The radioprotectant nano-genistein enhances radiotherapy efficacy of lung tumors in mice
Highlight box
Key findings
• In these preclinical studies using a NSCLC mouse xenograft model, nano-genistein was demonstrated to be radioprotective of normal lung tissue but not radioprotective of tumors. Nano-genistein appeared to also improve the efficacy of radiotherapy by sensitizing tumors.
• Nano-genistein was also shown to be safe following continuous oral dosing for 10–20 weeks.
What is known and what is new?
• Radiotherapy for NSCLC is often dose limiting due to therapy-related toxicities.
• In this study, we evaluated the therapeutic utility of radioprotectant, nano-genistein, for NSCLC by demonstrating that it is radioprotectant of lung tissue, but not tumors.
What is the implication, and what should change now?
• The positive safety and efficacy results, along with previous preclinical investigations of nano-genistein, provided the rationale to evaluate nano-genistein as a radioprotectant for patients with NSCLC undergoing chemoradiotherapy in a multicenter phase 1b/2a trial (NCT02567799).
Introduction
Lung cancer affects nearly a quarter million people annually in the United States and accounts for more cancer-related deaths than any other cancer type (1). Over 85% of lung cancers are non-small cell lung cancer (NSCLC) (2). NSCLC treatment regimens are multi-modal and commonly involve surgical resection, chemotherapy and/or immunotherapy, and radiotherapy. Despite recent advances in radiation treatment technologies, damage to the surrounding normal tissue during NSCLC radiotherapy remains a common cause of dose-limiting toxicities (3,4). A significant adverse effect caused by NSCLC radiotherapy is radiation-induced damage to the normal lung tissue, manifesting as acute or subacute pneumonitis and late pulmonary fibrosis (5). These can result in respiratory declines and overall reduced quality of life following treatment. It is, therefore, necessary to evaluate treatment strategies that may mitigate the toxicities-associated with radiotherapy, without hindering the efficacy of the treatment.
Genistein oral nanosuspension (nano-genistein) is a promising radioprotectant and radiomitigator that has demonstrated efficacy in mitigating radiation-induced lung damage in mouse models (6). The nano-genistein formulation consists of synthetic genistein nanoparticles formulated into an aqueous suspension suitable for oral self-administration. Nano-genistein mitigates radiation-induced tissue damage via genistein’s activity as a highly selective estrogen receptor beta (ERb) agonist, subsequently regulating gene expression and cell signaling to promote cell survival and anti-inflammatory effects (7-9). No data to date assessed differential radioprotection of normal tissues versus tumor, and the clinical utility of nano-genistein would be diminished if the radioprotective properties also extend to tumors. Therefore, we evaluated nano-genistein in a mouse xenograft model of NSCLC. Here, we present proof-of-concept preclinical studies confirming that nano-genistein does not protect a lung adenocarcinoma in mice from acute thoracic or abdominal radiation. This manuscript is written following the ARRIVE reporting checklist (available at https://tlcr.amegroups.com/article/view/10.21037/tlcr-22-856/rc).
Methods
Animals
Experiments were performed under Institutional Animal Care & Use Committee (IACUC) with Protocol No. 1222 granted by the Henry Ford Health System Research Administration, in compliance with national and institutional guidelines for the care and use of animals. All mice were housed and managed by Association for Assessment and Accreditation of Laboratory Animal Care (AAALAC)-approved animal facilities at Henry Ford Health System (Detroit, MI, USA). Female athymic CD1 nu/nu mice (Charles River Laboratories, Wilmington, MA, USA), 6–7 weeks old, were housed four per cage and provided food/water ad libitum. Mouse chow was phytoestrogen-free (not containing soy), Teklad 2914 rodent chow (Harlan Laboratories, Haslett, MI, USA). Rooms were maintained with a 12-hour light/12-hour dark cycle (on 5 am, off 5 pm) and kept at 72±2 ℉ with 40%±5% relative humidity. Treatment groups started with N=12 animals/group.
Nano-genistein and vehicle for animal studies
Nano-genistein (BIO 300 Oral Suspension, Humanetics Corporation, Minneapolis, MN, USA) is a wet-milled nanosuspension containing 325 mg/mL synthetic genistein with a d(50) particle-size distribution of less than 0.2 µm, 5% Povidone K17 (weight/weight; w/w), 0.2% Polysorbate 80 (w/w) in 50 mM phosphate buffered saline (61 mM sodium chloride). The control article (“vehicle”) was nano-genistein without the active ingredient, synthetic genistein.
