A narrative review from gut to lungs: non-small cell lung cancer and the gastrointestinal microbiome
Introduction
Lung cancer is one of the leading causes of cancer related death in both men and women (1). Traditionally, cancer formation has been associated with spontaneous mutations during DNA replication that may be exacerbated by environmental factors, such as exposure to ultraviolet (UV) radiation, toxic substances, and diet (2). The genomic model of drug target discovery has been critical in developing a multitude of different small molecule inhibitors targeting different molecular oncogenic drivers in non-small cell lung cancer (NSCLC). However, beyond the genomic model of cancer therapy, the paradigm of leveraging the immune system to eradicate cancer has manifested in the clinic with monoclonal antibody-based therapies, specifically immune checkpoint inhibitors (ICI), which have heralded immunotherapy as a major pillar of treatment for patients without an actionable oncogene driver, especially in unresectable or metastatic NSCLC (3).
In the past decades, studies have explored the role of microorganisms as a key determinant across the entire spectrum of health. In 2008, the Human Microbiome Project, funded by the National Institute of Health, aimed to characterize the human microbiome. They isolated DNA from different organs from healthy adults and conducted 16s rRNA and meta-genomics shotgun sequencing on these samples (4,5). Using these data, several studies linked the human microbiome from varied organs to chronic diseases including Crohn’s disease, ulcerative colitis, asthma, and chronic obstructive pulmonary disease (COPD), amongst others (4,6-10). Since then, higher-throughput sequencing, meta-genomics, and metabolomics have allowed researchers to label and archive the gut microbiome, predict its function, and predict its metabolic byproducts, respectively, and have greatly improved our understanding on the role of the microbiome (11). Gut microbiota refers to the heterogenous population of commensal microorganisms such as bacteria, fungi, viruses, and protozoans that populate the gastrointestinal tract, and are responsible for essential life functions such as metabolizing food components and drugs, vitamin production, and protecting against gut infiltration by pathogenic gut bacteria (11).
There is a complex interface between the gastrointestinal microbiota, host endocrine, nervous, and immune system (12). Host hormones modify gut microbiota composition during stress responses, which impact gastrointestinal motility and digestive function (13). Similarly, gut microbiota secretes hormones detected by the gut-brain-immune-axis, allowing them to modulate the host’s metabolism of drugs and their immune system, as well as protect against pathological gastrointestinal bacteria, which potentially cause 20% of tumorigenesis (13,14). Using this complex interface, one well-studied mechanism by which gut microbiota affects cancer genesis is via microbial derived short chain fatty acids (15,16). For example, gut bacterial butyrate and propionate inhibit the host tumor cell’s histone deacetylases which have an anti-cancer effect in colorectal cancer and lymphoma (15,16). Another example is bacterial lipopolysaccharide in gram-negative bacteria activating host cell surface receptor toll like receptor 4, which in turn activates a T cell mediated response against cancer cells (17). Additionally, gut microbiota can also act as a tumor promoter. For example, Heliobacter pylori produces protein CagA, one of the earliest gastrointestinal pathogen-made proteins contributing to human cancer (18,19). Furthermore, gut dysbiosis allows bacterial pathogens to expand and release toxins which induce DNA breaks, cause genomic instability, and potentiate cancer genesis in predisposed cells (20-22). It can also interfere with DNA repair pathways and DNA damage response leading to induction of pathogenic p53 degradation via the secretion of enzymes (23). These historical data highlight the inter-relatedness between the gut microbial ecosystem and its host across multiple physiologic systems, and that any disruption in homeostasis has the possibility of promoting health or disease.
Historically, it was understood that cancer could be instigated via the generation of metabolites and byproducts that are not only essential for host homeostasis, but also in potentiating treatment outcomes (23-27). This is especially well studied in the case of gut populating bacteria or gut microbiota. For example, pathological dysbacteriosis of gut microbiota modulates the gut epithelium and immune system by triggering inflammation from the production of high levels of toxins, contributing to tumorigenesis in cancer (20). Similarly, several gut microorganisms have been shown to improve lung cancer prognosis, whether it is probiotics improving gut dysbiosis in cancer patients receiving chemotherapy and radiation, or a correlation between the gut microbial milieu and its potentiating effect (both beneficial and deleterious) on immunotherapy (28-30). In contrast, use of antibiotics or developing specific bacterial infections may decrease the effectiveness of immunotherapy in cancer (30,31).
More recently, a new light has been cast on altering gut microbial composition to improve outcomes in cancer. With the prevalent use of ICI in many cancer subtypes, including NSCLC, there has been a keen interest in studying the relation and effect of the gut microbiome on immunotherapy given the bidirectional relation between the gut microbiome and immunity. The microbiome field has been re-invigorated in the past 5 to 10 years because of multiple landmark pre-clinical studies demonstrating and re-iterating the role of gut microbial diversity and gut microbial taxa enrichment in influencing response to ICI. Consistently, the data showed that responders to ICI had greater gut microbial diversity and a relative abundance of specific gut microbiota (32-35). In fact, recently published early phase human studies demonstrated that transplanting stool microbiota from patients with melanoma responding to ICI into those that were resistant or refractory to ICI to begin with (i.e., non-responders), led to a significant subset of these non-responding patients to become sensitized to the same ICI treatment, thereby demonstrating proof-of-concept that fecal microbiota transplant can modulate the systemic and tumor immune milieu in humans (35,36).
Given these data, it is becoming evident that a full characterization of the gut microbiome during tumor suppression and growth, and how this relates to the immune and metabolic response, will be a major pillar of cancer-related research. Therefore, our objective for this paper is to set the stage by discussing the links between microbiome biology and lung cancer and to provide a narrative review of recent developments in this space. Specifically, we will present a discussion on the link between the gut microbiome and lung cancer, as well as patient, tumor, and gut microbiome characteristics affecting the efficacy of ICI in NSCLC. We present the following article in accordance with the Narrative Review reporting checklist (available at https://tlcr.amegroups.com/article/view/10.21037/tlcr-22-595/rc).
Methods
We used PubMed, EMBASE, and ClinicalTrials.gov to conduct a search using the following terms: Non-small cell lung cancer (NSCLC), gut, and microbio* (the asterix represents a wildcard symbol that extends the search to include all items that start with “microbio”). We reviewed all studies published until July 11, 2022. We manually screened the resulting articles from PubMed and EMBASE meeting the criteria outlined in Table 1. We used the search terms on ClinicalTrials.gov to identify ongoing trials.
Table 1
Items | Specification |
---|---|
Date of search | July 11, 2022 |
Databases and other sources searched | PubMed, EMBASE, Clinical Trials.Gov |
Search terms used | Non-small cell lung cancer (NSCLC), gut, microbio* |
Timeframe | Indefinite-July 11, 2022 |
Inclusion and exclusion criteria | • Population: patients (age 18 years and older) with NSCLC |
• Concept: impact of gut microbiome on pathophysiology, outcomes, and treatments | |
• Context: original articles, abstracts, and reviews in English language | |
Selection process | The selection was conducted by the two authors who concurred on the studies included |
*, represents a wildcard symbol that extends the search to include all items that start with “microbio”. NSCLC, non-small cell lung cancer; MeSH, Medical Subject Headings.
Results
PubMed
In PubMed, 26 of 27 citations met the study eligibility criteria, of which 24 studies presented original research data. The majority of the studies (50%, 12/24) focused on the impact of antibiotics on the outcomes of patients with NSCLC treated with immunotherapy. Other studies addressed the impact of other concomitant therapies on immunotherapy outcomes in NSCLC (20.8%, 5/24) and the impact of gut microbiome composition on lung carcinogenesis (16.7%, 4/24). Two studies each addressed the negative impact of antibiotics on gut microbiome and the influence of gut microbiome diversity on immunotherapy efficacy in lung cancer. One study examined the association of abnormal bowel movement patterns on immunotherapy efficacy. Finally, one study is ongoing and has not presented their results yet.
EMBASE
Of 37 eligible studies, one was a study protocol, and 36 studies presented original research data. Eight (22.2%) discussed the impact of gut microbiome on lung carcinogenesis and 2.8% (1/36) discussed the impact of chemotherapy on gut microbiome. The impact on immunotherapy by gut microbiome composition and by concurrent therapies were discussed in 38.9% (14/36) and 33.3% (12/36) of studies, respectively. Amongst studies discussing concurrent therapies, most studies focused on antibiotics (8/36, 22.2%) and proton pump inhibitors (PPIs) (5/36, 13.9%). A smaller number of studies researched the impact of probiotics on immunotherapy (2/36, 5.6%).
