KRAS mutation predict response and outcome in advanced non-small cell lung carcinoma without driver alterations receiving PD-1 blockade immunotherapy combined with platinum-based chemotherapy: a retrospective cohort study from China
Introduction
Lung cancer is the most frequently occurring cancer in men and the leading cause of cancer-related mortality in men and women, with an estimated 1.8 million of deaths in 2020 (1). Approximately 80–85% of lung cancers are non-small cell lung carcinomas (NSCLCs) (2). Personalized treatment for advanced NSCLC has been evolving over the last decade, and biomarker-based evidence of molecular pathways and/or tumor oncogene addictions is mandated for the allocation of specific targeted therapies. Anti-programmed death 1 (PD-1)/programmed death-ligand 1 (PD-L1) treatment with or without platinum-based chemotherapy has become the first-line therapy for NSCLC without driver gene mutations (3). According to multiple studies (4,5), the objective response rate for PD-1/PD-L1 inhibitors is approximately 20% in unscreened NSCLC cases. Consequently, there is an urgent need to develop efficient biomarkers that can be used to select patients who will benefit from immunotherapy, thereby providing appropriate treatment, minimizing toxicity, and reducing unnecessary costs. The detection of PD-L1 protein expression in tumor cells by immunohistochemistry (IHC) offers a means of predicting responses to immune checkpoint inhibitors (ICIs) (6), with previous studies highlighting the relevance of tumor mutational burden (TMB) in response to PD-1 blockade therapy (7), DNA mismatch repair (MMR) deficiency (8) and the intensity of CD8+ T cell infiltrates (9) being proposed as distinct biomarkers of responses to anti-PD-1/PD-L1 therapies. However, ever-changing immune system poses unique challenges to biomarker development.
Kirsten rat sarcoma (KRAS) is a murine sarcoma virus oncogene. There are 3 genes related to human tumors in the ras gene family, including H-ras, K-ras, and N-ras. Among ras genes, KRAS is the most frequent mutation in malignant tumors, especially in colorectal carcinoma, NSCLC, and pancreatic ductal carcinoma (10). KRAS mutation usually indicates poorer efficacy of traditional chemotherapy when compared with KRAS wild-type tumors (11). With the discovery by Ostrem, Shokat, and colleagues of the switch II pocket on the surface of the active and inactive forms of KRAS, we now have an improved understanding of the complex interactions involved in the RAS family of signaling proteins which has led to the development of a number of promising direct KRAS G12C inhibitors, such as sotorasib and adagrasib (12). However, there are still many difficulties in the treatment of NSCLC patients with KRAS mutations, on account of the co-occurring mutations, variability in outcomes, duration of response, and mechanisms of resistance. An unplanned subgroup analysis in the CheckMate 057 study (4) found patients treated with ICIs had longer progression-free survival (PFS) than chemotherapy-treated NSCLC patients with KRAS mutations during second-line treatment. Subsequently, the OAK study data (13) and the results of Mazieres and colleagues (14) indicated that immunotherapy showed favorable clinical outcomes in KRAS-mutant NSCLC patients. However, a larger retrospective study (15) suggested that the clinical benefit of ICIs was similar in NSCLC patients with and without KRAS mutations. Thus, the efficacy of anti-PD-1/PD-L1 ICIs in patients with KRAS mutations remains a controversial topic. To help understand this issue, we conducted a retrospective study of Chinese patients with advanced NSCLC who received immunotherapy and platinum-based chemotherapy as first-line treatment and who did not carry oncogenic driver genes for targeted therapies to explore whether KRAS mutation status could predict the effects of immunotherapy. We present the following article in accordance with the STROBE reporting checklist (available at https://tlcr.amegroups.com/article/view/10.21037/tlcr-22-655/rc).
Methods
Population
In this retrospective cohort study, all clinical data were extracted from the medical records of patients with advanced NSCLC who received first-line treatment with a PD-1 blockade therapy and platinum-based chemotherapy at the First Affiliated Hospital of Guangzhou Medical University in China between January 2019 and March 2020 and for whom molecular data were available. Clinical follow-up ended on March 1, 2021. Eligible patients also met the following criteria:
- Patients had a clinical and pathological diagnosis of NSCLC (stage IV);
- Molecular tests showed the absence of genomic variants associated with oncogenic driver alterations for which there are targeted therapies, including sensitizing epidermal growth factor receptor (EGFR) mutation, anaplastic lymphoma kinase (ALK) rearrangement, c-ros oncogene-1 receptor kinase (ROS1) rearrangement, v-raf murine sarcoma viral oncogene homolog B1 (BRAF) V600E mutation, neurotrophic tyrosine kinase (NTRK) gene fusion, mesenchymal-epithelial transition (MET) exon 14 skipping mutation, and rearranged during transfection (RET) rearrangement.
All eligible patients were microsatellite stable in the study.
- Patients received 4–6 cycles of pembrolizumab (2 mg/kg), carboplatin (AUC 5 or 6), and paclitaxel (200 mg/m2) for squamous or pemetrexed (500 mg/m2) for non-squamous every 3 weeks in first-line treatment. Patients with stable disease or objective remission continued to receive pembrolizumab monotherapy until the disease progressed;
- A follow-up was conducted to record survival status and disease progression.