Nano-genistein was diluted with vehicle such that mice received either 200 mg/kg in volumes ranging from 0.07–0.10 mL or 400 mg/kg in volumes ranging from 0.14–0.20 mL, depending on animal weight. Drug dilutions were prepared weekly. Nano-genistein or vehicle were administered by oral gavage daily using 20-gauge × 1.5 inch oral animal feeding cannula (Cadence, Inc., Cranston, RI, USA). Individual animal dosing was determined based on the weekly average body weight of each experimental group.
Murine xenograft studies
Study 1—thoracic irradiation model
In the first study, human A549 adenocarcinoma lung cancer (NSCLC) xenografts were implanted subcutaneously in mice dorsally within the upper torso, and the radiation field encompassed both the tumor xenograft and lung. For tumor cell implantation, 2×106 A549 cells were mixed 1:1 in Matrigel (100 mL total) and injected into each tumor site. Tumor volumes were recorded twice weekly by a researcher blinded to treatment groups using external measurements of the tumor dimensions. External measurements were used to calculate the tumor volume using the formula for the volume of an ellipse [(4/3)pR1•R2•R3] where R1, R2, and R3 are the orthogonal radii of the tumor. This calculation was based on a modification and extension of a previously used method (10) and similar to other published ellipsoid formulas used to calculate tumor volumes (11). The third dimension (R3) was estimated from an average of R1 and R2 [R3 = (R2 + R1)/2] in order to avoid bias from choosing the smaller or larger of the two dimensions. Animals with ulcerated tumors were euthanized and excluded from the study from the time of ulceration. Tumor ulceration occurred unpredictably and independently of the tumor size. In addition, following tumor initiation the animals were randomized into treatment cohorts such that each cohort had a similar mean tumor volume and standard deviation.
Radiation was delivered once the mean tumor volume for each treatment group reached 400 mm3. Thoracic irradiation was delivered using opposed lateral-beam geometry at a dose rate of 2.7 Gy/min. The radiation exposure conformed to a 2 cm wide field along the length of the mouse centered on the lung using a commercial collimator. A single 12.5 Gy radiation dose was delivered to anesthetized mice (60 mg/kg ketamine and 6 mg/kg xylazine, i.p.) using a 5000 Ci 137Cs small animal irradiator (Mark I, J. L. Shepherd, San Fernando, CA, USA). Mice were irradiated in groups of 8. Radiation uniformity was maintained by delivering half the dose with the left side of the mouse closest to the source and half the dose with the right side of the mouse closest to the source. Nano-genistein or vehicle treatment was administered 1 h (±5 min) prior to radiation exposure. A single 12.5 Gy dose was chosen based on previous studies that indicated this dose can cause detectable lung tissue damage in addition to delaying tumor growth without completely ablating the tumor (6,12).
The 12.5 Gy dose accuracy was confirmed by measuring the radiation dose from the 137Cs irradiator using 1 mm3 LiF microcube dosimeters (thermo-luminescent dosimeters; TLDs) and Gafchromic radiation-sensitive film and comparing the dose to that from a calibrated clinical 6 MV linear particle accelerator (LINAC). For the 137Cs irradiator, this dose corresponds to an exposure time of approximately 4.68 min. The dosimetry analysis confirmed the desired dose; film exposed to the 137Cs source at 12.5 Gy had an optical density within 1% of film exposed to the same dose from a calibrated 6 MV clinical irradiator. The dose from the 137Cs irradiator measured using TLDs were ±3% of that from the calibrated 6 MV clinical irradiator.
On the day of irradiation, mice were first administered their respective nano-genistein or vehicle treatment and then irradiated 1 h (±5 min) after dosing. At the time of irradiation, there was N=10–12 animals/group due to tumor ulcerations. In this first study, nano-genistein dosing started 7 days prior to A549 tumor cell implantation and continued for 10 weeks (5 days/week). The primary outcome measure was tumor growth. Body weights were collected twice weekly throughout the study. Lung, skin adjacent to the tumor, uterus, esophagus and tumor tissues were collected from animals at euthanasia and embedded in paraffin. Tissue samples from 5 animals/group were hematoxylin and eosin (H&E) stained and submitted to Consulting Tox/Path, LLC for independent histopathological review. Severity of microscopic findings in the tissues was graded on a 5-point Likert scale, and the distribution of the finding (focal, multifocal, diffuse) was reported.
Study 2—abdominal irradiation model
In this study, A549 cells were implanted subcutaneously in the dorsal rear flank to facilitate animal handling. Similar to the above experiment, 2×106 A549 cells were mixed 1:1 in Matrigel (100 mL total) and injected into each tumor site. Tumor volumes were recorded twice weekly, animals with ulcerated tumors were handled as described above and animals were randomized into treatment groups so that each group had a similar mean tumor volume and standard deviation.
