Changes in serum transforming growth factor-beta concentration as a predictive factor for radiation-induced lung injury onset in radiotherapy-treated patients with locally advanced lung cancer
Introduction
Radiation-induced lung injury (RILI) is a non-infectious lung injury that usually occurs within the field of radiation after chest radiation therapy, which may subsequently heal or be prolonged. In the initial phase, interstitial shadows, such as ground-glass attenuation, and reticular shadows appear in the lungs within the irradiation field. In this period, the symptoms are often still asymptomatic to mild. During the next organizing phase, patchy consolidation occurs in the same area as the irradiated area, regardless of the anatomical boundaries of the lung areas and lobes. When the degree of patchy consolidation is severe, various respiratory symptoms appear. After this phase, the fibrotic phase occurs, and linear scarring, increased density consolidation, and decreased lung volume are observed in line with the irradiated area. During this phase, the symptoms of the acute phase disappear and stabilize; however, respiratory function may be impaired if the volume loss is marked. The clinical course of the disease is usually stable during follow-up, but in cases of severe symptoms, treatment with steroids is required, and the disease may spread to the irradiated area, becoming severe and rarely fatal (1).
Although we have experienced various RILI patterns at our hospital, it remains difficult to predict the RILI onset. Moreover, it is necessary to elucidate the pathophysiology of RILI. The pathology of RILI involves free radicals and DNA damage, which causes oxidative stress, angiopathy, and inflammation. Persistent inflammation causes various pathological changes, which may be influenced by various cytokines, including transforming growth factor-beta (TGF-β) (1-3). We aimed to investigate whether serum levels of TGF-β, Krebs von Denlungen-6 (KL-6), and granulocyte colony-stimulating factor (G-CSF) could predict RILI onset in patients who had received radiation therapy for locally advanced lung cancer. We present the following article in accordance with the STROBE reporting checklist (available at https://tlcr.amegroups.com/article/view/10.21037/tlcr-22-229/rc).
Methods
Study design and patients
This single-center prospective observational study was conducted from November 2016 to August 2019 at the Kyoto Prefectural University Hospital on patients with locally advanced lung cancer who underwent thoracic radiation therapy. The study was conducted in accordance with the Declaration of Helsinki (revised in 2013). The study protocol was approved by the Ethics Committee of Kyoto Prefectural University of Medicine (No. ERB-C-679). All the patients provided informed consent.
We included patients who did or did not receive chemotherapy. There were 21 patients who had received chest radiation therapy (RT) for locally advanced lung cancer, including Stages I to III (Union for International Cancer Control; Tumor-Node-Metastasis (TNM) classification and staging of lung cancer, 8th edition). Of the 21 patients, four received stereotactic radiation therapy (SRT) and 17 received intensity-modulated radiation therapy (IMRT). Of the four patients who received stereotactic irradiation, one received it in combination with chemotherapy and three received radiation therapy alone; all of which with a total dose, number of fractions, and duration of treatment of 48 Gy, 4 fr, and one week, respectively. Of the 17 patients who received IMRT, five received hyper-fractionated intensity modulated radiation therapy (HF-IMRT) and 12 received standard fractionated intensity-modulated radiation therapy (SF-IMRT). One patient in the SF-IMRT group had to be stopped immediately after irradiation due to an adverse event. Excluding this patient, the mean total dose, number of fractions, and duration of treatment were 55.76 Gy, 35.6 fr, and 3.6 weeks, respectively, (45–64 Gy, 30–40 fr, 3–4 weeks) for the HF-IMRT group and 57.1 Gy, 28.1 fr, and 5.8 weeks for the SF-IMRT group, respectively (40–64 Gy, 19–40 fr, 4–8 weeks). We measured serum cytokine levels (TGF-β, KL-6, and G-CSF) in each patient before therapy, as well as 2 weeks, 4 weeks, 8 weeks, 12 weeks, 16 weeks, 6 months, and 1 year after irradiation. TGF-β levels were analyzed using the Quantikine® ELISA kit Human TGF-β1 Immunoassay. G-CSF levels were analyzed using the Quantikine® ELISA kit Human G-CSF Immunoassay. KL-6 measurement was outsourced to SRL Inc (Shinjuku Mitsui Building, 2-1-1 Nishishinjuku, Shinjuku-ku, Tokyo 163-0408. http://www.srl-group.co.jp/english/). The patients were monitored up until one year after irradiation. RILI grading was based on the term “pneumonitis” in National Cancer Institute Common Terminology Criteria for Adverse Events (NCI-CTCAE) version 5.0. Patients diagnosed with RILI Grade 2 or higher were started on prednisolone at 0.5–0.8 mg/kg/day, titrated down by 5 mg/day over 1–2 weeks according to symptoms. Before the 2, 4, and 6 weeks after initiation of steroid administration, the patients underwent outpatient examinations, blood tests, and treatment monitoring by diagnostic imaging. We analyzed the following variables: age, sex, body mass index (BMI), Eastern Cooperative Oncology Group performance status (ECOG PS), pathology, smoking, Brinkman index, % vital capacity (%VC), %forced expiratory volume in one (%FEV1), tumor size (Response Evaluation Criteria in Solid Tumors v1.1), albumin, TNM stages, dose-volume histogram (DVH) parameters, chemotherapy administration, total radiation dose, and RILI grade. TNM staging was performed using the TNM stage classification system version 8.