The study was conducted in accordance with the Declaration of Helsinki (as revised in 2013). The study was approved by ethics committees of Guangzhou Institute of Respiratory Health (2021 No. K-15), and individual consent for this retrospective analysis was waived.
Clinicopathological variables
Clinicopathologic and molecular features, including age at diagnosis, sex, Eastern Cooperative Oncology Group (ECOG) performance status (PS), smoking status, histology, oncogene-driver subtype, brain metastases, liver metastases, PD-L1 tumor proportion score (TPS), TMB, date of first diagnosis, date of starting therapy, date of disease progression, date of death (if applicable), and date of last contact, were recorded.
Mutation analyses
Next-generation sequencing (NGS) was performed on tumor tissue or cytological samples to test the presence of oncogenic drive. Patients with oncogenic driver events for which there are targeted therapies, including sensitizing EGFR mutation, ALK rearrangement, ROS1 rearrangement, BRAF V600E mutation, NTRK gene fusion, MET exon 14 skipping mutation, and RET rearrangement, were excluded from the cohort. The eligible patients were assigned into KRAS mutant and non-KRAS mutant groups based on the molecular oncogenic drive tests.
PD-L1 expression
Expression of the PD-L1 protein in tumor cells was tested using anti-human PD-L1 (Dako 22C3, Agilent Technologies, Santa Clara, CA, USA) on Dako Autostainer Link 48. PD-L1 TPS was used to evaluate PD-L1 expression, as the percentage of PD-L1-positive tumor cells that showed partial or complete membrane staining in the overall tumor sections.
TMB calculation
TMB (i.e., the total number of mutations per coding area of a tumor genome) (16), assessed using a 1,021-cancer relevant gene panel (Department of Medical Center, Geneplus-Beijing, Beijing, China), was evaluated in patients with matched normal controls. The calculated value was then expressed as mutations per megabase (muts/Mb), with low TMB <5 muts/Mb and high TMB ≥5 muts/Mb.
Statistical analyses and objectives
The data in this study are presented as the medians (ranges) of continuous variables and were compared via Mann-Whitney U test. Categorical parameters are expressed as numbers and percentages and compared using χ2 or Fisher’s exact test with the Marascuilo procedure. The primary endpoint was overall survival (OS) in the KRAS mutant and non-KRAS mutant groups, and the secondary objective was PFS. OS was calculated as the interval from the start of treatment until death due to any cause or the last follow-up. PFS was measured from the date of the start of treatment to disease progression or death due to any cause. The curves for PFS and OS were plotted using the Kaplan-Meier method and compared via a log-rank test. We used Cox proportional hazards models for univariate and multivariate analysis to estimate clinicopathological features, PD-L1 TPS, TMB and KRAS mutation types for their associations with OS and PFS. Independent variables with P values <0.05 in the univariate analysis were enrolled in multivariate analysis. Two-sided, P values <0.05 were defined statistically significant. Confidence intervals (CIs) were calculated at a 95% CI. All statistical analyses were performed using SPSS v. 25.0 (IBM Corporation, Armonk, NY, USA).
Results
Clinicopathology, mutation status, PD-L1 expression, and TMB in patients with advanced NSCLC
From January 2019 to March 2020, 80 eligible patients were enrolled in this study, including 14 with KRAS mutations, 9 with KRAS/tumor protein p53 (TP53) co-mutations, 46 with TP53 mutations, 1 with a single-copy loss of serine/threonine kinase 11 (STK11), 1 with mitogen-activated protein kinase kinase 1 (MAP2K1) deletion, 2 with copy losses of cyclin-dependent kinase inhibitor 2A/2B (CDKN2A/2B), 1 with co-occurring mutations in TP53 and phosphatase and tensin homologue (PTEN) single-copy loss, and 6 who were oncogene driver-negative (Figure 1). Based on their mutation status, the patients were classified into two groups: a KRAS mutant group (N=23, 28.8%) and a non-KRAS mutant group (N=57, 51.2%). Among the KRAS mutant group, 9 different subtypes were identified, including G12C (N=5, 21.74%), G12V (N=5, 21.74%), G12A (N=2, 8.70%), G12D (N=4, 17.39%), G12S (N=2, 8.70%), G12R (N=1, 4.35%), G13C (N=2, 8.70%), Q61R (N=1, 4.35%), and Q61H (N=1, 4.35%) (Figure 2).
In our cohort, the median age was 63 years (range, 28–85 years). There were 71 (88.8%) men and 9 (11.2%) women. Among the patients, 27 (33.8 %) were current smokers, 31 (38.8%) were former smokers, and 22 (27.5%) had never smoked. Brain metastasis was detected in 17 (21.2%) patients, while 18 (22.5%) had liver metastasis. A total of 26 (32.5%) patients had a history of thoracic radiotherapy. Within the cohort, 52.5% (42/80) of patients had adenocarcinomas, 31.3% (25/80) had squamous cell carcinomas, and 16.3% (13/80) had NSCLC that was not otherwise specified. At the start of therapy, the majority of patients had an ECOG PS of 1 (58.8%), and the remaining had a PS of 0 (41.3%).