The delivered radiation field for this model was termed “abdominal radiation” because it was a 2 cm field that encompassed the subcutaneously implanted flank tumor, gastrointestinal (GI) tract and uterus. As described above, a single 12.5 Gy dose was delivered using the same irradiator, radiation geometry, and assessment of radiation accuracy. At the time of irradiation, there was N=9–12 animals/group due to tumor ulcerations. Mice received one of two treatment schedules for nano-genistein or vehicle dosing, (I) animals were dosed starting after A549 implantation when tumors reached a mean volume of 75 mm3 or (II) animals were dosed starting 7 days prior to abdominal irradiation (tumors reached approximately 400 mm3 on the day of irradiation). Nano-genistein dosing continued for 20 or 16 weeks, respectively (7 days/week). Body weights were collected twice weekly throughout the study.
As above in experiment 1, tumor growth was the primary outcome measure and body weights were collected twice weekly. To investigate the protective effects of nano-genistein, the skin directly above the tumor and the terminal ileum were collected at euthanasia and were submitted for independent histopathological review (Consulting Tox/Path, LLC). For these analyses, 3 skin and 3 terminal ileum tissue samples were H&E stained from each treatment group that received nano-genistein or vehicle starting at the time tumors reached 75 mm3. Severity of findings in the tissues was graded on a 5-point Likert scale as described above.
Statistical analysis
For the xenograft studies, sample sizes were based on previously published studies in a similar model evaluating a related genistein product (10). Individual animal tumor volumes were compared relative to the tumor volume on the day of irradiation. Similarly, individual animal body weights were compared relative to the animal body weight on the day of tumor implantation. In order to avoid the limitations of statistically testing each individual timepoint in these studies, such as changes in sample size due to animal attrition, a mixed-effects analysis of variance (ANOVA), also known as a repeated measures ANOVA, was used. The mixed-effects ANOVA compared the changes in mean relative tumor volume or mean relative body weight over time between treatment groups. Survival was evaluated by Kaplan-Meier curves. Animals that were euthanized due to tumor ulcerations were censored for survival analyses. Kaplan-Meier curves were compared by log-rank test. All statistical analyses were completed using GraphPad Prism 9 (Dotmatics, Boston, MA, USA), and statistical significance was defined as P<0.05.
Results
Tumor growth
In the first study, mice received thoracic irradiation approximately 5–6 weeks after tumor implantation. At the time of irradiation, tumors were 392±248 mm3 (vehicle), 429±245 mm3 (nano-genistein, 200 mg/kg), and 440±316 mm3 (nano-genistein, 400 mg/kg). Individual animal tumor volumes were normalized to the volume on the day of thoracic irradiation and plotted by treatment group versus days post-irradiation (Figure 1A,1B). Statistical comparisons are provided in Table 1. Nano-genistein (200 or 400 mg/kg/day) as a monotherapy had no effect on tumor growth (Figure 1A,1B; blue symbols). Radiation alone, or in combination with nano-genistein (200 mg/kg/day), had a similar inhibitory effect on tumor growth (Figure 1A). Radiation in combination with 400 mg/kg/day nano-genistein improved the efficacy of radiation compared to radiation alone (P=0.0025; Figure 1B; Table 1). Survival analysis during the BIO 300 dosing period post-irradiation shows minimal differences between the treatment groups (Figure 1C,1D). The lone exception are the animals that received nano-genistein (400 mg/kg/day) alone without radiation which have worse survival compared to the irradiated, nano-genistein (400 mg/kg/day) treated animals (log-rank test, P=0.021; Figure 1D). However, the animals that received nano-genistein (400 mg/kg/day) alone without radiation did not have significantly worse survival than the unirradiated vehicle-treated animals (log-rank test, P=0.084).