Pulmonologists performed diagnosis, treatment selection, and subsequent follow-ups, while radiologists planned the method and range of radiation therapy. All follow-up computed tomography (CT) scans were evaluated by radiologists and pulmonologists. Patients with new infiltration or cord-like shadows on CT and “Grade 2: Symptomatic and requiring therapeutic intervention” or higher in CTCAE v5.0 “Pneumonia” were classified as RP+, and those with “Grade 1: no therapeutic intervention required” or lower were classified as RP−. Patients who could not complete the irradiation due to some adverse events were excluded from the analysis.
Statistical analysis
Statistical analyses were performed using IBM SPSS Advanced Statistics software (version 25; SPSS Inc., Chicago, IL, USA). Patient characteristics at the participation time were presented as standard descriptive statistics. Mann-Whitney U test was performed to identify the significant clinical factors related to the Grade 2 and 3 RILI and to compare the cytokine levels at each time point between the Grade 0 and 1 RILI group (RP− group) and Grade 2 and 3 RILI group (RP+ group). Relative ratios at each time point were defined as follows: for 2 weeks after RT finish and cytokine levels at 2 weeks after RT finish/cytokine levels at pre-RT. Statistical significance was set at P>0.05.
Results
Baseline characteristics of the patients
Among the 21 patients, one was excluded since treatment was interrupted due to Grade 4 neutropenia and septic shock resulting from pyelonephritis on the third day of irradiation (during 12 Gy irradiation) (Figure 1). Of the 20 patients, 10 were treated with RT alone (six with SRT, three with SF-IMRT, and one with HF-IMRT) and 14 were treated with chemotherapy (one with SBRT, nine with SF-IMRT, and four with HF-IMRT). Of the patients in the combined chemotherapy group, two were treated with cisplatin (CDDP) combined and 12 with carboplatin (CBDCA) combined. One patient received one course of platinum combination therapy, one received two courses, one received three courses, and 12 received four courses. Seven, four, eight, and one patient were in Grade 0 (non-symptomatic), 1, 2, and 3, respectively (Table 1). Grade 2 and 3 RILI groups (RP+ group) required steroid therapy. Treatment with prednisolone was initiated at 0.5–0.8 mg/kg/day depending on symptoms, with a mean duration of treatment of 19.1 weeks (4–31 weeks); three patients continued maintenance therapy at 5–10 mg/day due to relapse of RP during steroid reduction. There were no significant between-group differences in age, sex, BMI, ECOG-PS, smoking index, %VC, and treatment with chemotherapy. There were no significant between-group differences in the total radiation dose and irradiation period, with the median irradiation doses in the RP+ and RP− groups being 57 and 60 Gy, respectively. Additionally, both groups include those who received radiotherapy only. Based on the histological classification, adenocarcinoma, squamous cell carcinoma, small cell carcinoma, and non-small cell lung cancer (NSCLC) were observed in four (36.4%), two (18.2%), three (27.3%), and two (18.2%) patients, respectively, in the RP− group. In the RP+ group, adenocarcinoma, squamous cell carcinoma, small cell carcinoma, and NSCLC were observed in two (22.2%), four (44.4%), one (11.1%), and three (33.3%) patients, respectively. Lymph node metastasis was described based on the eighth edition of the TNM classification. Compared with the RP− group, the RP+ group showed significantly higher values of the DVH parameters V20 and V5 (P=0.03, P=0.02).
