokines (5,9). Although the contributions of CAFs to LC progression have been studied (5,9), the molecular basis of SCC (e.g., CAF-related proteins that promote tumor progression and metastasis) has not been fully elucidated.
Characterization of CAF phenotypes, including TME-related factors and their impact on outcomes, has attracted much interest (5,9,10). Many reports have also described the prognostic effects of single CAF-related markers in NSCLC (6), and various activating proteins secreted from CAFs have been shown to promote cancer invasion and metastasis (5,9). The impact of CAFs varies according to the specific marker, with contradictory results reported (5,9). This may be explained by the heterogeneity of CAFs within the same tumor, where different markers can be expressed by functionally distinct CAFs (5).
In this study, we evaluated whether the CAF phenotype could be determined based on analysis of various CAF- and epithelial-mesenchymal transition (EMT)-related markers expressed in CAFs, including α-smooth muscle actin (α-SMA), CD10, podoplanin, fibroblast-specific protein 1 (FSP1), platelet-derived growth factor receptor (PDGFR) α, PDGFRβ, adipocyte enhancer-binding protein 1 (AEBP1), fibroblast activation protein (FAP), tenascin-C, Zinc finger E-box binding homeobox 1 (ZEB1), and twist homolog 1 gene (TWIST1). We also assessed whether CAF-related protein expression contributed to clinical outcomes in patients with lung SCC. We aimed to identify heterogeneous expression of CAF-related proteins in lung SCC and evaluate whether such expression patterns affected prognosis in patients with lung SCC. These findings may contribute to evaluation of the molecular mechanisms of resistance and the associations of CAF-related marker expression patterns with prognosis. We present the following article in accordance with the REMARK reporting checklist (available at https://tlcr.amegroups.com/article/view/10.21037/tlcr-22-10/rc).
Methods
Patients
Among patients who underwent curative surgery for LC at Iwate Medical University Hospital from January 2010 to December 2016, we enrolled a consecutive series of 108 patients with lung SCC for whom paraffin-embedded tissues were relatively well preserved, medical records were complete, and patient survival had been followed-up. Tumor markers were examined. The end of the follow-up period was May 2021 (median follow-up period: 1,547 days; maximum: 4,060 days; minimum: 40 days). Histological diagnosis of SCC was performed according to the 2021 version of the World Health Organization histological classification (8,9). Patient survival was confirmed through the National Cancer Registry and by telephone interviews. The clinicopathological findings of patients with LC were further confirmed by reviewing patient medical records and pathology files. The associations of clinicopathological parameters and immunohistochemical findings with survival were investigated for all 108 patients. Clinicopathological findings of LC are summarized in Table 1. Only patients with pathological stages (p-stage) I–III disease were included. Patients who underwent pre-operative chemotherapy and patients with asynchronous or simultaneous LC were excluded. These findings were obtained from the medical records of patients admitted at Iwate Medical University. The study was conducted in accordance with the Declaration of Helsinki (as revised in 2013). This study was approved by the local ethics committee of Iwate Medical University (approval No. MH2021-047), and all patients provided informed consent.
Table 1
Characteristics | Values |
---|---|
Age median, years [range] | 72 [51–87] |
Sex | |
Man | 100 (92.6%) |
Woman | 8 (7.4%) |
Smoking history | |
Yes | 105 (97.2%) |
No | 3 (2.8%) |
Co-morbidities | |
COPD | |
Yes | 43 (39.8%) |
No | 65 (60.2%) |
ILD | |
Yes | 24 (22.2%) |
No | 84 (77.8%) |
Tumor marker | |
CEA | |
Positive | 67 (62.0%) |
Negative | 41 (38.0%) |
CYFRA | |
Positive | 71 (65.7%) |
Negative | 37 (34.3%) |
p-stage | |
I | 55 (50.9%) |
II and III | 53 (49.1%) |
Lymph node metastasis | |
Positive | 31 (28.7%) |
Negative | 77 (71.3%) |
Vascular invasion | |
Positive | 32 (29.6%) |
Negative | 76 (70.4%) |
Pleural invasion | |
Positive | 27 (25.0%) |
Negative | 81 (75.0%) |
Lymphatic invasion | |
Positive | 14 (13.0%) |
Negative | 94 (87.0%) |
Postoperative adjuvant therapy | |
Yes | 61 (56.5%) |
Chemotherapy | 57 (52.8%) |
Chemoradiotherapy | 3 (2.8%) |
Radiation therapy | 1 (0.9%) |
No | 47 (43.5%) |
CD3/CD8 ratio | |
High | 57 (52.8%) |
Low | 51 (47.2%) |
Disease-free survival, days, median [range] | 1,281 [40–4,060] |
Overall survival, days, median [range] | 1,547 [40–4,060] |
Data are presented as number (percentage). COPD, chronic obstructive pulmonary disease; ILD, interstitial lung disease; CEA, carcinoembryonic antigen; CYFRA, cytokeratin fragment; p-stage, pathological stage.
Among pulmonary comorbidities, chronic obstructive pulmonary disease (COPD) was found in 43 of 108 (39.8%) cases of lung SCC, whereas interstitial lung disease (ILD), including interstitial pneumonia, was observed in 24 of 108 (22.2%) cases of lung SCC.
Determination of overall survival and disease-free survival
LC-specific survival, defined as cause of death from LC, was used as overall survival. In addition, recurrence-free survival, which excluded secondary cancers, was adopted to measure disease-free survival. The duration of disease-free survival was determined based on the presence/absence of metastasis, determined during the follow-up period (3–4 times/year) using computed tomography.
Sample size determination
The sample size required to identify patient outcomes was determined to be at least 100 cases using JMP Pro 16.1 software (SAS, Tokyo, Japan). The statistical power (detection power) was set to 0.8, which is commonly used in medical studies.
