False positivity in break apart fluorescence in-situ hybridization due to polyploidy
Highlight box
Key findings
• In liver cell nuclei the number of FISH/CISH signals increases with nuclear size due to physiological polyploidy and is related to section thickness.
• Tumour cells with higher nuclear size and higher ploidy levels have an increased chance of single signals.
What is known and what is new?
• In FISH even samples negative for translocations show a certain number of cells with single signals due to stereological constraints. Therefore, a cut-off of ≥15% of the nuclei with an aberrant pattern is used as threshold for positivity.
• Tumours with high ploidy levels harbour an increased risk of false positive FISH results.
What is the implication, and what should change now?
• False FISH results can have important implications for diagnosis, classification and prediction of cancer therapy and are potentially harmful for the patient.
• In polyploidy, the currently proposed cut-off should only be used with caution and the result should be confirmed by an additional technique.
Introduction
In-situ hybridization (ISH) is a method to detect DNA or RNA by binding complementary probes to the region(s) of interest. For visualization, labelling of the complementary probes is performed with either fluorescent probes (fluorescence in-situ hybridization, FISH) or non-fluorescent probes with additional immunohistochemical stains (chromogenic in-situ hybridization, CISH). Over the years, FISH and CISH became important diagnostic tools in the detection of chromosomal anomalies such as abnormal chromosome copy numbers (aneusomy), chromosome gains and losses, amplifications and translocations. Detection of those chromosomal anomalies has important implications for diagnosis and therapy in various diseases, especially in the classification of tumours and in prediction of cancer therapy [reviewed in (1)]. In non-small cell lung cancer (NSCLC) prominent examples of therapeutically relevant rearrangements were initially ALK and ROS1 followed by RET and NTRK1-3 detected by FISH and treated with tyrosine kinase inhibitors (TKIs) such as crizotinib, alectinib and larotrectinib (2-9).
Along with the use of ISH analyses for prognosis and treatment of individual patients with targeted therapy, technical as well as interpretational difficulties have become more obvious and challenging, even for experienced specialists. Next to general pre-analytical errors such as fixation problems and inadequate tissue quality, there are method specific analytical and postanalytical/interpretational errors that can lead to false negative or false positive results.
In dual-colour break-apart FISH of histologic sections the relative number of tumour cell nuclei with split signals and/or single signals is evaluated. In case of ALK, a split/break apart is defined as two signals having a distance greater than two signal diameters (10). However, it has been shown that in case of ALK FISH in adenocarcinoma of the lung the split length varies and may lead to artefacts and especially short splits can be difficult to distinguish from background noise (11). Furthermore, even in samples negative for translocations or other anomalies, not all nuclear cross sections show two fused signals due to stereological constraints. This has led to a cut-off of ≥15% of the nuclei with an aberrant pattern as threshold for positivity for genomic rearrangements (12).
During routine practice there are occasional cases that, in retrospect, show false positivity for FISH related to polyploidy (increase in copy number of the entire chromosome) and aneuploidy. The aim of this study is to gain more insight into the challenges in interpretation of ISH results in the presence of polyploid/aneuploid cells. We first created a theoretical model concerning the actual nuclear amount of a cell evaluated by ISH analysis. Secondly, we investigated the relationship between nuclear size, ploidy and section thickness in normal human hepatocytes that are often physiologically polyploid. Finally, we evaluated the relationship between nuclear size, ploidy and occurrence of single signals in FISH analyses in NSCLC.
Here we show that in healthy cells and in tumour cells, nuclear size and ploidy are positively correlated and that with increasing nuclear size and ploidy levels single signals occur significantly more often. Therefore, we state that in FISH analyses of tumours with a large amount of polyploid/aneuploid giant cells, a cut-off of 15% is insufficient and can be misleading. Cases with polyploidy/aneuploidy should be critically re-evaluated and orthogonal methods should be used. We present the following article in accordance with the MDAR reporting checklist (available at https://tlcr.amegroups.com/article/view/10.21037/tlcr-22-516/rc).