Clinical trials
The study of the gut microbiome as it relates to NSCLC and other cancers has largely shifted its focus onto the immune-oncology space. Of the thirty-one trials listed in ClinicalTrials.gov resulted from our search outline (Table 2), 25 met our eligibility criteria based on the “Population (or Participants), Concept, and Context” model. The remainder of studies focused on novel probiotic treatments, fecal transplants, and using gut microbiome as a biomarker in predicting immunotherapy response. The most common immunotherapies explored were nivolumab, pembrolizumab, and durvalumab. One study explored the impact of gut microbiome on toxicity from immunotherapy and two studies explored lifestyle factors that intersected with both lung cancer and gut microbiome, such as meal intervention and sleep apnea.
Table 2
Study title | Study type | Age (years) | Interventions | Outcome measures | NCT number |
---|---|---|---|---|---|
Gut Microbiota and Cancer Immunotherapy Response | Observational | 18–75 | Other: Response to anti-PD-1/PD-L1 | Diversity and composition of gut microbiota | NCT04682327 |
Other: Non-response to anti-PD-1/PD-L1 | Concentration of peripheral blood mononuclear cells | ||||
Concentration of tumor immune related cytokines | |||||
Incidence of anti-PD-1/PD-L1 related adverse events | |||||
Effects of Probiotics on the Gut Microbiome and Immune System in Operable Stage I-III Breast or Lung Cancer | Interventional | ≥18 | Procedure: biopsy | Length and adherence of probiotics | NCT04857697 |
Procedure: biospecimen collection | Percentage of CD8+, CD4+, and T-reg cells | ||||
Drug: probiotic | Cytokine counts | ||||
Procedure: therapeutic conventional surgery | |||||
Gut Microbiota Reconstruction for NSCLC Immunotherapy | Interventional | 18–75 | Procedure: capsulized fecal microbiota transplant | Incidence of FMT-related adverse events | NCT05008861 |
Drug: anti-programmed cell death protein 1/programmed death-ligand 1 monoclonal antibody | Incidence of anti-PD-1/PD-L1-related adverse events | ||||
Drug: platinum based chemotherapy | Changes in diversity and composition of gut microbiota | ||||
Efficiency of FMT engraftment | |||||
Changes in concentration of peripheral blood mononuclear cells | |||||
Changes in concentration of tumor immune related cytokines | |||||
Objective response rate | |||||
Supplementation of n-3 PUFA in the Modulation of Lean Mass in Patients with Lung Cancer Receiving a High-protein Diet | Interventional | 20–90 | Drug: placebo | Change/maintenance in overall skeletal muscle density | NCT04965129 |
Drug: fish oil | Change in muscle mass | ||||
Red blood cell omega-3 fatty acid concentrations (EPA and DHA) | |||||
Muscle strength | |||||
Change in fecal microbiome composition | |||||
Change in levels of miRNA 133 | |||||
Identification of a Predictive Metabolic Signature of Response to Immune Checkpoint Inhibitors in Non-Small Cell Lung Carcinoma | Observational | 18–100 | Other: immune signature in serum associated to the metabolic signature | Identification of the change from baseline metabolic signature as predictive factor of the ICI response at 6 months or at the tumoral progression | NCT04189679 |
Genetic: meta-genomic signature of intestinal flora | Identification of the link between the Meta-genomic and immune signatures and the metabolic signature at 6 months or at the tumoral progression | ||||
Identification of the link between the Meta-genomic and immune signatures and ICI response at 6 months or at the tumoral progression | |||||
Description of the profile change of Meta-genomic signature | |||||
Description of the profile change of Immune signature at 2 months or at the tumoral progression | |||||
Identification of the link between the profile change of meta-genomic signature and the ICI response at 2 months or at the tumoral progression | |||||
Identification of change of immune signature and the ICI response at 2 months or at the tumoral progression | |||||
Description of overall survival under ICI at 6 months or at the tumoral progression | |||||
Description of the survival without progression under ICI at 6 months or at the tumoral progression | |||||
The Effect of Chronic Stress on Efficacy of ICIs in ¢ó-IV Lung Cancer Patients | Observational | ≥18 | Other: gut microbiota detection | ORR | NCT05477979 |
Other: peripheral immune cells signature detection | PFS | ||||
Effect of chronic stress on efficacy of immune checkpoint inhibitors | |||||
OS | |||||
Effect of chronic stress on quality of life | |||||
Lactobacillus Bifidobacterium V9(Kex02)Improving the Efficacy of Carilizumab Combined With Platinum in Non-small Cell Lung Cancer Patients | Interventional | 18–70 | Other: V9 | Objective remission rate | NCT05094167 |
Other: placebo | PFS | ||||
OS | |||||
Microbiota and the Lung Cancer | Interventional | 18–80 | Other: sampling | Difference in diversity of the lungs and upper airways microbiota | NCT03068663 |
Effect of chemotherapy on microbiota (by comparing before and after chemotherapy) | |||||
Inflammatory status | |||||
Effect of microbiota on pulmonary immune cells | |||||
Immune Response Under Immunotherapy in Metastatic NSCLC: Sputum, Blood Samples and Microbioata Study | Observational | ≥18 | Other: blood | Immune and inflammatory response in the blood | NCT04804137 |
Other: sputum | Immune and inflammatory response in the airways | ||||
Other: saliva | Analysis of gut microbiota | ||||
Other: stool | Analysis of lung microbiota | ||||
Camu-Camu Prebiotic and Immune Checkpoint Inhibition in Patients with Non-small Cell Lung Cancer and Melanoma | Interventional | ≥18 | Biological: Camu Camu Capsules (Camu Camu powder encapsulated (500 mg each) + ICI | Incidence of treatment-related adverse events (safety and tolerability) in patients with NSCLC and melanoma | NCT05303493 |
Objective response rate in the NSCLC and melanoma cohort by RECIST criteria | |||||
Microbiota Transplant in Advanced Lung Cancer Treated with Immunotherapy | Interventional | ≥18 | Dietary supplement: microbiota transplant plus anti PD1 therapy | Measure of safety | NCT04924374 |
Drug: anti PD1 therapy | Measure of efficacy | ||||
Black Raspberry Nectar for the Prevention of Lung Cancer, BE WELL Study | Interventional | 55–77 | Procedure: biospecimen collection | Recruitment rates | NCT04267874 |
Dietary supplement: nutritional supplementation | Study adherence | ||||
Drug: placebo administration | Inflammatory markers found in nasal brushings | ||||
Other: questionnaire administration | Changes in stool microbe relative abundances | ||||
Biologic responses to the black raspberry (BRB) nectar | |||||
The Gut Microbiome and Immune Checkpoint Inhibitor Therapy in Solid Tumors | Observational | ≥18 | Drug: checkpoint inhibitor, immune | Change in microbiome composition from baseline to after cycle 2 of checkpoint therapy (6–8 weeks) by analyzing longitudinally-collected stool specimens of 800 patients with primary NSCLC, MM, RCC, and TNBC | NCT05037825 |
Microbiome samples correlation | |||||
Microbiome correlation to blood biomarkers | |||||
Blood samples correlation | |||||
A Clinico-biological Database of Lung Cancers | Observational | “Child, Adult, Older Adult” | Procedure: tissue biopsies | Objective response rate assessed by CT scan | NCT03387865 |
Procedure: blood sampling | Disease progression rate assessed by CT scan | ||||
Genetic: DNA banking | PFS | ||||
OS | |||||
Treatment toxicity | |||||
Role of Gut Microbiome and Fecal Transplant on Medication-Induced GI Complications in Patients with Cancer | Interventional | ≥18 | Other: best practice | Difference in stool microbiome pattern | NCT03819296 |
Other: biospecimen collection | Incidence of AE of FMT (Project 3) | ||||
Procedure: endoscopic procedure | Immune profile factors associated with onset of ICI-related colitis in blood and colon tissue | ||||
Genetic factors associated with onset of ICI-related colitis in blood and colon tissue | |||||
Endoscopic and histologic features of ICI-related colitis before and after medical treatment | |||||
Changes of ICI-related symptoms | |||||
Changes of quality of life | |||||
Cytokine features that are associated with good response to FMT | |||||
Genetic factors associated with onset of ICI-related colitis in blood and colon tissue | |||||
Changes of endoscopic and histologic features of ICI-related colitis before and after FMT | |||||
Evaluating Modifiable Biomarkers for the Prediction of Immunotherapy Response and Toxicity | Observational | ≥18 | Procedure: biospecimen collection | Performance of the microbiome as predictive indicator of clinical benefit (OS, PFS) | NCT04954885 |
Other: physical performance testing | Performance of the microbiome as prognostic indicator of clinical benefit (OS, PFS) | ||||
Other: questionnaire administration | Performance of functional status as predictive indicator of clinical benefit (PFS) | ||||
Performance of functional status as prognostic indicator of clinical benefit (PFS) | |||||
ARGONAUT: Stool and Blood Sample Bank for Cancer Patients | Observational | ≥18 | Drug: immunotherapy | Determine whether the microbiome composition can predict progression-free survival | NCT04638751 |