Chemotherapeutic treatment after surgery for lung SCC
Following surgery, tegafur uracil was administered to patients with lung SCC (29/108 cases), whereas platinum-doublet chemotherapy, including carboplatin plus gemcitabine, tegafur/gimeracil/oteracil, or paclitaxel, was administered to patients with lung SCC (28/108 cases). Chemoradiotherapy was performed in three patients with lung SCC. Only one patient received radiation therapy after operation. The other 47 patients did not receive additional chemotherapy following surgery.
Construction of tissue microarrays (TMAs)
Paraffin-embedded tissues used for construction of TMAs were stored at room temperature. These materials showed sufficient quality for biological assessments, including high DNA and RNA quality. TMAs were created using a manual tissue array (Azumaya Co., Tokyo, Japan). We selected invasive areas with strong desmoplastic fibrosis for analysis by immunohistochemistry. Representative histological features of lung SCC with strong desmoplasia are shown in Figure 1. Five-millimeter-thick tissue cores were collected from target lesions and placed into recipient blocks containing 12 cores, including 10 cancer tissues and 2 control tissues. After construction, 3-µm-thick sections were prepared and stained with hematoxylin and eosin using the initial slides to verify the histological diagnosis. Serial sections were cut from the TMA blocks for immunohistochemical staining.
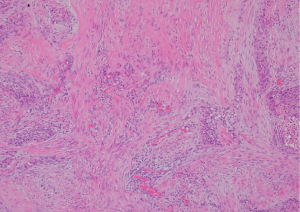
Immunohistochemistry
Three-micron-thick tissue sections were cut from paraffin-embedded blocks and placed on charged slides, which were dried and melted in an oven at 62 ℃ for 20 min. After deparaffinization and rehydration, the sections were heated in Envision FLEX target retrieval solution (pH 6.0 or 9.0; Dako, Glostrup, Denmark) for 20 min and washed twice for 5 min in phosphate-buffered saline (PBS). Hydrogen peroxide (3%) was used to block endogenous peroxidase activity for 5 min. Nonspecific binding was blocked with 1.5% normal serum in PBS for 35 min at room temperature. The antibodies used in this study are listed in Table S1. Immunohistochemistry was carried out using the DAKO Envision+ system (12). The specimens were heated in citrate buffer (pH 6.0) 3 times for 5 min each using a microwave (H2500; Microwave Processor; Azumaya) at 750 W before incubation with antibodies. The antigen-antibody reaction was visualized using an enhanced polymer-based detection system. Hematoxylin was used as the counterstain. Desmoplastic tissues surrounding invasive colorectal cancer (CRC) and ILD were used as a positive control. The detailed methods were described previously (12).
Assessment of immunohistochemical results
The immunopositivity of the stromal fibroblastic compartment of each tumor was examined for α-SMA, CD10, podoplanin, FSP1, PDGFRα, PDGFRβ, AEBP1, FAP, tenascin-C, ZEB1, and TWIST1. Inflammatory cells were carefully excluded from the analysis. Only nuclear positivity for ZEB1 and TWIST1 was considered significant, whereas only cytoplasmic expression of α-SMA, CD10, podoplanin, FSP1, PDGFRα, PDGFRβ, AEBP1, FAP and tenascin-C was regarded as positive. The immunostaining intensity and area were evaluated separately. The immunostaining intensity for fusiform stromal cells was classified into four categories according to staining intensity as follows: negative, weak, moderate, and strong. The immunostaining area for fusiform stromal cells was semiquantified as follows: 0%, 1–25%, 26–50%, and 51–100%. The combination of intensity and area was scored (Table S2). A score of more than 4 was considered a positive score. Determination of the score was performed by expert diagnostic pathologists (NY, MO, TS) who were blinded to the study endpoint. If results among the pathologists were discordant, a discussion meeting was held, and a consensus was reached. Finally, all markers used in the current study had been identified as CAF- and EMT-related markers in a previous study (12).
Hierarchical cluster analysis of the expression of CAF- and EMT-related markers
Hierarchical cluster analysis was performed to group the samples according to immunohistochemical expression levels, thereby achieving maximal homogeneity for each group and the greatest difference between groups using open-access clustering software (Cluster 3.0 software; bonsai.hgc.jp/~mdehoon/software/cluster/software.htm). The clustering algorithm was set to centroid linkage clustering.
Statistical analysis
Data were analyzed using JMP Pro 16.1 software (SAS). Fisher’s exact test was used to compare the immunohistochemical positivity of each marker and clinicopathological findings with each subgroup. Age distributions among subgroups were examined using Mann-Whitney U tests. Kaplan-Meier analyses were performed using log-rank tests for survival analyses. The level of significance was set at P<0.05.
Univariate and multivariate analyses were conducted with Cox proportional hazards models to identify significant differences for prediction of overall and disease-free survival. The level of significance was set at P<0.05, and the confidence interval (CI) was determined at the 95% level.
Study design
The current study was a retrospective study (Figure S1). Among the lung SCCs examined, 142 lung SCC samples were initially evaluated; 108 lung SCC samples were ultimately included after exclusion of 34 cases based on exclusion criteria (asynchronous or simultaneous cancer, 8 cases; pre-operative chemotherapy, 2 cases; prognosis unknown, 13 cases; death within 30 days, 2 cases; loss of histological specimens, 9 cases). First, 108 patients with lung SCC were used to identify the associations of 11 CAF- and EMT-related markers with patient survival using immunohistochemistry. Second, cluster analysis was performed to stratify the lung SCCs based on expression patterns to exclude arbitrary results. As a result, some subgroups were expected to be segregated. Third, we attempted to identify the examined markers to characterize the subgroups. Finally, we examined the associations of CAF- and EMT-related markers with patient outcomes, including disease-free and overall survival, using univariate and multivariate analyses. The CD3/CD8 ratio was also examined to evaluate the associations of EMT-related marker expression with immune status in the TME.