Methods
Tissue samples
A patient with NSCLC was initially diagnosed with rearrangements in both the ALK and the ROS1 gene detected by FISH analysis. Later in time the patient was referred to the Amsterdam University Medical Center, Location VUmc Amsterdam, for second opinion. The original diagnostic samples were retrieved for revision and further analysed. The study was conducted in accordance with the Declaration of Helsinki (as revised in 2013). The study was approved by the privacy functionary of Amsterdam University Medical Center, and the Medical Ethics Review Committee of VU University Medical Center confirmed that this study did not fall within their scope of responsibility (No. IRB00002991). Individual consent for this retrospective analysis was waived. At the time of publication, the patient was deceased and patient data were anonymised. Furthermore, a cohort of NSCLC cases showing polyploidy from Universitätsspital Basel and another NSCLC cohort examined by CellCarta (Antwerp, Belgium) were included in the study. The laboratory works in concordance with the ISO 15189 norm and diagnostic data were anonymised. As a control, liver tissue without disease was selected. All samples were neutral buffered formalin-fixed and paraffin-embedded (FFPE).
Immunohistochemistry
Immunohistochemistry was performed on 3 µm thick tissue sections. For P80 ALK immunohistochemistry clone 5A4 (Monosan, Uden, The Netherlands, catalogue number MONX10639) was used and diluted 1/50 in Dako Diluent. Antigen retrieval was performed using a PT module high pH buffer for 20 minutes at 97 ℃, and staining took place in the automated staining system Autostainer link 48 (Agilent/Dako, Santa Clara, USA). For ROS1 immunohistochemistry clone D4D6 (Cell Signaling Technology Inc, Beverly, USA, catalogue number 3287) was used and diluted 1/50 in Ventana diluent. Staining took place in the fully automated staining system BenchMark ULTRA (Roche/Ventana, Almere, The Netherlands). After dehydration with ethanol 100% and xylene the slides were covered with an automated Slide Coverslipper (Sakura Finetek Europe, Alphen aan de Rijn, The Netherlands).
At CellCarta, clone D5F3 (Ventana Medical Systems Inc., Tucson, USA, catalogue number 790-4796) was used for ALK immunohistochemistry. The assays were performed according to the manufacturer’s instructions (CE-IVD, Roche Diagnostics, Vilvoorde, Belgium) in combination with the OptiView DAB IHC Detection kit and OptiView Amplification Kit as a fully automated immunohistochemistry assay on the Ventana BenchMark XT automated slide staining device.
FISH/CISH
FISH was performed using the commercial kits Vysis 2p23 ALK Break Apart FISH Probe Kit (Abbott, Hoofddorp, The Netherlands), 6q22 sureFISH ROS1 Break Apart Probe (Agilent, Amstelveen, The Netherlands) and Histology FISH Accessory Kit (Agilent, Amstelveen, The Netherlands) respectively.
From NSCLC usually 4 µm thick sections were cut. From the liver control, 2 and 5 µm thick sections were used. All sections were mounted on coated Tomo adhesion microscope slides (Matsunami Glass, Osaka, Japan) and dried for a minimum of 60 min at 37 ℃ and 60 min at 60 ℃. Paraffin sections were deparaffinized with xylene (3×5 min), rehydrated in ethanol 100% (4×5 min) and washed in demiwater. After washing in Dako wash buffer, slides were pre-treated with 2-(N-morpholino) ethanesulfonic acid (MES) buffer at 100 ℃ for 10 min, washed in Dako wash buffer and treated with pepsin (Dako) at 37 ℃ (ALK: 3 min for biopsies and 5 min for resections; ROS1: 5 min for biopsies and for 10 min for resections). After washing, sections were dehydrated with ethanol 100% for 1 min and dried at room temperature (RT). Following denaturation (5 min at 82 ℃), hybridization was performed overnight (minimum of 20 h at 40 ℃). Post-hybridization washes were performed in 0.1 × SSC/0.1% NP-40, 2×5 min at RT, 2×5 min at 50 ℃ and 1×5 min at RT. Subsequently, sections were dehydrated with ethanol 100% and dried at RT. Counterstaining and mounting was performed manually with 4',6-diamidino-2-phenylindole (DAPI; 0.8 µg/mL) in Vectashield medium. Stained sections were stored at −20 ℃.
MET/CH7 CISH was performed on 2 and 4 µm thick sections using the fully automated staining system BenchMark ULTRA (Roche/Ventana, Almere, The Netherlands). After washing in EZ prep solution (Roche Diagnostics NL, Almere, The Netherlands) for 5 min, a solution of dishwashing detergent for 5 min and rinsing in tap water for 5 min, sections were dried at 37 ℃ for 30 min. Following, sections were covered with an automated Slide Coverslipper (Sakura Finetek Europe).