Drug: chemotherapeutic agent | Identify correlations between microbiome composition and immune markers | ||||
Determine whether the microbiome composition can predict overall survival | |||||
Build a library of samples and data for future research | |||||
A First-in-human (FIH) Combination Treatment Study With a Single Dose Level of BMC128 | Interventional | ≥18 | Drug: BMC128 | Number and severity of drug-related treatment emergent AEs | NCT05354102 |
Drug: nivolumab | |||||
Nutricare Plus a Medically Tailored Meal Intervention Among Patients With Lung Cancer | Interventional | 18–100 | Behavioral: nutritional counseling | Nutritional intake | NCT04986670 |
Other: medically tailored meals | Weight | ||||
Behavioral: nutrition prescription | Treatment compliance | ||||
Treatment-related toxicities | |||||
Hospitalizations and ED visits | |||||
Patient-reported symptoms | |||||
Patient quality of life | |||||
Patient-reported functional outcomes | |||||
Obstructive Sleep Apnea Influences Efficacy of PD-1-Based Immunotherapy Against Non-Small Cell Lung Cancer | Observational | ≥18 | ORR, PFS, OS | NCT04743752 | |
Compared the baseline sleep monitor results between Group NSCLC and Group OSA+NSCLC | |||||
Factors associated with ORR in NSCLC patients | |||||
Factors associated with OS and PFS in NSCLC patients | |||||
Compared the baseline level of lymphocytes classification and count between Group NSCLC and Group OSA+NSCLC | |||||
Compared the baseline level of inflammatory biomarkers between Group NSCLC and Group OSA+NSCLC | |||||
The association between OSA and baseline inflammatory biomarkers, peripheral lymphocytes classification and count | |||||
Compared the baseline gut microbiome between Group NSCLC and Group OSA+NSCLC | |||||
The association between OSA and the diversity of gut microbiome | |||||
Safety and Potential Efficacy of MS-20 In Combination With Pembrolizumab for the Treatment of NSCLC | Interventional | ≥20 | Drug: MS-20 | The incidence of treatment-emergent AE | NCT04909034 |
Drug: Placebo | ORR, PFS, DCR, DOR | ||||
Bioforte Technology for in Silico Identification of Candidates for a New Microbiome-based Therapeutics and Diagnostics | Observational | ≥18 | Biological: Collection of stool, blood (PBMC) and biopsy (FFPE) | Microbial diversity in stool samples | NCT04136470 |
Number of Responders and Non-responders on immunotherapy (evaluated using RECIST 1.1 criteria) | |||||
Development and Analysis of a Stool Bank for Cancer Patients | Observational | ≥18 | Drug: pembrolizumab injection | Checkpoint inhibitor response | NCT04291755 |
Durvalumab(MEDI4736) After chemoradiotherapy(DART) for NSCLC-a Translational and Biomarker Study | Interventional | ≥18 | Drug: durvalumab injection | Impact of TMB measured in the tumour tissue and blood samples, on the hazard | NCT04392505 |
Predict association between Tumour Mutational Burden, TMB, measured in tumour tissue and blood samples and clinical response | |||||
Specific RNA profiles predict response to treatment | |||||
Impact of molecular profiles in urine on response to treatment | |||||
Analysis of pre-treatment and under-treatment samples may identify biomarkers for predicting which patients will benefit from treatment with durvalumab after chemoradiation | |||||
The durvalumab treatment following chemoradiation will induce T cell responses against antigens expressed in each patient´s tumor | |||||
A possible change in PD-L1 status will be explored | |||||
The durvalumab treatment following chemoradiation has acceptable safety and tolerability in NSCLC patients | |||||
A Pilot Study to Explore the Role of Gut Flora in Metastatic Mesothelioma | Observational | “Child, Adult, Older Adult” | Other: no intervention | Correlation of microbiome to disease via relative abundance found in microbiome sequencing | NCT04214015 |
Stereotactic Ablative Radiotherapy with Nivolumab for Early-Stage Operable Non-Small Cell Lung Cancer | Interventional | ≥18 | Combination product: nivolumab | Percentage of pathologic complete response after pre-operative therapy | NCT04271384 |
Major pathological response | |||||
Safety: treatment-related AEs with continuous toxicity measure as per CTCAE v4.0 | |||||
ORR, OS | |||||
Relapse-free survival | |||||
Resectability rate | |||||
30-day surgical mortality | |||||
Number of pathologic positive lymph nodes | |||||
Correlated translational endpoints | |||||
Gender Difference in side effects of immunotherapy: a Possible Clue to Optimize cancer treatment | Observational | ≥18 | Drug: immunotherapy | Immune-related severe (grade ≥2) AEs | NCT04435964 |
Immune-related AEs | |||||
The Clinical Study of Modern Therapies on Flora in Body Fluids and Blood of Malignant Tumor Patients | Observational | 18-85 | Diagnostic test: flora analysis | The change of diversity of intestinal flora in faeces during therapy | NCT04202848 |
The change of diversity of urethral flora in urine during therapy | |||||
The change of diversity of oral flora in pharyngeal swab during therapy | |||||
The change of diversity of flora in blood during therapy | |||||
The change of abundance of intestinal flora in faeces during therapy | |||||
The change of abundance of urethral flora in urine during therapy | |||||
The change of abundance of oral flora in pharyngeal swab in urine during therapy | |||||
The change of abundance of flora in blood during therapy | |||||
The change of concentration of purine metabolites in urine during therapy | |||||
The change of concentration of P-hydroxyphenylalanine metabolites in urine | |||||
The change of the number of tumor necrosis factor-α in blood during the therapy | |||||
The change of the number of immune cell marker (CD3, CD4, CD8, etc.) in blood during the therapy | |||||
The change of the number of C-reactive protein in blood during the therapy | |||||
The change of the number of tumor marker in blood during the therapy | |||||
The change of the number of Gastrin in blood during the therapy | |||||
Microbiome Immunotherapy Toxicity and Response Evaluation | Observational | ≥18 | Drug: nivolumab | Can the microbiome signature predict PFS of 1 year or greater | NCT04107168 |
Drug: pembrolizumab | Can the microbiome signature predict PFS | ||||
Drug: ipilimumab | Can the microbiome signature OS | ||||
Can the microbiome signature to predict relapse | |||||
Does the microbiome correlate with treatment efficacy | |||||
Correlate microbiome findings with incidence and characteristics of immune-related adverse events | |||||
Correlation microbiome findings and known characteristics of patients | |||||
Control for the microbiome of cancer patients | |||||
Build a library of biological samples for future research | |||||
Establishment of the Human Intestinal and Salivary Microbiota Biobank - Oncologic Diseases | Observational | 18-75 | Other: biological sample collection | Biological samples collection for establishment of the first National Microbiome Biobank | NCT04698161 |
Other: questionnaire | |||||
Other: medical examination | |||||
Comprehensive Outcomes for After Cancer Health | Interventional | ≥18 | Behavioral: digital health coaching program | Acceptability of coaching intervention | NCT05349227 |
Device: fitbit | Feasibility of coaching intervention | ||||
Change in self-efficacy for managing chronic disease | |||||
Change in the National Institutes of Health PROMIS Short Form v2.0 Physical Function 10a | |||||
Change in Quality of Life as measured by The Functional Assessment of Cancer Therapy - General v4 | |||||
Change in anxiety as measured by the PROMIS Anxiety Short Form 8a | |||||
Change in Depression as measured by the PROMIS Depression Short Form 8a | |||||
Change in Cognitive Function as measured by the PROMIS Cognitive Function Short Form 8a | |||||
Change in Sleep-related Impairment as measured by the PROMIS Sleep related Impairment Short Form 8a | |||||
Change in the PROMIS Brief Sexual Function & Satisfaction Measure Male | |||||
Change in the PROMIS Brief Sexual Function & Satisfaction Measure Female | |||||
Change in The Comprehensive Score for Financial Toxicity | |||||
Change in Economic Strain and Resilience in Cancer | |||||
Change in symptom burden as measured by the Edmonton Symptom Assessment System-Revised |
NSCLC, non-small cell lung cancer; NCT, National Clinical Trial; PUFA, polyunsaturated fatty acids; EPA, eicosapentaenoic acid; DHA, docosahexaenoic acid; anti-PD-1/PD-L1, Anti-programmed cell death protein 1/programmed death-ligand 1 monoclonal antibody; FMT, fecal microbiota transplant; miRNA, microRNA; ICI, immune checkpoint inhibitor; ORR, objective response rate; PFS, progression free survival; OS, overall survival; RECIST, Response evaluation criteria in solid tumors; MM, melanoma; RCC, renal cell carcinoma; TNBC, triple negative breast cancer; DNA, Deoxyribonucleic acid; CT, computed tomography; AE, adverse event; OSA, obstructive sleep apnea; DCR, Disease Control Rate; DOR, Duration of Response; PBMC, Peripheral blood mononuclear cells; FFPE, Formalin-Fixed Paraffin-Embedded; TMB, tumor mutational burden; RNA, Ribonucleic acid; CTCAE, Common Terminology Criteria for Adverse Events; PROMIS, Patient Reported Outcomes Measurement Information System.
