Significance of circulating tumor cells in lung cancer: a narrative review
Introduction
Although great progress has been made in the treatment of cancer patients, metastatic disease is frequently not amenable to any further therapy (1,2). Metastasis takes place in several steps comprising cancer cell intravasation, migration, extravasation and establishment of secondary lesions (Figure 1). Because the course of tumor metastasis is difficult to follow in patients, the sequence of steps resulting in metastases has not been elucidated in detail so far (3). Primary tumors shed cells into the circulation, termed circulating tumor cells (CTCs), which migrate and spread to distant sites to establish metastases (4). Therefore, metastasis represent the growth of distinct cancer cells that are present in parent tumors (5). Discrete genetic clones eventually forming metastases can be generated in tumors early, preceding the diagnosis of cancer (6). CTCs were suggested as potential founders of metastatic lesions more than 100 years ago (7). These cells are found in most advanced malignancies, although their numbers are relatively low in the circulation except for small cell lung cancer (SCLC) and inflammatory breast cancer (IBC) (8,9). Counting and molecular characterization of CTCs were expected to allow for early detection of tumor dissemination and for personalized therapy according to tumor markers and chemosensitivity (10). The techniques to identify CTCs represent a type of “Liquid Biopsy” that can be repeated easily to follow the course of the disease in contrast to invasive biopsies (10,11). However, the latest approaches to CTCs have concentrated largely on the different methods of enrichment of these rare cells and correlation to prognosis and response to therapy although basic questions such as the mechanisms of the release of tumor cells, their fate in the circulation, the characteristics of metastasis-inducing CTCs and their ways of extravasation are not fully characterized (Figure 1). This review concentrates on the role of CTCs in SCLC and non-small cell lung cancer (NSCLC) as well as the involvement of the angiogenic switch in shedding of CTCs. SCLC and NSCLC represent two extremes of a high and low count of CTCs, respectively. We present the following article in accordance with the Narrative Review reporting checklist (available at https://tlcr.amegroups.com/article/view/10.21037/tlcr-22-712/rc).
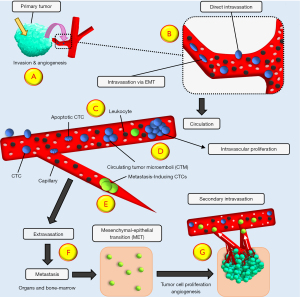
Methods
This narrative literature review used publications cited in PubMed (https://pubmed.ncbi.nlm.nih.gov/) and EuroPMC (https://europepmc.org/), as well as own work, to identify studies and literature reviews covering SCLC using specific terms (Table 1). Studies published in peer reviewed journals were used. Specific terms used include small cell lung cancer (SCLC), non-small cell lung cancer (NSCLC), circulating tumor cells (CTCs) and angiogenesis.
Table 1
Specification | Items |
---|---|
Date of search | September 26th, 2022 |
Timeframe | January 1st, 2015 to September 23th, 2022 |
Databases and other sources searched | PubMed, Euro PMC and own work |
Search terms used | “SCLC”, “NSCLC”, “CTC” and “Angiogenesis” |
Inclusion and exclusion criteria | Original Investigations and Reviews in English language were included |
Selection process | Selected by all authors |
Detection and enrichment of CTCs
Tumors shed cancer cells but CTCs are rare even in patients with malignancies (12,13). The numbers of CTCs range from a few cells to a few hundred CTCs per 10 mL blood with exception of SCLC and Inflammatory Breast Cancer (IBC) (8,9). The daily shedding of 1–4 million CTCs per gram of tumor tissue has been reported for a breast cancer xenograft model that may not truly reflect actual human tumors (14,15). As cancer cells are heterogeneous, CTCs may have large variations in the expression of surface biomarkers (16). Therefore, strategies have been developed for the enrichment and detection of CTCs using techniques either based on cell surface markers or physical characteristics of the cancer cells (17). Despite of a large number of methods described, approval of the FDA is limited to the CellSearch© system, that detects CTCs according to their expression of EpCAM using 7.5 mL of blood (18). The CellSearch© system employs on immunomagnetic enrichment of EpCAM-positive tumor cells and confirmation of their characteristics by a demonstration of the presence of cytokeratins, a positive DAPI nuclear staining and negativity for the leucocyte marker CD45 (18,19). A poorer prognosis has been predicted for patients exhibiting CellSearch© CTC counts of more than 5, 5 and 3 CTCs/7.5 ml blood for breast, pancreatic and colon cancer, respectively. The CTC marker EpCAM is a transmembrane glycoprotein that is expressed by the majority of breast, colorectal, and prostate cancers but is absent in blood cells (16). Alternative CTC antigens such as keratin 19, tumor-specific antigen 9, and progastrin-releasing peptides have been reported (20). The detection of CTCs is challenging due to their extremely low frequency in the circulation. Even in metastatic disease, the CellSearch© system failed to find CTCs in ~20% of prostate, ~25% of breast, and ~50% of colon cancer patients (21). Furthermore, the CellSearch© detection system dismisses EpCAM-negative CTC subpopulations of putative significance for tumor dissemination.
Marker-independent CTC detection techniques rely on physical characteristics of CTCs such as cell size, deformability, electric charge, flow properties and others (22). The relatively small size differences between leukocytes (6.2–9.4 µm), leukemic blood cells (8.9–15.3 µm) and solid tumor cells (11.7–23.8 µm) complicate the separation of CTCs (23). These variant approaches detect larger numbers of CTCs of potential aggressive and invasive properties compared to the CellSearch© system (17,24,25). Therefore, these methods recognize CTCs with low or negative EpCAM expression in contrast to the CellSearch© (9). Size-dependent CTC enrichments comprise “Isolation by the size of epithelial tumor cells” (ISET®), diverse filtration devices, such as Metacell, ScreenCellCyto and the Parsortix™ system, among others. A host of recent reviews summarize the wide range of techniques to assess CTCs in various malignant diseases (26-28). However, in contrast to cell-free DNA (cfDNA; circulating DNA fragments released by tumor cells), enriched CTCs constitute viable tumor cells that can be tested for DNA, RNA and protein expression (29). Additionally, CTC analysis at single-cell resolution has been reported to offer valuable insights into tumor heterogeneity (28). Due to clinical and technical restrictions, the precision of CTC detection methods by repetitive sampling has been rarely confirmed.
CTCs should become trapped in capillaries because their size is larger than the diameter of microvessels (30). However, the small SCLC CTCs may pass into capillaries and clusters consisting of several tumor cells were reported to migrate as single-file chain-like cell assembly (31,32). In addition to single cell CTCs, Circulating Tumor Microemboli (CTM)/Clustered Circulating Tumor Cells (CTC) can be detected in cancer patients (33). These CTMs were suggested to stem from the intravasation of tumor cells that have migrated as cluster and have entered the circulation via “leaky” and irregular tumor vessels that are a characteristic of highly angiogenic tumors (8,33).
Breast cancer cells monitored through cancer progression in an experimental model showed that the majority of CTC clusters have undergone hypoxia, while single CTCs are mostly normoxic (34). Inhibition of VEGF results in tumor shrinkage, but elevates intratumoral hypoxia, with increased release of CTC clusters shedding and formation of metastases. Tumor hypoxia and defective angiogenesis are key factors affecting cancer progression (35). In a tumor, hypoxia in the core and poorly vascularized regions generally upregulate cell-cell junction proteins of cancer cells, promoting intravasation of CTCs clusters rather than individual CTCs. In breast cancer, clusters of heterogenous tumor cells have been reported to hold a markedly increased metastatic potential (36). Tumor cell clusters are rapidly cleared from the circulation and their high metastatic potential is possibly associated with their trapping in microvessels (31,37). Tumor colonies were primarily released in a murine model by breast cancer xenografts rather than generated by aggregation in the circulation (36). These CTC clusters comprise 2 to 50 tumor cells, fibroblasts, endothelial cells and leukocytes with improved survival in the circulation in addition to the higher metastatic potential (36). Arrest of cancer cells in microvessels may lead to the formation of firm attachments, such directing CTCs to specific organs (Figure 1) (38). Liver and brain tissues containing a high number of small capillaries are a frequent target for metastatic seeding (39). Some CTCs interact with platelets to form a shell around them most likely protecting them from shear forces in the circulation (26,33,40-42). CTCs survive in the circulation for very short times, typically less than 24 h. Driemel et al. reported that that the expression of EpCAM by CTCs correlates positively with high proliferative activity (43). A contradictory investigation found low expression of the proliferative marker Ki-67 in CTCs, indicating a dormant state (44). Elimination of CTCs from circulation seem to be effected by immune cell attacks, shear stress, anoikis and the absence of growth factors (45,46). A fraction of CTCs is capable of colonizing distant organs and to persist as disseminated tumor cells (DTCs) that in rare cases progress to establish metastases. The fate of DTCs in regard to survival, growth and resistance to therapy is dependent on the tumor microenvironment.
The numbers of CTCs and DTCs are highly correlated, although the frequency of DTCs was mostly higher (46). Blood analyses seem to represent only a ‘snapshot’ of tumor cell dissemination (47). The major difficulties for an early tumor detection is the rarity of CTCs at the nonmetastatic stage and the low blood volume (<10 mL) available for routine tests (24,25,48). Since CTCs are absent in many metastatic patients and only a very small fraction of CTCs has metastatic potential, CTC counting alone is insufficient in staging and prognosis of the malignant diseases. Biomarkers of metastasis-inducing CTC subsets that assess homing and colonization potential need yet to be identified (49-51).