Tumor PD-L1 protein expression was analyzed using a 1% cutoff, with 26 (32.5%) patients negative and 54 (67.5%) positive for PD-L1 expression, of which 43 (53.8%) exhibited low PD-L1 expression (TPS between 1–49%) and 11 (13.8%) exhibited high expression (TPS ≥50%). The median TMB for the entire cohort was 7.29 muts/Mb, with a range of 0.08–44.8 muts/Mb. Among the 80 patients, 26 patients (32.5%) had low TMB (<5 muts/Mb), and 54 patients (67.5%) had high TMB (≥5 muts/Mb). Overall, there were 36 (45.0%) cases that satisfied both criteria: PD-L1-positive expression of TPS ≥1% and TMB ≥5 muts/Mb.
Baseline characteristics were similar between the KRAS and the non-KRAS mutant groups, except for radiotherapy status. More patients (52.2%) in the KRAS mutant group received radiotherapy than in the non-KRAS mutant group (P=0.017). The proportion of patients who received radiotherapy was also significantly greater in the high-TMB group than in the low-TMB group (P=0.023). The PD-L1-negative group had a significantly higher rate of liver metastasis than the PD-L1-positive group (P=0.017). The recorded clinicopathologic features are summarized in Table 1.
Table 1
Characteristics | Overall (n=80) | KRAS mutant | PD-L1 | TMB (muts/Mb) | ||||||||
---|---|---|---|---|---|---|---|---|---|---|---|---|
Yes (n=23) | Non (n=57) | P value | <1% (n=26) | ≥1% (n=54) | P value | <5 (n=26) | ≥5 (n=54) | P value | ||||
Sex | 0.491 | 0.388 | 0.462 | |||||||||
Male | 71 (88.8) | 21 (91.3) | 50 (87.7) | 24 (92.3) | 47 (87.0) | 22 (84.6) | 49 (90.7) | |||||
Female | 9 (11.3) | 2 (8.7) | 7 (12.3) | 2 (7.7) | 7 (13.0) | 4 (15.4) | 5 (9.3) | |||||
ECOG PS score | 0.207 | 0.894 | 0.186 | |||||||||
0 | 33 (41.3) | 12 (52.2) | 21 (36.8) | 11 (42.3) | 22 (40.7) | 8 (30.8) | 25 (46.3) | |||||
1 | 47 (58.8) | 11 (47.8) | 36 (63.2) | 15 (57.7) | 32 (59.3) | 18 (69.2) | 29 (53.7) | |||||
Smoking status | 0.388 | 0.356 | 0.408 | |||||||||
Current | 27 (33.8) | 8 (34.8) | 19 (33.3) | 7 (26.9) | 20 (37.0) | 11 (42.3) | 16 (29.6) | |||||
Former | 31 (38.8) | 11 (47.8) | 20 (35.1) | 13 (50.0) | 18 (33.3) | 10 (38.5) | 21 (38.9) | |||||
Never | 22 (27.5) | 4 (17.4) | 18 (31.6) | 6 (23.1) | 16 (29.6) | 5 (19.2) | 17 (31.5) | |||||
Histology | 0.336 | 0.904 | 0.459 | |||||||||
Squamous | 25 (31.3) | 10 (43.5) | 15 (26.3) | 9 (34.6) | 16 (29.6) | 10 (38.5) | 15 (27.8) | |||||
Adenocarcinoma | 42 (52.5) | 10 (43.5) | 32 (56.1) | 13 (50.0) | 29 (53.7) | 12 (46.2) | 30 (55.6) | |||||
Other | 13 (16.3) | 3 (13.0) | 10 (17.5) | 4 (15.4) | 9 (16.7) | 4 (15.4) | 9 (16.7) | |||||
PD-L1 TPS | 0.502 | 0.866 | ||||||||||
TPS <1% | 26 (32.5) | 9 (39.1) | 17 (29.8) | – | – | – | 8 (30.8) | 18 (33.3) | ||||
1%≤ TPS <50% | 43 (53.8) | 10 (43.5) | 33 (57.9) | – | – | 15 (57.7) | 28 (51.9) | |||||
TPS ≥50% | 11 (13.8) | 4 (17.4) | 7 (12.3) | – | – | 3 (11.5) | 8 (14.8) | |||||
TMB (muts/Mb) | 7.29 [0.08–44.8] | 9.25 [0.08–35.78] | 6.53 [0.14–44.8] | 0.244 | 7.20 [0.78–26.13] | 7.42 [0.08–44.80] | 0.644 | – | – | – | ||
TMB <5 | 26 (32.5) | 6 (26.1) | 20 (35.1) | 0.671 | 8 (30.8) | 18 (33.3) | 0.767 | – | – | – | ||
5≤ TMB <20 | 49 (61.3) | 15 (65.2) | 34 (59.6) | 17 (65.4) | 32 (59.3) | – | – | |||||
TMB ≥20 | 5 (6.3) | 2 (8.7) | 3 (5.3) | 1 (3.8) | 4 (7.4) | – | – | |||||
Liver metastasis | 0.487 | 0.018 | 0.932 | |||||||||
Yes | 18 (22.5) | 4 (17.4) | 14 (24.6) | 10 (38.5) | 8 (14.8) | 6 (23.1) | 12 (22.2) | |||||
No | 62 (77.5) | 19 (82.6) | 43 (75.4) | 16 (61.5) | 46 (85.2) | 20 (76.9) | 42 (77.8) | |||||
Brain metastasis | 0.368 | 0.323 | 0.539 | |||||||||
Yes | 17 (21.2) | 3 (13.0) | 14 (24.6) | 9 (34.6) | 13 (24.1) | 6 (23.1) | 16 (29.6) | |||||
No | 63 (78.8) | 20 (87.0) | 43 (75.4) | 17 (65.4) | 41 (75.9) | 20 (76.9) | 38 (70.4) | |||||
Radiotherapy | 0.017 | 0.819 | 0.023 | |||||||||
Yes | 26 (32.5) | 12 (52.2) | 14 (24.6) | 8 (30.8) | 18 (33.3) | 4 (15.4) | 22 (40.7) | |||||
No | 54 (67.5) | 11 (47.8) | 43 (75.4) | 18 (69.2) | 36 (66.7) | 22 (84.6) | 32 (59.3) | |||||
KRAS mutation | 0.421 | 0.437 | ||||||||||
Yes | 23 (28.7) | – | – | – | 9 (34.6) | 14 (25.9) | 6 (23.1) | 17 (31.5) | ||||
No | 57 (71.3) | – | – | 17 (65.4) | 40 (74.1) | 20 (76.9) | 37 (68.5) |
The data are expressed as median [range] or n (%). KRAS, Kirsten rat sarcoma; ECOG, Eastern Cooperative Oncology Group; PS, performance status; PD-L1, programmed death-ligand 1; TPS, tumor proportion score; TMB, tumor mutational burden.