For the CellCarta cohort, the Vysis ALK Break Apart FISH Probe Kit was used (Abbot Molecular, Wavre, Belgium) according to the manufacturer’s instructions, with slight, extensively validated modifications.
Quantitative analysis
In VUmc for diagnostic purposes a pathologist evaluates tumour content with the scorers before evaluating the FISH results. Signal counting is conducted primarily under the fluorescence microscope to avoid artifacts introduced by image capture and the single 3' and/or split signals are considered positive for break apart. Two readers, blinded to the others results, separately score each case. In this study readers were also blinded for the results of ancillary testing.
Representative pictures of MET CISH labelled liver sections were taken with a Leica DC500 (Leica Microsystems, Heidelberg, Germany) using a 400× magnification and pictures of ALK and ROS1 FISH of the different tissue sections were taken with a Leica DM5500B using a 1,000× magnification. Nuclear diameter was measured and the number of signals per nucleus was manually counted. For CISH analyses the number of red signals (CH7) was counted. For FISH analyses the number of double signals (fusion signals) and single signals (also called isolated orange /green signals) was counted.
The FISH slides of the CellCarta cohort were scanned with the BioView system.
For the CellCarta cohort, the tumour region was indicated and tumour content estimated by a pathologist on an HE stained slide. These data were consulted as guidance for FISH scanning and analysis. The FISH slides were scanned and analysed with the BioView software (version 3.7.0.8, BioView, Israel), according to the instructions of the Vysis ALK Break Apart FISH Probe Kit insert.
RNA analysis
The patient slides referred to VUmc were sent to Dublin (SF) for examination with the Ion Torrent Oncomine assay (Thermo Fisher Scientific, Waltham, USA).
For the CellCarta cohort RNA analysis was performed with the Archer® FusionPlex® Comprehensive Thyroid and Lung (CTL) Kit (ArcherDX, Boulder, USA). Total RNA was extracted from FFPE material using the High Pure FFPET RNA Isolation Kit (Roche Diagnostics). The Fastq files were analysed with both the ArcherDX Analysis software and the Sophie Genetics’ Archer CTL analysis pipeline.
Copy number profiling
Copy number aberration profiling was performed with shallow sequencing and DNA isolated from FFPE samples at the Department of Pathology of the Amsterdam UMC, Location VUmc, as described previously (13).
Statistical analysis
Spearman’s rank correlation (ρ) was computed to investigate the correlation between the number of signals of interest with nuclear size. Differences between two groups were assessed with the Mann-Whitney U-test and differences between more than two groups with the Kruskal-Wallis test for non-normally distributed data. Dichotomous data was compared between groups with the chi-square test. Post-hoc comparisons were corrected for multiple testing with the Bonferroni correction. P values <0.05 were considered significant. Statistical analyses were done in SPSS version 26 (IBM Corp., Armonk, NY, USA).
Results
Index case with false positive ALK and ROS1 FISH
A patient diagnosed with metastasized NSCLC presumed rearrangements in both the ALK and the ROS1 gene detected by FISH analysis in predictive testing, with 37% positive cells and 30% positive cells respectively. After chemotherapy, the patient was treated with an ALK inhibitor and follow-up revealed progressive disease. After referral, revision of the pathology examination showed the morphology of a giant cell carcinoma, a variant of pleomorphic carcinoma of the lung. FISH analysis was repeated on the same block. The average number of co-localization signals in the large tumour cell nuclei for ALK and ROS1 was 7.6 and 9.5, respectively (range, 1–30). The tumour cells were negative in repeated testing for ALK and ROS1 by immunohistochemistry. Examination with an orthogonal method (Ion Torrent Oncomine Assay) did not demonstrate translocations. Thus, repeated and additional orthogonal testing proved a false positive test for ALK and ROS1 FISH, possibly caused by high aneuploidy.