CTCs and tumor dissemination
Tumor cell dissemination comprises invasion through the basement membrane during malignant progression, intravasation into blood, survival in the circulation defending immune cells and shear stress, arrest in capillaries of organs and extravasation followed by formation of micrometastases and secondary lesions (Figure 1) (52-54). It has been calculated that no more than 0.01% of CTCs are capable of setting metastases (45). Evidence for a metastatic potential of CTCs has been derived from breast cancer xenografts and enriched CTC populations from SCLC that are tumorigenic in immunocompromised mice (8,49).
The small fraction of CTCs with metastases-inducing properties has been explained by destruction of CTCs in the circulation by shear stress and immunological attack as well as a slow rate of extravasation at a secondary organ (5,55). In respect to shear stress, CTCs survive the size-dependent enrichment by the Parsortix system that relies on the pumping of blood through a chip with 6–10 µm restrictions at a pressure that is at least comparable to the diastolic blood pressure (99 mbar) (13,56). At least in case of SCLC with excessive numbers of SCLC the CTCs seem to be less immunogenic despite a host of mutations induced by tobacco consumption (57). A report has described the frequent extravasation of CTCs that eventually remain inactive in the target tissue (39). Furthermore, with exception of SCLC, IBC and heavily metastasized patients, the actual CTC count is low due to a low rate of shedding. The presence of CTCs alone does not prove metastasis, since most CTCs are rapidly removed from blood and extravasation at distant sites ends unproductively in most instances. Cellular immobilization in capillaries frequently results in cell death, dormancy as DTC and very rarely in clinically detectable metastases (5).
The identification of the fraction of rare CTCs which have the potential to initiate metastases is difficult. From data of 38,715 breast cancer patients a tumor doubling time of 1.7±0.9 months was calculated between detection of a primary tumor to overt metastasis (14). For T1B breast cancer cases, a metastatic efficiency of 1 metastasis formed per 60 million CTCs was estimated. Thus, the primary tumor would have to be removed at a size of 3 mm, corresponding to 9±6 CTC/L to prevent dissemination. Firstly, the tumor will not be detected clinically and, secondly, the sensitivity of CTC detection would have to be improved manifold and combined with techniques reducing false positive results. In conclusion, it will not be possible in most cases to find and characterize the primary metastasis-inducing CTCs that exist long before the tumor is detected in clinics. The high numbers of CTCs in metastasized patients may likewise stem from the primary tumor and all secondary lesions.
Mechanisms of the intravasation of CTCs
Intravasation via EMT
Solid tumor cells are supposed to intravasate via passive or active approaches (58,59). The frequently used term “shedding” for this release of tumor cells into the circulation is an indicator of the lack of detailed knowledge. Most CTCs may be released by mechanical forces due to tumor growth or surgery (60). In one proposed model of intravasation, cells at the invasive front of tumors undergo epithelial-mesenchymal transition (EMT) and enter nearby blood vessels (Figure 1). The mesenchymal phenotype is distinguished by spindle-shaped cells with higher expression of vimentin and downregulation of cell adhesion molecules that may actively cross the tumor stroma and enter blood vessels (35,61,62). Thus, EMT constitutes a cell regulatory program which enables selected epithelial cells to acquire invasive potency and resistance to cell death for dissemination (58,63-66). For single cells and small clusters, a mesenchymal spindle-cell morphology facilitates the migration through stromal tissue and in the periphery cells after EMT seem to be more tolerant to shear stress by the expression of vimentin and α-actin (39). EMT of cancer cells seem to be triggered by cytokines released from tumor-infiltrating immune cells (67). Tumor cell intravasation mediated by macrophages appear in breast cancer in the absence of angiogenesis (68).
The linear intravasation model states that some cancer cells mutate and develop the preconditions for invasiveness (69,70). Accordingly, EMT may constitute a bottleneck for recruiting CTCs at the invasive front of tumors delaying the dissemination after some time of tumor development (61,71,72). Accordingly, dissemination of cancer cells via EMT occurs later in tumor development and would require a reversal of EMT via mesenchymal-epithelial transition (MET) after extravasation since metastases of epithelial tumors are EpCAM-positive again (4,73). The support for the EMT-mediated intravasation comes mainly from in vitro studies and experimental animal models but the in vivo data favoring this model are scarce and a partial alteration in the expression of mesenchymal markers does not indicate a true phenotypic switch (71,74). Fractions of EpCAM-negative CTCs that had undergone (partial) EMT have been claimed to possess increased metastatic potential and chemoresistance as well as greater prognostic value. CTC detection methods relying on epithelial EpCAM would miss this potentially critical CTC subpopulation (18).
EMT is seldom detected in tumor pathological preparations, although a high number of these invading cancer cells should be present, and it remains debatable whether this in vitro model has an in vivo counterpart (71,73,75,76). That cells bearing a host of random gene mutations intravasate, survive immune cell attacks, extravasate at distal sites and eventually recapitulate an intact epithelial phenotype seems unlikely (58,73). Moreover, many changes in protein expression associated with EMT are detectable in most non-metastatic benign tumors (77). The significance of EpCAM-positive EpCAM-low CTCs has been investigated by correlating CellSearch© and ISET detection with the prognosis of cancer patients (78,79). Approximately 70% of prostate cancer patients and 64% of breast cancer patients had in total ≥5 EpCAM-positive and/or EpCAM-low CTCs and castration-resistant prostate cancer patients with ≥5 EpCAM-positive CTC had shorter overall survival versus those with <5 EpCAM-positive CTC. Observations in NSCLC, breast and prostate cancer demonstrated that both EpCAM+ and EpCAM− CTC populations are frequently present. However, in patients with metastatic NSCLC and prostate cancer EpCAM+, CK+ CTC, and not the EpCAM−, CK+ are correlated with a poor outcome (80). In conclusion, results of these experiments indicate that the presence of EpCAMlow CTC had no relation with overall survival.
Intravasation via core tumor vessels
Furthermore, clinical and experimental data suggest early tumor dissemination shortly after neoangiogenesis and intravasation of tumor cells in the tumor core region (81). CTCs directly intravasate through fenestrated and irregular tumor vessels and, therefore, the metastasis-initiating cells may establish secondary lesions a long time before the clinical detection of cancer (Figure 1). This model of cancer cell intravasation fits better to the intravasation of larger CTC clusters and apoptotic CTCs. Furthermore, this eliminates the necessity for a complex EMT/MET phenotypic change and the movement of groups of CTCs as single files through tissue. Intravasation of CTCs via EMT after active crossing of tumor stroma is expected to result in fully viable and not apoptotic cells. Marker-independent CTC enrichment techniques demonstrate a variety of CTC EMT partial phenotypes with minor upregulation in vimentin and downregulation in E-cadherin without a true cell-type transformation (82,83). Furthermore, the number of CTCs that have intravasated seems to be correlated to the microvessel density of the tumor but not to the tumor mass.
Intravasation in the tumor core occurs early and high numbers of CTCs may disseminate into the blood circulation via passive shedding (81,84-86). Single DTCs exist in the lymph nodes or bone marrow of women with a history of early-stage breast cancer that show no clinical of metastasis or tumor recurrence (81). Peaks of the release of CTCs seem to correspond to the time angiogenic switch during the early, preinvasive stage of tumor growth (87). Intravasation of CTCs is facilitated by the irregular and leaky blood vessels formed during the tumor neoangiogenetic process (38,88). Programmed necrosis (necroptosis) of endothelial cell induced by tumor cells may be involved in this process (89). Leaky vessels in the primary tumor facilitate the collective migration and intravasation of CTC clusters with less resistance compared to an EMT-mediated migration in solid tumor stroma.
Intravasation cannot be fully simulated in vitro and is rarely observable in vivo but can be modeled in appropriate animal experiments (68). Deryugina and Kiosses investigated the structure of intravasated cells within fibrosarcomas in mice ears and chick embryos and localized intravasation almost exclusively in the tumor core and intratumoral vasculature (81,90,91). The great majority of intravasation events was found within the new and immature blood vessels in the tumor core, completely independent from any putative intravasation of the invasive front. It was concluded that possible vasculotropic cancer cells invading the tumor-adjacent stroma and intravasate into coalescing blood vessels are of minor significance for cancer dissemination. This type of intravasation forwards the generation of metastases, immediately after the first neoangiogenic vessels are formed (92). These blood vessels are typically aberrant, marked by irregular branching, distorted structure, intermittent blood flow, leakiness, and abnormal levels of endothelial cell death (88). The size of such pores of neoangiogenetic tumor vessels varies from 100 nm to 2 µm depending on the tumor entity (60,93).
The quantification of human cancer growth rates in the past showed that metastasis must be initiated a long time before the primary tumor diagnosis because metastases were too large to be initiated at a late stage of tumor history (14,85,94). Tumor volume doubling times of approximately 60–200 days are up to two times faster for metastatic lesions (85,95). For breast cancer, a median time from resection to distant metastasis of 35 and 20 months has been calculated for patients with T1 and T3 tumors, respectively, instead of a presupposed time span of 12 years (96,97). Early release of CTCs fits better to the observed time course of tumor development by setting the start of tumor dissemination to several years before actual primary tumor diagnosis. Furthermore, the clonal diversity of DTCs which may require prolonged time to develop again indicates an early dissemination of CTCs. Accordingly, the occurrence of several large brain metastases in connection with a very small primary tumor is quite frequently reported (98). Furthermore, cancers of unknown primary sites account to up of 5–10% of diagnoses (99).
