Targeting complement C5a to improve radiotherapy sensitivity in non-small cell lung cancer
Highlight box
Key findings
• RT was shown to promote the release of C5a from tumor cells and led to up-regulation of C5aR1 expression via the AKT/NF-κB pathway. Inhibition of the combination of complement C5a and C5aR could improve RT sensitivity.
What is known and what is new?
• Recent studies have identified synergistic combinations of C5aR signal blockade with chemotherapy, checkpoint inhibitors and other antitumor therapy.
• We found that C5a produced by tumor cells could combine with C5aR1 to lead to the generation of radioresistance.
What is the implication, and what should change now?
• Our work provides evidence that the combination of RT and C5aR blockade represents a new window of opportunity to promote an anti-tumor therapeutic effect in NSCLC. C5aR1 inhibitors might be effective sensitizers for RT in the clinic. Conclusions based on animal experiments needed to be validated by further clinical studies.
Introduction
Lung cancer represents a leading cause of cancer-associated deaths globally, with high rates of morbidity and mortality (1). It is estimated that 2 million new cases of lung cancer are diagnosed annually with approximately 1.76 million deaths globally (2). Radiotherapy (RT) is one of the most critical modalities for the treatment of all stages of lung cancer (3). Despite the significant progress in radiation technology, the overall prognosis of RT for lung cancer remains far from satisfactory (4). Tumor local and distant relapse after RT is one of the critical contributors to poor prognosis. Numerous studies have demonstrated that many cancer patients develop radioresistance in a period of treatment, which can be induced by intrinsic and extrinsic factors (5,6). The antitumor effects of RT are dependent upon the participation of innate and adaptive components of the immune system (7,8). RT can induce immunosuppression by depleting immunocompetent cells that are within irradiated volume and by upregulating the expression of programmed cell death ligand 1 (PD-L1) to generate resistance to the immune system (9,10). Radioresistance is associated with the modulation of immune responses (4). RT could destroy radiosensitive immune cells and keep radioresistant immune cells alive, thereby altering the proportion of immune cells in the tumor microenvironment (TME), thus contributing to immunosuppressive status (11). RT develops immunosuppressive effect to enhance the removal depletion of CD8+ T cells to induce radioresistance (10). Lymphocyte dysfunction can be related to the inferior prognosis and weaker anti-tumor immune response in patients receiving RT (12). In addition, RT can stimulate the recruitment of polymorphonuclear neutrophils (PMNs) to the TME and neutrophils form extracellular traps (NETs) to promote tumor metastasis, which is thought to induce radioresistance (13). The change of immune status in pro- and anti-tumor immune cells can also affect RT sensitivity (14). LncRNA KCNQ1OT1 has been clarified as a therapeutic target to improve RT sensitivity (15). It is becoming increasingly clear that reversing tumor resistance to RT can dramatically improve the efficacy of tumor-targeting RT. However, the underlying molecular mechanisms of radioresistance have remained elusive and developing an effective strategy to overcome radioresistance has continued to be a significant challenge.
The complement system is a major constituent of innate immunity and bridge between innate and adaptive immunity, which takes part in immune defense, immune surveillance, and homeostasis (16,17). Complement can mainly be activated by 3 distinct pathways, namely, the lectin pathway (LP), the classical pathway (CP), and the alternative pathway (AP) (18). Mounting evidence suggests that complement activation in the TME of many types of cancers has been implicated in tumor growth, recurrence, and metastasis (16,19,20). As a complement activation product, C5a is closely connected with tumor progression, serving a regulatory role in the TME (21-23). C5aR1 (CD88) is the specific receptor of C5a, expressing on the surface of various cell membranes (24). C5a interacts with C5aR1 to promote M2 (pro-tumorigenic) polarization of tumor-associated macrophages (TAMs) and influence the recruitment of myeloid-derived suppressor cells (MDSCs) to stimulus exhaustion of CD8+ T cells (25,26). Thus, the depletion of complement might reprogram an immunosuppressive TME to impede tumor progression (27). In murine models, it has been demonstrated that complement activated by chemotherapy and anti-programmed cell death protein 1 (PD-1)/PD-L1 antibodies induces tumor-mediated immunosuppression in a C5a-dependent manner (26,28). However, little is known about the relationship between C5a/C5aR axis and RT.