Table 1
Variables | All (n=20), n (%) | RP− (n=11), n (%) | RP+ (n=9), n (%) | P value |
---|---|---|---|---|
Age, years | ||||
Median [range] | 71 [68–86] | 76 [54–85] | 0.57 | |
≤70 | 8 (40.0) | 4 (20.0) | 4 (20.0) | |
>70 | 12 (60.0) | 7 (35.0) | 5 (25.0) | |
Sex | ||||
Male | 15 (75.0) | 9 (45.0) | 6 (30.0) | 0.46 |
Female | 5 (25.0) | 2 (10.0) | 3 (15.0) | |
BMI, kg/m2, mean ±SD | 23.0±1.1 | 22.4±2.4 | 0.65 | |
ECOG PS | ||||
0 | 11 (55.0) | 6 (30.0) | 5 (25.0) | |
1 | 9 (45.0) | 5 (25.0) | 4 (20.0) | |
Pathology | ||||
Adenocarcinoma | 6 (30.0) | 4 (20.0) | 2 (10.0) | |
Squamous cell | 6 (30.0) | 2 (10.0) | 4 (20.0) | |
Small cell | 4 (20.0) | 3 (15.0) | 1 (5.0) | |
Non-small cell | 4 (20.0) | 2 (10.0) | 2 (10.0) | |
Smoking | ||||
Yes | 17 (85.0) | 9 (45.0) | 8 (40.0) | |
No | 3 (15.0) | 2 (10.0) | 1 (5.0) | |
Brinkman index, median [range] | 850 [400–3,060] | 690 [50–1,600] | 0.16 | |
%VC | ||||
Mean ± SD | 89.9±9.1 | 92.6±13.0 | 0.66 | |
%VC <80 | 4 (20.0) | 2 (10.0) | 2 (10.0) | |
%FEV1, mean ± SD, % | 80.3±13.2 | 95.8±21.4 | 0.11 | |
FEV1% (FEV1%G <70) | 8 (40.0) | 6 (30.0) | 2 (10.0) | 0.07 |
Tumor size, mean ± SD, mm | 40±23 | 47±28 | 0.60 | |
Albumin, mean ± SD, g/dL | 3.5±0.4 | 3.6±0.4 | 0.88 | |
Lymph node status (8th, TNM) | ||||
N0 | 8 (40.0) | 5 (25.0) | 3 (15.0) | |
N1 | 2 (10.0) | 2 (10.0) | 0 (0) | |
N2 | 5 (25.0) | 3 (15.0) | 2 (10.0) | |
N3 | 5 (25.0) | 1 (5.0) | 4 (20.0) | |
Clinical Stage (8th, TNM) | ||||
I | 7 (35.0) | 5 (25.0) | 2 (10.0) | |
II | 1 (5.0) | 1 (5.0) | 0 (0) | |
III | 12 (60.0) | 5 (25.0) | 7 (35.0) | |
DVH parameters, mean ± SD, % | ||||
V20* | 14.1±5.2 | 21.2±6.5 | 0.03 | |
V5 | 27.8±4.7 | 36.1±8.2 | 0.02 | |
Treatment | ||||
Single RT | 6 (30.0) | 4 (20.0) | 2 (10.0) | |
Single SRT | 3 (15.0) | 2 (10.0) | 1 (5.0) | |
Single SF-IMRT | 2 (10.0) | 2 (10.0) | 0 (0) | |
Single HF-IMRT | 1 (5.0) | 0 (0.0) | 1 (5.0) | |
Combination chemotherapy | 14 (70.0) | 7 (35.0) | 7 (35.0) | 0.90 |
+ SBRT | 1 (5.0) | 1 (5.0) | 0 (0) | |
+ SF-IMRT | 9 (45.0) | 4 (20.0) | 5 (25.0) | |
+ HF-IMRT | 4 (20.0) | 2 (10.0) | 2 (10.0) | |
Chemotherapy regimens | ||||
CDDP combined | 2 (10.0) | 2 (10.0) | 0 (0) | |
CBDCA combined | 11 (55.0) | 4 (20.0) | 7 (35.0) | |
Total radiation dose (Gy), median [range] | 57 [40–66] | 60 [45–64] | 0.19 | |
RILI grade | ||||
0 | 7 (35.0) | – | ||
1 | 4 (20.0) | – | ||
2 | – | 8 (40.0) | ||
3 | – | 1 (5.0) |
*, Vx, ratio of lung volume irradiated above xGy to total lung volume. VC, vital capacity; FEV1, forced expiratory volume in one second, DVH, dose volume histogram; SRT, stereotactic radiation therapy; SF-IMRT, standard fractionated intensity-modulated radiation therapy; HF-IMRT, hyper-fractionated intensity-modulated radiation therapy; CDDP, cisplatin; CBDCA, carboplatin; RILI, radiation-induced lung injury.