Experimental patient-derived xenograft (PDX) models
CTCs enriched by negative selection have been grown in immunocompromised mice as CTC-derived xenografts (CDX) (100). CDXs derived from different patients shared genomic alterations, but showed marked heterogeneity within tumors and between distinct PDX (101,102). As drawbacks, SCLC PDX are not administered orthotopically, develop under murine background and lack interaction with human immune effector cells (103). Our own experiments have demonstrated tumorigenicity of the first SCLC permanent CTC lines (BHGc7, 10 and 16) in NOD-SCID mice but high variability of marker expression and chemosensitivity of recultivated PDX suspensions in dependence of time to tumor formation and specific localization of the PDX (unpublished observation).
The scarcity of actual metastasis-inducing CTCs has hindered a characterization of these CTCs in vitro, except in short term expansion and as PDX (19). However, SCLC may display an excessive number of CTCs in advanced stage and this allowed us to establish 8 permanent CTC lines in vitro which could be employed to characterize several aspects of CTCs (104-108). Provided that the calculations giving the frequency of metastasis-inducing CTCs as 0.01% are valid then extremely high CTC counts are necessary to have a few of such cancer cells present. This condition seems to be fulfilled only for extensively metastasized patients and, especially for SCLC. Our SCLC CTC cell lines have been established from 10 ml blood of patients with extended disease containing several hundreds to thousands of CTCs in total. A recent overview of the published attempts to culture CTCs of different tumor entities has been published by Shimada et al. (109). It was concluded that negative selection of CTCs, hypoxia, 3D growth conditions are necessary for the long-term culture of CTC although no definite methods are described. Furthermore, CTCs were assigned hybrid epithelial/mesenchymal phenotypes and characteristics of stem cell-like properties. Yu et al. previously suggested that CTC perish through senescence after a few cell divisions in monolayer cultures and growth in suspension and hypoxia are required as implemented for stem cell cultures (51).
In most cases, CTC were kept in short-term cultures, whereas our panel of SCLC CTC cell lines has now been cultivated from 1–4 years so far. These cell lines keep their characteristics in respect to morphology, growth rate and phenotype, SCLC markers, chemosensitivity and expression of proteins. The lines were established in 2D culture under normoxic conditions without enrichment since normal blood cells perish in cell culture and cancer cells are left. Hypoxic conditions and 3D culture seems not to be appropriate for cells having existed in the circulation but may be suitable for DTCs. The SCLC CTC panel does not express stem cell antigens or characteristics and all of these cells exhibit high levels of EpCAM (110). These SCLC CTC cells are unique in spontaneous generation of large tumorospheres containing resistant quiescent and hypoxic cells in their interior (Figure 2) (104). The permanent growth of these pure and tumorigenic SCLC CTC populations indicates their representation of stable metastasis-inducing cancer cell lines.
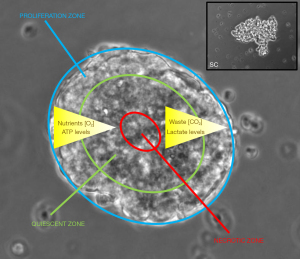
Extravasation
The molecular mechanisms that regulate extravasation are poorly characterized (111). Coumans et al. have developed a model for primary breast cancer to estimate the size of the tumor and corresponding CTC numbers before formation of the first metastases (14). The CTC number for breast cancer before surgery has been calculated as 0.03 CTC/mL (14). The CTC mean intravasation rate of a 8 mm tumor is 280 CTC/h · g tumor indicating a requirement of approximately 60 million CTCs for the establishment of a single metastases formed. Although a metastatic efficiency rate has not been finally determined in humans, a fraction of under 0.01% of the CTCs has been suggested to set secondary lesions, most likely due to cell death in the circulation and non-productive extravasation.
The extravasation of CTCs is a complex process, that is initiated with the adhesion of CTCs to the endothelium of blood vessel and progresses with transendothelial migration to establish secondary lesions in distant organs (Figure 1). CTCs may pass through the endothelial junctions forcefully one at a time (112). An alternative mechanism, angiopellosis, consists of active remodeling of vascular ECs to form a sheet around cancer cells and active expulsion of the CTCs out of the circulation (113). After becoming trapped in capillaries, CTCs first form weak adhesions to the endothelium, involving several pairs of cell ligands and receptors, comprising selectins, cadherins, integrins, and immunoglobulins (114). First contact of CTCs to the endothelium is mediated by neuronal cadherin (N-cadherin) that is expressed by both cancer cells and endothelial cells (115). In addition, the flow rate and shear force trigger remodeling of the ECs and promote extravasation (116). Tumor cells trapped in the pulmonary capillaries initially show luminal proliferation but intravascular colonies eventually rupture the capillary wall and allow for the invasion of the cancer cells into host tissue (117,118).
Detection of early metastatic disease and prognosis
Blood-borne dissemination of cancer cells seems to take place early in tumor development, which may commend CTCs as marker for early detection. CTCs are scarce in non-metastatic patients and, therefore, detection for CTCs at early stages is not sensitive enough, especially limited by the larger amounts of blood required (119). Whereas few CTC-like cells were detected in healthy donors and for benign diseases a large range of CTC occurs in cancer patients (120). CTCs may be missed by sampling small blood volumes or released intermittently and avoid detection (98). The CTC detection cutoff in nonmetastatic patients has been set at ≥1 CTC/7.5 mL of blood for the CellSearch© system, in contrast to the cutoff in the metastatic setting with ≥5 CTC/7.5 mL of blood. The closest association between CTC count and tumor size is found for T4d inflammatory breast cancers. In a multicenter analysis of 3,173 patients with localized breast cancer using CellSearch© the percentage of patients with at least one CTC was 20%, while at least five CTCs were detected in half of the patients with metastatic disease (121).
For the CellSearch© system, the prognostic CTC limit for colon, breast and prostate cancer patients has been reported as 3–5 CTCs/7.5 mL blood suggesting a limited shedding of tumor cells (18). In case of COPD, detection of premalignant CTCs has been reported (122). However, the frequency of a malignant progression in COPD patients was approximately 1 in 200 patients and a large number of people need to be screened with considerable effort to detect a few high risk patients (123). Ilie et al. reported that among 168 COPD patients followed annually with spiral CT scans, all 5 positive patients developed lung cancer during follow-up (122). The application of “Isolation by Size of Epithelial Tumor Cells” (ISET) for selection of CTCs to differentiate benign from malignant lung lesions using a cutoff of 25 cells detected CTCs in 90% of patients with malignant and 5% of patients with benign disease (124,125). The ISET method proved insensitive to predict the subsequent development of cancers, as only 2 of the 13 lung cancers and 0 of the 13 extrapulmonary cancers found within 2 years had positive CTC counts. So far, the search for CTCs has not been demonstrated to be useful in cancer screening. The pilot study in patients with COPD generated public attention, but the results of the later validation study yielded negative results (122,126).
Clearly, the CTCs circulating in cancer patients differ from the bulk of primary tumor cells and as such may represent a poor surrogate population assessing response to therapy (127). In 35% of colorectal cancer cases, metastases shared common traits with the original tumors, whereas 65% were derived from independent subclones (128). The SCLC CTCs proved to be chemosensitive in form of single cells but the tumors which have relapsed after primary treatment exhibit a general drug resistance (129,130). Therefore, successful reduction of the CTCs correlates not necessarily with response of the resident lesions. Since metastases are formed by less than 0.01% of the CTCs, single-cell analysis to identify the true metastasis-inducing subpopulation will be demanding. In addition, processes directing cell adhesion and protein-protein interaction for physical manners of resistance may be difficult to be identified at the level of the transcriptome. One solution may be the in vitro expansion of viable CTCs and their genetic and phenotypic characterization (104).
CTCs are also reported to be detectable in patients with early-stage cancers and may have prognostic significance (131-133). One or more CTCs are found in 20% of node-negative and 24% of stage 1–3 breast cancer patients (48,134,135). Baseline CTC counts were not correlated with tumor size for different cancer types and a proliferation index from 1 to 80% of CTCs has been described by Ki67 staining (136). CTCs numbers were reported to possess prognostic significance in several tumor types (18,19). Assessment of CTCs suggests a correlation of high CTC counts with shorter disease-free survival and a poorer prognosis as well as a response marker to chemotherapy upon a drop in CTC counts (19,24,69,119). Correlation of high CTC counts with a poor prognosis has been described for various types of cancers, including colorectal, breast, lung and pancreatic cancer (12,24). The presence of ≥3 CTCs per 7.5 mL of peripheral blood indicated a shorter of progression-free survival (PFS) (137). Hou et al. found that patients with SCLC and a CTC count of ≥50 CTCs/7.5 mL prior to chemotherapy had a poorer clinical outcome in terms of OS than those with a CTC count <50 CTC/7.5 mL (HR =2.5) (33). A European multicenter study in 550 patients with NSCLC reported ≥2 CTCs in 27% patients and 13% with ≥5 per 7.5 mL of blood using the CellSearch© system (85). These CTC counts were associated with reduced PFS (≥2 CTCs: HR =1.7, ≥5 CTCs: HR =2.2) and OS (≥2 CTCs: HR =2.2, ≥5 CTCs: HR =2.8), in good agreement with other studies (138,139).
CTCs counts may constitute a surrogate marker of the response to chemotherapy showing a rapid decline in CTC counts with up to a 50% reduction (140). In a meta-analysis comprising 2,239 breast cancer patients, the CTC count before neoadjuvant chemotherapy had a negative impact on patient survival, as patients with one to five or more CTCs hold a HR of death of 1.1–6.25, respectively (141). However, the role of CTC counts in diagnostic and treatment guidance remain to be defined (142). For instance, in metastatic breast cancer, clinical practice guidelines do not recommend currently the use of CTCs for the selection of treatments (143).