RT may release negative regulatory factors to construct an immunosuppressive microenvironment. It has been shown that complement can be released by various immune cells (29). Both innate and adaptive immune components participate in the antitumor activity of RT (7). In cancer therapy, it appears necessary for avoiding tumor-immune escape during the generation of CD8+ T cell cross-priming (30). RT has been understood to promote the positive regulatory factor high mobility group protein B1 (HMGB-1) to promote anti-tumor immunity (31). However, it has remained unclear whether C5a, as a negative regulator that leads to inhibitory TME in tumors, is associated with RT resistance. It has been reported that combination RT with anti C1 treatment significantly prolonged survival in glioblastoma mouse models (32). Up to now, the role of the C5a/C5aR1 axis in cancer radioresistance and relevant regulatory mechanisms have not been investigated. In recent years, studies have investigated the release of complement from tumor cells, but little attention has been given to the correlation between complement release and RT. Thus, given the function of complement C5a in tumor progress, exploring the changes in the TME induced by RT-mediated complement activation may provide a novel opinion on the factors restricting antitumor effects of RT.
The main goal of this research was exploring the changes and mechanism in the TME induced by RT-mediated complement activation. It is anticipated that better understanding of such mechanisms may provide a novel perspective and multiple therapeutic targets for reversing radioresistance. We present this article in accordance with the ARRIVE reporting checklist (available at https://tlcr.amegroups.com/article/view/10.21037/tlcr-23-258/rc).
Methods
Mice
We purchased 6-week-age female C57BL/6J wild-type (WT) mice from Vital River Company (Beijing, China). All mice were housed under specific-pathogen-free conditions at the animal facility of The First Affiliated Hospital of Shandong First Medical University & Shandong Provincial Qianfoshan Hospital. All animal care and protocols were approved by the Institutional Animal Care and Use Committee of The First Affiliated Hospital of Shandong First Medical University & Shandong Provincial Qianfoshan Hospital (No. 2022-S303), in compliance with the Guide for the Care and Use of Laboratory Animals published by the US National Institutes of Health (NIH; Bethesda, MD, USA; publication No. 96-01). A protocol was prepared before the study without registration.
Cell culture
Lewis lung carcinoma (LLC) tumor cells (mouse lung cancer cells) were cultured in Dulbecco’s modified Eagle medium [DMEM; Biological Industries (BI), Kibbutz Beit Haemek, Israel] containing 10% heat-inactivated fetal bovine serum (FBS; BI, Israel) plus 1% penicillin and streptomycin (BI, Israel) at 37 ℃ with 5% CO2. The cells were routinely examined to be mycoplasma free. LLC cells were purchased from Boster Company (Pleasanton, CA, USA; catalog No. CX0056).
In vivo experiment models
To explore the antitumor effects of RT and blockade of C5aR signaling, 1×106 LLC cells were subcutaneously injected into the right hindlimb of experimental mouse. The animals were randomly entered into vivo experiments when the longest diameter of tumors reached 6–8 mm (n=4 or 5 per group, randomly). On days 14, 16, and 18, local RT with a dose of 8 Gy was performed using a RS 2000 pro Biological X-ray Irradiator (Rad Source Technologies, Buford, GA, USA). The total radiation dose was, thus, 24 Gy. Mice were anesthetized with 3 µL/g 10% chloral hydrate prior to RT. For blockade of C5aR signaling, the inhibitor of C5aR (W-54011; MedChemExpress, Monmouth Junction, NJ, USA; catalog No. HY-16992A), which had been resuspended in dimethyl sulfoxide (DMSO) and then diluted in normal saline, was intraperitoneally injected at 5.0 mg/kg/day on days 15, 17, 19, 21, and 23 post tumor radiation. Blinded experiments were not used in this study. Tumor sizes were measured 3 times a week by digital caliper in length and width and the tumor volume was calculated using the formula V (mm3) = length × width2/2. Mice were assessed daily and were weighted twice a week to ensure good health. Mice that lost more than 20% of body weight and become moribund were euthanized. All mice were euthanized by injection of chloral hydrate and tumors and spleens were resected, weighed, and processed for RNA, proteins, flow cytometry, histology, and immunohistochemistry (IHC).