Between-group comparison of serum TGF-β levels
The 20 participants comprised individuals who received radiation monotherapy and chemoradiation combination therapy, with the irradiation period differing across the individuals. Therefore, during irradiation, we did not compare each period after treatment initiation but rather compared each irradiation dose. Serum TGF-β levels were measured before irradiation and during 15–29 and 30–48 Gy irradiation, as well as 0–2, 3–6, 7–10, 18–25, and 44–51 weeks after irradiation (Figure 2). There was no significant between-group difference in the pre-irradiation TGF-β levels, which showed variability. There was no significant between-group difference in the post-irradiation TGF-β levels. TGF-β levels were not correlated with the smoking index, BMI, %FEV1, albumin, and tumor size. Moreover, a comparative study of lymph node metastasis with N0, 1 vs. N2, 3 revealed no significant between-group difference in TGF-β levels. There was no significant between-group difference in TGF-β levels immediately before RILI onset. TGF-β levels immediately before onset were not correlated with BMI, %FEV1, or serum albumin levels. As aforementioned, the pre-radiation TGF-β levels varied across individuals before irradiation, which impeded a comparative study for predicting the onset of RILI. Therefore, we conducted a comparative study based on the relative ratio of the pre-radiation TGF-β levels.
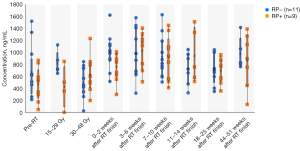
Between-group comparison of the relative to baseline of serum TGF-β levels
We performed between-group comparisons of the transition of the relative to baseline of the pre-irradiation serum TGF-β levels (Figure 3). During 30–48 Gy irradiation, the relative ratio of the pre-irradiation serum TGF-β levels was significantly higher in the RP+ group than in the RP− group (median ± SD: 1.7±1.0 vs. 0.7±0.3, P=0.01). The relative ratio of the pre-irradiation serum TGF-β levels during 30–48 Gy irradiation was not significantly correlated with the DVH parameters V5 and V20, which were also significantly different in the univariate analysis (P=0.1; P=0.1). We did not perform a logistic regression analysis given the small sample size. Additionally, the receiver operating characteristic (ROC) curve for the presence or absence of Grade 2 or higher RILI according to the TGF-β ratio during 30–48 Gy irradiation showed a moderately high AUC of 0.861 (Figure 4). The cut-off value of the Grade 2 or higher RILI group was 1.31, with a sensitivity and specificity of 75% and 100%, respectively. Moreover, the positive predictive value was 75%, with <1.31 and ≥1.31 being negative and positive, respectively. The negative predictive value was 83.3%. These findings suggested that a relative ratio of ≥1.31 at the time of 30–48 Gy irradiation could predict the onset of RILI of Grade 2 or higher. There was no significant between-group difference in the relative TGF-β ratio immediately before RILI onset; moreover, it was not associated with the smoking index, BMI, FEV1, or albumin.
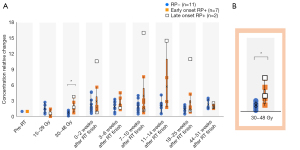
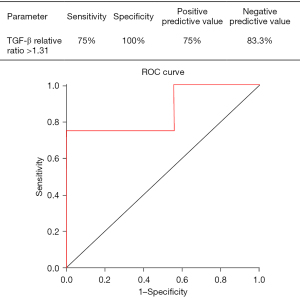
There were wide variations in the relative ratio of TGF-β levels in late-onset RILI
Nine patients with Grade 2 or higher RILI were divided according to the time of onset, followed by the comparison of clinical parameters and the TGF-β relative ratios. Specifically, seven patients developed early-onset disease by 14 weeks after irradiation, while two patients developed late-onset disease more than six months after irradiation (Figure 5). We performed between-group comparisons of changes in TGF-β cytokine levels (Figure 3). The RP− and early onset groups showed less variation throughout the year. Among the two cases in the late-onset group, one had large long-term fluctuations after the 30–48 Gy irradiation. This patient had undergone chemoradiotherapy for NSCLC; however, there was no recurrence or other adverse events for 1 year. However, this study had a small sample size, and further studies are warranted.