Angiogenesis and CTCs
Several mouse models of tumorigenesis revealed an angiogenic switch that is initiated during the early stages of tumor development, indicating that the regulation of angiogenesis is a potentially rate-limiting step in the development of many solid tumors (35). Tumor vessels formed in response to upregulation of VEGF are fenestrated and leaky as well as accompanied by a disorganized or loose basement membrane (144). These conditions typically lead to high interstitial pressures, escalated tissue hypoxia and further release of additional VEGF. The endothelial fenestrations formed are of dimensions ranging from 200 to 2,000 nm (145). The efficacy of the drug delivery into cancer cells is mostly dependent on angiogenesis. All these changes promote the enhanced permeability and retention effect (EPR) effect of the cancer cells (146).
Tumor neoangiogenesis forms immature blood vessels that facilitate the intravasation of CTCs (147). Shed tumor cells built cell aggregates in blood vessels due to intravascular proliferation (148). The ability for intravasation of mammary carcinoma cells showed no correlation with the size of the primary tumor (68). Generally, the microvessel count of tumors and density grade correlate with distant metastases. In breast cancer, a prognostic indicator for survival is the degree of angiogenesis (149,150). Breast cancer cells exhibiting increased levels of angiogenic growth factors show higher aggressiveness and an elevated risk of the invasive breast cancer (35). The microvessel density (MVD) of surgical samples of invasive breast cancer may be a predictor of metastasis or relapse. Assessment of the microvessel densities of breast carcinomas of 49 patients (30 with metastases and 19 without) demonstrated that for each increase of 10 microvessels a 1.17-fold increase in the risk of distant metastasis has been observed (150).
VEGF is the most important angiogenesis-provoking cytokines and is overexpressed in solid cancers. In metastatic breast cancer, patients with serum-VEGF levels (sVEGF) ≥367 pg/mL and ≥5 CTCs had the shortest overall survival (OS) compared to patients with lower sVEGF and non-elevated CTCs (151). VEGF-B promotes metastasis through the remodeling of tumor vessels to pseudo-normalized vasculatures of high leakiness and knockdown of this mediator resulted in increased perivascular cell coverage and reduced pulmonary metastasis of human melanomas (152). Despite retarded growth of primary tumors, VEGF-B markedly promotes metastasis. High levels of VEGF-B in patients with lung squamous cell carcinoma and melanoma correlated with poor survival (152).
Intravasation requires interactions between tumor cells, perivascular cells and possibly inflammatory cells (153,154). Microvessels are barriers for the invasion and tumor cells secrete mediators that restructure the vessels to become more permissive for intravasation through the reduction of perivascular cell coverage of the vessels. VEGF-B promotes cancer metastasis at the level of intravasation animals bearing VEGF-B-positive tumors have high CTCs counts (152). VEGF-B–induced cancer cell intravasation is accompanied by tissue hypoxia and attraction of inflammatory macrophages. Our group has shown that SCLC CTCs recruit macrophages (155). Similarly, angiopoietin-2 increases vascular permeability by damaging pericytes through the induction of superoxide. Analysis of intravasation in distinct areas of primary tumors demonstrated that the majority (>90%) of tumor cell shedding events were localized to the interior angiogenic core depending on damaged vessels (90).
SCLC and CTCs
SCLC is a neuroendocrine tumor that has a 2-year survival rate below 10% due to metastatic spread in most patients at first presentation (156). SCLC shows high sensitivity to first-line chemotherapy consisting of platinum drugs/etoposide regimens but relapses within approximately one year and responds poorly to further treatment. This tumor is distinguished by extreme numbers of CTCs with a mean count of 400 CTCs/7.5 mL blood and peak values of up to several thousand cells/7.5 mL blood (8). For SCLC at advanced metastatic state, a CTC count of 1,589±5,565 (mean ± SD) in 7.5 mL of blood was also published (157).
High blood perfusion of the lung, invasive growth of the tumors and inflammation characterized by the recruitment of macrophages seems to contribute to the shedding of CTCs (158). The SCLC CTCs are small (approximately 8 µm) compared to other tumors and, thus, can more easily migrate through capillaries and recirculate. The excessive numbers of CTCs in advanced SCLC patients are expected to include a number of actual metastasis-inducing cells and, therefore, we could establish a panel of 8 permanent SCLC CTC in vitro (110,159). The cancer stem cell hypothesis assumes that a subpopulation of rare stem cells survive the initial chemotherapy and are responsible for tumor recurrences after elimination of the bulk tumor tissue (160). However, SCLC CTCs lack stem cell markers, such as CD133, CD44, ABCG2 and the expression of vimentin causing a hybrid EMT phenotype indicates no real cell-type transformation (83,161). The in vitro expanded SCLC CTCs form spontaneously large multicellular aggregates, termed tumorospheres, that contain quiescent and hypoxic cells in the inner layers and are much more chemoresistant compared to the same cells in form of single cell suspensions (104). SCLC tumors are typically found in smokers with high tobacco consumption and the cancer cells exhibit numerous mutations (162). In this context, the controlled transformation to a mesenchymal phenotype and its reversal following extravasation is an unlikely mechanism (73). Our results with the SCLC CTCs cell lines have shown the production of angiogenic factors, like CHI3L1 and VEGF and proteolytic enzymes (MMPs, cathepsins) that enables them to disseminate without mesenchymal switch (163). In case of SCLC CTCs, small cell aggregates may become trapped in capillaries, especially of liver and brain, and extravasate by physical pressure force upon reaching larger sizes (164).
SCLC patients (37/38) have rare CTC subpopulations co-expressing vascular endothelial-cadherin (VE-cadherin) and cytokeratins consistent with vasculogenic mimicry (VM), a process whereby tumor cells form endothelial-like vessels (165). Higher levels of VM in limited stage SCLC patients were reported to be associated with a poorer overall survival possibly due to increased access of CTCs to the circulation. However, using VE-cadherin as a VM biomarker its expression in SCLC cytokeratin (CK)-positive CTCs was assessed using the ISET method that may enrich SCLC cells with less specificity.
NSCLC and CTCs
Among lung cancer patients, NSCLC blood samples enclose very low numbers of CTCs in contrast to SCLCs. In a study of Li et al. blood samples of NSCLC patients were obtained on the first day of treatment and during chemotherapy or targeted therapy cycles for CTCs detection. Of 100 patients, 48 were identified to be CTC-positive at baseline defined as one or more CTCs per 7.5 mL (166). A higher CTC-positive rate was found for stage IV NSCLC patients compared to stage III patients (69% vs. 40%). CTC clusters, defined as aggregates with ≥2 CTCs, were significantly correlated with disease control rate. Patients were divided into those with low (<4 CTCs, LL) and high CTC counts (≥4 CTCs, HL) yielding significant shorter OS and PFS in high count group. A number of CTC ≥50 were detected using ISET in pre-surgery blood samples from 102/208 (49%) patients undergoing curative tumor removal for NSCLC with significantly shorter DFS and OS (167). The large number of “CTCs” in this case was associated with the ISET technique that isolates more cells than the CellSearch© system but of unclear significance. In a study by Ichimura et al. patients undergoing planned surgery for lung cancer were recruited (168). Samples were collected at recruitment, before treatment and approximately 3 months later. CTC numbers counted at recruitment were 1.4±0.4, 1.8±1.2, 1.3±0.6 and 7.4±5.1 (mean ± SE) in clinical stages I, II, III and IV, respectively. At baseline, 51 (62.96%) patients were positive for high definition (HD)-CTCs with a median of 2.20 (mean 26.21±15.64) (169). The baseline CTC count exhibited no correlation with patient characteristics. In a review by Qian et al. cutoff values for prognosis of 10 studies were 5 CTCs for CellSearch© and 15–25 for other methods (170). Similarly, Kejik et al. cited limits of 5, 5, 1-2 and 8 CTCs for a total of more than 200 patients (171). The CTC count was approximately twice as high for NSCLC patients as for patients with benign lung diseases carrying nonmalignant “CTCs” (pneumonia, pulmonary tuberculosis, bronchiectasis, or pneumothorax) or healthy subjects (172). However, due to a number of highly sensitive techniques to detect CTCs, a few CTCs appearing sporadically in the circulation of NSCLC patients proved obviously sufficient to infer prognostic and predictive statements. However, despite of 15 years of research on CTCs in NSCLC this test did not enter into routine clinical guidance for the choice of therapeutic options.
Conclusions
Despite a wide range of techniques available to count and identify CTCs their clinical applications in diagnosis and guidance for therapy are still limited. In principle, the various methods to enrich and detect CTCs are tedious and time-consuming and in conjunction with the lack of a standardization of the counts and characteristics of these cells show limited potential in the clinical setting. Absence of CTCs even in metastatic patients, detection in late phases of the tumor development after dissemination has occurred and poor resemblance of the bulk tumors in assessment of response and prognosis put the costs, effort and manual input into question. Especially, the use of CTCs for tumor screening and early detection seems not possible due to few positive results of debatable significance. Furthermore, patients with zero CTC detected at a given time point may have been registered as false negative cases (173). Repeated blood draws to clarify the temporal distribution of CTCs in patients are not tolerable for the patients. However, in contrast to studies on circulating nucleic acids, exosomes, or other blood biomarkers, the analysis of CTCs can define their cellular genetics, epigenetics, transcriptomics, and protein profile. However, due to the host of non-validated methods and increased work load, the use of CTCs numbers as prognostic and predictive biomarkers lags behind the utilization of cell-free DNA (cf-DNA) in plasma in daily practice of oncologists (157,174).