Survival analysis
The median OS for the entire cohort was 17.6 months (95% CI: 14.4–20.8 months). The 0.5-, 1-, and 2-year survival rates were 92.5%, 72.5%, and 43.8%, respectively. Thirty-five patients survived until the last follow-up. A total of 70 (87.5%) patients exhibited disease progression during the study period, with a median PFS of 9.8 months for all subjects (95% CI: 9.1–10.5). The 0.5-year and 1-year PFS rates were 67.5% and 38.0%, respectively. Patients in the KRAS mutant group had a median OS of 21.0 (95% CI: 19.4–22.6) months and PFS of 12.8 (95% CI: 9.8–15.8) months, and in the non-KRAS mutant group, patients had an OS of 15.9 (95% CI: 13.0–18.9) months and PFS of 9.7 (95% CI: 7.1–12.3) months. The OS and PFS of the KRAS mutant group were significantly greater than those of the non-KRAS mutant group (P<0.05) (Figure 3A,3B). However, no significant differences were observed in terms of 1- and 2-year survival rates between the KRAS mutant and non-KRAS mutant groups (87.0% vs. 66.7%, χ2=3.384, P=0.066; 56.5% vs. 38.6%, χ2=2.140, P=0.144).
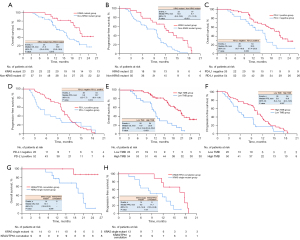
OS in the PD-L1-negative group was shorter than that in the PD-L1-positive group (15.2 vs. 20.6 months, P=0.009), while there were no significant differences in PFS between PD-L1-negative and PD-L1-positive groups among advanced NSCLC patients (5.6 vs. 10.7 months, P=0.214) (Figure 3C,3D). Upon further study, according to the TMB cutoff of 5 muts/Mb, the OS and PFS of the high-TMB group were significantly longer than those in the low-TMB group (20.8 vs. 11.9 months, P<0.001; 10.8 vs. 5.9 months, P=0.007) (Figure 3E,3F). In the KRAS mutant group, the KRAS single mutant and KRAS/TP53 co-mutation subgroups were divided by oncogene gene mutation status, revealing that the OS and PFS of the KRAS/TP53 co-mutation subgroup were significantly greater than those of the KRAS single mutant subgroup (P<0.05) (Figure 3G,3H). The results of survival analysis suggested that the survival of patients with advanced NSCLC without driver alterations who were treated with PD-1 blockade therapy along with chemotherapy as first-line treatment might have been subject to many confounding factors, including KRAS mutation status, PD-L1 expression, and TMB.
Univariate and multivariate analyses of the clinicopathologic variables of survival outcomes
Univariate analyses showed that non-brain metastasis, PD-L1 TPS ≥1%, TMB ≥5 muts/Mb, PD-L1 TPS ≥1% plus TMB ≥5 muts/Mb, KRAS mutation, and longer PFS were favorable for OS in qualified patients. The results of multivariate analyses showed that brain metastasis [hazard ratio (HR) =0.232, 95% CI: 0.102–0.530; P=0.001], TMB (HR =5.675, 95% CI: 1.948–16.535; P=0.001), KRAS mutation (HR =2.552, 95% CI: 1.141–5.708; P=0.023), and PFS (HR =0.822, 95% CI: 0.753–0.898; P<0.001) were independent predictors of OS in patients with advanced NSCLC who did not have driver alterations and who underwent PD-1 blockade therapy and platinum-based chemotherapy (Table 2). Additionally, univariate analyses revealed that non-liver metastasis, TMB ≥5 muts/Mb, PD-L1 TPS ≥1% plus TMB ≥5 muts/Mb, KRAS mutation, and KRAS/TP53 co-mutation were beneficial for PFS in the study cohort, while further multivariate analyses demonstrated that liver metastasis (HR =0.344, 95% CI: 0.191–0.619; P<0.001) and KRAS/TP53 co-mutation (HR =0.220, 95% CI: 0.067–0.725; P=0.013) had prognostic value for PFS in advanced NSCLC patients (Table 3).