List of all candidate variables initially examined
For prediction of patient outcomes, pulmonary complications, tumor marker, p-stage, and immunohistochemical markers were examined, and subgroups were stratified using immunohistochemical expression patterns.
Assessment of the CD3/CD8 ratio is described in the Appendix 1.
Results
Basic demographic characteristics, standard prognostic variables, and tumor markers
There were no significant differences in age distribution compared with that in previous studies. The lung SCC samples examined in this study were from older patients owing to advanced smoking history in Japanese man. We compared CAF- and EMT-related markers with p-stage of LC as a standard prognostic factor. Representative immunohistochemical features are shown in Figure 2.
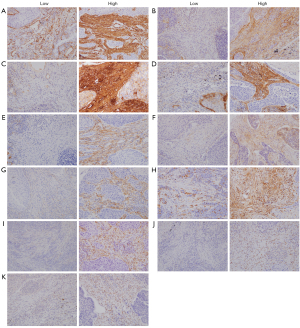
Kaplan-Meier analyses of clinicopathological findings based on disease-free and overall survival
Although the presence/absence of COPD was not correlated with disease-free and overall survival, that of ILD was closely associated with disease-free and overall survival (Figure S2A,S2B). Additionally, p-stage was also correlated with disease-free and overall survival (Figure S2C,S2D). Tumor markers, including carcinoembryonic antigen (CEA) and cytokeratin fragment (CYFRA), showed no significant association.
Hierarchical clustering based on marker scores
We performed hierarchical clustering based on marker scores to evaluate differences in the expression patterns of CAF- and EMT-related markers in patients with lung SCC. Two distinct subgroups were stratified (Figure 3; subgroups 1 and 2). There were significant differences in the frequencies of disease-free survival and overall survival between subgroups 1 and 2 (Table 2). However, no significant differences were found for other clinicopathological factors.
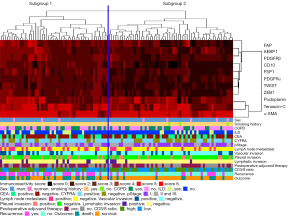
Table 2
Variable | Subgroup 1 | Subgroup 2 | P value |
---|---|---|---|
Total | 50 | 58 | |
Age median, years [range] | 72 [51–84] | 72 [54–87] | 0.5958 |
Sex | 0.7224 | ||
Man | 47 (94%) | 53 (91.4%) | |
Woman | 3 (6%) | 5 (8.6%) | |
Smoking history | 0.2471 | ||
Yes | 50 (100%) | 55 (94.8%) | |
No | 0 | 3 (5.2%) | |
COPD | 0.4363 | ||
Yes | 22 (44%) | 21 (36.2%) | |
No | 28 (56%) | 37 (63.8%) | |
ILD | 0.4872 | ||
Yes | 13 (26%) | 11 (19%) | |
No | 37 (74%) | 47 (81%) | |
CEA | 0.5512 | ||
Positive | 33 (66%) | 34 (58.6%) | |
Negative | 17 (34%) | 24 (41.4%) | |
CYFRA | 0.1072 | ||
Positive | 37 (74%) | 34 (58.6%) | |
Negative | 13 (26%) | 24 (41.4%) | |
p-stage | 0.0531 | ||
I | 20 (40%) | 35 (60.3%) | |
II and III | 30 (60%) | 23 (39.7%) | |
Lymph node metastasis | 0.139 | ||
Positive | 18 (36%) | 13 (22.4%) | |
Negative | 32 (64%) | 45 (77.6%) | |
Vascular invasion | 0.402 | ||
Positive | 17 (34%) | 15 (25.9%) | |
Negative | 33 (66%) | 43 (74.1%) | |
Pleural invasion | 0.2763 | ||
Positive | 15 (30%) | 12 (20.7%) | |
Negative | 35 (70%) | 46 (79.3%) | |
Lymphatic invasion | 0.1641 | ||
Positive | 9 (18%) | 5 (8.6%) | |
Negative | 41 (82%) | 53 (91.4%) | |
Postoperative adjuvant therapy | 0.5613 | ||
Yes | 30 (60%) | 31 (53.5%) | |
No | 20 (40%) | 27 (46.6%) | |
CD3/CD8 ratio | 1 | ||
High | 26 (52%) | 31 (53.4%) | |
Low | 24 (48%) | 27 (46.6%) | |
Recurrence | <0.0001 | ||
Yes | 31 (62%) | 13 (22.4%) | |
No | 19 (38%) | 45 (77.6%) | |
Outcome | 0.0010 | ||
Death | 25 (50%) | 11 (19%) | |
Survival | 25 (50%) | 47 (81%) |
Data are presented as number (percentage). COPD, chronic obstructive pulmonary disease; ILD, interstitial lung disease; CEA, carcinoembryonic antigen; CYFRA, cytokeratin fragment; p-stage, pathological stage.
Kaplan-Meier analyses were performed to determine the associations of disease-free and overall survival with subgroups. Patients in subgroup 1 had poorer disease-free survival than patients in subgroup 2 (P<0.0001; Figure S2E). Additionally, overall survival was correlated with subgroup 1 (P=0.0033; Figure S2F).