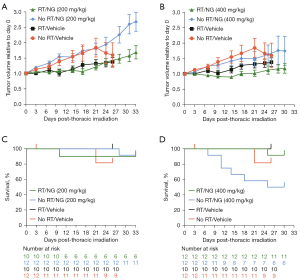
Table 1
Study | Radiation | Comparator 1 | Comparator 2 | P value |
---|---|---|---|---|
1 | Thoracic | No RT/vehicle | 12.5 Gy RT/vehicle | 0.2201 |
1 | Thoracic | No RT/vehicle | No RT/NG [200 mg/kg/day] | 0.9385 |
No RT/vehicle | 12.5 Gy RT/NG [200 mg/kg/day] | 0.0295 | ||
RT/vehicle | No RT/NG [200 mg/kg/day] | 0.0008 | ||
RT/vehicle | 12.5 Gy RT/NG [200 mg/kg/day] | 0.0560 | ||
1 | Thoracic | No RT/vehicle | No RT/NG [400 mg/kg/day] | 0.7878 |
No RT/vehicle | 12.5 Gy RT/NG [400 mg/kg/day] | <0.0001 | ||
RT/vehicle | No RT/NG [400 mg/kg/day] | 0.2215 | ||
RT/vehicle | 12.5 Gy RT/NG [400 mg/kg/day] | 0.0025 | ||
2 | Abdominal | No RT/vehicle | 12.5 Gy RT/vehicle | <0.0001 |
2 | Abdominal | No RT/vehicle | 12.5 Gy RT/NG [200 mg/kg/day] | <0.0001 |
No RT/vehicle | 12.5 Gy RT/NG [200 mg/kg/day], −7 d | <0.0001 | ||
RT/vehicle | 12.5 Gy RT/NG [200 mg/kg/day] | <0.0001 | ||
RT/vehicle | 12.5 Gy RT/NG [200 mg/kg/day], −7 d | <0.0001 | ||
2 | Abdominal | No RT/vehicle | No RT/NG [400 mg/kg/day] | 0.9999 |
No RT/vehicle | 12.5 Gy RT/NG [400 mg/kg/day] | <0.0001 | ||
No RT/vehicle | 12.5 Gy RT/NG [400 mg/kg/day], −7 d | <0.0001 | ||
RT/vehicle | No RT/NG [400 mg/kg/day] | 0.0021 | ||
RT/vehicle | 12.5 Gy RT/NG [400 mg/kg/day] | 0.0013 | ||
RT/vehicle | 12.5 Gy RT/NG [400 mg/kg/day], −7 d | 0.2155 |
All analyses were conducted using mixed-effects ANOVA. RT, radiation; NG, nano-genistein; −7 d, 7 days prior to irradiation; ANOVA, analysis of variance.
In the second study, all treatment groups received abdominal irradiation 31 days after the tumor volume reached 75 mm3. The mean ± standard error of the mean (SEM) tumor volume across all study arms at the time of irradiation was 470±295 mm3, and individual animal tumor volumes were normalized as described above. Nano-genistein treatment, in combination with radiation, at 200 mg/kg starting 24 h after tumor establishment (75 mm3) or 7 days prior to irradiation significantly reduced tumor growth relative to radiation alone (Figure 2A, Table 1). Similar to the first study, nano-genistein (400 mg/kg) treatment alone did not impact tumor growth compared to vehicle treatment (P=0.9999; Figure 2B, Table 1). Nano-genistein treatment, in combination with radiation, at 400 mg/kg starting after tumor establishment synergized with radiation to significantly reduce tumor growth (Figure 2B, Table 1). Survival analysis reveal no significant differences between treatment groups and their respective controls (Figure 2C,2D).
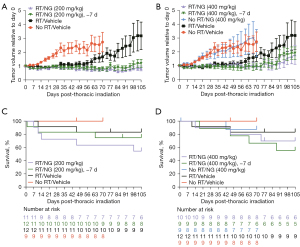
Changes in body weight
In the second study involving abdominal irradiation, the field of radiation included the GI tract, and thus changes in body weight were also assessed. We found that both dose levels and both dose schedules of nano-genistein resulted in significant improvements in mean body weight over time compared to irradiated animals treated with vehicle alone (Figure 3A,3B). In the first xenograft study, significant weight loss was not anticipated due to the radiation field location; however, there was an apparent decrease in mean body weight in each group following thoracic irradiation (Figure 3C). While all treatment groups had statistically different changes in mean body weight over time relative to vehicle (P<0.05), it appears that animals treated with 400 mg/kg maintained body weight better than vehicle treated animals in both studies.
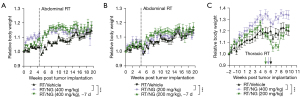
Histopathology
In study 1 where animals received thoracic irradiation, all vehicle- and nano-genistein-treated animals displayed evidence of increased alveolar septal wall thickness with increased collagen, with the exception of the groups that received sham irradiation. The lungs of animals that received thoracic irradiation and vehicle treatment displayed clear signs of multifocal or diffuse congestion, alveolar macrophage infiltration, thickening of the septal wall, alveolar fibrin, pneumocyte hypertrophy, and overall reduction in alveolar space (Figure 4, Table 2). In contrast, animals that received nano-genistein (200 or 400 mg/kg/day) had markedly improved lung histology (Figure 4). The major finding in these animals was minimal thickening of the septal wall, and any instances of macrophage infiltration were minimal to mild and focal in distribution (Table 2). From a safety perspective, the uterus, esophagus, and skin of the sham-irradiated animals were also examined. Microscopic observations of the skin included minimal mixed cellular or neutrophilic inflammation and decreased or increased epidermal thickness, and in the esophageal lumen there was bacteria present in all groups. These findings were considered to be incidental with no relationship to any particular treatment group. Findings in tumor tissue included cystic areas of degeneration and necrosis with or without inflammation in all animals (Table S1). These findings were also not related to any particular treatment group. Importantly, no treatment-associated adverse effects were observed following 10-week of nano-genistein dosing for either the 200 or the 400 mg/kg/day groups.