In vitro experiment models
LLC cells were grown overnight in 6-well culture plates (2×105 per well) and separately irradiated 0, 4, and 8 Gy using RS 2000pro Biological X-ray Irradiator (Rad Source). At 24 and 48 hours later, the supernatant was discarded, and the corresponding cells were collected for further quantitative real-time polymerase chain reaction (qRT-PCR).
qRT-PCR
Total messenger RNA (mRNA) was extracted from irradiation-treated cells, freshly pulverized tumor and spleen using FastPure Cell/Tissue Total RNA Isolation Kit V2 (Vazyme, Nanjing, China; catalog No. RC 112-01). Then, we used HiScript III RT SuperMix for qPCR (+gDNA wiper) (Vazyme; catalog No. R323-01) to synthesize complementary DNA (cDNA) according to the manufacturer’s protocol. The RT-qPCR sequences of each gene primers were as follows: C5a, forward: 5'-GGGAAGCTGCTGATGAGAAT-3', reverse: 5'-CTTTCACCAGGTTGGCATTG-3'; C5aR, forward: 5'-GTGTCCCTGGCCTACATCAA-3', reverse: 5'-AGGAGTCGTCCATGGAAACC-3'; tumour necrosis factor alpha (TNF-α), forward: 5'-CATGCGTCCAGCTGACTAAA-3', reverse: 5'-TCCCCTTCATCTTCCTCCTT-3'; interleukin-6 (IL-6), forward: 5'-CCACTTCACAAGTCGGAGGCTTA-3', reverse: 5'-TGCAAGTGCATCATCGTTGTTC-3'; IL-12, forward: 5'-GTGAACCTCACCTGTGACACGC-3', reverse: 5'-TGAATACTTCTCATAGTCCCTTTGG-3'; glyceraldehyde 3-phosphate dehydrogenase (GAPDH), forward: 5'-GGTGCCTGTCGTTGTGTTC-3', reverse: 5'-GCTCCTTCTGGTGCTGTTG-3'; β-actin, forward: 5'-GGCTGTATTCCCCTCCATCG-3', reverse: 5'-CCAGTTGGTAACAATGCCATGT-3'. Relative gene expressions were normalized against β-actin and GAPDH and calculated by 2−ΔΔCt method (33).
Western blotting
Protein extraction from mouse tumor tissues were performed following standard protocols. The protein concentrations were estimated by the BCA Protein Assay Kit (Solarbio, Beijing, China; catalog No. PC0020) to ensure equal amounts of protein were subjected to immunoblotting. Samples were resolved on 10% sodium dodecyl sulfate (SDS) polyacrylamide gradient gel, transferred onto a polyvinylidene difluoride (PVDF) membrane (Solarbio) and then incubated with the primary and secondary antibodies. The following primary antibodies were used: anti-C5aR (Abcam, Cambridge, MA, USA; 1:500), anti-AKT (Proteintech, Rosemont, IL, USA; 1:1,000), p-AKT (Proteintech; 1:500), IκBα [Cell Signaling Technology, Danvers, MA, USA (CST); 1:1,000], p-IκBα (CST; 1:1,000), β-actin (Boster; 1:1,000). Finally, an enhanced chemiluminescence (ECL) reagent (Boster, catalog No. AR1173) was used to detect labeled protein bands. The bank densities were quantified by ImageJ software (version 1.62; NIH, USA).
Flow cytometry
Tumor tissues and spleens with or without RT were removed on day 3 after tumor radiation. Spleens were pulverized with a tissue homogenizer. Tumors were manually minced with scissors, digested with collagenase IV (1 mg/mL, Solarbio, catalog No. C8160) and deoxyribonuclease (DNase) I (0.2 mg/mL, Solarbio, catalog No. D8071) at 37 ℃ for 30 minutes, then passed through 70-µm cell nylon mesh to acquire single-cell suspensions. Single cells prepared as above were centrifuged at 3,500 rpm for 5 minutes, followed by erythrocyte lysis with RBC lysis buffer (BioGems, Westlake Village, CA, USA) to react at room temperature for 15 minutes under strict exclusion of light. After centrifugation at 500 g for 5 minutes, the supernatant was discarded and the cells were resuspended in sample diluent. Following that, lymphocytes were obtained by mouse visceral organ tissues lymphocyte separation solution kit (TBD, Beijing, China; catalog No. LTS1092P) for further analysis according to the manufacturer’s protocols.