In our comparison of disease-free survival with clinicopathological findings, including subgroup analyses, 3 clinicopathological findings, i.e., presence of ILD, p-stage (II and III > I), and subgroup (subgroup 1 > subgroup 2), were identified in univariate analysis and retained in multivariate analysis (Table 3—left-sided column and second column). For overall survival, the same 3 clinicopathological findings were identified in univariate and multivariate analyses (Table 3—third column and right-sided column). Finally, positive values of CEA and CYFRA were not retained in univariate analysis of disease-free and overall survival.
Table 3
Variable | Univariate analysis | Multivariate analysis | Univariate analysis | Multivariate analysis | |||||||||||
---|---|---|---|---|---|---|---|---|---|---|---|---|---|---|---|
HR | 95% CI | P value | HR | 95% CI | P value | HR | 95% CI | P value | HR | 95% CI | P value | ||||
Sex | |||||||||||||||
Man vs. woman | 2.081 | 0.612–13.130 | 0.2745 | – | – | – | 3.268 | 0.661–59.691 | 0.1738 | – | – | – | |||
p-stage | |||||||||||||||
II and III vs. I | 2.315 | 1.261–4.399 | 0.0066 | 2.243 | 1.199–4.197 | 0.0115 | 2.797 | 1.405–5.939 | 0.0031 | 2.555 | 1.246–5.239 | 0.0105 | |||
COPD | |||||||||||||||
Yes vs. no | 0.741 | 0.396–1.384 | 0.3467 | – | – | – | 0.581 | 0.280–1.207 | 0.1457 | – | – | – | |||
ILD | |||||||||||||||
Yes vs. no | 2.500 | 1.322–4.725 | 0.0048 | 2.539 | 1.338–4.818 | 0.0044 | 2.188 | 1.082–4.423 | 0.0293 | 2.073 | 1.021–4.207 | 0.0437 | |||
CEA | |||||||||||||||
Positive vs. negative | 1.546 | 0.823–2.904 | 0.1754 | – | – | – | 1.739 | 0.851–3.553 | 0.1294 | – | – | – | |||
CYFRA | |||||||||||||||
Positive vs. negative | 0.933 | 0.504–1.728 | 0.8262 | – | – | – | 1.021 | 0.510–2.045 | 0.954 | – | – | – | |||
Vascular invasion | |||||||||||||||
Positive vs. negative | 1.798 | 0.935–3.361 | 0.0769 | – | – | – | 1.508 | 0.720–3.009 | 0.2661 | – | – | – | |||
Pleural invasion | |||||||||||||||
Positive vs. negative | 1.274 | 0.640–2.396 | 0.4556 | – | – | – | 1.064 | 0.485–2.158 | 0.8699 | – | – | – | |||
Lymphatic invasion | |||||||||||||||
Positive vs. negative | 1.833 | 0.814–3.707 | 0.1345 | – | – | – | 1.986 | 0.831–4.220 | 0.1161 | – | – | – | |||
Postoperative adjuvant therapy | |||||||||||||||
Yes vs. no | 0.865 | 0.477–1.568 | 0.6331 | – | – | – | 0.893 | 0.462–1.727 | 0.7371 | – | – | – | |||
CD3/CD8 ratio | |||||||||||||||
High vs. low | 1.186 | 0.650–2.164 | 0.5791 | – | – | – | 1.654 | 0.839–3.261 | 0.1464 | – | – | – | |||
Subgroup | |||||||||||||||
Subgroup 1 vs. 2 | 3.341 | 1.786–6.620 | 0.0001 | 3.075 | 1.595–5.927 | 0.0008 | 2.775 | 1.397–5.884 | 0.0032 | 2.523 | 1.264–5.371 | 0.0082 |
HR, hazard ratio; CI, confidence interval; p-stage, pathological stage; COPD, chronic obstructive pulmonary disease; ILD, interstitial lung disease; CEA, carcinoembryonic antigen; CYFRA, cytokeratin fragment.
Comparison of individual markers with each subgroup
The positive ratios of FAP (P=0.0109), CD10 (P<0,0028), PDGFRβ (P=0.0001), FSP1 (P=0.0005), AEBP1 (P=0.0005), ZEB1 (P=0.0078), and TWIST1 (P<0.0001) expression in CAFs were significantly higher in subgroup 1 than in subgroup 2 (Figure 4).
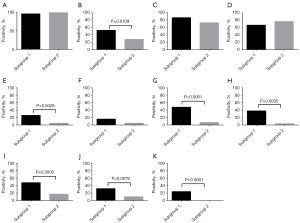
Association of various markers with patient survival
To determine whether the clinicopathological variables and expression patterns of the examined markers were independent predictors of clinical outcomes in patients with lung SCC, we used univariate analysis for preliminary screening of the variables, followed by a stepwise logistic regression of the risk of mortality with the significant univariate correlators (predictors). Univariate analysis (Table 4—left-sided column) identified 7 factors, including presence of ILD, p-stage, AEBP1, FAP, PDGFRβ, tenascin-C, and TWIST1, as being associated with disease-free survival in patients with lung SCC. Finally, we found that presence of ILD, p-stage, and AEBP1 remained significantly correlated with disease-free survival in multivariate analysis, even after controlling for the other variables (Table 4—second column). Next, we examined the associations of these factors with overall survival. Although presence of ILD, p-stage, FAP, tenascin-C, and TWIST1 were identified as significant factors in univariate analysis of lung SCC, only presence of ILD and p-stage were retained in multivariate analysis (Table 4—third column and right-sided column).