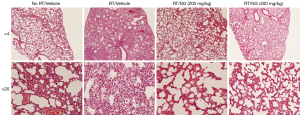
Table 2
Lung microscopic observations and time of euthanasia | Treatment group | ||||||||||||||||||||||||||||||||||
---|---|---|---|---|---|---|---|---|---|---|---|---|---|---|---|---|---|---|---|---|---|---|---|---|---|---|---|---|---|---|---|---|---|---|---|
No RT/vehicle | 12.5 Gy RT/vehicle | No RT/NG (200 mg/kg) | 12.5 Gy RT/NG (200 mg/kg) | No RT/NG (400 mg/kg) | 12.5 Gy RT/NG (400 mg/kg) | ||||||||||||||||||||||||||||||
Euthanasia (weeks post-RT) | 5.4 | 2.5 | 2.9 | 4.4 | 3.7 | 8.0 | 11.4 | 6.0 | 8.7 | 8.7 | 4.7 | 4.7 | 4.7 | 7.0 | 9.0 | 1.9 | 7.0 | 9.7 | 1.9 | 9.0 | 1.9 | 1.9 | 3.4 | 4.7 | 2.7 | 3.1 | 5.3 | 5.3 | 5.3 | 5.3 | |||||
Alveolar hemorrhage | 1M | 1M | |||||||||||||||||||||||||||||||||
Alveolar edema | 2M | 1M | |||||||||||||||||||||||||||||||||
Alveolar fibrin | 1M | ||||||||||||||||||||||||||||||||||
Congestion | 1D | 1D | 1D | 1D | 1D | ||||||||||||||||||||||||||||||
Increased alveolar macrophages | 1M | 3D | 2F | 1F | 1M | 1M | 1M | ||||||||||||||||||||||||||||
Increased septal thickness | 2D | 3D | 1M | 1D | 2D | 1D | 1M | 2M | 1D | 1D | 1M | 1M | 1M | 1D | 2M | ||||||||||||||||||||
Mixed cellular infiltrate | 1M | ||||||||||||||||||||||||||||||||||
Pneumocyte hyperplasia | 3M | ||||||||||||||||||||||||||||||||||
Unremarkable (no findings) | 0 | 0 | 0 | 0 | 0 | 0 | 0 | 0 | 0 | 0 | 0 | 0 | 0 |
Each column represents an individual animal. Severity: 0, normal; 1, minimal; 2, mild; 3, moderate. Distribution: F, focal; M, multifocal; D, diffuse. RT, radiation; NG, nano-genistein.
In the second study, histopathology was limited to the skin and terminal ileum from animals that received nano-genistein or vehicle starting when tumors reached 75 mm3. Histopathology review concluded that there were no nano-genistein related effects of the skin adjacent to the tumor or the terminal ileum (Table S2, Figure S1). Minimal to mild and multifocal to diffuse amyloid-like material was observed in the lamina propria of the ileum in all mice in all groups, but otherwise it was normal in appearance. Amyloid deposition is a common finding in various organs, especially the ileum, in mice and may be related to age and/or inflammation.
Discussion
The soy isoflavone, genistein, has been studied for decades as a potential therapeutic for various cancers and inflammatory diseases, such as asthma, because of its anti-inflammatory, antioxidant and radioprotective properties (13-15). Despite its intriguing biological activity, genistein has yet to be translated into use as a clinical therapeutic. This is in large part due to its poor water solubility and oral bioavailability (16). In animal studies, genistein is often solubilized in polyethylene glycol (PEG) 400 to improve oral bioavailability, but this is not a feasible option for human dosing given the strong laxative properties of PEG 400. To overcome the inherently poor oral pharmacokinetic properties of genistein, a proprietary process for nanomilling synthetic genistein was developed to produce a genistein nanosuspension suitable for oral administration (nano-genistein) (6,17). This formulation has a reduced average particle size of approximately 100-fold compared to standard genistein, allowing for improved bioavailability.