Research on CTCs is hindered by the rarity and heterogeneity of these cancer cells and the low number of short-or long-term cultured specimen. Therefore, assignation of their biologic significance and their participation in all steps of dissemination is unclear and poorly characterized. CTCs appear early in tumor neoangiogenesis years before the primary tumor or metastases are detected clinically in patients by symptoms, biomarkers and imaging. In respect to the intravasation of cancer cells into the circulation, transformation to a mesenchymal phenotype (EMT) seems to be dispensable as tumor cells can easily pass into leaky newformed vessels in cores already at small tumor sizes. Accordingly, the inverse process of EMT, namely MET, for extravasation is not required (73). The assumption that CTCs are representative of the bulk tumors is questionable. For example, in metastatic SCLC the CTCs are chemosensitive but responses of the bulk tumor to chemotherapy are poor leading ultimately to a dismal prognosis (104). Of course, CTCs are easily accessible to high concentrations of chemotherapeutics in the circulation, in contrast to poorly vascularized tumors or spontaneously formed tumorospheres. Ultimately, the CTC count seems to be linked to the prognosis of tumor patients via the extent of neoangiogenesis.
Acknowledgments
We wish to thank Dr. T. Hohenheim and Dr. W. Occam (both retired and not linked to any institution) for enduring endorsement.
Funding: None.
Footnote
Reporting Checklist: The authors have completed the Narrative Review reporting checklist. Available at https://tlcr.amegroups.com/article/view/10.21037/tlcr-22-712/rc
Peer Review File: Available at https://tlcr.amegroups.com/article/view/10.21037/tlcr-22-712/prf
Conflicts of Interest: All authors have completed the ICMJE uniform disclosure form (available at https://tlcr.amegroups.com/article/view/10.21037/tlcr-22-712/coif). GH serves as an unpaid editorial board member of Translational Lung Cancer Research from September 2021 to August 2023. The other authors have no conflicts of interest to declare.
Ethical Statement: The authors are accountable for all aspects of the work in ensuring that questions related to the accuracy or integrity of any part of the work are appropriately investigated and resolved.
Open Access Statement: This is an Open Access article distributed in accordance with the Creative Commons Attribution-NonCommercial-NoDerivs 4.0 International License (CC BY-NC-ND 4.0), which permits the non-commercial replication and distribution of the article with the strict proviso that no changes or edits are made and the original work is properly cited (including links to both the formal publication through the relevant DOI and the license). See: https://creativecommons.org/licenses/by-nc-nd/4.0/.
References
- Eccles SA, Welch DR. Metastasis: recent discoveries and novel treatment strategies. Lancet 2007;369:1742-57. [Crossref] [PubMed]
- Wan L, Pantel K, Kang Y. Tumor metastasis: moving new biological insights into the clinic. Nat Med 2013;19:1450-64. [Crossref] [PubMed]
- Brabletz T, Lyden D, Steeg PS, et al. Roadblocks to translational advances on metastasis research. Nat Med 2013;19:1104-9. [Crossref] [PubMed]
- Talmadge JE, Fidler IJ. AACR centennial series: the biology of cancer metastasis: historical perspective. Cancer Res 2010;70:5649-69. [Crossref] [PubMed]
- Fidler IJ. Cancer biology is the foundation for therapy. Cancer Biol Ther 2005;4:1036-9. [Crossref] [PubMed]
- Zhao ZM, Zhao B, Bai Y, et al. Early and multiple origins of metastatic lineages within primary tumors. Proc Natl Acad Sci U S A 2016;113:2140-5. [Crossref] [PubMed]
- Caixeiro NJ, Kienzle N, Lim SH, et al. Circulating tumour cells--a bona fide cause of metastatic cancer. Cancer Metastasis Rev 2014;33:747-56. [Crossref] [PubMed]
- Hodgkinson CL, Morrow CJ, Li Y, et al. Tumorigenicity and genetic profiling of circulating tumor cells in small-cell lung cancer. Nat Med 2014;20:897-903. [Crossref] [PubMed]
- Mego M, Gao H, Cohen EN, et al. Circulating Tumor Cells (CTC) Are Associated with Defects in Adaptive Immunity in Patients with Inflammatory Breast Cancer. J Cancer 2016;7:1095-104. [Crossref] [PubMed]
- Chen L, Bode AM, Dong Z. Circulating Tumor Cells: Moving Biological Insights into Detection. Theranostics 2017;7:2606-19. [Crossref] [PubMed]
- Kaiser J. Medicine. Cancer's circulation problem. Science 2010;327:1072-4. [Crossref] [PubMed]
- Ju S, Chen C, Zhang J, et al. Detection of circulating tumor cells: opportunities and challenges. Biomark Res 2022;10:58. [Crossref] [PubMed]
- Miller MC, Robinson PS, Wagner C, et al. The Parsortix™ Cell Separation System-A versatile liquid biopsy platform. Cytometry A 2018;93:1234-9. [Crossref] [PubMed]
- Coumans FA, Siesling S, Terstappen LW. Detection of cancer before distant metastasis. BMC Cancer 2013;13:283. [Crossref] [PubMed]
- Butler TP, Gullino PM. Quantitation of cell shedding into efferent blood of mammary adenocarcinoma. Cancer Res 1975;35:512-6. [PubMed]
- Gires O, Pan M, Schinke H, et al. Expression and function of epithelial cell adhesion molecule EpCAM: where are we after 40 years? Cancer Metastasis Rev 2020;39:969-87. [Crossref] [PubMed]
- Rawal S, Yang YP, Cote R, et al. Identification and Quantitation of Circulating Tumor Cells. Annu Rev Anal Chem (Palo Alto Calif) 2017;10:321-43. [Crossref] [PubMed]
- Andree KC, van Dalum G, Terstappen LW. Challenges in circulating tumor cell detection by the CellSearch system. Mol Oncol 2016;10:395-407. [Crossref] [PubMed]
- Bardelli A, Pantel K. Liquid Biopsies, What We Do Not Know (Yet). Cancer Cell 2017;31:172-9. [Crossref] [PubMed]
- Liu S, Zhou F, Shen Y, et al. Fluid shear stress induces epithelial-mesenchymal transition (EMT) in Hep-2 cells. Oncotarget 2016;7:32876-92. [Crossref] [PubMed]
- Coumans FA, Ligthart ST, Uhr JW, et al. Challenges in the enumeration and phenotyping of CTC. Clin Cancer Res 2012;18:5711-8. [Crossref] [PubMed]
- Gabriel MT, Calleja LR, Chalopin A, et al. Circulating Tumor Cells: A Review of Non-EpCAM-Based Approaches for Cell Enrichment and Isolation. Clin Chem 2016;62:571-81. [Crossref] [PubMed]
- Sharma S, Zhuang R, Long M, et al. Circulating tumor cell isolation, culture, and downstream molecular analysis. Biotechnol Adv 2018;36:1063-78. [Crossref] [PubMed]
- Joosse SA, Gorges TM, Pantel K. Biology, detection, and clinical implications of circulating tumor cells. EMBO Mol Med 2015;7:1-11. [Crossref] [PubMed]
- Alix-Panabières C, Pantel K. Challenges in circulating tumour cell research. Nat Rev Cancer 2014;14:623-31. [Crossref] [PubMed]
- Xiao J, Pohlmann PR, Isaacs C, et al. Circulating Tumor Cells: Technologies and Their Clinical Potential in Cancer Metastasis. Biomedicines 2021;9:1111. [Crossref] [PubMed]
- Wu T, Cheng B, Fu L. Clinical Applications of Circulating Tumor Cells in Pharmacotherapy: Challenges and Perspectives. Mol Pharmacol 2017;92:232-9. [Crossref] [PubMed]
- Keller L, Pantel K. Unravelling tumour heterogeneity by single-cell profiling of circulating tumour cells. Nat Rev Cancer 2019;19:553-67. [Crossref] [PubMed]
- Heitzer E, Auer M, Ulz P, et al. Circulating tumor cells and DNA as liquid biopsies. Genome Med 2013;5:73. [Crossref] [PubMed]
- Chaffer CL, San Juan BP, Lim E, et al. EMT, cell plasticity and metastasis. Cancer Metastasis Rev 2016;35:645-54. [Crossref] [PubMed]
- Au SH, Storey BD, Moore JC, et al. Clusters of circulating tumor cells traverse capillary-sized vessels. Proc Natl Acad Sci U S A 2016;113:4947-52. [Crossref] [PubMed]
- Langley RR, Fidler IJ. The seed and soil hypothesis revisited--the role of tumor-stroma interactions in metastasis to different organs. Int J Cancer 2011;128:2527-35. [Crossref] [PubMed]
- Hou JM, Krebs MG, Lancashire L, et al. Clinical significance and molecular characteristics of circulating tumor cells and circulating tumor microemboli in patients with small-cell lung cancer. J Clin Oncol 2012;30:525-32. [Crossref] [PubMed]
- Donato C, Kunz L, Castro-Giner F, et al. Hypoxia Triggers the Intravasation of Clustered Circulating Tumor Cells. Cell Rep 2020;32:108105. [Crossref] [PubMed]
- Hanahan D, Folkman J. Patterns and emerging mechanisms of the angiogenic switch during tumorigenesis. Cell 1996;86:353-64. [Crossref] [PubMed]
- Aceto N, Bardia A, Miyamoto DT, et al. Circulating tumor cell clusters are oligoclonal precursors of breast cancer metastasis. Cell 2014;158:1110-22. [Crossref] [PubMed]
- Li X, Shi J, Gao Z, et al. Biophysical studies of cancer cells' traverse-vessel behaviors under different pressures revealed cells' motion state transition. Sci Rep 2022;12:7392. [Crossref] [PubMed]
- Reymond N, d'Água BB, Ridley AJ. Crossing the endothelial barrier during metastasis. Nat Rev Cancer 2013;13:858-70. [Crossref] [PubMed]
- Luzzi KJ, MacDonald IC, Schmidt EE, et al. Multistep nature of metastatic inefficiency: dormancy of solitary cells after successful extravasation and limited survival of early micrometastases. Am J Pathol 1998;153:865-73. [Crossref] [PubMed]
- Duda DG, Duyverman AM, Kohno M, et al. Malignant cells facilitate lung metastasis by bringing their own soil. Proc Natl Acad Sci U S A 2010;107:21677-82. [Crossref] [PubMed]
- Cho EH, Wendel M, Luttgen M, et al. Characterization of circulating tumor cell aggregates identified in patients with epithelial tumors. Phys Biol 2012;9:016001. [Crossref] [PubMed]
- McCarthy N. Platelets assist in extravasation. Nat Cell Biol 2013;15:1033. [Crossref]
- Driemel C, Kremling H, Schumacher S, et al. Context-dependent adaption of EpCAM expression in early systemic esophageal cancer. Oncogene 2014;33:4904-15. [Crossref] [PubMed]
- Song J, Yu Z, Dong B, et al. Clinical significance of circulating tumour cells and Ki-67 in renal cell carcinoma. World J Surg Oncol 2021;19:156. [Crossref] [PubMed]
- Micalizzi DS, Maheswaran S, Haber DA. A conduit to metastasis: circulating tumor cell biology. Genes Dev 2017;31:1827-40. [Crossref] [PubMed]
- Müller V, Stahmann N, Riethdorf S, et al. Circulating tumor cells in breast cancer: correlation to bone marrow micrometastases, heterogeneous response to systemic therapy and low proliferative activity. Clin Cancer Res 2005;11:3678-85. [Crossref] [PubMed]
- Xu L, Shamash J, Lu YJ. Circulating Tumor Cells: A Window to Understand Cancer Metastasis, Monitor and Fight Against Cancers. J Can Res Updates 2015;4:13-29.