Table 4
Variable | Univariate analysis | Multivariate analysis | Univariate analysis | Multivariate analysis | |||||||||||
---|---|---|---|---|---|---|---|---|---|---|---|---|---|---|---|
HR | 95% CI | P value | HR | 95% CI | P value | HR | 95% CI | P value | HR | 95% CI | P value | ||||
p-stage | |||||||||||||||
II and III vs. I | 2.315 | 1.261–4.399 | 0.0066 | 2.232 | 1.163–4.283 | 0.0158 | 2.797 | 1.405–5.939 | 0.0031 | 2.479 | 1.168–5.262 | 0.0181 | |||
ILD | |||||||||||||||
Positive vs. negative | 2.500 | 1.322–4.725 | 0.0048 | 2.279 | 1.158–4.488 | 0.0171 | 2.188 | 1.082–4.423 | 0.0293 | 2.078 | 1.020–4.234 | 0.0441 | |||
AEBP1 | |||||||||||||||
Positive vs. negative | 2.971 | 1.617–5.525 | 0.0005 | 2.252 | 1.128–4.494 | 0.0214 | 1.933 | 0.980–3.763 | 0.0569 | – | – | – | |||
CD10 | |||||||||||||||
Positive vs. negative | 1.242 | 0.532–2.563 | 0.5915 | – | – | – | 1.074 | 0.402–2.415 | 0.8754 | – | – | – | |||
FAP | |||||||||||||||
Positive vs. negative | 2.137 | 1.165–3.967 | 0.0144 | 1.258 | 0.631–2.510 | 0.5142 | 2.278 | 1.170–4.534 | 0.0156 | 1.567 | 0.772–3.182 | 0.2137 | |||
PDGFR-α | |||||||||||||||
Positive vs. negative | 1.844 | 0.750–3.909 | 0.1684 | – | – | – | 1.551 | 0.527–3.678 | 0.3899 | – | – | – | |||
PDGFR-β | |||||||||||||||
Positive vs. negative | 2.184 | 1.143–4.050 | 0.0190 | 1.015 | 0.428–2.406 | 0.9723 | 1.477 | 0.690–2.976 | 0.3024 | – | – | – | |||
Podoplanin | |||||||||||||||
Positive vs. negative | 0.828 | 0.441–1.646 | 0.5756 | – | – | – | 0.937 | 0.464–2.047 | 0.8618 | – | – | – | |||
FSP1 | |||||||||||||||
Positive vs. negative | 1.118 | 0.502–2.244 | 0.7699 | – | – | – | 1.183 | 0.500–2.504 | 0.6822 | – | – | – | |||
α-SMA* | |||||||||||||||
Positive vs. negative | – | – | – | – | – | – | – | – | – | – | – | – | |||
Tenascin-C | |||||||||||||||
Positive vs. negative | 2.538 | 1.094–7.389 | 0.0284 | 1.847 | 0.681–5.009 | 0.228 | 3.187 | 1.137–13.303 | 0.0250 | 2.452 | 0.690–8.710 | 0.1655 | |||
TWIST1 | |||||||||||||||
Positive vs. negative | 2.551 | 1.095–5.253 | 0.0316 | 1.536 | 0.536–4.403 | 0.4245 | 2.649 | 1.059–5.784 | 0.0385 | 1.641 | 0.681–3.956 | 0.2699 | |||
ZEB1 | |||||||||||||||
Positive vs. negative | 1.151 | 0.535–2.261 | 0.7029 | – | – | – | 1.227 | 0.541–2.528 | 0.6050 | – | – | – |
*, could not analyze, why almost cases were positive expression of the marker. HR, hazard ratio; CI, confidence interval; p-stage, pathological stage; ILD, Interstitial lung disease; AEBP1, adipocyte enhancer-binding protein 1; FAP, fibroblast activation protein; PDGFR, platelet-derived growth factor receptor; FSP1, fibroblast-specific protein 1; α-SMA, α-smooth muscle actin; TWIST1, twist homolog 1 gene; ZEB1, Zinc finger E-box binding homeobox 1.
Association of subgroups with the CD3/CD8 ratio in lung SCC
The CD3/CD8 ratio was not correlated with subgroups classified based on prognosis (worse prognosis: subgroup 1 > subgroup 2; Table 2). Moreover, the CD3/CD8 ratio was not correlated with prognosis (not retained in univariate analysis; Table 3).
Discussion
The CAF phenotype, which is determined by the expression patterns of CAF- and EMT-related markers, may vary based on the type of markers selected in each specific study (5,13,14). Therefore, the CAF phenotype may depend on the type of immunohistochemical scoring system used (12). In a previous study, the dominant staining intensity was calculated in positive cells, whereas another study used an index combining both staining intensity and extent (15,16). Such differences in the scoring system may in part explain the differences observed between these two studies (15,16). Indeed, the use of different scoring criteria in biomarker research and subsequent difficulties in comparing studies are problems preventing the validation of biomarkers (5). However, in this study, the phenotype we identified could exclude the arbitrariness of classification and consequently became an objective classification of the CAF phenotype. Additionally, the current phenotype, stratified based on multiple CAF-related markers, identified the relationship between heterogeneous expression of CAF-related markers, which are affected by chemotherapy response, and patient prognosis.
The CAF phenotype is closely associated with the expression patterns of the immunohistochemical markers examined in this study (12). In this study, the prognosis in patients with LC was closely associated with the immunohistochemical expression patterns of CAF- and EMT-related markers. This finding suggested that the heterogeneous expression of environmental markers may affect prediction of prognosis in patients with lung SCC. Cancer is a dynamic disease in terms of biological characteristics (17). During disease progression, cancers generally become more heterogeneous (5). As a result, the bulk tumor may contain a diverse collection of cells with distinct molecular signatures and differential levels of sensitivity to treatment, and varying patient outcomes may be observed (5). Thus, our findings suggested that clinical assessment of tumor heterogeneity may facilitate the development of more effective personalized therapies.