Table 2
Variables | Univariable analysis | Multivariable analysis | |||||||
---|---|---|---|---|---|---|---|---|---|
HR | 95% CI | Wald | P value | HR | 95% CI | Wald | P value | ||
Sex, male vs. female | 1.083 | (0.425–2.761) | 0.028 | 0.867 | – | – | – | – | |
Age | 1.004 | (0.975–1.035) | 0.082 | 0.774 | – | – | – | – | |
ECOG PS score, 0 vs. 1 | 0.762 | (0.419–1.385) | 0.797 | 0.372 | – | – | – | – | |
Histology | |||||||||
Squamous vs. non-squamous | 1.566 | (0.861–2.849) | 2.158 | 0.142 | – | – | – | – | |
Adenocarcinoma vs. non-adenocarcinoma | 0.687 | (0.381–1.239) | 1.556 | 0.212 | – | – | – | – | |
Smoking status, never vs. current/former | 0.818 | (0.411–1.631) | 0.325 | 0.569 | – | – | – | – | |
Liver metastasis, no vs. yes | 0.571 | (0.279–1.167) | 2.359 | 0.125 | – | – | – | – | |
Brain metastasis, no vs. yes | 0.392 | (0.201–0.763) | 7.586 | 0.006 | 0.232 | (0.102–0.530) | 12.009 | 0.001 | |
PD-L1 TPS <1% vs. TPS ≥1% | 2.220 | (1.194–4.127) | 6.348 | 0.012 | 2.183 | (0.811–5.875) | 2.389 | 0.122 | |
TMB <5 muts/Mb vs. TMB ≥5 muts/Mb | 0.300 | (0.164–0.549) | 15.250 | 0.000 | 5.675 | (1.948–16.535) | 10.126 | 0.001 | |
PD-L1 <1% or TMB <5 muts/Mb vs. PD-L1 ≥1% and TMB ≥5 muts/Mb | 3.716 | (1.925–7.173) | 15.309 | 0.000 | 0.940 | (0.228–3.868) | 0.007 | 0.931 | |
PFS | 0.779 | (0.717–0.846) | 35.296 | 0.000 | 0.822 | (0.753–0.898) | 19.066 | 0.000 | |
Oncogene driver subgroup | |||||||||
Non-KRAS mutant vs. KRAS mutant | 2.471 | (1.210–5.047) | 6.169 | 0.013 | 2.552 | (1.141–5.708) | 5.205 | 0.023 | |
TP53 mutant vs. non-TP53 mutant | 0.902 | (0.485–1.677) | 0.106 | 0.744 | – | – | – | – | |
KRAS/TP53 co-mutation vs. others | 0.082 | (0.011–0.598) | 6.086 | 0.082 | – | – | – | – | |
Radiotherapy, no vs. yes | 1.487 | (0.788–2.806) | 1.498 | 0.221 | – | – | – | – |
ECOG, Eastern Cooperative Oncology Group; PS, performance status; PD-L1, programmed death-ligand 1; TPS, tumor proportion score; TMB, tumor mutational burden; PFS, progression-free survival; KRAS, Kirsten rat sarcoma; TP53, tumor protein p53; HR, hazard ratio; CI, confidence interval.
Table 3
Variables | Univariable analysis | Multivariable analysis | |||||||
---|---|---|---|---|---|---|---|---|---|
HR | 95% CI | Wald | P value | HR | 95% CI | Wald | P value | ||
Sex, male vs. female | 1.568 | (0.670–3.666) | 1.076 | 0.300 | – | – | – | – | |
Age | 1.007 | (0.984–1.032) | 0.364 | 0.546 | – | – | – | – | |
ECOG PS score, 0 vs. 1 | 0.778 | (0.480–1.262) | 1.035 | 0.309 | – | – | – | – | |
Histology | |||||||||
Squamous vs. non-squamous | 1.463 | (0.870–2.458) | 2.060 | 0.151 | – | – | – | – | |
Adenocarcinoma vs. non-adenocarcinoma | 1.017 | (0.633–1.635) | 0.005 | 0.943 | – | – | – | – | |
Smoking status, never vs. current/former | 0.822 | (0.472–1.430) | 0.481 | 0.488 | – | – | – | – | |
Liver metastasis, no vs. yes | 0.416 | (0.237–0.729) | 9.379 | 0.002 | 0.344 | (0.191–0.619) | 12.692 | 0.000 | |
Brain metastasis, no vs. yes | 0.629 | (0.363–1.089) | 2.738 | 0.098 | – | – | – | – | |
PD-L1 TPS <1% vs. TPS ≥1% | 1.384 | (0.825–2.324) | 1.515 | 0.218 | – | – | – | – | |
TMB <5 muts/Mb vs. TMB ≥5 muts/Mb | 1.990 | (1.196–3.310) | 7.017 | 0.008 | 1.668 | (0.830–3.349) | 2.067 | 0.151 | |
PD-L1 <1% or TMB <5 muts/Mb vs. PD-L1 ≥1% and TMB ≥5 muts/Mb | 1.658 | (1.024–2.683) | 4.232 | 0.040 | 1.493 | (0.758–2.941) | 1.343 | 0.247 | |
Oncogene driver subgroup | |||||||||
Non-KRAS mutant vs. KRAS mutant | 2.829 | (1.552–5.157) | 11.519 | 0.001 | 1.508 | (0.776–2.931) | 1.469 | 0.225 | |
TP53 mutant vs. non-TP53 mutant | 0.976 | (0.575–1.655) | 0.008 | 0.927 | – | – | – | – | |
KRAS/TP53 co-mutation vs. others | 0.169 | (0.059–0.483) | 11.019 | 0.001 | 0.220 | (0.067–0.725) | 6.197 | 0.013 | |
Radiotherapy, no vs. yes | 1.385 | (0.833–2.305) | 1.575 | 0.210 | – | – | – | – |
ECOG, Eastern Cooperative Oncology Group; PS, performance status; PD-L1, programmed death-ligand 1; TPS, tumor proportion score; TMB, tumor mutational burden; KRAS, Kirsten rat sarcoma; TP53, tumor protein p53.