Discussion
Impact of gut microbiome composition on lung carcinogenesis
Lung carcinogenesis is influenced by several microbiomes, including those from lung and sputum as shown in Figure 1 (37-41). For example, in a prospective study, pulmonary lobectomy samples from twenty-nine patients with pulmonary squamous cell carcinoma or adenocarcinoma who did not receive antibiotics or neoadjuvant chemotherapy within 3 months prior to surgery and had a tumor larger than 2 cm were tested against tumor-adjacent healthy tissue from the same patients (42). They were also tested against an experimental control accounting for all steps of the extraction and sequencing protocol for each patient to manage any interference in data from contaminants. Interestingly, the bacterial composition was different not only between tumor cells and the negative sample, but also within the tumor cells and healthy tissue of the same patient. Furthermore, Escherichia-Shigella, Faecalibacterium, Pseudomonas, Enterobacteriaceae, Alloprevotella, and Brevundimonas, which are normally enteric genera (arising from the bowel) were uniquely present in these lung cancer samples (42).
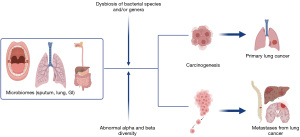
Similarly, while it is evident from previous literature that the gut microbiome has some impact on lung carcinogenesis, we sought to examine the specific characteristics of gut microbiota that influenced it. Although the pathophysiology is not known, various hypotheses exist, including “the gut-lung axis” and immunomodulation, amongst others. The “gut-lung axis” was explained in detail in a systematic review on gut microbiome dysbiosis and pulmonary immunology (43). We have briefly summarized this proposed mechanism in Figure 2. Specifically, it postulated that gut microbiome alterations would result in gastrointestinal tract dendritic cells processing specific antigen cells, leading to proliferation and expansion of various T cell subsets against them. In turn, these T cells would cause altered systemic inflammation by producing cytokines that migrate to other organs, including the lung, using immune homing molecules, thus contributing to the development of conditions such as COPD, asthma, and acute respiratory distress syndrome (43).
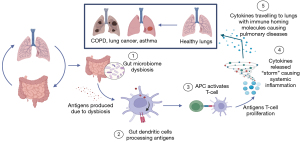
Knowing this connection between gut microbiome dysbiosis and lung carcinogenesis, it is important to identify which characteristics of the gut microbiome specifically are involved in this mechanism. A critical component of gut microbiota is butyrate-producing bacteria in the gut, which are involved in positive immunomodulation and improving gut barrier functions (44). A prospective case-control study comprising 60 patients (30 patients diagnosed with NSCLC and their matched controls, aged approx. 66–67 years old), examined the association of the eight most abundant butyrate-producing gut bacteria with NSCLC (45). They collected their stool samples and performed qualitative polymerase chain reaction (PCR) to measure gut butyrate-producing bacteria in the two groups. They found higher levels of Faecalibacterium prausnitzii, Clostridium leptum, Clostridial cluster I, Ruminococcus spp., Clostridial cluster XIVa, and Roseburia spp. in the healthy controls. Furthermore, Roseburia spp. and Clostridial cluster IV were inversely correlated with higher monocytes and leukocytes, respectively, which are potential independent negative prognostic markers in lung cancer; no other correlations between other bacteria or prognostic markers were found (45,46). These findings are supported by another prospective observational study of 70 Japanese patients with advanced NSCLC receiving single-agent immunotherapy whose fecal samples and antibiotic usage were collected at baseline whereby they examined the association of clinical outcomes with gut microbiome diversity in patients without antibiotic exposure for a “natural history” (47). They noted Ruminococcaceae UCG 13 and Agathobacter enrichment, both butyrate-producing bacteria, were associated with longer overall survival (OS) and progression-free survival (PFS) (47). Additionally, they reported decreased alpha diversity was associated with decreased OS. This observation was supported by another study of 121 patients comprising 30 sputum and 29 fecal samples from healthy controls and 66 sputum and 85 fecal samples from patients with NSCLC (48). They found many differences between the types of sputum and gut bacteria in patients with and without NSCLC. For example, sixteen genera of bacteria were in low quantity in sputum samples from patients with NSCLC in comparison to their healthy counterparts. Also, patients with NSCLC had less diversity in the type of bacteria in their sputum and gut samples. When comparing the sputum and gut samples in patients with NSCLC, the microbial markers or certain common bacterial taxa were similar between the groups, but they only shared one genus, Streptococcus. While sputum and fecal samples also differed in other categories like number of phyla present, the most important difference was that sputum samples were more predictive of patient stratification than fecal samples. Approximately 75% of the top twenty genera that contributed to predictive models for NSCLC outcomes came from sputum samples (48). Lastly, there was significant beta diversity, defined as the difference in microbiome between two bacterial communities, between sputum from patients with metastatic NSCLC to brain vs. other sites and its dysbiosis was more associated with brain metastases than in fecal samples. Both sputum and fecal samples were similarly enriched with Pseudomonas in patients with brain metastases (48).
In summary, gut microbiome composition and diversity in patients with NSCLC seems to be different than in their healthy counterparts, and gut microbiome dysbiosis may influence lung carcinogenesis. However, this review also identified that other sources of microbial dysbiosis, such as in the sputum, may be more associated with NSCLC and are becoming important future areas of research.
Impact of antibiotics on gut microbiome
As illustrated in the previous section, gut microbiome dysbiosis plays a major role in lung carcinogenesis. One risk factor for gut microbial dysbiosis is the use of antibiotics (47). One study reported decreased alpha diversity and different fecal microbiome composition with antibiotic use: Abundance of Clostridia (Ruminococcaceae UCG 13, Clostridiales, and Agathobacter) in patients without antibiotics, and abundance of Hungatella in those treated with antibiotics (47). Interestingly, this was also noted in blood microbiome composition and patients with NSCLC who received antibiotics. In a prospective trial of 72 patients with advanced NSCLC treated with nivolumab, blood microbiome analysis showed that patients who did not receive antibiotics within 2 months prior to and one month of starting nivolumab had higher levels of Solibacteres, which was associated with tumor response in comparison to their antibiotic-treated counterparts (49). Based on these data, it is evident that antibiotics can modify microbiome composition, especially gut microbiome, and may have an impact on the disease trajectory of NSCLC.