On the molecular level, genistein has been shown to regulate proteins such as NF-kB, IL-1b, COX2, p53, and p21 (9,18-20). These effects can be attributed to genistein’s activation of ERb which promote anti-inflammatory effects, DNA damage response, and cell cycle regulation (21-23). Radiation-induced pneumonitis is caused by damage to the alveolar epithelium and vascular endothelium resulting in the release of pro-inflammatory and pro-fibrotic cytokines (5). Oxidative stress, DNA damage and cytokine release leads to hypoxia, pulmonary edema and an increase in lung density. If the injury is not managed, it can progress to pulmonary fibrosis. Genistein has the potential to mitigate the development of these pathologies by counteracting oxidative stress and reducing inflammation. Further, the differential function and expression of DNA damage response proteins (e.g., p53) and pro-inflammatory pathways (e.g., NF-kB), likely allow genistein to prevent or mitigate radiation damage of normal tissues without protecting tumors. Genistein has even been shown to have anti-tumor effects, including synergizing with radiation to kill cancer cells (20,24-26). Nano-genistein was also shown to inhibit growth of prostate cancer tumors alone or in combination with radiation in mouse xenograft models (27).
The present study demonstrated that daily nano-genistein doses of 200–400 mg/kg did not protect lung tumors from radiation treatment; on the contrary, significant radiosensitization was observed when tumors were grown in the flank (a location that allowed tumors to grow for several months). Nano-genistein was also shown to protect the normal lung tissues at both dose levels (200 and 400 mg/kg/day), as evidenced by the reduction in pulmonary congestion, inflammation and collagen deposition. However, future studies will need to be carried out for a longer period in order to better evaluate the drug’s effect on lung fibrosis, which can take up to 6 months to develop in mice (28). Importantly, nano-genistein was well-tolerated by animals in both studies, and no toxic effects were observed in animals at nano-genistein doses up to 400 mg/kg/day administered up to 7 days/week for 20 weeks. The mortality observed in the thoracic irradiation study in unirradiated animals that received 400 mg/kg/day nano-genistein was not considered treatment-related since the results were not reproduced in unirradiated animals that received the same dose, but for a longer duration, in the abdominal irradiation study. This supports the safety of extended continuous daily dosing of nano-genistein. Animals treated with nano-genistein appeared to better maintain body weight following irradiation, particularly in the second xenograft study where the radiation field included the GI tract. Translationally, a >5% weight loss can result in worse clinical outcomes for patients with NSCLC treated with concurrent chemoradiotherapy (29).
Limitations to this study are the use of a single cell line, a single fixed radiation dose instead of fractionated radiation, and the use of a single stain (H&E) to assess histological tissue damage. Future studies with a syngeneic mouse model (e.g., LLC1 cells) should be considered. Studies in using animals with an intact immune system will be important in evaluating the drugs mechanism. Stains such as Masson’s trichrome stain for collagen should also be considered. Additionally, there are several caveats in translating these findings to the clinic, including starting nano-genistein dosing prior to or immediately following tumor implantation and only exposing animals to a single dose of radiation when NSCLC is clinically treated with fractionated radiotherapy. However, previous preclinical studies demonstrated that nano-genistein protects lung tissue from radiation exposure (6) and showed that nano-genistein can also sensitize multiple prostate xenografts to radiation-induced tumor killing (27).
Conclusions
In conclusion, this study demonstrated that daily oral administration of nano-genistein is safe and not radioprotective of NSCLC tumors. Nano-genistein also sensitized tumors to acute radiation exposure and promoted stable body weight following irradiation. Taken together, this body of evidence supports the continued investigation of nano-genistein as a radioprotectant candidate for use during radiotherapy for patients with NSCLC, and these findings served as the basis of a multicenter phase 1b/2a trial of nano-genistein with concurrent chemoradiation for locally advanced NSCLC (NCT02567799).
Acknowledgments
The authors thank John Zenk, MD for his role in study conception and interpretation. John Zenk, MD passed away prior to the publication of this article. The authors also thank the veterinary pathology expertise provided by Roger E. Wells, DVM, MS, DACVP, Toxicologic Pathologist at Consulting Tox/Path, LLC, and Melissa Ingram, PhD, for providing the initial draft of the manuscript.
Funding: Research reported in this study was supported by the National Cancer Institute Small Business Innovation Research award (No. HHSN261201200078C). The content is solely the responsibility of the authors and does not necessarily represent the official views of the National Cancer Institute.