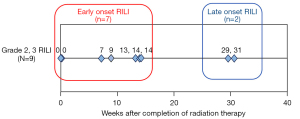
Serum KL-6 and G-CSF levels are unsuitable for predicting RILI onset
We observed no significant between-group difference in the absolute and relative ratios of serum KL-6 levels (Figure 6A,6B); moreover, there were not correlated with TGF-β levels. Similarly, there was no significant between-group difference in the absolute and relative ratios of serum G-CSF levels (Figure 6C,6D). The absolute G-CSF levels at the time of 30–48 Gy irradiation showed significant differences between with and without chemotherapy. There was no correlation of the absolute and relative ratio of G-CSF levels with other clinical parameters. Regarding the serum G-CSF levels, two cases deviated from the normal distribution and showed markedly high values. However, both cases had acute inflammation, which coincided with the increased levels. The first patient did not develop RILI and showed a marked increase in serum G-CSF levels (concentration relative change >800) at the same time as peritonitis due to small intestinal perforation and intra-abdominal abscess. Additionally, the second patient developed febrile neutropenia (FN) associated with chemotherapy, which coincided with a marked increase in serum G-CSF levels (concentration relative change 132.3). Radiotherapy was completed after the improvement of FN; moreover, the patient developed Grade 2 RP immediately after completion.
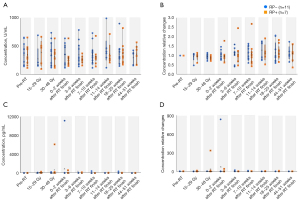
Discussion
First, compared with the RP− group, the RP+ group had a significantly higher relative ratio of TGF-β levels at the time of 30–48 Gy irradiation (P=0.01); moreover, a relative ratio of TGF-β level ≥1.31 could predict the onset of Grade 2 or higher RILI. Second, KL-6 and G-CSF could not predict RILI onset. Third, patients with late-onset RILI showed a tendency of fluctuations in the relative ratio of TGF-β levels.
A relative ratio of TGF-β of ≥1.31 can predict RILI onset
Compared with the RP− group, the RP+ group showed a significantly higher relative ratio of TGF-β levels at the time of 30–48 Gy irradiation (P=0.01). Further, a relative ratio of TGF-β levels of ≥1.31 could predict the onset of Grade 2 or higher RILI. In RILI, TGF-β contributes to oxidative stress, angiopathy, inflammation due to free radicals, and DNA damage after irradiation. Sustained TGF-β signaling activation causes activation of the SMAD signaling pathway, which stimulates fibroblast proliferation and extracellular matrix (ECM) deposition. This results in a more hypoxic environment and promotes fibrosis (3). Static fibroblasts with ECM, type I collagen, and fibronectin are activated by external stresses, including ROS, TGF-β, hypoxia, and other cytokines. Activated fibroblasts are plastic and are characterized by a secretory phenotype, which results in different cross-linking of ECMs and production of fibronectin, TGF-β, CCL-5, and IL-6. Additionally, activated fibroblasts promote cytoskeletal remodeling, which suggests that TGF-β is significantly involved in late-onset RILI. Although there have been studies on RILI-related cytokine levels, it is difficult to identify significant factors due to variations in the evaluation timings and patient characteristics (1,4). A recent study reported a significant increase in G-CSF, IL-6, and interferon-gamma levels at 3 weeks after chemoradiotherapy for NSCLC. However, there was no significant increase in the TGF-β levels and their relative ratio during irradiation (5). However, this previous study performed a 3-month follow-up after irradiation and did not include patients with late-onset RP. Contrastingly, we included two cases of late-onset RILI, 6 months after the end of irradiation. Taken together, the relative ratio of the serum TGF-β levels at 30–48 Gy irradiation to immediately before irradiation could be a useful predictor of RILI onset, including late-onset RILI.
Serum KL-6 and G-CSF levels are not predictive markers for RILI onset
Our study found no significant differences in the serum KL-6 and G-CSF levels between the RP+ and RP− groups.
KL-6 is a glycoprotein that belongs to the MUC1 mutation of ≥5,000 kDa. It is mainly produced by type II alveolar epithelial cells in the lungs; further, interstitial lung disease is characterized by increased serum KL-6 levels (6,7). Increased serum KL-6 levels reflect the damage and production of epithelial cells; additionally, it could be useful as a biomarker for diseases, such as idiopathic pulmonary fibrosis, hypersensitivity pneumonitis, sarcoidosis, and collagen disease-related interstitial pneumonitis (IP) (7-10). In addition to KL-6, SP-A, SP-D, and MCP-1 are serum markers for interstitial pneumonia; however, KL-6 is the most sensitive (94%) marker for interstitial pneumonia, with high specificity (96%) (11). In addition, KL-6 is correlated with the proportion of frosted glass shadows in the lung field area (measured in five cross-sections); further, it is more strongly correlated with fibrotic lesions (the number of areas with traction bronchiectasis) (12). Patients with IP shadows on CT as well as high serum KL-6 and SP-D levels before stereotactic body radiation therapy showed a high rate of developing severe RP (13). These previous findings suggested that KL-6 could be a predictive marker for RILI onset.