Factors influencing immunotherapy efficacy in NSCLC
Gut microbiome diversity and composition
Given the practice changing and revolutionary improvements in survival outcomes achieved with first line immunotherapy in NSCLC, it is critical to maximize these benefits by identifying factors that may modify its efficacy. Gut microbiome composition and diversity seems to be an essential theme. One of the earlier studies examining this was conducted in forty-two Chinese patients with advanced NSCLC who underwent treatment with nivolumab after disease progression on 1–2 lines of treatment (30). DNA was extracted from fecal samples collected at baseline and every 2 weeks thereafter until disease progression on nivolumab. Those with disease progression at first clinical assessment were classified as non-responders, and those with stable disease or partial response based on RECIST 1.1 criteria after 3 cycles that was confirmed on an additional evaluation 4 weeks later were classified as responders. There were seven microbial genera that were differentially abundant at baseline in treatment responders and non-responders: Higher measures of Ruminococcus were seen in non-responders to nivolumab, and higher measures of Alistipes, Prevotella copri, Bifidobacterium longum, Lachnobacterium, Lachnospiraceae, and Shigella were noted in treatment responders (30). However, there was no significant change in microbiome composition in subsequent fecal measurements after baseline measurement in either the responder or the non-responder group with nivolumab treatment (30). Furthermore, it was noted that higher alpha diversity independently led to improved PFS on nivolumab, and that beta diversities were different in responders and non-responders. Finally, the peripheral T cell and natural killer cell signatures were higher in patients with higher gut microbiome diversity. This suggests an association between gut microbiome and immunomodulation, which may explain the improved outcomes in patients with certain types of gut microbiota. Similar differences have been reported in several other studies in lung cancer and melanoma (30,32), including a recent prospective study where stool samples were collected from patients with advanced NSCLC prior to starting treatment with pembrolizumab (n=42), nivolumab (n=4), and sintilimab (n=17) (50). They also demonstrated higher beta diversity and differential bacterial composition in patients with PFS greater than 6 months on immunotherapy.
Effect of concomitant therapies on immunotherapy efficacy in NSCLC
Probiotics
One potential approach to improving therapeutic efficacy of immunotherapy is by modulating gut microbiota composition with metabolite-producing probiotics, which have demonstrated efficacy in improving indigestion, constipation, and other markers of gut dysbiosis. A retrospective analysis of 118 Japanese patients with advanced NSCLC who received nivolumab, pembrolizumab, or atezolizumab were compared in the following subgroups: those receiving probiotic treatment with Clostridium butyricum within 6 months of immunotherapy treatment and/or concurrently with immunotherapy (n=39) vs. those who did not (n=79) (51). Clostridium butyricum has been shown to produce butyric acid. This improves gut dysbiosis and increases commensal Bifidobacterium, which in turn has been shown to promote dendritic cell function and T-cell–directed antitumor immunity, and in a murine model, has demonstrated improved tumor-directed immunotherapy efficacy (52-54). They showed that patients who received probiotics concurrently with immunotherapy had longer PFS (median 250 days) compared to those without probiotics (median 101 days) (51). The probiotic subgroup also had a significantly longer OS (median not reached vs. 361 days) (51). Interestingly, in the same study, in patients who received antibiotics concurrently with immunotherapy (n=46), known to be associated with worse immunotherapy outcomes, the inclusion of Clostridium butyricum (22 of 46 antibiotic-treated patients) was associated with improved OS and PFS (51). This study also found a favorable improvement in OS and PFS with probiotic administration in patients receiving immunotherapy who received concomitant PPIs. However, another study has suggested that immunotherapy outcomes may be worsened by the co-administration of PPIs (n=25, PFS HR 0.52, OS HR 0.42) or by co-administration of both PPIs and antibiotics (n=11, PFS HR 0.42, OS HR 0.25) (55). Conversely, improvements in OS and PFS was also noted with the administration of a different probiotic, ginseng polysaccharides, in a rat model (56). The hypothesis originated from data on long term administration of ginseng as a dietary supplement showing increasing measures of Bifidobacterium, Allobaculum, Lactobacillus, Clostridium and Parasutterella (57). Specifically, the ginseng polysaccharides increased measures of Lactobacillus and Bacteroides and demonstrated was associated with activation of macrophages, T cells and natural killer cells (57).
Given these data are limited by pre-clinical or retrospective studies and small sample size, these data are hypothesis generating. Nonetheless, probiotics could be an excellent additive therapy to immunotherapy to counteract diminished efficacy due to exposure to antibiotics and PPIs. The optimal probiotic agent or cocktail and delivery mechanism and schedules need to be elucidated in prospective randomized control trials.
PPIs
PPIs have also been shown to alter gut microbiome (58,59). This has a broad reaching impact given the prevalence of PPIs in symptomatic management of treatment-related gastritis in patients with lung cancer. In this context, there have been several recent studies examining the association between PPIs and immunotherapy efficacy. A pooled post hoc analysis of the OAK and POPLAR trials examined 1,512 patients with platinum-resistant advanced NSCLC who were treated with atezolizumab or docetaxel. PPI use was associated with both lower OS (median 9.6 vs. 14.5 months) and PFS (median 1.9 vs. 2.8 months) in patients who received atezolizumab, but this deleterious effect was not seen in the docetaxel group (60). A similar post hoc analysis of IMpower150 studied 1,202 chemotherapy-naïve patients with metastatic non-squamous NSCLC that were randomized to atezolizumab plus carboplatin plus paclitaxel (ACP), bevacizumab plus carboplatin plus paclitaxel (BCP), or atezolizumab plus BCP (ABCP), of which 441 patients received PPIs. They reported significantly worse OS (HR 1.53) and PFS (HR 1.34) in the pooled atezolizumab group (ACP and ABCP), but not in the BCP group (61). Similarly, another study noted a higher all-cause mortality associated with PPI use in a cohort of 2,963 patients treated with immunotherapy after nth lines of therapy for advanced NSCLC. Concurrent PPI use was defined as PPI use within 30-day before, up to and including the date of immunotherapy start (62).
Conversely, retrospective data in smaller population samples of advanced NSCLC treated with anti-PD-1/PD-L1 based therapies failed to show a difference in PFS or OS based on concurrent PPI use (63,64). In one of the studies, there were several subgroups of treatment combinations, such as anti-PD-1 monotherapy (n=47), anti-PD-1 and chemotherapy (n=33), and anti-PD-1 and anti-angiogenic agent (n=19) (63). PFS and OS outcomes did not differ across the treatment subgroups in relation to concomitant PPI use. This was also the case in a retrospective cohort study of adult patients with metastatic NSCLC, melanoma, renal cell carcinoma (RCC), transitional cell carcinoma, or head and neck squamous cell carcinoma (n=233) treated with nivolumab or pembrolizumab therapy (65). Interestingly, these negative studies largely included patients treated with nivolumab and pembrolizumab (anti-PD1 monoclonal antibody) whereas the association of concomitant PPI use and worsened ICI outcomes reported in the OAK, POPLAR, and Impower 150 studies were with atezolizumab (anti-PD-L1 monoclonal antibody). Other limitations in cross-study comparisons include significant differences in study sample size (atezolizumab studies were much larger), study population, and study design.
Antibiotics
Antibiotic usage has an impact on immunotherapy outcomes. In a retrospective study of both renal cell carcinoma (RCC) (n=121) and NSCLC (n=239) patients receiving immunotherapy as nth line treatment at two institutions in Europe and USA, 20% and 13% in the RCC and NSCLC groups received antibiotics, with β-lactams being the most commonly administered class of antibiotics (82% in RCC and 32% in NSCLC) (66). In patients with NSCLC, concurrent antibiotic and immunotherapy usage was associated with decreased PFS (median 1.9 vs. 3.8months) and OS (median 7.9 vs. 24.6 months). Similar results were also reported in other retrospective studies which also identified β-lactams as the most frequently administered antibiotics (67). While there were some studies that did not identify this difference, they were limited by the number of antibiotic-treated subjects (68,69). Importantly, a recent prospective study of NSCLC (n=119), melanoma (n=38), and other tumor types (n=39) receiving ICI therapy sought to answer this question and showed that prior antibiotic treatment was associated with worse OS (median 2 vs. 26 months) and higher percentage of treatment-refractory participants (81% vs. 44%) (70). This effect was independent of tumor site, disease burden, and performance status.