Footnote
Reporting Checklist: The authors have completed the ARRIVE reporting checklist. Available at https://tlcr.amegroups.com/article/view/10.21037/tlcr-22-856/rc
Data Sharing Statement: Available at https://tlcr.amegroups.com/article/view/10.21037/tlcr-22-856/dss
Peer Review File: Available at https://tlcr.amegroups.com/article/view/10.21037/tlcr-22-856/prf
Conflicts of Interest: All authors have completed the ICMJE uniform disclosure form (available at https://tlcr.amegroups.com/article/view/10.21037/tlcr-22-856/coif). CBS serves as an unpaid editorial board member of Translational Lung Cancer Research from July 2017 to June 2024. MDK, AAS and BM are current employees of Humanetics Corporation and inventors on 4 Humanetics Patents and 3 Humanetics PCT applications. The research presented in this manuscript was financially support by SBIR grant (No. HHSN261201200078C), of which MDK was the PI on the award. MDK and AAS are supported by 5 additional grants that were awarded to Humanetics Corporation; however, none of these grants supported, including financially, the research presented in this manuscript. BM currently receives payments to support research unrelated to this manuscript from 3 other companies. BM was formerly the President of American Radium Society, Inc., and this role is unrelated to the current manuscript. SLB received funding support from the NIH grant that supported the studies in the manuscript and receives royalty payments from Humanetics Corporation for intellectual property unrelated to the manuscript. The other authors have no conflicts of interest to declare.
Ethical Statement: The authors are accountable for all aspects of the work in ensuring that questions related to the accuracy or integrity of any part of the work are appropriately investigated and resolved. Animal experiments were performed under Institutional Animal Care & Use Committee (IACUC) with Protocol No. 1222 granted by the Henry Ford Health System Research Administration, in compliance with national and institutional guidelines for the care and use of animals.
Open Access Statement: This is an Open Access article distributed in accordance with the Creative Commons Attribution-NonCommercial-NoDerivs 4.0 International License (CC BY-NC-ND 4.0), which permits the non-commercial replication and distribution of the article with the strict proviso that no changes or edits are made and the original work is properly cited (including links to both the formal publication through the relevant DOI and the license). See: https://creativecommons.org/licenses/by-nc-nd/4.0/.
References
-
. Available online: https://seer.cancer.gov/statistics/interactive.htmlNational Cancer Institute Surveillance, Epidemiology and End Results Program - Miller KD, Siegel RL, Lin CC, et al. Cancer treatment and survivorship statistics, 2016. CA Cancer J Clin 2016;66:271-89. [Crossref] [PubMed]
- Simone CB 2nd. Thoracic Radiation Normal Tissue Injury. Semin Radiat Oncol 2017;27:370-7. [Crossref] [PubMed]
- Verma V, Simone CB 2nd, Werner-Wasik M. Acute and Late Toxicities of Concurrent Chemoradiotherapy for Locally-Advanced Non-Small Cell Lung Cancer. Cancers (Basel) 2017;9:120. [Crossref] [PubMed]
- Yan Y, Fu J, Kowalchuk RO, et al. Exploration of radiation-induced lung injury, from mechanism to treatment: a narrative review. Transl Lung Cancer Res 2022;11:307-22. [Crossref] [PubMed]
- Jackson IL, Zodda A, Gurung G, et al. BIO 300, a nanosuspension of genistein, mitigates pneumonitis/fibrosis following high-dose radiation exposure in the C57L/J murine model. Br J Pharmacol 2017;174:4738-50. [Crossref] [PubMed]
- Landauer MR, Harvey AJ, Kaytor MD, et al. Mechanism and therapeutic window of a genistein nanosuspension to protect against hematopoietic-acute radiation syndrome. J Radiat Res 2019;60:308-17. [Crossref] [PubMed]
- Jones JW, Jackson IL, Vujaskovic Z, et al. Targeted Metabolomics Identifies Pharmacodynamic Biomarkers for BIO 300 Mitigation of Radiation-Induced Lung Injury. Pharm Res 2017;34:2698-709. [Crossref] [PubMed]