Histological type is correlated with prognosis in patients with lung adenocarcinoma (4,8). For example, patient outcomes were improved in papillary/acinar carcinoma with lepidic changes compared with those in solid tumors without lepidic changes (8). However, such histological markers have not yet been used in pathological diagnosis of lung SCC. In the current study, we attempted to establish histological markers predicting clinical outcomes in patients with lung SCC. Analysis of immunohistochemical expression may be a simple approach for examining biological markers using paraffin-embedded tissues, which are widely used in routine pathology diagnosis. We believe that lung SCC may be a suitable model for identifying the expression patterns of TME-related markers.
AEBP1 is a ubiquitously expressed, multifunctional protein that is expressed at particularly high levels in pre-adipocytes and macrophages (18). AEBP1 has been implicated in various human malignancies, including glioblastoma, melanoma, gastric cancer, and CRC (18). Recently, AEBP1 was found to be overexpressed in stromal cells of CRC, where it promoted proliferation, migration, invasion, and metastasis by activating nuclear factor-κB signaling (18). Furthermore, Yorozu et al. (18) showed that endothelial cells present in the cancer stroma are closely associated with tumor angiogenesis in CRC. In the current study, however, AEBP1 overexpression was shown to be upregulated in CAFs of patients with lung SCC. These results suggested that AEBP1 may have oncogenic effects in not only epithelial tumor cells but also stromal cells. In addition, our current findings implied that AEBP1 may be a therapeutic target in lung SCC, which was further supported by the observation that AEBP1 upregulation conferred acquired resistance to BRAF (V600E) inhibition in melanoma (19).
Few other studies have evaluated the regrading of expression patterns of CAF-related markers in CAFs. In one recent study, the expression of the extensively characterized CAF marker podoplanin in CAFs was shown to be closely associated with the immune microenvironment in early lung SCC (20). Although podoplanin is widely used as a lymphatic epithelial marker (21), podoplanin-positive fibroblasts in cancer have been shown to enhance the invasive properties of carcinoma cells and to play important roles in the remodeling of the ECM (22). In addition, podoplanin expression is significantly associated with survival, suggesting potential differences in prognostic relevance based on NSCLC histological subtype (5). Furthermore, Chen et al. (23) revealed that FAP-α expression is involved in microvessel and lymphatic vessel density in lung SCC. FAP is a type II integral membrane serine protease involved in ECM remodeling and tumor cell migration and has been used as a marker of activated fibroblasts in a number of studies (5,24). By contrast, one univariate study demonstrated that increased FAP expression in CAFs was associated with improved survival in NSCLC (25). However, this is the first study to identify the association of expression patterns for an extensive panel of CAF-related markers with prognosis in patients with lung SCC.
In the current study, ILD was closely associated with patient survival, including disease-free and overall survival (26). Our findings were consistent with the shared common risk factors between LC and ILD, including smoking and chemical exposure (26). According to a previous study, ILD, which is detected during surgery, is an important risk factor for lung SCC. In addition, a previous study showed that the histological distribution of ILD in LC is lower than that in adenocarcinoma, but higher than that in SCC (26). We suggest that the close association of ILD plus lung SCC with patient survival may depend on the extent of smoking. In addition, advanced fibrosis occurring in ILD may enhance the invasive ability of CAFs, as is observed in LC.
CAF-induced resistance to chemotherapy and radiotherapy in LC is closely associated with several factors, including cytokines, chemokines, growth factors, and exosomes (27,28). The molecular mechanisms mediating resistance to chemotherapy and radiotherapy have been evaluated (27-30). Cytokines and chemokines are inflammatory mediators secreted by cancer cells or CAFs in the TME and can stimulate tumor-promoting processes, including proliferation, metastasis, and progression, in an autocrine or paracrine manner (27-30). In addition, the cytokines and chemokines in the TME are strongly related to chemoresistance and poor prognosis in patients with cancer (27,28). In lung adenocarcinoma cells in vivo and in vitro, IL-11 was found to be able to protect cancer cells from cisplatin-induced apoptosis and thus promote their chemoresistance (27-29). As a result, CAFs treated with cisplatin confer chemoresistance to LC cells (27-29). Furthermore, cancer-secreted TGF-β can enhance the transition of resident fibroblasts into CAFs, and CAF-secreted TGF-β is involved in cancer therapy resistance in cancer cells (27-29). Finally, various studies have examined the roles of exosomes in cancer progression (27,30). The function of CAF-derived exosomes in cancer therapy resistance was initially investigated in CRC (27,30). Hu et al. (30) reported that CAF-derived exosomes promote drug resistance by mediating the activation of the Wnt signaling pathway in CSCs in CRC. Understanding the molecular mechanisms mediating chemoresistance by CAFs will become even more important in this field.
In the current study, we examined the associations of the CD3/CD8 ratio with subgroups stratified according to CAF-related proteins and with prognosis in patients with lung SCC. However, the CD3/CD8 ratio, which is closely associated with prognosis in patients with LC (31-33), was not correlated with subgroup in patients with lung SCC. This finding suggested that the expression pattern of CAF-related proteins secreted from CAFs is independent of the CD3/CD8 ratio. Furthermore, the current results implied that the CD3/CD8 ratio may not be associated with poor patient outcomes.