Discussion
In this retrospective study, we explored whether KRAS mutation status predicted the effects of first-line ICI treatment and platinum-based chemotherapy in advanced NSCLC patients who did not carry any known oncogenic driver genes for targeted therapies. We also analyzed the correlation between clinicopathology and KRAS mutation status, PD-L1 expression, and TMB.
In our study, the median OS and PFS of the KRAS mutant group were significantly greater than those of the non-KRAS mutant group, which may indicate that the presence of KRAS mutation was correlated with longer PFS and OS in advanced NSCLC patients who underwent PD-1 blockade therapy and platinum-based chemotherapy in first-line treatment. Clinically, PD-L1 expression and TMB are most commonly used as predictive biomarkers for immunotherapy (17,18). In our study, 13.8% of the patients exhibited high expression of PD-L1 (TPS ≥50%), and more than half of the subjects expressed PD-L1 TPS between 1% and 50%. Meanwhile, the median TMB was 7.29 muts/Mb, which was higher than that reported from southwest China (5 muts/Mb) (19). Only 6.3% of the patients exhibited a TMB of ≥20 muts/Mb, with the highest TMB being 44.8 muts/Mb, and the TMB of most patients ranging from 5–20 muts/Mb. These results indicated that OS in the PD-L1-positive group was greater than that in the PD-L1-negative group, while OS and PFS in the group with TMB ≥5 muts/Mb were also prolonged.
The results of Cox multivariate analyses showed that brain metastasis, TMB, KRAS mutation status, and PFS were independent predictors of OS in our cohort, while liver metastasis and KRAS/TP53 co-mutation predicted PFS. Although the OS of the PD-L1-positive group (TPS ≥1%) showed favorable clinical outcomes (survival) with immunotherapy and chemotherapy compared to that of the PD-L1-negative group (20.6 vs. 15.2 months; P=0.009), the performance of PD-L1 was not satisfactory in subsequent Cox multivariate analyses. Our results suggested that patients with liver metastasis at the start of treatment tended to experience earlier disease progression, while those with brain metastasis had a lower OS rate. Interestingly, the baseline data showed that the rate of liver metastasis in the PD-L1-negative group was significantly higher than that in the PD-L1-positive group (38.5% vs. 14.8%, P=0.018). However, unlike liver metastasis, PD-L1 expression did not affect the PFS of patients treated with immunotherapy and chemotherapy when examined via Cox multivariate analyses.
In contrast to PD-L1 expression, high TMB (≥5 muts/Mb) and KRAS mutation had greater predictive value for OS in the advanced NSCLC cohort treated with immunotherapy and chemotherapy. Some studies have shown that NSCLC patients with KRAS mutations who were treated with PD-1 blockade may have greater survival due to the correlation between KRAS mutations and smoking (4,20), and other studies (21) have indicated that patients with KRAS mutations were more likely to be smokers than patients with non-KRAS mutations. However, in our study, we found no correlation between KRAS mutations and smoking status. In some previous studies (22,23), a significant correlation was found between smoking status and TMB, and smokers had higher TMB. In our study, no significant difference was observed between the low-TMB and high-TMB groups in terms of smoking status, nor were significant differences in the level of TMB between the KRAS and the non-KRAS mutant groups observed, which was inconsistent with the results reported by Xiang et al. of higher TMB in the KRAS mutant group (24). This suggests that KRAS mutation status and TMB level are 2 independent predictors of OS among patients treated with immunotherapy and chemotherapy. These results may also partly explain why the patients benefited from ICI therapy regardless of their PD-L1 status in previous trial (13). Additionally, we noted a high proportion of radiotherapy in both the KRAS mutant and TMB ≥5 muts/Mb groups (52.2% vs. 24.6%, P=0.017; 40.7% vs. 15.4%, P=0.023).
In this study, we enrolled patients in the KRAS mutant group with single KRAS mutations and with KRAS/TP53 co-mutations. We performed subgroup analysis of the KRAS mutation group and found that the OS and PFS of the KRAS/TP53 co-mutation group were significantly greater than those of the KRAS single mutant group, which was similar to the findings presented in The Cancer Genome Atlas (25). Moreover, Cox proportional hazards regression analyses showed that KRAS/TP53 co-mutation predicted PFS in this cohort, which indicated that patients with such co-mutations received a durable clinical benefit from immunotherapy and chemotherapy. However, this finding was obtained from a limited number of subjects during subgroup analysis, and larger prospective studies are needed to confirm these results. We also found a nearly identical ratio of major KRAS subtypes—G12C (5, 21.74%), G12V (5, 21.74%), and G12D (4, 17.39%)—as previously reported (26). Due to the limited number of patients, we were unable to conduct a separate analysis of each subtype.