- Rack B, Schindlbeck C, Jückstock J, et al. Circulating tumor cells predict survival in early average-to-high risk breast cancer patients. J Natl Cancer Inst 2014;106:dju066. [Crossref] [PubMed]
- Baccelli I, Schneeweiss A, Riethdorf S, et al. Identification of a population of blood circulating tumor cells from breast cancer patients that initiates metastasis in a xenograft assay. Nat Biotechnol 2013;31:539-44. [Crossref] [PubMed]
- Khoo BL, Grenci G, Jing T, et al. Liquid biopsy and therapeutic response: Circulating tumor cell cultures for evaluation of anticancer treatment. Sci Adv 2016;2:e1600274. [Crossref] [PubMed]
- Yu M, Bardia A, Aceto N, et al. Cancer therapy. Ex vivo culture of circulating breast tumor cells for individualized testing of drug susceptibility. Science 2014;345:216-20. [Crossref] [PubMed]
- Hamidi H, Ivaska J. Every step of the way: integrins in cancer progression and metastasis. Nat Rev Cancer 2018;18:533-48. [Crossref] [PubMed]
- Zavyalova MV, Denisov EV, Tashireva LA, et al. Intravasation as a Key Step in Cancer Metastasis. Biochemistry (Mosc) 2019;84:762-72. [Crossref] [PubMed]
- Dasgupta A, Lim AR, Ghajar CM. Circulating and disseminated tumor cells: harbingers or initiators of metastasis? Mol Oncol 2017;11:40-61. [Crossref] [PubMed]
- Leblanc R, Peyruchaud O. Metastasis: new functional implications of platelets and megakaryocytes. Blood 2016;128:24-31. [Crossref] [PubMed]
- Obermayr E, Agreiter C, Schuster E, et al. Molecular Characterization of Circulating Tumor Cells Enriched by A Microfluidic Platform in Patients with Small-Cell Lung Cancer. Cells 2019;8:880. [Crossref] [PubMed]
- Hamilton G, Rath B. Immunotherapy for small cell lung cancer: mechanisms of resistance. Expert Opin Biol Ther 2019;19:423-32. [Crossref] [PubMed]
- Nieto MA, Huang RY, Jackson RA, et al. EMT: 2016. Cell 2016;166:21-45. [Crossref] [PubMed]
- Tsuji T, Ibaragi S, Hu GF. Epithelial-mesenchymal transition and cell cooperativity in metastasis. Cancer Res 2009;69:7135-9. [Crossref] [PubMed]
- McDonald DM, Baluk P. Significance of blood vessel leakiness in cancer. Cancer Res 2002;62:5381-5. [PubMed]
- Kalluri R, Weinberg RA. The basics of epithelial-mesenchymal transition. J Clin Invest 2009;119:1420-8. [Crossref] [PubMed]
- Thiery JP, Lim CT. Tumor dissemination: an EMT affair. Cancer Cell 2013;23:272-3. [Crossref] [PubMed]
- Polyak K, Weinberg RA. Transitions between epithelial and mesenchymal states: acquisition of malignant and stem cell traits. Nat Rev Cancer 2009;9:265-73. [Crossref] [PubMed]
- Huang D, Du X. Crosstalk between tumor cells and microenvironment via Wnt pathway in colorectal cancer dissemination. World J Gastroenterol 2008;14:1823-7. [Crossref] [PubMed]
- Barriere G, Fici P, Gallerani G, et al. Circulating tumor cells and epithelial, mesenchymal and stemness markers: characterization of cell subpopulations. Ann Transl Med 2014;2:109. [PubMed]
- Yeung KT, Yang J. Epithelial-mesenchymal transition in tumor metastasis. Mol Oncol 2017;11:28-39. [Crossref] [PubMed]
- Suarez-Carmona M, Lesage J, Cataldo D, et al. EMT and inflammation: inseparable actors of cancer progression. Mol Oncol 2017;11:805-23. [Crossref] [PubMed]
- Wyckoff JB, Wang Y, Lin EY, et al. Direct visualization of macrophage-assisted tumor cell intravasation in mammary tumors. Cancer Res 2007;67:2649-56. [Crossref] [PubMed]
- Klein CA. Parallel progression of primary tumours and metastases. Nat Rev Cancer 2009;9:302-12. [Crossref] [PubMed]
- Cairns J. Mutation selection and the natural history of cancer. Nature 1975;255:197-200. [Crossref] [PubMed]
- Jolly MK, Ware KE, Gilja S, et al. EMT and MET: necessary or permissive for metastasis? Mol Oncol 2017;11:755-69. [Crossref] [PubMed]
- Valastyan S, Weinberg RA. Tumor metastasis: molecular insights and evolving paradigms. Cell 2011;147:275-92. [Crossref] [PubMed]
- Seyfried TN, Huysentruyt LC. On the origin of cancer metastasis. Crit Rev Oncog 2013;18:43-73. [Crossref] [PubMed]
- Hamilton G, Hochmair M, Rath B, et al. Small cell lung cancer: Circulating tumor cells of extended stage patients express a mesenchymal-epithelial transition phenotype. Cell Adh Migr 2016;10:360-7. [Crossref] [PubMed]
- Bacac M, Stamenkovic I. Metastatic cancer cell. Annu Rev Pathol 2008;3:221-47. [Crossref] [PubMed]
- Garber K. Epithelial-to-mesenchymal transition is important to metastasis, but questions remain. J Natl Cancer Inst 2008;100:232-3, 239. [Crossref] [PubMed]
- Larue L, Bellacosa A. Epithelial-mesenchymal transition in development and cancer: role of phosphatidylinositol 3' kinase/AKT pathways. Oncogene 2005;24:7443-54. [Crossref] [PubMed]
- de Wit S, van Dalum G, Lenferink AT, et al. The detection of EpCAM(+) and EpCAM(-) circulating tumor cells. Sci Rep 2015;5:12270. [Crossref] [PubMed]
- de Wit S, Manicone M, Rossi E, et al. EpCAM(high) and EpCAM(low) circulating tumor cells in metastatic prostate and breast cancer patients. Oncotarget 2018;9:35705-16. [Crossref] [PubMed]
- de Wit S, Rossi E, Weber S, et al. Single tube liquid biopsy for advanced non-small cell lung cancer. Int J Cancer 2019;144:3127-37. [Crossref] [PubMed]
- Deryugina EI, Kiosses WB. Intratumoral Cancer Cell Intravasation Can Occur Independent of Invasion into the Adjacent Stroma. Cell Rep 2017;19:601-16. [Crossref] [PubMed]
- Vardas V, Politaki E, Pantazaka E, et al. Epithelial-to-mesenchymal transition of tumor cells: cancer progression and metastasis. Int J Dev Biol 2022;66:277-83. [Crossref] [PubMed]
- Hamilton G, Moser D, Hochmair M. Metastasis: Circulating Tumor Cells in Small Cell Lung Cancer. Trends Cancer 2016;2:159-60. [Crossref] [PubMed]
- Turajlic S, Swanton C. Metastasis as an evolutionary process. Science 2016;352:169-75. [Crossref] [PubMed]
- Friberg S, Mattson S. On the growth rates of human malignant tumors: implications for medical decision making. J Surg Oncol 1997;65:284-97. [Crossref] [PubMed]
- Bockhorn M, Jain RK, Munn LL. Active versus passive mechanisms in metastasis: do cancer cells crawl into vessels, or are they pushed? Lancet Oncol 2007;8:444-8. [Crossref] [PubMed]
- Folkman J. Role of angiogenesis in tumor growth and metastasis. Semin Oncol 2002;29:15-8. [Crossref] [PubMed]
- Carmeliet P, Jain RK. Principles and mechanisms of vessel normalization for cancer and other angiogenic diseases. Nat Rev Drug Discov 2011;10:417-27. [Crossref] [PubMed]
- Strilic B, Yang L, Albarrán-Juárez J, et al. Tumour-cell-induced endothelial cell necroptosis via death receptor 6 promotes metastasis. Nature 2016;536:215-8. [Crossref] [PubMed]
- Deryugina EI, Quigley JP. Tumor angiogenesis: MMP-mediated induction of intravasation- and metastasis-sustaining neovasculature. Matrix Biol 2015;44-46:94-112. [Crossref] [PubMed]
- Bobek V, Kolostova K, Pinterova D, et al. A clinically relevant, syngeneic model of spontaneous, highly metastatic B16 mouse melanoma. Anticancer Res 2010;30:4799-803. [PubMed]
- Friedl P, Wolf K. Plasticity of cell migration: a multiscale tuning model. J Cell Biol 2010;188:11-9. [Crossref] [PubMed]
- Jain RK, Munn LL, Fukumura D. Measuring vascular permeability in mice. Cold Spring Harb Protoc 2013;2013:444-6. [Crossref] [PubMed]
- Collins VP, Loeffler RK, Tivey H. Observations on growth rates of human tumors. Am J Roentgenol Radium Ther Nucl Med 1956;76:988-1000. [PubMed]