While a single course of antibiotic exposure could lead to reduced OS as suggested in previous studies, there is data to suggest that the negative association with antibiotics may be related to cumulative exposure (i.e., dose dependent). A single-site retrospective study of patients with advanced melanoma (n=179), NSCLC (n=64), and RCC (n=48) who received “cumulative courses” antibiotics, defined by concurrent or successive antibiotics’ administration for >7 days reported significantly worse PFS (median 2.8 vs. 6.3 months) and OS (median 6.3 vs. 21.7 months) compared to those who received a single course of antibiotics (71). While prospective data is lacking here, there is more supportive retrospective data from a study of 157 patients with NSCLC that received immunotherapy (72). They were stratified based on early exposure to antibiotics (early immunotherapy period, EIOP; n=27), defined by antibiotic use 1 month before and up to 3 months after starting immunotherapy, and Antibiotic-Immunotherapy Exposure Ratio (AIER) defined by ratio between days on antibiotic and days on immunotherapy. When looking at the 46 patients who received antibiotics concurrent to immunotherapy, independent of time, median AIER was 4.2% and patients with a higher AIER had worse PFS and OS, independent of line of immunotherapy and performance (72). However, they showed no association between EIOP and PFS or OS, which is inconsistent with previously discussed literature on antibiotics decreasing ICI efficacy (72).
While there is a clear association between antibiotic use and immunotherapy efficacy, studies are suggesting that the degree of impact on the gut microbiome and immunotherapy efficacy may depend on the type of antibiotic used. One retrospective cohort study of 340 patients with NSCLC treated with immunotherapy not only showed that those who received antibiotics concurrently (38%) within 30 days prior to starting immunotherapy had a shorter OS than those without antibiotic exposure (median 266 vs. 455 days), it also showed that patients treated with fluoroquinolones had a shorter OS in comparison to patients receiving other broad-spectrum antibiotics (median 121 vs. 370 days) (73). On the other hand, the previously mentioned retrospective study that noted a worsened OS with β-lactams had a smaller sample size and was not powered to draw a conclusion (67).
Further elucidation of the relation of antimicrobial treatment, the host microbiome, and the immune response will require going beyond examining the dichotomous paradigm of antibiotics vs. no antibiotics. As emerging data have already suggested, dose and duration of antibiotic exposure, class or type of antibiotic, and the context of antibiotic exposure (host, disease, and treatment related factors) are key areas to expand upon. The future of clinical practice may require the incorporation of the gut-endocrine-metabolic-immune axis in addition to typical pharmacodynamic and pharmacokinetic properties of drugs when considering therapeutic options for patients.
Bowel movement patterns
In addition to identifying microbial taxa associated with clinical response to ICI, some studies have explored other biomarkers of immunotherapy response (28). For example, a small retrospective study of 40 patients examined the correlation between abnormal bowel movement patterns (n=20: patients with abnormal bowel movements and n=20: normal bowel movements) and ICI efficacy (74). Their study rationale was based on prior studies showing improved fecal microbiome diversity and composition associated with normal Bristol Stool Scale-based stool consistency and frequency (75,76). They defined abnormal stool patterns as: “(i) constipation condition according to Common Terminology Criteria for Adverse Events version 4.0 for more than three days in a week before and after ICI administration; or (ii) taking oral laxatives during ICI treatment (74).” Patients without stool abnormality had longer time to treatment failure and OS (median 204 vs. 31.5 days, 511 vs. 79 days, respectively) suggesting its use as a potential novel patient-based biomarker of immunotherapy efficacy (74). Once again, as with probiotics, further prospective data validating its use is required before its implementation into clinical settings.
Future directions
Our current knowledge on the microbiome gut-lung-immune axis suggests this is an important direction to pursue, from the pathophysiology to the clinical impact of modulating the gut microbiome on lung cancer treatments. Knowing the strong association of the microbiome on survival outcomes in NSCLC, it would be important to understand how factors such as geography, race, dietary pattern, past medical conditions, and gastrointestinal surgeries modify the microbiome, and how they may be responsible for both safeguarding against NSCLC, but also promoting it, depending on the clinical context. Furthermore, it would be helpful to evaluate, through randomized controlled trials (RCT), whether increasing butyrate-producing bacteria in the gut, for example, decreases the risk of developing NSCLC. Our narrative review also identified microbiota in organs other than the lower gastrointestinal tract that may be more prognostic for NSCLC outcomes. Careful study of the temporal and geographic relationship between host and tumor microbiome, cancer cells, and the immune system is warranted. Ultimately, rigorously designed RCTs of promising therapeutics such as fecal microbial transplant (35,36) and precision-nutrition strategies such as bespoke resistant-starch supplementation based on the target host’s gut microbiome (77,78), need to be conducted.
Another area highlighted in this review was the impact of antibiotics and other medications (e.g., PPI) on NSCLC prognosis and treatment, and this seems to be also related with a reduction in good commensal organisms and increased gut dysbiosis. Importantly, several studies have demonstrated a link between antibiotics, a significant change in gut microbiome composition, and decreasing immunotherapy efficacy. Certainly, this highlights the importance of considering the use of concomitant medications such as antibiotics, PPIs, and corticosteroids with lung cancer therapy, especially in the context of immunotherapy.
Lastly, readily measurable clinical symptoms and signs such as bowel movement patterns may be useful surrogate markers for immunotherapy efficacy. This was reported in a single study, but clinical factors such as stool transit time may be relevant in gut microbiome-related cancer treatment outcomes, and further study would be interesting. It may be worthwhile to collect bowel movement patterns (constipation and/or diarrhea) in future immunotherapy trials.
Conclusions
Emerging data support the importance of the gut microbiome and its impact on lung cancer and the efficacy of its treatments, especially ICI therapy. Studies show that factors such as antibiotics and PPIs reduce alpha diversity and alter the relative abundance of microbial genera or taxa in the host’s microbiome. The bidirectional alteration of gut microbiome and immunity supports the hypothesis that modulating the host microbiome may allow us to influence immunotherapy outcomes in NSCLC. This is additionally supported by data showing that probiotics improve some of the deleterious effects from antibiotics and PPIs, and that patient factors, such as abnormal characteristics of bowel movements, independently decrease OS on immunotherapy. Furthermore, susceptibility to changes in treatment outcomes as they relate to antibiotics and PPI exposure may depend on the immunotherapy agent used [anti-PD-L1 (atezolizumab) vs. anti-PD1 (pembrolizumab, nivolumab)]. Larger prospective studies are needed to confirm these observations and identify other important tumor, host, and extrinsic factors (i.e., medication exposure) that may impact immunotherapy efficacy.
Acknowledgments
Funding: None.
Footnote
Reporting Checklist: The authors have completed the Narrative Review reporting checklist. Available at https://tlcr.amegroups.com/article/view/10.21037/tlcr-22-595/rc
Peer Review File: Available at https://tlcr.amegroups.com/article/view/10.21037/tlcr-22-595/prf
Conflicts of Interest: Both authors have completed the ICMJE uniform disclosure form (available at https://tlcr.amegroups.com/article/view/10.21037/tlcr-22-595/coif). HS has received travel awards from Canadian Clinical Trials Group and Canadian Lung Cancer Conference. TLN has received academic research funds from sources without industry or commercial ties. In the past 3 years, TLN has participated in several Advisory boards with Novartis and Knight Therapeutics, has received payment for providing an educational talk to Takeda Canada staff about their product brigatinib, and has received a research grant from Takeda Oncology for an IIT in 2017. The authors have no other conflicts of interest to declare.
Ethical Statement: The authors are accountable for all aspects of the work in ensuring that questions related to the accuracy or integrity of any part of the work are appropriately investigated and resolved.
Open Access Statement: This is an Open Access article distributed in accordance with the Creative Commons Attribution-NonCommercial-NoDerivs 4.0 International License (CC BY-NC-ND 4.0), which permits the non-commercial replication and distribution of the article with the strict proviso that no changes or edits are made and the original work is properly cited (including links to both the formal publication through the relevant DOI and the license). See: https://creativecommons.org/licenses/by-nc-nd/4.0/.
References
- Canadian Cancer Society. Lung Cancer Statistics. Accessed on July 11, 2022. Available online: https://cancer.ca/en/cancer-information/cancer-types/lung/statistics
- Anand P, Kunnumakkara AB, Sundaram C, et al. Cancer is a preventable disease that requires major lifestyle changes. Pharm Res 2008;25:2097-116. [Crossref] [PubMed]
- National Comprehensive Cancer Network. Non-Small Cell Lung Cancer. Accessed on July 11, 2022. Available online: https://www.nccn.org/guidelines/category_1
- Nagasaka M, Sexton R, Alhasan R, et al. Gut microbiome and response to checkpoint inhibitors in non-small cell lung cancer-A review. Crit Rev Oncol Hematol 2020;145:102841. [Crossref] [PubMed]
- The Integrative Human Microbiome Project. Nature 2019;569:641-8. [Crossref] [PubMed]
- Huang YJ, Nelson CE, Brodie EL, et al. Airway microbiota and bronchial hyperresponsiveness in patients with suboptimally controlled asthma. J Allergy Clin Immunol 2011;127:372-381.e1-3.