There were some limitations to this study. First, heterogeneous expression of CAF- and EMT-related markers is an important issue for assessment of immunohistochemical results (34). In the current study, we evaluated immunohistochemical expression in invasive areas of tumor samples. Such invasive areas are thought to be suitable for obtaining reproducible results at the invasive area. Second, we selected markers to evaluate in the current study. Thus, subjective results may be expected. In addition, TGF-β, which plays key roles in the EMT, was not examined. However, we selected reliable and reproducible markers to identify the biological characteristics of CAFs (12). For discovery of new CAF-related markers, genome-wide analysis may contribute to comprehensive evaluation of mRNA expression occurring in the cancer stromal tissue. In our experience, isolation of the surrounding stromal cells containing CAFs may enable identification of appropriate mRNA expression that is closely associated with prognosis in patients with lung SCC. However, despite great efforts, the discovery of new CAF-related markers is actually very difficult, even when using genome-wide analysis. Further studies are needed in the near future. Third, a second cohort should be used for validation analyses, and the population size evaluated in this study was small. However, decreases in cigarette smoking in developed countries may result in fewer cases of lung SCC (8). Larger cohorts should be evaluated in the near future. Finally, in the current study, we did not perform analysis of TME-related markers of lung adenocarcinoma. In our future studies, we will plan to examine the association of immunohistochemical expression patterns with prognosis in patients with lung adenocarcinoma.
In conclusion, in this study, we examined the association of immunohistochemical expression patterns of CAF- and EMT-related markers with prognosis in patients with lung SCC. Specific subgroups stratified based on the expression patterns of the examined CAF-related markers may have applications in prediction of prognosis in patients with lung SCC following resection. More importantly, overexpression of AEBP1 was found to be an independent prognostic factor in patients with lung SCC. Overall, our findings suggested that the expression of CAF- and EMT-related markers in CAFs may be helpful for predicting patient survival in lung SCC. In addition, our findings suggested that AEBP1 signaling, particularly in the context of AEBP1 upregulation, could be a useful therapeutic target in lung SCC. Taken together, this study provides insights into the actual roles of CAF-related proteins in determination of outcomes in patients with lung SCC because of our focus on the functional aspects of CAFs present at the invasive area. Additionally, our study takes into account that the expression of specific CAF-related proteins may play important roles in cancer progression via TME formation.
Acknowledgments
We gratefully acknowledge the technical assistance and support of members of the Department of Molecular Diagnostic Pathology, Iwate Medical University.
Funding: None.
Footnote
Reporting Checklist: The authors have completed the REMARK reporting checklist. Available at https://tlcr.amegroups.com/article/view/10.21037/tlcr-22-10/rc
Data Sharing Statement: Available at https://tlcr.amegroups.com/article/view/10.21037/tlcr-22-10/dss
Peer Review File: Available at https://tlcr.amegroups.com/article/view/10.21037/tlcr-22-10/prf
Conflicts of Interest: All authors have completed the ICMJE uniform disclosure form (available at https://tlcr.amegroups.com/article/view/10.21037/tlcr-22-10/coif). The authors have no conflicts of interest to declare.
Ethical Statement: The authors are accountable for all aspects of the work in ensuring that questions related to the accuracy or integrity of any part of the work are appropriately investigated and resolved. The study was conducted in accordance with the Declaration of Helsinki (as revised in 2013). This study was approved by the local ethics committee of Iwate Medical University (approval No. MH2021-047), and all patients provided informed consent.
Open Access Statement: This is an Open Access article distributed in accordance with the Creative Commons Attribution-NonCommercial-NoDerivs 4.0 International License (CC BY-NC-ND 4.0), which permits the non-commercial replication and distribution of the article with the strict proviso that no changes or edits are made and the original work is properly cited (including links to both the formal publication through the relevant DOI and the license). See: https://creativecommons.org/licenses/by-nc-nd/4.0/.
References
- Inamura K. Lung Cancer: Understanding Its Molecular Pathology and the 2015 WHO Classification. Front Oncol 2017;7:193. [Crossref] [PubMed]
- Cancer Research UK. 2019. Available online: https://www.cancerresearchuk.org/health-professional/cancer-statistics/statistics-by-cancer-type/lung- cancer
- Sung H, Ferlay J, Siegel RL, et al. Global Cancer Statistics 2020: GLOBOCAN Estimates of Incidence and Mortality Worldwide for 36 Cancers in 185 Countries. CA Cancer J Clin 2021;71:209-49. [Crossref] [PubMed]
- Molina JR, Yang P, Cassivi SD, et al. Non-small cell lung cancer: epidemiology, risk factors, treatment, and survivorship. Mayo Clin Proc 2008;83:584-94. [Crossref] [PubMed]
- Irvine AF, Waise S, Green EW, et al. Characterising cancer-associated fibroblast heterogeneity in non-small cell lung cancer: a systematic review and meta-analysis. Sci Rep 2021;11:3727. [Crossref] [PubMed]
- Hecht SS. Tobacco smoke carcinogens and lung cancer. J Natl Cancer Inst 1999;91:1194-210. [Crossref] [PubMed]
- Warth A, Botling, J, Chung JH. et al. Squamous cell carcinoma. In: WHO Classification of The Thoracic Tumours. 5th edition. Lyon: International Agency for Research on Cancer, 2021:89-96.
- Matsubara D, Kadota, H. MacMahon H, et al. Adenocarcinomas. In: WHO Classification of The Thoracic Tumours. 5th edition. Lyon: International Agency for Research on Cancer, 2021:60-3.