- Spratt JS, Meyer JS, Spratt JA. Rates of growth of human neoplasms: Part II. J Surg Oncol 1996;61:68-83. [Crossref] [PubMed]
- Engel J, Eckel R, Kerr J, et al. The process of metastasisation for breast cancer. Eur J Cancer 2003;39:1794-806. [Crossref] [PubMed]
- Jones T, Neboori H, Wu H, et al. Are breast cancer subtypes prognostic for nodal involvement and associated with clinicopathologic features at presentation in early-stage breast cancer? Ann Surg Oncol 2013;20:2866-72. [Crossref] [PubMed]
- Popper HH. Progression and metastasis of lung cancer. Cancer Metastasis Rev 2016;35:75-91. [Crossref] [PubMed]
- van de Wouw AJ, Janssen-Heijnen ML, Coebergh JW, et al. Epidemiology of unknown primary tumours; incidence and population-based survival of 1285 patients in Southeast Netherlands, 1984-1992. Eur J Cancer 2002;38:409-13. [Crossref] [PubMed]
- Simpson KL, Stoney R, Frese KK, et al. A biobank of small cell lung cancer CDX models elucidates inter- and intratumoral phenotypic heterogeneity. Nat Cancer 2020;1:437-51. [Crossref] [PubMed]
- Kimbung S, Loman N, Hedenfalk I. Clinical and molecular complexity of breast cancer metastases. Semin Cancer Biol 2015;35:85-95. [Crossref] [PubMed]
- Morrow CJ, Trapani F, Metcalf RL, et al. Tumourigenic non-small-cell lung cancer mesenchymal circulating tumour cells: a clinical case study. Ann Oncol 2016;27:1155-60. [Crossref] [PubMed]
- Park D, Wang D, Chen G, et al. Establishment of Patient-derived Xenografts in Mice. Bio Protoc 2016;6:e2008. [Crossref] [PubMed]
- Klameth L, Rath B, Hochmaier M, et al. Small cell lung cancer: model of circulating tumor cell tumorospheres in chemoresistance. Sci Rep 2017;7:5337. [Crossref] [PubMed]
- Rath B, Plangger A, Krenbek D, et al. Rovalpituzumab tesirine resistance: analysis of a corresponding small cell lung cancer and circulating tumor cell line pair. Anticancer Drugs 2022;33:300-7. [Crossref] [PubMed]
- Hochmair M, Rath B, Klameth L, et al. Effects of salinomycin and niclosamide on small cell lung cancer and small cell lung cancer circulating tumor cell lines. Invest New Drugs 2020;38:946-55. [Crossref] [PubMed]
- Rath B, Klameth L, Plangger A, et al. Expression of Proteolytic Enzymes by Small Cell Lung Cancer Circulating Tumor Cell Lines. Cancers (Basel) 2019;11:114. [Crossref] [PubMed]
- Hamilton G. Comparative characteristics of small cell lung cancer and Ewing's sarcoma: a narrative review. Transl Lung Cancer Res 2022;11:1185-98. [Crossref] [PubMed]
- Shimada Y, Sudo T, Akamatsu S, et al. Cell Lines of Circulating Tumor Cells: What Is Known and What Needs to Be Resolved. J Pers Med 2022;12:666. [Crossref] [PubMed]
- Stickler S, Rath B, Hochmair M, et al. Changes of protein expression during tumorosphere formation of small cell lung cancer circulating tumor cells. Oncol Res 2023;31:13-22. [Crossref]
- Cheng X, Cheng K. Visualizing cancer extravasation: from mechanistic studies to drug development. Cancer Metastasis Rev 2021;40:71-88. [Crossref] [PubMed]
- Allen TA, Asad D, Amu E, et al. Circulating tumor cells exit circulation while maintaining multicellularity, augmenting metastatic potential. J Cell Sci 2019;132:jcs231563. [Crossref] [PubMed]
- Allen TA, Gracieux D, Talib M, et al. Angiopellosis as an Alternative Mechanism of Cell Extravasation. Stem Cells 2017;35:170-80. [Crossref] [PubMed]
- Follain G, Osmani N, Azevedo AS, et al. Hemodynamic Forces Tune the Arrest, Adhesion, and Extravasation of Circulating Tumor Cells. Dev Cell 2018;45:33-52.e12. [Crossref] [PubMed]
- Li G, Satyamoorthy K, Herlyn M. N-cadherin-mediated intercellular interactions promote survival and migration of melanoma cells. Cancer Res 2001;61:3819-25. [PubMed]
- Jain RK, Martin JD, Stylianopoulos T. The role of mechanical forces in tumor growth and therapy. Annu Rev Biomed Eng 2014;16:321-46. [Crossref] [PubMed]
- Paku S, Laszlo V, Dezso K, et al. The evidence for and against different modes of tumour cell extravasation in the lung: diapedesis, capillary destruction, necroptosis, and endothelialization. J Pathol 2017;241:441-7. [Crossref] [PubMed]
- Al-Mehdi AB, Tozawa K, Fisher AB, et al. Intravascular origin of metastasis from the proliferation of endothelium-attached tumor cells: a new model for metastasis. Nat Med 2000;6:100-2. [Crossref] [PubMed]
- Rolfo C, Castiglia M, Hong D, et al. Liquid biopsies in lung cancer: the new ambrosia of researchers. Biochim Biophys Acta 2014;1846:539-46. [PubMed]
- Allard WJ, Matera J, Miller MC, et al. Tumor cells circulate in the peripheral blood of all major carcinomas but not in healthy subjects or patients with nonmalignant diseases. Clin Cancer Res 2004;10:6897-904. [Crossref] [PubMed]
- Janni WJ, Rack B, Terstappen LW, et al. Pooled Analysis of the Prognostic Relevance of Circulating Tumor Cells in Primary Breast Cancer. Clin Cancer Res 2016;22:2583-93. [Crossref] [PubMed]
- Ilie M, Hofman V, Long-Mira E, et al. "Sentinel" circulating tumor cells allow early diagnosis of lung cancer in patients with chronic obstructive pulmonary disease. PLoS One 2014;9:e111597. [Crossref] [PubMed]
- Cilli A, Ozkaynak C, Onur R, et al. Lung cancer detection with low-dose spiral computed tomography in chronic obstructive pulmonary disease patients. Acta Radiol 2007;48:405-11. [Crossref] [PubMed]
- Vona G, Sabile A, Louha M, et al. Isolation by size of epithelial tumor cells: a new method for the immunomorphological and molecular characterization of circulatingtumor cells. Am J Pathol 2000;156:57-63. [Crossref] [PubMed]
- Fiorelli A, Accardo M, Carelli E, et al. Circulating Tumor Cells in Diagnosing Lung Cancer: Clinical and Morphologic Analysis. Ann Thorac Surg 2015;99:1899-905. [Crossref] [PubMed]
- Marquette CH, Boutros J, Benzaquen J, et al. Circulating tumour cells as a potential biomarker for lung cancer screening: a prospective cohort study. Lancet Respir Med 2020;8:709-16. [Crossref] [PubMed]
- Stoecklein NH, Klein CA. Genetic disparity between primary tumours, disseminated tumour cells, and manifest metastasis. Int J Cancer 2010;126:589-98. [Crossref] [PubMed]
- Naxerova K, Reiter JG, Brachtel E, et al. Origins of lymphatic and distant metastases in human colorectal cancer. Science 2017;357:55-60. [Crossref] [PubMed]
- Meikle CK, Kelly CA, Garg P, et al. Cancer and Thrombosis: The Platelet Perspective. Front Cell Dev Biol 2017;4:147. [PubMed]
- Hamilton G, Rath B, Holzer S, et al. Second-line therapy for small cell lung cancer: exploring the potential role of circulating tumor cells. Transl Lung Cancer Res 2016;5:71-7. [PubMed]
- Rhim AD, Mirek ET, Aiello NM, et al. EMT and dissemination precede pancreatic tumor formation. Cell 2012;148:349-61. [Crossref] [PubMed]
- Hüsemann Y, Klein CA. The analysis of metastasis in transgenic mouse models. Transgenic Res 2009;18:1-5. [Crossref] [PubMed]
- Eyles J, Puaux AL, Wang X, et al. Tumor cells disseminate early, but immunosurveillance limits metastatic outgrowth, in a mouse model of melanoma. J Clin Invest 2010;120:2030-9. [Crossref] [PubMed]
- Nagrath S, Sequist LV, Maheswaran S, et al. Isolation of rare circulating tumour cells in cancer patients by microchip technology. Nature 2007;450:1235-9. [Crossref] [PubMed]