The vast majority of the patients enrolled in this study were men, with only 11.3% of women, and the proportion of men in the KRAS mutant group was 91.3%. In total, more than half of the patients enrolled in the study cohort had adenocarcinomas, which may be related to the high incidence of adenocarcinoma itself. Furthermore, the likelihood of adenocarcinoma with gene mutation is higher in East Asian populations In this study, we did not observe any differences in pathological types between the KRAS and non-KRAS mutant groups. A similar proportion of squamous cell carcinoma and adenocarcinoma were found in the KRAS mutant group, while most of the studies available in the literature have reported that KRAS mutations mainly occur in adenocarcinoma (27).
Our study has several limitations. First, since our study was a retrospective study, selection bias was inevitable. Second, all of our molecular tests were conducted at a single institution. Not all patients who had a clinical and pathological diagnosis of NSCLC in our hospital received oncogenic driver gene detection due to the high cost of molecular detection; therefore, the patients enrolled in our cohort may not represent the general population. The lack of monochemotherapy as a control group and small number of patients were also limitations that must be factored into any conclusions. Considering these limitations, multicenter, prospective, randomized, controlled trials with a greater number of patients should be conducted to validate our findings. At the current time, Sotorasib and other KRAS directed therapy are being investigated in patients with KRAS G12C mutated locally advanced or metastatic NSCLC who have received at least one prior systemic therapy. As the development of KRAS directed therapy continues in the clinic and moves to earlier lines of therapy alone or in combination with chemoimmunotherapy, it will be of interest to the medical and scientific community as well as patients to understand the implications of KRAS status on selection and sequencing of therapy in this group of patients and this should be considered in the design of prospective trials.
Conclusions
In this retrospective study of 80 advanced (stage IV) NSCLC patients who did not carry oncogenic driver genes for targeted therapies and who received ICIs combined with chemotherapy as first-line treatment, those of KRAS mutant group had greater PFS and OS relative to those of the non-KRAS mutant group. TMB and KRAS mutation status were identified as independent predictors of OS in the studied cohort, which suggests that they may be useful as predictive biomarkers for immunotherapy efficacy. Patients with liver metastasis tended to experience an earlier disease progression, while those with brain metastasis had lower OS rates.
Acknowledgments
The authors appreciate the academic support from the AME Lung Cancer Collaborative Group.
Funding: This work was supported by the Science and Technology Program of Guangzhou (No. 201803010024).
Footnote
Reporting Checklist: The authors have completed the STROBE reporting checklist. Available at https://tlcr.amegroups.com/article/view/10.21037/tlcr-22-655/rc
Data Sharing Statement: Available at https://tlcr.amegroups.com/article/view/10.21037/tlcr-22-655/dss
Conflicts of Interest: All authors have completed the ICMJE uniform disclosure form (available at https://tlcr.amegroups.com/article/view/10.21037/tlcr-22-655/coif). The authors have no conflicts of interest to declare.
Ethical Statement: The authors are accountable for all aspects of the work in ensuring that questions related to the accuracy or integrity of any part of the work are appropriately investigated and resolved. The study was conducted in accordance with the Declaration of Helsinki (as revised in 2013). The study was approved by ethics committees of Guangzhou Institute of Respiratory Health (2021 No. K-15), and individual consent for this retrospective analysis was waived.
Open Access Statement: This is an Open Access article distributed in accordance with the Creative Commons Attribution-NonCommercial-NoDerivs 4.0 International License (CC BY-NC-ND 4.0), which permits the non-commercial replication and distribution of the article with the strict proviso that no changes or edits are made and the original work is properly cited (including links to both the formal publication through the relevant DOI and the license). See: https://creativecommons.org/licenses/by-nc-nd/4.0/.