- Altorki NK, Markowitz GJ, Gao D, et al. The lung microenvironment: an important regulator of tumour growth and metastasis. Nat Rev Cancer 2019;19:9-31. [Crossref] [PubMed]
- Mittal V, El Rayes T, Narula N, et al. The Microenvironment of Lung Cancer and Therapeutic Implications. Adv Exp Med Biol 2016;890:75-110. [Crossref] [PubMed]
- Tan Z, Xue H, Sun Y, et al. The Role of Tumor Inflammatory Microenvironment in Lung Cancer. Front Pharmacol 2021;12:688625. [Crossref] [PubMed]
- Hashimoto M, Uesugi N, Osakabe M, et al. Expression Patterns of Microenvironmental Factors and Tenascin-C at the Invasive Front of Stage II and III Colorectal Cancer: Novel Tumor Prognostic Markers. Front Oncol 2021;11:690816. [Crossref] [PubMed]
- Wang L, Cao L, Wang H, et al. Cancer-associated fibroblasts enhance metastatic potential of lung cancer cells through IL-6/STAT3 signaling pathway. Oncotarget 2017;8:76116-28. [Crossref] [PubMed]
- Ishii G, Ochiai A, Neri S. Phenotypic and functional heterogeneity of cancer-associated fibroblast within the tumor microenvironment. Adv Drug Deliv Rev 2016;99:186-96. [Crossref] [PubMed]
- Kilvaer TK, Khanehkenari MR, Hellevik T, et al. Cancer Associated Fibroblasts in Stage I-IIIA NSCLC: Prognostic Impact and Their Correlations with Tumor Molecular Markers. PLoS One 2015;10:e0134965. [Crossref] [PubMed]
- Qiu X, Chen D, Liu Y, et al. Relationship between stromal cells and tumor spread through air spaces in lung adenocarcinoma. Thorac Cancer 2019;10:256-67. [Crossref] [PubMed]
- Fortunato A, Boddy A, Mallo D, et al. Natural Selection in Cancer Biology: From Molecular Snowflakes to Trait Hallmarks. Cold Spring Harb Perspect Med 2017;7:a029652. [Crossref] [PubMed]
- Yorozu A, Yamamoto E, Niinuma T, et al. Upregulation of adipocyte enhancer-binding protein 1 in endothelial cells promotes tumor angiogenesis in colorectal cancer. Cancer Sci 2020;111:1631-44. [Crossref] [PubMed]
- Hu W, Jin L, Jiang CC, et al. AEBP1 upregulation confers acquired resistance to BRAF (V600E) inhibition in melanoma. Cell Death Dis 2013;4:e914. [Crossref] [PubMed]
- Suzuki J, Aokage K, Neri S, et al. Relationship between podoplanin-expressing cancer-associated fibroblasts and the immune microenvironment of early lung squamous cell carcinoma. Lung Cancer 2021;153:1-10. [Crossref] [PubMed]
- Breiteneder-Geleff S, Soleiman A, Kowalski H, et al. Angiosarcomas express mixed endothelial phenotypes of blood and lymphatic capillaries: podoplanin as a specific marker for lymphatic endothelium. Am J Pathol 1999;154:385-94. [Crossref] [PubMed]
- Hu G, Wang S, Xu F, et al. Tumor-infiltrating podoplanin+ fibroblasts predict worse outcome in solid tumors. Cell Physiol Biochem 2018;51:1041-50. [Crossref] [PubMed]
- Chen L, Chen M, Han Z, et al. Clinical significance of FAP-α on microvessel and lymphatic vessel density in lung squamous cell carcinoma. J Clin Pathol 2018;71:721-8. [Crossref] [PubMed]
- Lee HO, Mullins SR, Franco-Barraza J, et al. FAP-overexpressing fibroblasts produce an extracellular matrix that enhances invasive velocity and directionality of pancreatic cancer cells. BMC Cancer 2011;11:245. [Crossref] [PubMed]
- Kilvaer TK, Rakaee M, Hellevik T, et al. Tissue analyses reveal a potential immune-adjuvant function of FAP-1 positive fibroblasts in non-small cell lung cancer. PloS One 2018;13:e0192157. [Crossref] [PubMed]
- Naccache JM, Gibiot Q, Monnet I, et al. Lung cancer and interstitial lung disease: a literature review. J Thorac Dis 2018;10:3829-44. [Crossref] [PubMed]
- Ham IH, Lee D, Hur H. Cancer-Associated Fibroblast-Induced Resistance to Chemotherapy and Radiotherapy in Gastrointestinal Cancers. Cancers (Basel) 2021;13:1172. [Crossref] [PubMed]
- Tao L, Huang G, Wang R, et al. Cancer-associated fibroblasts treated with cisplatin facilitates chemoresistance of lung adenocarcinoma through IL-11/IL-11R/STAT3 signaling pathway. Sci Rep 2016;6:38408. [Crossref] [PubMed]
- Zhang H, Xie C, Yue J, et al. Cancer-associated fibroblasts mediated chemoresistance by a FOXO1/TGFβ1 signaling loop in esophageal squamous cell carcinoma. Mol Carcinog 2017;56:1150-63. [Crossref] [PubMed]
- Hu Y, Yan C, Mu L, et al. Fibroblast-Derived Exosomes Contribute to Chemoresistance through Priming Cancer Stem Cells in Colorectal Cancer. PloS One 2015;10:e0125625. [Crossref] [PubMed]
- Mei Z, Liu Y, Liu C, et al. Tumour-infiltrating inflammation and prognosis in colorectal cancer: systematic review and meta-analysis. Br J Cancer 2014;110:1595-605. [Crossref] [PubMed]
- Mazzaschi G, Madeddu D, Falco A, et al. Low PD-1 Expression in Cytotoxic CD8+ Tumor-Infiltrating Lymphocytes Confers an Immune-Privileged Tissue Microenvironment in NSCLC with a Prognostic and Predictive Value. Clin Cancer Res 2018;24:407-19. [Crossref] [PubMed]
- Kim H, Kwon HJ, Han YB, et al. Increased CD3+ T cells with a low FOXP3+/CD8+ T cell ratio can predict anti-PD-1 therapeutic response in non-small cell lung cancer patients. Mod Pathol 2019;32:367-75. [Crossref] [PubMed]
- Wu F, Fan J, He Y, et al. Single-cell profiling of tumor heterogeneity and the microenvironment in advanced non-small cell lung cancer. Nat Commun 2021;12:2540. [Crossref] [PubMed]