G-CSF is comprised of approximately 180 amino acid residues (14-16); moreover, its receptor is located on progenitor cells in the bone marrow. Upon receptor stimulation by G-CSF, there is cell differentiation and proliferation into mature granulocytes. Additionally, it induces survival, proliferation/differentiation, and function of neutrophil progenitor cells and mature neutrophils, which causes a marked increase in the levels of neutrophil inflammation. Regarding the association between radiotherapy and G-CSF, pulmonary toxicity development after G-CSF treatment has been reported (17). Moreover, patients with RILI show a high neutrophil-to-lymphocyte ratio. G-CSF-activated neutrophils can cause pulmonary toxicity by attacking the hilar vascular wall of the alveoli, which is associated with progression to RILI (18). Radiotherapy is usually discontinued when using G-CSF preparations. A previous study observed that G-CSF levels peaked in the lung, bronchoalveolar lavage, and serum samples obtained from RILI-sensitive mice early after radiation exposure; further, there was a correlation between tissue and serum cytokine levels. This indicated that serum G-CSF levels could be a predictive marker for radiotoxicity (19).
Nonetheless, our findings revealed no significant between-group differences in the serum KL-6 and G-CSF levels.
As for KL-6, previous studies have demonstrated a correlation between the incidence of RP and increased serum KL-6 and SP-D levels (13,20). The incidence of severe RILI has decreased from 18.8% in 2005–2006 to 3.5%, which could be attributed to prior screening methods for KL-6 screening and IP shadows (13). We did not include cases with IP shadows; furthermore, since the baseline KL-6 levels were within the normal range, patients at risk of RILI who had high KL-6 levels during the pretreatment screening were excluded. For these reasons, it is considered that there was no correlation between the incidence of RP and increased serum KL-6 level.
As for G-CSF, regarding the two patients with markedly high serum G-CSF levels, the onset time of small intestinal perforation, peritonitis, intra-abdominal abscess, and febrile neutropenia coincided with the time of markedly increased G-CSF levels. Serum G-CSF levels reflect RILI and various inflammatory changes also, including neutropenia and other chemotherapy-induced infectious diseases. As suggested by Ao et al. (19), serum G-CSF levels may predict relatively early-onset RILI; however, they cannot be used alone since they are easily affected by other factors.
TGF-β as a predictor marker for late-onset RILI
Compared with the early-onset group, the late-onset group showed greater fluctuations in the TGF-β ratio, especially at the time of 30–48 Gy irradiation, immediately after irradiation, and 2–3 months after irradiation. As aforementioned, few studies have performed long-term follow-up from the start of irradiation to one year; additionally, the fluctuation of the TGF-β ratio in late-onset RP during and after irradiation remains unclear. In our study, the two patients with late-onset RP showed a tendency of a high relative ratio of TGF-β levels at the time of 30–48 Gy irradiation. This suggests that a high relative ratio of TGF-β levels can predict RP development, especially late-onset RILI. Careful follow-up imaging examination should be performed even 6 months after the end of irradiation.
RILI in combination with radiation therapy and immune checkpoint inhibitors (ICIs)
This study included one case of ICI monotherapy as second-line treatment and two cases of combined use of CCRT and durvalumab combination therapy during the follow-up period.
While the benefits of ICIs in the treatment of lung cancer are clear, there are concerns regarding RILI in radiation and ICI combination therapy. In fact, the PACIFIC trial showed a higher incidence of RP in the durvalumab group than in the placebo group (20% vs. 15.8%) (21). Moreover, another multicenter prospective cohort study of 20 patients with Grade 1 RP before the start of durvalumab after CCRT found that three patients (15%) experienced Grade 3 or higher adverse events within 3 months of starting durvalumab. Moreover, three patients (15%) had Grade 2 or higher non-infectious RP. Although durvalumab may be a viable treatment option for this patient population, its long-term safety remains unclear (22). Nivolumab administration to patients who have undergone chest radiation therapy was found to cause the radiation pneumonia recall phenomenon, with delayed imaging findings, including RP (23,24). In this study, three patients underwent ICI therapy after radiation, and none developed RILI for one year after irradiation; moreover, the TGF-β or G-CSF levels did not significantly decrease. Our study included only a few cases and did not provide a comprehensive view of ICIs and RILI, but further studies on ICIs and RILI are needed as more cases of radiation immunotherapy are expected in the future.