In liver cells the number of signals in CISH and FISH increases with nuclear size and is dependent on section thickness
Nuclear size was measured and the number of red signals (CH7) in MET CISH and double signals (fusion signals) in ALK FISH was manually counted in control liver sections of varying section thickness (2 µm and 4 µm/5 µm). The range of nuclear size was similar in MET CISH and ALK FISH in as well 2 µm (MET n=189; 4.5–17.3 µm, ALK n=90; 4.5–15.5 µm) as in 4 µm/5 µm (MET n=236; 4.5–15.8 µm, ALK n=75; 5.5–18.5 µm) thick sections. The maximum number of signals of interest (red signals in MET CISH and double signals in ALK FISH) in 2 µm and 4 µm/5 µm was 7 and 9 for MET CISH and 8 and 7 for ALK FISH respectively. In MET CISH as well as in ALK FISH the number of the signals of interest positively correlated (ρ≥0.42, all P<0.001) with nuclear size (Figure 1A-1H, Figure 2A). Categorizing nuclear size into three groups: <8 µm, 8–11 µm and >11 µm, revealed significantly more signals of interest in larger nuclei (all P<0.001; Figure 2A). Whereas the average number of signals of interest in nuclei <8 µm was ≤1.4 and the median 1, regardless of method or section thickness, the average number of signals of interest in nuclei >11 µm was ≥3.1 and the median 3 or more.
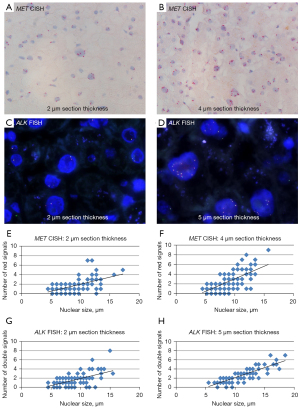
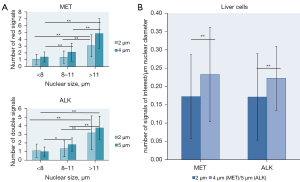
In MET CISH the average number of red MET gene signals per nucleus in slices with a section thickness of 2 µm was 1.5 (median 1) with an average nuclear size of 8.3 µm (median 7.5 µm). This corresponds with 0.17 red signals per µm nuclear diameter (median 0.17), which is lower than in sections with a thickness of 4 µm, where the average number of red signals per µm nuclear diameter was 0.23 (median 0.24), the average number of red signals per nucleus 1.9 (median 2) and the average nuclear size 7.8 µm (median 7.5 µm); (P<0.001). Quantification of ALK FISH revealed a similar trend (P<0.001): the average number of double signals per µm nuclear diameter for 2 and 5 µm thick sections was 0.17 and 0.22, respectively (median 0.15 and 0.23) (Figure 2B).
Overall, irrespective of the ISH method used, the number of signals increases with nuclear size related to physiological polyploidy and is also related to section thickness.
In tumour cell nuclei the chance of single signals increases with higher ploidy levels and larger nuclear size
To further explore the relation between nuclear size and number of double signals (fusion signals), a pulmonary carcinoid tumour with prominent variation in nuclear size was examined with ALK FISH and ROS1 FISH (Figure 3A,3B). The range of nuclear size varied in ALK FISH from 6 to 30.5 µm (n=172, median 13 µm) and in ROS1 FISH from 4 to 36.5 µm (n=159, median 10.5 µm). As indicated in Figure 3C,3D, the positive correlation (ρ≥0.73, all P<0.001) between nuclear size and ploidy in liver cells was confirmed in the carcinoid cell nuclei. The median number of double signals per nucleus was 2 for ALK (range, 0–20) and 3 for ROS1 (range, 0–53). Assuming a random distribution of the signals in a sphere nucleus and a section thickness of 5 µm, the calculated number of signals for the whole nucleus was 4.8 for ALK and 8.9 for ROS1. These numbers are close to the polyploid scale of 2, 4, 8, 16 etc., due to repeated genome duplication. In order to check for the gene copy numbers by FISH, an alternative method was used. With shallow sequencing a ratio for ROS1: ALK of 1.8 was obtained, confirming the ratio (8.9/4.8=1.85) obtained by FISH.
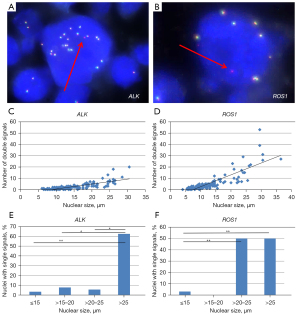
The relation between the number of single 5' and 3' signals and nuclear size is shown in Figure 3E,3F. Categorizing nuclear size into four groups revealed that the fraction of carcinoid nuclei with single signals increased drastically in tumour cells with large nuclear diameter (in ALK nuclear diameter >25 µm, in ROS1 nuclear diameter >20 µm). Note that in nuclei with a size >25 µm the fraction of nuclei with single signals was ≥50%.