- Ha CT, Li XH, Fu D, et al. Genistein nanoparticles protect mouse hematopoietic system and prevent proinflammatory factors after gamma irradiation. Radiat Res 2013;180:316-25. [Crossref] [PubMed]
- Gallo D, Zannoni GF, De Stefano I, et al. Soy phytochemicals decrease nonsmall cell lung cancer growth in female athymic mice. J Nutr 2008;138:1360-4. [Crossref] [PubMed]
- Rodallec A, Vaghi C, Ciccolini J, et al. Tumor growth monitoring in breast cancer xenografts: A good technique for a strong ethic. PLoS One 2022;17:e0274886. [Crossref] [PubMed]
- Storozhuk Y, Sanli T, Hopmans SN, et al. Chronic modulation of AMP-Kinase, Akt and mTOR pathways by ionizing radiation in human lung cancer xenografts. Radiat Oncol 2012;7:71. [Crossref] [PubMed]
- Goh YX, Jalil J, Lam KW, et al. Genistein: A Review on its Anti-Inflammatory Properties. Front Pharmacol 2022;13:820969. [Crossref] [PubMed]
- Rasheed S, Rehman K, Shahid M, et al. Therapeutic potentials of genistein: New insights and perspectives. J Food Biochem 2022;46:e14228. [Crossref] [PubMed]
- Singh VK, Seed TM. BIO 300: a promising radiation countermeasure under advanced development for acute radiation syndrome and the delayed effects of acute radiation exposure. Expert Opin Investig Drugs 2020;29:429-41. [Crossref] [PubMed]
- Yang Z, Kulkarni K, Zhu W, et al. Bioavailability and pharmacokinetics of genistein: mechanistic studies on its ADME. Anticancer Agents Med Chem 2012;12:1264-80. [Crossref] [PubMed]
- Salem AM, Jackson IL, Gibbs A, et al. Interspecies Comparison and Radiation Effect on Pharmacokinetics of BIO 300, a Nanosuspension of Genistein, after Different Routes of Administration in Mice and Non-Human Primates. Radiat Res 2022;197:447-58. [Crossref] [PubMed]
- Zhu Q, Zhang W, Mu D, et al. Effects of genistein on lipopolysaccharide-induced injury of mouse alveolar epithelial cells and its mechanism. Biosci Biotechnol Biochem 2020;84:544-51. [Crossref] [PubMed]
- Zhang Z, Wang CZ, Du GJ, et al. Genistein induces G2/M cell cycle arrest and apoptosis via ATM/p53-dependent pathway in human colon cancer cells. Int J Oncol 2013;43:289-96. [Crossref] [PubMed]
- Jiang H, Fan J, Cheng L, et al. The anticancer activity of genistein is increased in estrogen receptor beta 1-positive breast cancer cells. Onco Targets Ther 2018;11:8153-63. [Crossref] [PubMed]
- Sareddy GR, Li X, Liu J, et al. Selective Estrogen Receptor β Agonist LY500307 as a Novel Therapeutic Agent for Glioblastoma. Sci Rep 2016;6:24185. [Crossref] [PubMed]
- Gong P, Madak-Erdogan Z, Li J, et al. Transcriptomic analysis identifies gene networks regulated by estrogen receptor α (ERα) and ERβ that control distinct effects of different botanical estrogens. Nucl Recept Signal 2014;12:e001. [Crossref] [PubMed]
- Warner M, Huang B, Gustafsson JA. Estrogen Receptor β as a Pharmaceutical Target. Trends Pharmacol Sci 2017;38:92-9. [Crossref] [PubMed]
- Zhang Z, Jin F, Lian X, et al. Genistein promotes ionizing radiation-induced cell death by reducing cytoplasmic Bcl-xL levels in non-small cell lung cancer. Sci Rep 2018;8:328. [Crossref] [PubMed]
- Yan H, Jiang J, Du A, et al. Genistein Enhances Radiosensitivity of Human Hepatocellular Carcinoma Cells by Inducing G2/M Arrest and Apoptosis. Radiat Res 2020;193:286-300. [Crossref] [PubMed]
- Liu X, Wang Q, Liu B, et al. Genistein inhibits radiation-induced invasion and migration of glioblastoma cells by blocking the DNA-PKcs/Akt2/Rac1 signaling pathway. Radiother Oncol 2021;155:93-104. [Crossref] [PubMed]
- Jackson IL, Pavlovic R, Alexander AA, et al. BIO 300, a Nanosuspension of Genistein, Mitigates Radiation-Induced Erectile Dysfunction and Sensitizes Human Prostate Cancer Xenografts to Radiation Therapy. Int J Radiat Oncol Biol Phys 2019;105:400-9. [Crossref] [PubMed]
- Bertho A, Dos Santos M, Braga-Cohen S, et al. Preclinical Model of Stereotactic Ablative Lung Irradiation Using Arc Delivery in the Mouse: Is Fractionation Worthwhile? Front Med (Lausanne) 2021;8:794324. [Crossref] [PubMed]
- Kim YJ, Song C, Eom KY, et al. Combined Chemoradiotherapy-induced Weight Loss Decreases Survival in Locally Advanced Non-small Cell Lung Cancer Patients. In Vivo 2019;33:955-61. [Crossref] [PubMed]