References
- Sung H, Ferlay J, Siegel RL, et al. Global Cancer Statistics 2020: GLOBOCAN Estimates of Incidence and Mortality Worldwide for 36 Cancers in 185 Countries. CA Cancer J Clin 2021;71:209-49. [Crossref] [PubMed]
- Zappa C, Mousa SA. Non-small cell lung cancer: current treatment and future advances. Transl Lung Cancer Res 2016;5:288-300. [Crossref] [PubMed]
- Hanna NH, Schneider BJ, Temin S, et al. Therapy for Stage IV Non-Small-Cell Lung Cancer Without Driver Alterations: ASCO and OH (CCO) Joint Guideline Update. J Clin Oncol 2020;38:1608-32. [Crossref] [PubMed]
- Borghaei H, Paz-Ares L, Horn L, et al. Nivolumab versus Docetaxel in Advanced Nonsquamous Non-Small-Cell Lung Cancer. N Engl J Med 2015;373:1627-39. [Crossref] [PubMed]
- Herbst RS, Baas P, Kim DW, et al. Pembrolizumab versus docetaxel for previously treated, PD-L1-positive, advanced non-small-cell lung cancer (KEYNOTE-010): a randomised controlled trial. Lancet 2016;387:1540-50. [Crossref] [PubMed]
- Topalian SL, Taube JM, Anders RA, et al. Mechanism-driven biomarkers to guide immune checkpoint blockade in cancer therapy. Nat Rev Cancer 2016;16:275-87. [Crossref] [PubMed]
- Goodman AM, Kato S, Bazhenova L, et al. Tumor Mutational Burden as an Independent Predictor of Response to Immunotherapy in Diverse Cancers. Mol Cancer Ther 2017;16:2598-608. [Crossref] [PubMed]
- Le DT, Uram JN, Wang H, et al. PD-1 Blockade in Tumors with Mismatch-Repair Deficiency. N Engl J Med 2015;372:2509-20. [Crossref] [PubMed]
- Tang H, Wang Y, Chlewicki LK, et al. Facilitating T Cell Infiltration in Tumor Microenvironment Overcomes Resistance to PD-L1 Blockade. Cancer Cell 2016;29:285-96. [Crossref] [PubMed]
- Takashima A, Faller DV. Targeting the RAS oncogene. Expert Opin Ther Targets 2013;17:507-31. [Crossref] [PubMed]
- Sun X, Li K, Zhao R, et al. Lung cancer pathogenesis and poor response to therapy were dependent on driver oncogenic mutations. Life Sci 2021;265:118797. [Crossref] [PubMed]
- Ostrem JM, Shokat KM. Direct small-molecule inhibitors of KRAS: from structural insights to mechanism-based design. Nat Rev Drug Discov 2016;15:771-785. [Crossref] [PubMed]
- Rittmeyer A, Barlesi F, Waterkamp D, et al. Atezolizumab versus docetaxel in patients with previously treated non-small-cell lung cancer (OAK): a phase 3, open-label, multicentre randomised controlled trial. Lancet 2017;389:255-65. [Crossref] [PubMed]
- Mazieres J, Drilon A, Lusque A, et al. Immune checkpoint inhibitors for patients with advanced lung cancer and oncogenic driver alterations: results from the IMMUNOTARGET registry. Ann Oncol 2019;30:1321-8. [Crossref] [PubMed]
- Jeanson A, Tomasini P, Souquet-Bressand M, et al. Efficacy of Immune Checkpoint Inhibitors in KRAS-Mutant Non-Small Cell Lung Cancer (NSCLC). J Thorac Oncol 2019;14:1095-101. [Crossref] [PubMed]
- Yarchoan M, Hopkins A, Jaffee EM. Tumor Mutational Burden and Response Rate to PD-1 Inhibition. N Engl J Med 2017;377:2500-1. [Crossref] [PubMed]
- Rimm DL, Han G, Taube JM, et al. A Prospective, Multi-institutional, Pathologist-Based Assessment of 4 Immunohistochemistry Assays for PD-L1 Expression in Non-Small Cell Lung Cancer. JAMA Oncol 2017;3:1051-8. [Crossref] [PubMed]
- Gandara DR, Paul SM, Kowanetz M, et al. Blood-based tumor mutational burden as a predictor of clinical benefit in non-small-cell lung cancer patients treated with atezolizumab. Nat Med 2018;24:1441-8. [Crossref] [PubMed]
- Ma Y, Li Q, Du Y, et al. Tumor Mutational Burden and PD-L1 Expression in Non-Small-Cell Lung Cancer (NSCLC) in Southwestern China. Onco Targets Ther 2020;13:5191-8. [Crossref] [PubMed]
- Rizvi NA, Hellmann MD, Snyder A, et al. Cancer immunology. Mutational landscape determines sensitivity to PD-1 blockade in non-small cell lung cancer. Science 2015;348:124-8. [Crossref] [PubMed]
- Chapman AM, Sun KY, Ruestow P, et al. Lung cancer mutation profile of EGFR, ALK, and KRAS: Meta-analysis and comparison of never and ever smokers. Lung Cancer 2016;102:122-34. [Crossref] [PubMed]
- Zhang XC, Wang J, Shao GG, et al. Comprehensive genomic and immunological characterization of Chinese non-small cell lung cancer patients. Nat Commun 2019;10:1772. [Crossref] [PubMed]
- Kytola V, Topaloglu U, Miller LD, et al. Mutational Landscapes of Smoking-Related Cancers in Caucasians and African Americans: Precision Oncology Perspectives at Wake Forest Baptist Comprehensive Cancer Center. Theranostics 2017;7:2914-23. [Crossref] [PubMed]
- Xiang L, Fu X, Wang X, et al. A Potential Biomarker of Combination of Tumor Mutation Burden and Copy Number Alteration for Efficacy of Immunotherapy in KRAS-Mutant Advanced Lung Adenocarcinoma. Front Oncol 2020;10:559896. [Crossref] [PubMed]
- Dong ZY, Zhong WZ, Zhang XC, et al. Potential Predictive Value of TP53 and KRAS Mutation Status for Response to PD-1 Blockade Immunotherapy in Lung Adenocarcinoma. Clin Cancer Res 2017;23:3012-24. [Crossref] [PubMed]
- Jia Y, Jiang T, Li X, et al. Characterization of distinct types of KRAS mutation and its impact on first-line platinum-based chemotherapy in Chinese patients with advanced non-small cell lung cancer. Oncol Lett 2017;14:6525-32. [Crossref] [PubMed]
- Jordan EJ, Kim HR, Arcila ME, et al. Prospective Comprehensive Molecular Characterization of Lung Adenocarcinomas for Efficient Patient Matching to Approved and Emerging Therapies. Cancer Discov 2017;7:596-609. [Crossref] [PubMed]