To establish the relation between single and double signals in cases with polyploidy or high aneuploidy, 9 additional NSCLC cases were counted from digital ALK FISH images, see Figure 4A-4D. The number of double and single signals from 219 nuclei revealed 1–30 double signals and up to 4 single signals. The relation between the number of double signals and the fraction of nuclei with single signals is shown in Figure 4E,4F. The percentage of nuclei with single signals was 7.5% and 12.6% in the groups of <5 and 5–<10 double signals, compared to 53.3% and 50% in the groups of 10–<15 and ≥15 double signals respectively.
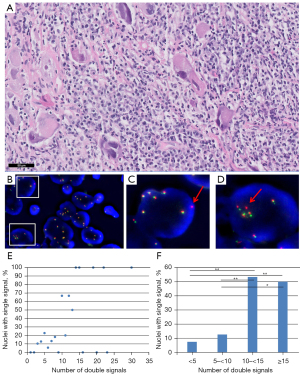
Thus, larger tumour cell nuclei have a higher rate of polyploidy/high aneuploidy and a higher fraction of single signals. As isolated 3' signals are one of the criteria for FISH positivity, nuclei with polyploidy/high aneuploidy may have higher chance to find more than 15% of the nuclei with single signals, leading to false positive FISH outcomes.
Borderline ALK FISH in lung cancer
Out of a total of 148 NSCLC samples with known IHC and FISH results, ten cases showed borderline ALK FISH results (FISH positivity of 15–20%). For nine out of these ten cases, discrepant results for ALK IHC and FISH testing were observed. Three of the cases with discrepant results for ALK IHC and FISH testing (positive for FISH and negative for IHC) were subsequently examined with the Archer® FusionPlex® CTL Kit. An overview of the 3 cases with orthogonal results is listed in Table 1, representative HE, ALK IHC and ALK FISH images are shown in Figure 5A-5G. The average and maximum numbers of orange and green signals indicated the presence of multiple copies of target gene ALK in all three cases. Rearrangement could not be demonstrated with the Archer® FusionPlex® CTL Kit, proving a false positive outcome in ALK FISH.
Table 1
Case | ALK FISH result | % positive nuclei | Average orange | Average green | Maximum orange/green | ALK IHC result | Archer result |
---|---|---|---|---|---|---|---|
1 | Positive | 15% | 1.91 | 1.82 | 4/4 | Negative | ALK negative |
2 | Positive | 18% | 3.95 | 3.87 | 13/12 | Negative | ALK negative |
3 | Positive | 15% | 2.72 | 2.60 | 6/5 | Negative | ALK negative |
FISH, fluorescence in-situ hybridization, IHC, immunohistochemistry.

Discussion
During routine practice we came across a case with false positivity for FISH, which in hindsight was related to polyploidy/high aneuploidy. By investigating the relation between polyploidy/high aneuploidy and break apart FISH analysis our study demonstrates that increased chromosome copy numbers are a cause of false positive outcomes in break apart FISH.
In practice experienced cytogeneticists and pathologists may be fully aware that polyploidy has impact on FFPE FISH interpretation, but less experienced colleagues may lack the morphologic background and may be prone to overdiagnosis in FISH. Moreover, the chance on false positivity with polyploidy has not been described before.
In the index case a dramatic increase in two genes (ALK and ROS1) was shown. Although the exact definition of polyploidy is an increased copy number of all chromosomes the most convincing explanation for the index case was polyploidy, taking into account that the two genes are situated on two different chromosomes. The alternative explanation of a very high gene/allele specific amplification is unlikely. The chance of finding even one gene with the very high amplification is in our opinion <1:100, for two genes in the same nucleus this amounts to <1:10,000. In combination with the exceptionally large size of the nuclear cross sections, the only likely explanation is polyploidy. The combination of polyploidy measured by total amount of DNA and nuclear size has been previously reported (14). Moreover, with only amplification of 2 genes (<0.0001% of total DNA) the nuclear size increase would be neglectable.