- Marri PR, Stern DA, Wright AL, et al. Asthma-associated differences in microbial composition of induced sputum. J Allergy Clin Immunol 2013;131:346-52.e1-3.
- Millares L, Ferrari R, Gallego M, et al. Bronchial microbiome of severe COPD patients colonised by Pseudomonas aeruginosa. Eur J Clin Microbiol Infect Dis 2014;33:1101-11. [Crossref] [PubMed]
- Huang YJ, Sethi S, Murphy T, et al. Airway microbiome dynamics in exacerbations of chronic obstructive pulmonary disease. J Clin Microbiol 2014;52:2813-23. [Crossref] [PubMed]
- Qiu P, Ishimoto T, Fu L, et al. The Gut Microbiota in Inflammatory Bowel Disease. Front Cell Infect Microbiol 2022;12:733992. [Crossref] [PubMed]
- Vivarelli S, Salemi R, Candido S, et al. Gut Microbiota and Cancer: From Pathogenesis to Therapy. Cancers (Basel) 2019;11:38. [Crossref] [PubMed]
- Carabotti M, Scirocco A, Maselli MA, et al. The gut-brain axis: interactions between enteric microbiota, central and enteric nervous systems. Ann Gastroenterol 2015;28:203-9. [PubMed]
- Sandrini S, Aldriwesh M, Alruways M, et al. Microbial endocrinology: host-bacteria communication within the gut microbiome. J Endocrinol 2015;225:R21-34. [Crossref] [PubMed]
- Jan G, Belzacq AS, Haouzi D, et al. Propionibacteria induce apoptosis of colorectal carcinoma cells via short-chain fatty acids acting on mitochondria. Cell Death Differ 2002;9:179-88. [Crossref] [PubMed]
- Wei W, Sun W, Yu S, et al. Butyrate production from high-fiber diet protects against lymphoma tumor. Leuk Lymphoma 2016;57:2401-8. [Crossref] [PubMed]
- Bhatt AP, Redinbo MR, Bultman SJ. The role of the microbiome in cancer development and therapy. CA Cancer J Clin 2017;67:326-44. [Crossref] [PubMed]
- Paulos CM, Wrzesinski C, Kaiser A, et al. Microbial translocation augments the function of adoptively transferred self/tumor-specific CD8+ T cells via TLR4 signaling. J Clin Invest 2007;117:2197-204. [Crossref] [PubMed]
- Blaser MJ, Perez-Perez GI, Kleanthous H, et al. Infection with Helicobacter pylori strains possessing cagA is associated with an increased risk of developing adenocarcinoma of the stomach. Cancer Res 1995;55:2111-5. [PubMed]
- Hatakeyama M. Structure and function of Helicobacter pylori CagA, the first-identified bacterial protein involved in human cancer. Proc Jpn Acad Ser B Phys Biol Sci 2017;93:196-219. [Crossref] [PubMed]
- Halazonetis TD. Constitutively active DNA damage checkpoint pathways as the driving force for the high frequency of p53 mutations in human cancer. DNA Repair (Amst) 2004;3:1057-62. [Crossref] [PubMed]
- Yao Y, Dai W. Genomic Instability and Cancer. J Carcinog Mutagen 2014;5:1000165. [PubMed]
- Frisan T. Bacterial genotoxins: The long journey to the nucleus of mammalian cells. Biochim Biophys Acta 2016;1858:567-75. [Crossref] [PubMed]
- Bergounioux J, Elisee R, Prunier AL, et al. Calpain activation by the Shigella flexneri effector VirA regulates key steps in the formation and life of the bacterium's epithelial niche. Cell Host Microbe 2012;11:240-52. [Crossref] [PubMed]
- Zhang YJ, Li S, Gan RY, et al. Impacts of gut bacteria on human health and diseases. Int J Mol Sci 2015;16:7493-519. [Crossref] [PubMed]
- Vaishnava S, Behrendt CL, Ismail AS, et al. Paneth cells directly sense gut commensals and maintain homeostasis at the intestinal host-microbial interface. Proc Natl Acad Sci U S A 2008;105:20858-63. [Crossref] [PubMed]
- Belkaid Y, Naik S. Compartmentalized and systemic control of tissue immunity by commensals. Nat Immunol 2013;14:646-53. [Crossref] [PubMed]
- Lynch SV, Pedersen O. The Human Intestinal Microbiome in Health and Disease. N Engl J Med 2016;375:2369-79. [Crossref] [PubMed]
- Magnúsdóttir S, Ravcheev D, de Crécy-Lagard V, et al. Systematic genome assessment of B-vitamin biosynthesis suggests co-operation among gut microbes. Front Genet 2015;6:148. [Crossref] [PubMed]
- Lee CS, Ryan EJ, Doherty GA. Gastro-intestinal toxicity of chemotherapeutics in colorectal cancer: the role of inflammation. World J Gastroenterol 2014;20:3751-61. [Crossref] [PubMed]
- Jin Y, Dong H, Xia L, et al. The Diversity of Gut Microbiome is Associated With Favorable Responses to Anti-Programmed Death 1 Immunotherapy in Chinese Patients With NSCLC. J Thorac Oncol 2019;14:1378-89. [Crossref] [PubMed]
- Moss SF. The Clinical Evidence Linking Helicobacter pylori to Gastric Cancer. Cell Mol Gastroenterol Hepatol 2016;3:183-91. [Crossref] [PubMed]
- Gopalakrishnan V, Spencer CN, Nezi L, et al. Gut microbiome modulates response to anti-PD-1 immunotherapy in melanoma patients. Science 2018;359:97-103. [Crossref] [PubMed]
- Matson V, Fessler J, Bao R, et al. The commensal microbiome is associated with anti-PD-1 efficacy in metastatic melanoma patients. Science 2018;359:104-8. [Crossref] [PubMed]
- Vétizou M, Pitt JM, Daillère R, et al. Anticancer immunotherapy by CTLA-4 blockade relies on the gut microbiota. Science 2015;350:1079-84. [Crossref] [PubMed]
- Davar D, Dzutsev AK, McCulloch JA, et al. Fecal microbiota transplant overcomes resistance to anti-PD-1 therapy in melanoma patients. Science 2021;371:595-602. [Crossref] [PubMed]
- Baruch EN, Youngster I, Ben-Betzalel G, et al. Fecal microbiota transplant promotes response in immunotherapy-refractory melanoma patients. Science 2021;371:602-9. [Crossref] [PubMed]
- Hosgood HD 3rd, Sapkota AR, Rothman N, et al. The potential role of lung microbiota in lung cancer attributed to household coal burning exposures. Environ Mol Mutagen 2014;55:643-51. [Crossref] [PubMed]
- Laroumagne S, Salinas-Pineda A, Hermant C, et al. Incidence and characteristics of bronchial colonisation in patient with lung cancer: a retrospective study of 388 cases. Rev Mal Respir 2011;28:328-35. [Crossref] [PubMed]
- Zeng XT, Xia LY, Zhang YG, et al. Periodontal Disease and Incident Lung Cancer Risk: A Meta-Analysis of Cohort Studies. J Periodontol 2016;87:1158-64. [Crossref] [PubMed]
- Michaud DS, Kelsey KT, Papathanasiou E, et al. Periodontal disease and risk of all cancers among male never smokers: an updated analysis of the Health Professionals Follow-up Study. Ann Oncol 2016;27:941-7. [Crossref] [PubMed]
- Yan X, Yang M, Liu J, et al. Discovery and validation of potential bacterial biomarkers for lung cancer. Am J Cancer Res 2015;5:3111-22. [PubMed]
- Dumont-Leblond N, Veillette M, Racine C, et al. Non-small cell lung cancer microbiota characterization: Prevalence of enteric and potentially pathogenic bacteria in cancer tissues. PLoS One 2021;16:e0249832. [Crossref] [PubMed]
- Samuelson DR, Welsh DA, Shellito JE. Regulation of lung immunity and host defense by the intestinal microbiota. Front Microbiol 2015;6:1085. [Crossref] [PubMed]
- Velasquez-Manoff M. Gut microbiome: the peacekeepers. Nature 2015;518:S3-11. [Crossref] [PubMed]
- Gui Q, Li H, Wang A, et al. The association between gut butyrate-producing bacteria and non-small-cell lung cancer. J Clin Lab Anal 2020;34:e23318. [Crossref] [PubMed]