This study had several limitations. First, the overall sample size was small, and there were only two cases with late-onset RILI. Therefore, future large-scale studies conducting multivariate analyses are needed. Second, in some cases, irradiation was discontinued due to other adverse events; moreover, data were missing at the time of analysis. Third, adjuvant steroid therapy might have had modification effects on some patients who received radiation monotherapy and chemoradiotherapy.
Conclusions
Our findings indicate that changes in serum TGF-β levels during 30–48 Gy irradiation can predict the onset of RILI of Grade 2 and higher even after >6 months. Thus, a long-term follow-up of these patients is necessary. Our findings could inform the prediction of late-onset RILI, which could mitigate delays in detection and intervention.
Acknowledgments
The authors sincerely appreciate all the physicians and patients who participated in this study.
Funding: None.
Footnote
Reporting Checklist: The authors have completed the STROBE reporting checklist. Available at https://tlcr.amegroups.com/article/view/10.21037/tlcr-22-229/rc
Data Sharing Statement: Available at https://tlcr.amegroups.com/article/view/10.21037/tlcr-22-229/dss
Peer Review File: Available at https://tlcr.amegroups.com/article/view/10.21037/tlcr-22-229/prf
Conflicts of Interest: All authors have completed the ICMJE uniform disclosure form (available at https://tlcr.amegroups.com/article/view/10.21037/tlcr-22-229/coif). TY reports receiving research grants from Ono Pharmaceutical Co., Ltd., Takeda Pharmaceutical Co. Ltd., Pfizer Inc., Boehringer Ingelheim Japan Inc., and Chugai Pharmaceutical Co., Ltd. KT reports receiving research grants from Chugai-Roche Co., and Ono Pharmaceutical Co.; personal fees from AstraZeneca Co., Chugai-Roche Co., MSD-Merck Co., Eli Lilly Co., Boehringer-Ingelheim Co., and Daiichi–Sankyo Co. The other authors have no conflicts of interest to declare.
Ethical Statement: The authors are accountable for all aspects of the work in ensuring that questions related to the accuracy or integrity of any part of the work are appropriately investigated and resolved. The study was conducted in accordance with the Declaration of Helsinki (revised in 2013). The study protocol was approved by the Ethics Committee of Kyoto Prefectural University of Medicine (No. ERB-C-679). All the patients provided informed consent.
Open Access Statement: This is an Open Access article distributed in accordance with the Creative Commons Attribution-NonCommercial-NoDerivs 4.0 International License (CC BY-NC-ND 4.0), which permits the non-commercial replication and distribution of the article with the strict proviso that no changes or edits are made and the original work is properly cited (including links to both the formal publication through the relevant DOI and the license). See: https://creativecommons.org/licenses/by-nc-nd/4.0/.
References
- Hanania AN, Mainwaring W, Ghebre YT, et al. Radiation-Induced Lung Injury: Assessment and Management. Chest 2019;156:150-62. [Crossref] [PubMed]
- Vallée A, Lecarpentier Y, Guillevin R, et al. Interactions between TGF-β1, canonical WNT/β-catenin pathway and PPAR γ in radiation-induced fibrosis. Oncotarget 2017;8:90579-604. [Crossref] [PubMed]
- Citrin DE, Prasanna PGS, Walker AJ, et al. Radiation-Induced Fibrosis: Mechanisms and Opportunities to Mitigate. Report of an NCI Workshop, September 19, 2016. Radiat Res 2017;188:1-20. [Crossref] [PubMed]
- Liu X, Shao C, Fu J. Promising Biomarkers of Radiation-Induced Lung Injury: A Review. Biomedicines 2021;9:1181. [Crossref] [PubMed]
- Jeong BK, Kim JH, Jung MH, et al. Cytokine Profiles of Non-Small Cell Lung Cancer Patients Treated with Concurrent Chemoradiotherapy with Regards to Radiation Pneumonitis Severity. J Clin Med 2021;10:699. [Crossref] [PubMed]