Certain tissues naturally harbour more polyploid cells than others. The most prominent example is the liver. During postnatal growth the liver parenchyma undergoes gradual polyploidization resulting in up to approximately 30–40% mainly tetraploid and octaploid cells (15-17). Therefore, we investigated liver cell nuclei of a control patient without underlying liver disease. As expected, in MET CISH as well as in ALK FISH analyses the number of double signals positively correlated with nuclear size. Larger nuclei harbour more detectable signals.
In theory every double signal has the same statistical chance of being cut apart by tissue sectioning and thus being falsely counted as a single signal. This implies that the statistical chance of a cell nucleus harbouring at least one single signal rises with higher numbers of double signals. It has been shown that in ALK negative tumours and even in normal lung tissue up to 11% of the cells score positive in break-apart FISH (10). Data from routine clinical practice indicate that up to 1/7 reported FISH ALK positive findings were false-positive (18,19). To deal with this stereological cutting phenomenon, the threshold has been set at minimally 15% of the tumour cell nuclei before interpreting a case as positive.
If we hypothesize that one out of ten normal and hypothetically diploid lung cells harbours a split signal, it would rise to two out of ten in tetraploid cells (assuming equally sized cells), four in octaploid cells and so on. In practice cells behave differently, but this example easily demonstrates that having a fixed cut-off, regardless the ploidy, is inadequate, and in practice likely leads to a false positive result in tumours with increased chromosome copy numbers.
A second theoretical point is that the number of double signals is dependent on section thickness. In liver cells we showed, that in 2 µm thick sections the average nuclear size and the number of signals were both significantly lower than in 5 µm thick sections. In a statistical model (Figure 6) it can be shown that the nuclear volume fraction is dependent on nuclear size and section thickness. A nucleus with a diameter of 5 µm for example has a chance of being fully (100%) in a 5 µm thick section, whereas in a 2 µm thick section only maximally 57% of the volume of the same nucleus can be presented in the section. In this case it results in a higher risk of a cutting artefact and single signals in the thinner section.
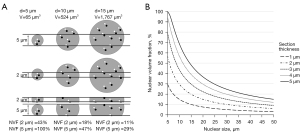
Thirdly, according to probe manufacturers, single signals as well as break-apart signals can be counted in tumour cells, resulting in a percentage of FISH positive cells. Thus, in case of a borderline negative break-apart pattern, truncation artefact might result in a shift to a borderline positive pattern.
As valuable as ISH analyses are, as careful one should be with the outcome, because technical as well as interpretational difficulties can make the analyses challenging, even for experienced specialists. Errors can occur as well in the pre-analytical, as in the analytical and post-analytical phase. General pre-analytical errors include fixation problems, inadequate tissue quality, sampling error, and low number of tumour cells. In the analytical phase technical difficulties are the main challenge. The post-analytical phase harbours interpretational difficulties, which are a common source of false results. For ALK FISH a split/break apart is commonly defined as two signals having a distance greater than two signal diameters (10). However, Martin et al. (11) showed that in the case of ALK FISH in adenocarcinoma of the lung the split length varies and may lead to artefacts and especially short splits can be difficult to distinguish from background noise (11). Furthermore, variations occurring between signal distances are related to nuclear features such as chromatin condensation, DNA stretching, or the three-dimensional position of a signal (11).
In samples negative for translocations or other anomalies, not all cells show the two fused signals due to procedural artefacts. This combined with clinical information led to defining cut-offs for the interpretation of FISH results. For ALK and ROS1 a threshold of ≥15% of the cells showing an aberrant pattern is commonly used to declare a sample positive for genomic rearrangements (12). However, due to technical difficulties and inter-observer variability (20,21) it is insufficient to use the 15% value as an absolute cut-off point, especially concerning therapeutic consequences. Therefore, in the case of ALK FISH it has been suggested to replace the cut-off of 15% by a borderline interval (22). In their model study von Laffert et al. showed that with the introduction of a borderline interval ranging from 10% to 20% the error rates of false classifications can be kept under 1% if at least 100 cells are evaluated (22). However, this model does not take increased chromosome copy numbers due to polyploidy or high aneuploidy into account.
The relevance of adequate knowledge in the technical application of FISH has been described in detail by Tibiletti before (23,24) and is emphasized in a recent study on the specificity of RET FISH analysis (25) as well as in the effect of an attenuated 3' signal, which may lead to an atypical pattern and possibly reduced sensitivity (26).