- Zhang WQ, Zhao SK, Luo JW, et al. Alterations of fecal bacterial communities in patients with lung cancer. Am J Transl Res 2018;10:3171-85. [PubMed]
- Hakozaki T, Richard C, Elkrief A, et al. The Gut Microbiome Associates with Immune Checkpoint Inhibition Outcomes in Patients with Advanced Non-Small Cell Lung Cancer. Cancer Immunol Res 2020;8:1243-50. [Crossref] [PubMed]
- Lu H, Gao NL, Tong F, et al. Alterations of the Human Lung and Gut Microbiomes in Non-Small Cell Lung Carcinomas and Distant Metastasis. Microbiol Spectr 2021;9:e0080221. [Crossref] [PubMed]
- Ouaknine Krief J, Helly de Tauriers P, Dumenil C, et al. Role of antibiotic use, plasma citrulline and blood microbiome in advanced non-small cell lung cancer patients treated with nivolumab. J Immunother Cancer 2019;7:176. [Crossref] [PubMed]
- Song P, Yang D, Wang H, et al. Relationship between intestinal flora structure and metabolite analysis and immunotherapy efficacy in Chinese NSCLC patients. Thorac Cancer 2020;11:1621-32. [Crossref] [PubMed]
- Tomita Y, Ikeda T, Sakata S, et al. Association of Probiotic Clostridium butyricum Therapy with Survival and Response to Immune Checkpoint Blockade in Patients with Lung Cancer. Cancer Immunol Res 2020;8:1236-42. [Crossref] [PubMed]
- Sivan A, Corrales L, Hubert N, et al. Commensal Bifidobacterium promotes antitumor immunity and facilitates anti-PD-L1 efficacy. Science 2015;350:1084-9. [Crossref] [PubMed]
- Takahashi M, Taguchi H, Yamaguchi H, et al. The effect of probiotic treatment with Clostridium butyricum on enterohemorrhagic Escherichia coli O157:H7 infection in mice. FEMS Immunol Med Microbiol 2004;41:219-26. [Crossref] [PubMed]
- Yasueda A, Mizushima T, Nezu R, et al. The effect of Clostridium butyricum MIYAIRI on the prevention of pouchitis and alteration of the microbiota profile in patients with ulcerative colitis. Surg Today 2016;46:939-49. [Crossref] [PubMed]
- Tomita Y, Goto Y, Sakata S, et al. Clostridium butyricum therapy restores the decreased efficacy of immune checkpoint blockade in lung cancer patients receiving proton pump inhibitors. Oncoimmunology 2022;11:2081010. [Crossref] [PubMed]
- Sun YF, Zhang X, Wang XY, et al. Effect of long-term intake of ginseng extracts on gut microbiota in rats. Zhongguo Zhong Yao Za Zhi 2018;43:3927-32. [PubMed]
- Sun Y. Structure and biological activities of the polysaccharides from the leaves, roots and fruits of Panax ginseng CA Meyer: an overview. Carbohydrate Polymers 2011;85:490-9. [Crossref]
- Imhann F, Bonder MJ, Vich Vila A, et al. Proton pump inhibitors affect the gut microbiome. Gut 2016;65:740-8. [Crossref] [PubMed]
- Jackson MA, Goodrich JK, Maxan ME, et al. Proton pump inhibitors alter the composition of the gut microbiota. Gut 2016;65:749-56. [Crossref] [PubMed]
- Chalabi M, Cardona A, Nagarkar DR, et al. Efficacy of chemotherapy and atezolizumab in patients with non-small-cell lung cancer receiving antibiotics and proton pump inhibitors: pooled post hoc analyses of the OAK and POPLAR trials. Ann Oncol 2020;31:525-31. [Crossref] [PubMed]
- Hopkins AM, Kichenadasse G, McKinnon RA, et al. Efficacy of first-line atezolizumab combination therapy in patients with non-small cell lung cancer receiving proton pump inhibitors: post hoc analysis of IMpower150. Br J Cancer 2022;126:42-7. [Crossref] [PubMed]
- Baek YH, Kang EJ, Hong S, et al. Survival outcomes of patients with nonsmall cell lung cancer concomitantly receiving proton pump inhibitors and immune checkpoint inhibitors. Int J Cancer 2022;150:1291-300. [Crossref] [PubMed]
- Zhao S, Gao G, Li W, et al. Antibiotics are associated with attenuated efficacy of anti-PD-1/PD-L1 therapies in Chinese patients with advanced non-small cell lung cancer. Lung Cancer 2019;130:10-7. [Crossref] [PubMed]
- Verschueren MV, van der Welle CMC, Tonn M, et al. The association between gut microbiome affecting concomitant medication and the effectiveness of immunotherapy in patients with stage IV NSCLC. Sci Rep 2021;11:23331. [Crossref] [PubMed]
- Peng K, Chen K, Teply BA, et al. Impact of Proton Pump Inhibitor Use on the Effectiveness of Immune Checkpoint Inhibitors in Advanced Cancer Patients. Ann Pharmacother 2022;56:377-86. [Crossref] [PubMed]
- Derosa L, Hellmann MD, Spaziano M, et al. Negative association of antibiotics on clinical activity of immune checkpoint inhibitors in patients with advanced renal cell and non-small-cell lung cancer. Ann Oncol 2018;29:1437-44. [Crossref] [PubMed]
- Ochi N, Ichihara E, Takigawa N, et al. The effects of antibiotics on the efficacy of immune checkpoint inhibitors in patients with non-small-cell lung cancer differ based on PD-L1 expression. Eur J Cancer 2021;149:73-81. [Crossref] [PubMed]
- Hamada K, Yoshimura K, Hirasawa Y, et al. Antibiotic Usage Reduced Overall Survival by over 70% in Non-small Cell Lung Cancer Patients on Anti-PD-1 Immunotherapy. Anticancer Res 2021;41:4985-93. [Crossref] [PubMed]
- Nyein AF, Bari S, Hogue S, et al. Effect of prior antibiotic or chemotherapy treatment on immunotherapy response in non-small cell lung cancer. BMC Cancer 2022;22:101. [Crossref] [PubMed]
- Pinato DJ, Howlett S, Ottaviani D, et al. Association of Prior Antibiotic Treatment With Survival and Response to Immune Checkpoint Inhibitor Therapy in Patients With Cancer. JAMA Oncol 2019;5:1774-8. [Crossref] [PubMed]
- Tinsley N, Zhou C, Tan G, et al. Cumulative Antibiotic Use Significantly Decreases Efficacy of Checkpoint Inhibitors in Patients with Advanced Cancer. Oncologist 2020;25:55-63. [Crossref] [PubMed]
- Galli G, Triulzi T, Proto C, et al. Association between antibiotic-immunotherapy exposure ratio and outcome in metastatic non small cell lung cancer. Lung Cancer 2019;132:72-8. [Crossref] [PubMed]
- Lu PH, Tsai TC, Chang JW, et al. Association of prior fluoroquinolone treatment with survival outcomes of immune checkpoint inhibitors in Asia. J Clin Pharm Ther 2021;46:408-14. [Crossref] [PubMed]
- Katayama Y, Yamada T, Tanimura K, et al. Impact of bowel movement condition on immune checkpoint inhibitor efficacy in patients with advanced non-small cell lung cancer. Thorac Cancer 2019;10:526-32. [Crossref] [PubMed]
- Vandeputte D, Falony G, Vieira-Silva S, et al. Stool consistency is strongly associated with gut microbiota richness and composition, enterotypes and bacterial growth rates. Gut 2016;65:57-62. [Crossref] [PubMed]
- Hadizadeh F, Walter S, Belheouane M, et al. Stool frequency is associated with gut microbiota composition. Gut 2017;66:559-60. [Crossref] [PubMed]
- Sobh M, Montroy J, Daham Z, et al. Tolerability and SCFA production after resistant starch supplementation in humans: a systematic review of randomized controlled studies. Am J Clin Nutr 2022;115:608-18. [Crossref] [PubMed]
- Dobranowski PA, Stintzi A. Resistant starch, microbiome, and precision modulation. Gut Microbes 2021;13:1926842. [Crossref] [PubMed]