- Lucci A, Hall CS, Lodhi AK, et al. Circulating tumour cells in non-metastatic breast cancer: a prospective study. Lancet Oncol 2012;13:688-95. [Crossref] [PubMed]
- Stott SL, Lee RJ, Nagrath S, et al. Isolation and characterization of circulating tumor cells from patients with localized and metastatic prostate cancer. Sci Transl Med 2010;2:25ra23. [Crossref] [PubMed]
- Rossi G, Ignatiadis M. Promises and Pitfalls of Using Liquid Biopsy for Precision Medicine. Cancer Res 2019;79:2798-804. [Crossref] [PubMed]
- Krebs MG, Sloane R, Priest L, et al. Evaluation and prognostic significance of circulating tumor cells in patients with non-small-cell lung cancer. J Clin Oncol 2011;29:1556-63. [Crossref] [PubMed]
- Punnoose EA, Atwal S, Liu W, et al. Evaluation of circulating tumor cells and circulating tumor DNA in non-small cell lung cancer: association with clinical endpoints in a phase II clinical trial of pertuzumab and erlotinib. Clin Cancer Res 2012;18:2391-401. [Crossref] [PubMed]
- Deutsch TM, Stefanovic S, Feisst M, et al. Cut-Off Analysis of CTC Change under Systemic Therapy for Defining Early Therapy Response in Metastatic Breast Cancer. Cancers (Basel) 2020;12:1055. [Crossref] [PubMed]
- Bidard FC, Michiels S, Riethdorf S, et al. Circulating Tumor Cells in Breast Cancer Patients Treated by Neoadjuvant Chemotherapy: A Meta-analysis. J Natl Cancer Inst 2018;110:560-7. [Crossref] [PubMed]
- Vasseur A, Kiavue N, Bidard FC, et al. Clinical utility of circulating tumor cells: an update. Mol Oncol 2021;15:1647-66. [Crossref] [PubMed]
- Van Poznak C, Harris LN, Somerfield MR. Use of Biomarkers to Guide Decisions on Systemic Therapy for Women With Metastatic Breast Cancer: American Society of Clinical Oncology Clinical Practice Guideline. J Oncol Pract 2015;11:514-6. [Crossref] [PubMed]
- Li H, Fan X, Houghton J. Tumor microenvironment: the role of the tumor stroma in cancer. J Cell Biochem 2007;101:805-15. [Crossref] [PubMed]
- Carmeliet P. VEGF as a key mediator of angiogenesis in cancer. Oncology 2005;69:4-10. [Crossref] [PubMed]
- Byrne JD, Betancourt T, Brannon-Peppas L. Active targeting schemes for nanoparticle systems in cancer therapeutics. Adv Drug Deliv Rev 2008;60:1615-26. [Crossref] [PubMed]
- Chung AS, Lee J, Ferrara N. Targeting the tumour vasculature: insights from physiological angiogenesis. Nat Rev Cancer 2010;10:505-14. [Crossref] [PubMed]
- Wong CW, Song C, Grimes MM, et al. Intravascular location of breast cancer cells after spontaneous metastasis to the lung. Am J Pathol 2002;161:749-53. [Crossref] [PubMed]
- Vartanian RK, Weidner N. Correlation of intratumoral endothelial cell proliferation with microvessel density (tumor angiogenesis) and tumor cell proliferation in breast carcinoma. Am J Pathol 1994;144:1188-94. [PubMed]
- Weidner N, Semple JP, Welch WR, et al. Tumor angiogenesis and metastasis--correlation in invasive breast carcinoma. N Engl J Med 1991;324:1-8. [Crossref] [PubMed]
- Banys-Paluchowski M, Witzel I, Riethdorf S, et al. The clinical relevance of serum vascular endothelial growth factor (VEGF) in correlation to circulating tumor cells and other serum biomarkers in patients with metastatic breast cancer. Breast Cancer Res Treat 2018;172:93-104. [Crossref] [PubMed]
- Yang X, Zhang Y, Hosaka K, et al. VEGF-B promotes cancer metastasis through a VEGF-A-independent mechanism and serves as a marker of poor prognosis for cancer patients. Proc Natl Acad Sci U S A 2015;112:E2900-9. [Crossref] [PubMed]
- Joyce JA, Pollard JW. Microenvironmental regulation of metastasis. Nat Rev Cancer 2009;9:239-52. [Crossref] [PubMed]
- Pollard JW. Tumour-educated macrophages promote tumour progression and metastasis. Nat Rev Cancer 2004;4:71-8. [Crossref] [PubMed]
- Hamilton G, Rath B, Klameth L, et al. Small cell lung cancer: Recruitment of macrophages by circulating tumor cells. Oncoimmunology 2015;5:e1093277. [Crossref] [PubMed]
- Semenova EA, Nagel R, Berns A. Origins, genetic landscape, and emerging therapies of small cell lung cancer. Genes Dev 2015;29:1447-62. [Crossref] [PubMed]
- Hofman V, Heeke S, Marquette CH, et al. Circulating Tumor Cell Detection in Lung Cancer: But to What End? Cancers (Basel) 2019;11:262. [Crossref] [PubMed]
- Hamilton G, Rath B. Smoking, inflammation and small cell lung cancer: recent developments. Wien Med Wochenschr 2015;165:379-86. [Crossref] [PubMed]
- Savarese-Brenner B, Heugl M, Rath B, et al. MUC1 and CD147 Are Promising Markers for the Detection of Circulating Tumor Cells in Small Cell Lung Cancer. Anticancer Res 2022;42:429-39. [Crossref] [PubMed]
- Fiori ME, Villanova L, De Maria R. Cancer stem cells: at the forefront of personalized medicine and immunotherapy. Curr Opin Pharmacol 2017;35:1-11. [Crossref] [PubMed]
- Hamilton G, Rath B. Mesenchymal-Epithelial Transition and Circulating Tumor Cells in Small Cell Lung Cancer. Adv Exp Med Biol 2017;994:229-45. [Crossref] [PubMed]
- Pleasance ED, Stephens PJ, O'Meara S, et al. A small-cell lung cancer genome with complex signatures of tobacco exposure. Nature 2010;463:184-90. [Crossref] [PubMed]
- Hamilton G, Rath B. Circulating tumor cell interactions with macrophages: implications for biology and treatment. Transl Lung Cancer Res 2017;6:418-30. [Crossref] [PubMed]
- Minder P, Zajac E, Quigley JP, et al. EGFR regulates the development and microarchitecture of intratumoral angiogenic vasculature capable of sustaining cancer cell intravasation. Neoplasia 2015;17:634-49. [Crossref] [PubMed]
- Williamson SC, Metcalf RL, Trapani F, et al. Vasculogenic mimicry in small cell lung cancer. Nat Commun 2016;7:13322. [Crossref] [PubMed]
- Li J. Significance of Circulating Tumor Cells in Nonsmall-Cell Lung Cancer Patients: Prognosis, Chemotherapy Efficacy, and Survival. J Healthc Eng 2021;2021:2680526. [Crossref] [PubMed]
- Gallo M, De Luca A, Maiello MR, et al. Clinical utility of circulating tumor cells in patients with non-small-cell lung cancer. Transl Lung Cancer Res 2017;6:486-98. [Crossref] [PubMed]
- Ichimura H, Nawa T, Yamamoto Y, et al. Detection of circulating tumor cells in patients with lung cancer using metallic micro-cavity array filter: A pilot study. Mol Clin Oncol 2020;12:278-83. [Crossref] [PubMed]
- Shishido SN, Carlsson A, Nieva J, et al. Circulating tumor cells as a response monitor in stage IV non-small cell lung cancer. J Transl Med 2019;17:294. [Crossref] [PubMed]
- Qian H, Zhang Y, Xu J, et al. Progress and application of circulating tumor cells in non-small cell lung cancer. Mol Ther Oncolytics 2021;22:72-84. [Crossref] [PubMed]
- Kejík Z, Kaplánek R, Dytrych P, et al. Circulating Tumour Cells (CTCs) in NSCLC: From Prognosis to Therapy Design. Pharmaceutics 2021;13:1879. [Crossref] [PubMed]
- Chen X, Zhou F, Li X, et al. Folate Receptor-Positive Circulating Tumor Cell Detected by LT-PCR-Based Method as a Diagnostic Biomarker for Non-Small-Cell Lung Cancer. J Thorac Oncol 2015;10:1163-71. [Crossref] [PubMed]
- Aceto N. Fluctuating numbers of circulating tumor cells in cancer patients and the meaning of zero counts. Oncotarget 2019;10:2658-9. [Crossref] [PubMed]
- Fici P. Cell-Free DNA in the Liquid Biopsy Context: Role and Differences Between ctDNA and CTC Marker in Cancer Management. Methods Mol Biol 2019;1909:47-73. [Crossref] [PubMed]