A limitation of the study is that with FISH analysis only individual genes/parts of chromosomes are analysed for variation in copy number (polysomy). As we focussed on polyploidy and the chance on false positive FISH, we did not examine the chance on false negative FISH. While samples from the liver could be examined in depth, for clinical samples this was limited.
Conclusions
ISH analyses are an important predictive tool to detect chromosomal anomalies. In non-small cell lung carcinoma cases false positivity due to high numbers of polyploid cells were found. Therefore, we state that the standard cut-off in FISH is inappropriate in case of polyploidy and should only be used with caution.
Acknowledgments
Marja Ramkema’s (Amsterdam University Medical Center, The Netherlands) support with FISH management and Dirk H.F.B. van Essen’s (Amsterdam University Medical Center, The Netherlands) help and advice with shallow sequencing experiments is greatly appreciated.
Funding: None.
Footnote
Reporting Checklist: The authors have completed the MDAR reporting checklist. Available at https://tlcr.amegroups.com/article/view/10.21037/tlcr-22-516/rc
Data Sharing Statement: Available at https://tlcr.amegroups.com/article/view/10.21037/tlcr-22-516/dss
Conflicts of Interest: All authors have completed the ICMJE uniform disclosure form (available at https://tlcr.amegroups.com/article/view/10.21037/tlcr-22-516/coif). ES is an employee of CellCarta, which is a profit organization that performs services for Pharma companies. LV is an expert panel member for Research Foundation – Flanders (FWO) and has stock options in CellCarta. MK is Chief Medical & Scientific officer and Shareholder of CellCarta. The other authors have no conflicts of interest to declare.
Ethical Statement: The authors are accountable for all aspects of the work in ensuring that questions related to the accuracy or integrity of any part of the work are appropriately investigated and resolved. The study was conducted in accordance with the Declaration of Helsinki (as revised in 2013). The study was approved by the privacy functionary of Amsterdam University Medical Center, and the Medical Ethics Review Committee of VU University Medical Center confirmed that this study did not fall within their scope of responsibility (No. IRB00002991). Individual consent for this retrospective analysis was waived. At the time of publication, the patient was deceased and patient data were anonymised.
Open Access Statement: This is an Open Access article distributed in accordance with the Creative Commons Attribution-NonCommercial-NoDerivs 4.0 International License (CC BY-NC-ND 4.0), which permits the non-commercial replication and distribution of the article with the strict proviso that no changes or edits are made and the original work is properly cited (including links to both the formal publication through the relevant DOI and the license). See: https://creativecommons.org/licenses/by-nc-nd/4.0/.
References
- Cheng L, Zhang S, Wang L, et al. Fluorescence in situ hybridization in surgical pathology: principles and applications. J Pathol Clin Res 2017;3:73-99. [Crossref] [PubMed]
- Soda M, Choi YL, Enomoto M, et al. Identification of the transforming EML4-ALK fusion gene in non-small-cell lung cancer. Nature 2007;448:561-6. [Crossref] [PubMed]
- Solomon B, Varella-Garcia M, Camidge DR. ALK gene rearrangements: a new therapeutic target in a molecularly defined subset of non-small cell lung cancer. J Thorac Oncol 2009;4:1450-4. [Crossref] [PubMed]
- Kwak EL, Bang YJ, Camidge DR, et al. Anaplastic lymphoma kinase inhibition in non-small-cell lung cancer. N Engl J Med 2010;363:1693-703. Erratum in: N Engl J Med 2011;364:588. [Crossref] [PubMed]
- Bubendorf L, Büttner R, Al-Dayel F, et al. Testing for ROS1 in non-small cell lung cancer: a review with recommendations. Virchows Arch 2016;469:489-503. [Crossref] [PubMed]
- Davies KD, Le AT, Theodoro MF, et al. Identifying and targeting ROS1 gene fusions in non-small cell lung cancer. Clin Cancer Res 2012;18:4570-9. [Crossref] [PubMed]