- Kohno N, Kyoizumi S, Awaya Y, et al. New serum indicator of interstitial pneumonitis activity. Sialylated carbohydrate antigen KL-6. Chest 1989;96:68-73. [Crossref] [PubMed]
- Global Burden of Disease Cancer Collaboration. Global, Regional, and National Cancer Incidence, Mortality, Years of Life Lost, Years Lived With Disability, and Disability-Adjusted Life-Years for 29 Cancer Groups, 1990 to 2016: A Systematic Analysis for the Global Burden of Disease Study. JAMA Oncol 2018;4:1553-68. [Crossref] [PubMed]
- Inoue Y, Barker E, Daniloff E, et al. Pulmonary epithelial cell injury and alveolar-capillary permeability in berylliosis. Am J Respir Crit Care Med 1997;156:109-15. [Crossref] [PubMed]
- Okada F, Ando Y, Honda K, et al. Comparison of pulmonary CT findings and serum KL-6 levels in patients with cryptogenic organizing pneumonia. Br J Radiol 2009;82:212-8. [Crossref] [PubMed]
- Ishikawa N, Hattori N, Yokoyama A, et al. Utility of KL-6/MUC1 in the clinical management of interstitial lung diseases. Respir Investig 2012;50:3-13. [Crossref] [PubMed]
- Ohnishi H, Yokoyama A, Kondo K, et al. Comparative study of KL-6, surfactant protein-A, surfactant protein-D, and monocyte chemoattractant protein-1 as serum markers for interstitial lung diseases. Am J Respir Crit Care Med 2002;165:378-81. [Crossref] [PubMed]
- Sakamoto K, Taniguchi H, Kondoh Y, et al. Serum KL-6 in fibrotic NSIP: Correlations with physiologic and radiologic parameters. Respir Med 2010;104:127-33. [Crossref] [PubMed]
- Yamashita H, Kobayashi-Shibata S, Terahara A, et al. Prescreening based on the presence of CT-scan abnormalities and biomarkers (KL-6 and SP-D) may reduce severe radiation pneumonitis after stereotactic radiotherapy. Radiat Oncol 2010;5:32. [Crossref] [PubMed]
- Burgess AW, Metcalf D. The nature and action of granulocyte-macrophage colony stimulating factors. Blood 1980;56:947-58. [Crossref] [PubMed]
- Tanaka K, Tamakoshi A, Sugawara Y, et al. Coffee, green tea and liver cancer risk: an evaluation based on a systematic review of epidemiologic evidence among the Japanese population. Jpn J Clin Oncol 2019;49:972-84. [Crossref] [PubMed]
- Jackson CJ, Tønseth KA, Utheim TP. Cultured epidermal stem cells in regenerative medicine. Stem Cell Res Ther 2017;8:155. [Crossref] [PubMed]
- Azoulay E, Attalah H, Harf A, et al. Granulocyte colony-stimulating factor or neutrophil-induced pulmonary toxicity: myth or reality? Systematic review of clinical case reports and experimental data. Chest 2001;120:1695-701. [Crossref] [PubMed]
- Lee YH, Choi HS, Jeong H, et al. Neutrophil-lymphocyte ratio and a dosimetric factor for predicting symptomatic radiation pneumonitis in non-small-cell lung cancer patients treated with concurrent chemoradiotherapy. Clin Respir J 2018;12:1264-73. [Crossref] [PubMed]
- Ao X, Zhao L, Davis MA, et al. Radiation produces differential changes in cytokine profiles in radiation lung fibrosis sensitive and resistant mice. J Hematol Oncol 2009;2:6. [Crossref] [PubMed]
- Goto K, Kodama T, Sekine I, et al. Serum levels of KL-6 are useful biomarkers for severe radiation pneumonitis. Lung Cancer 2001;34:141-8. [Crossref] [PubMed]
- Antonia SJ, Villegas A, Daniel D, et al. Overall Survival with Durvalumab after Chemoradiotherapy in Stage III NSCLC. N Engl J Med 2018;379:2342-50. [Crossref] [PubMed]
- Sugimoto T, Fujimoto D, Sato Y, et al. Durvalumab for patients with unresectable stage III non-small cell lung cancer and grade 1 radiation pneumonitis following concurrent chemoradiotherapy: a multicenter prospective cohort study. Invest New Drugs 2021;39:853-9. [Crossref] [PubMed]
- Shibaki R, Akamatsu H, Fujimoto M, et al. Nivolumab induced radiation recall pneumonitis after two years of radiotherapy. Ann Oncol 2017;28:1404-5. [Crossref] [PubMed]
- Cousin F, Desir C, Ben Mustapha S, et al. Incidence, risk factors, and CT characteristics of radiation recall pneumonitis induced by immune checkpoint inhibitor in lung cancer. Radiother Oncol 2021;157:47-55. [Crossref] [PubMed]