- Yasuda H, de Figueiredo-Pontes LL, Kobayashi S, et al. Preclinical rationale for use of the clinically available multitargeted tyrosine kinase inhibitor crizotinib in ROS1-translocated lung cancer. J Thorac Oncol 2012;7:1086-90. [Crossref] [PubMed]
- Shaw AT, Ou SH, Bang YJ, et al. Crizotinib in ROS1-rearranged non-small-cell lung cancer. N Engl J Med 2014;371:1963-71. [Crossref] [PubMed]
- Lindeman NI, Cagle PT, Aisner DL, et al. Updated Molecular Testing Guideline for the Selection of Lung Cancer Patients for Treatment With Targeted Tyrosine Kinase Inhibitors: Guideline From the College of American Pathologists, the International Association for the Study of Lung Cancer, and the Association for Molecular Pathology. Arch Pathol Lab Med 2018;142:321-46. [Crossref] [PubMed]
- Camidge DR, Kono SA, Flacco A, et al. Optimizing the detection of lung cancer patients harboring anaplastic lymphoma kinase (ALK) gene rearrangements potentially suitable for ALK inhibitor treatment. Clin Cancer Res 2010;16:5581-90. [Crossref] [PubMed]
- Martin V, Bernasconi B, Merlo E, et al. ALK testing in lung adenocarcinoma: technical aspects to improve FISH evaluation in daily practice. J Thorac Oncol 2015;10:595-602. [Crossref] [PubMed]
- Thunnissen E, Bubendorf L, Dietel M, et al. EML4-ALK testing in non-small cell carcinomas of the lung: a review with recommendations. Virchows Arch 2012;461:245-57. [Crossref] [PubMed]
- Vincenten JPL, van Essen HF, Lissenberg-Witte BI, et al. Clonality analysis of pulmonary tumors by genome-wide copy number profiling. PLoS One 2019;14:e0223827. [Crossref] [PubMed]
- Thunnissen FB, Peterse JL, Buchholtz R, et al. Polyploidy in pleomorphic adenomas with cytological atypia. Cytopathology 1992;3:101-9. [Crossref] [PubMed]
- Gentric G, Desdouets C. Polyploidization in liver tissue. Am J Pathol 2014;184:322-31. [Crossref] [PubMed]
- Duncan AW, Hanlon Newell AE, Smith L, et al. Frequent aneuploidy among normal human hepatocytes. Gastroenterology 2012;142:25-8. [Crossref] [PubMed]
- Kudryavtsev BN, Kudryavtseva MV, Sakuta GA, et al. Human hepatocyte polyploidization kinetics in the course of life cycle. Virchows Arch B Cell Pathol Incl Mol Pathol 1993;64:387-93. [Crossref] [PubMed]
- Savic S, Diebold J, Zimmermann AK, et al. Screening for ALK in non-small cell lung carcinomas: 5A4 and D5F3 antibodies perform equally well, but combined use with FISH is recommended. Lung Cancer 2015;89:104-9. [Crossref] [PubMed]
- Gao X, Sholl LM, Nishino M, et al. Clinical Implications of Variant ALK FISH Rearrangement Patterns. J Thorac Oncol 2015;10:1648-52. [Crossref] [PubMed]
- McLeer-Florin A, Lantuéjoul S. Why technical aspects rather than biology explain cellular heterogeneity in ALK-positive non-small cell lung cancer. J Thorac Dis 2012;4:240-1. [PubMed]
- von Laffert M, Penzel R, Schirmacher P, et al. Multicenter ALK testing in non-small-cell lung cancer: results of a round robin test. J Thorac Oncol 2014;9:1464-9. [Crossref] [PubMed]
- von Laffert M, Stenzinger A, Hummel M, et al. ALK-FISH borderline cases in non-small cell lung cancer: Implications for diagnostics and clinical decision making. Lung Cancer 2015;90:465-71. [Crossref] [PubMed]
- Tibiletti MG. Specificity of interphase fluorescence in situ hybridization for detection of chromosome aberrations in tumor pathology. Cancer Genet Cytogenet 2004;155:143-8. [Crossref] [PubMed]
- Tibiletti MG. Interphase FISH as a new tool in tumor pathology. Cytogenet Genome Res 2007;118:229-36. [Crossref] [PubMed]
- Radonic T, Geurts-Giele WRR, Samsom KG, et al. RET Fluorescence In Situ Hybridization Analysis Is a Sensitive but Highly Unspecific Screening Method for RET Fusions in Lung Cancer. J Thorac Oncol 2021;16:798-806. [Crossref] [PubMed]
- Smuk G, Pajor G, Szuhai K, et al. Attenuated isolated 3' signal: A highly challenging therapy relevant ALK FISH pattern in NSCLC. Lung Cancer 2020;143:80-5. [Crossref] [PubMed]