In order to detect C5aR expression and proportions of cytotoxic T lymphocytes (CTLs; CD8+) on day 3 after RT, cells were harvested and treated with phycoerythrin (PE) anti-mouse CD88 (C5aR) antibody (BioLegend, San Diego, Ca, USA) and fluorescein isothiocyanate (FITC) anti-mouse CD8 antibody (BioLegend) for 30 minutes at 4 ℃. Cells were then resuspended in phosphate-buffered saline [PBS; 0.05% bovine serum albumin (BSA)] and fixed in 4% paraformaldehyde fixative (Solarbio). All samples were assayed by FACSAria III Cell Sorter [Becton, Dickinson, and Co. (BD) Biosciences, Franklin Lakes, NJ, USA] and data acquired was performed with FlowJo Software (Tree Star, Ashland, OR, USA).
Cell sorting
Single-cell suspensions from tumor tissue were obtained as described earlier. CD8+ CTLs were isolated by magnetic bead cell sorting using mouse CD8a positive selection kit II Protocal (EasySepTM, catalog No. 18953) according to the manufacturer’s instructions. TRIzol reagent (Invitrogen, Carlsbad, CA, USA) was added to the obtained cells, which were then stored at −80 ℃ until further processing.
Histology and IHC staining
For histology, tumor tissues were fixed in 4% formalin, embedded in paraffin, sliced into 4-µm sections and stained with hematoxylin and eosin (H&E).
For IHC staining, after a set of processes including dewaxing, rehydration, antigen retrieval with citrate buffer pH 6.0, endogenous peroxidase blocking with 3% H2O2 for 10 minutes, followed by incubating with a primary anti-C5 antibody (Affinity, Amherst, NH, USA; 1:50) overnight at 4 ℃ and then with a secondary antibody (Zhongshan Goldenbridge Biotechnology Company, Beijing, China; catalog No. SP-9001) for 30 minutes at 37 ℃. Immunodetection of the slides was performed by 3,3'-diaminobenzidine (DAB). All images were captured with an Olympus LCX100 Imaging System (Olympus, Tokyo, Japan). Results were analyzed with ImageJ software (version 1.62; NIH).
Immunofluorescence (IF) staining
Paraffin-embedded tissues were prepared at 4 µm thickness. After processes of dewaxing, rehydration, and antigen retrieval with ethylenediaminetetraacetic acid (EDTA) antigen retrieval buffer (pH 8.0) (Servicebio, Wuhan, China; G1206), the slides were then blocked with blocking solution [PBS with 10% fetal calf serum (FCS) and 0.1% TritonX] at room temperature for 1 hour, then washed in PBS and incubated overnight at 4 ºC in the appropriate primary anti-C5aR antibody (Abcam, 1:500) and anti-CD8a antibody (Boster, 1:50) diluted in blocking solution. Sections were then washed with PBS and incubated for 1 hour at room temperature with secondary antibody, treated with 4',6-diamidino-2-phenylindole (DAPI) (Servicebio, catalog No. G1012) to stain cell nuclei and add spontaneous fluorescence quenching reagent (Servicebio, catalog No. G1221). Images were acquired by laser confocal scanning microscope system (Eclipse C1; Nikon, Tokyo, Japan).
Enzyme-linked immunosorbent assay (ELISA)
According to the statistical significance, a mouse complement C5a ELISA Kit (Boster, catalog No. EK0987) was used to measure the level of mouse C5a in blood and tumor tissue homogenates. Mouse blood was left to clot on ice for 30 minutes and was subsequently centrifuged at 1,000 g at 4 ℃ for 15 minutes to obtain the serum. Tumor tissue homogenates and serum were further centrifuged again at 12,000 rpm and were stored at −80 ℃ for analysis. The sensitivity of this assay was <1 pg/mL. All procedures were performed according to the manufacturer’s instructions.
Statistical analysis
No statistical methods were used to predetermine the size of the samples. Statistical analysis was performed with GraphPad Prism 8.0 (GraphPad Software, San Diego, CA, USA) and SPSS statistical software 26.0 (IBM Corp., Armonk, NY, USA). Unpaired Student’s t-tests and one-way analysis of variance (ANOVA) were used to evaluate P values. Data were performed as the mean ± standard deviation (SD). Statistical significance was indicated when P<0.05.
Results
RT induced inflammatory and immune pathways and upregulated C5aR1 expression in CD8+ T cells
We investigated the recruitment of tumor specific CTLs cells in irradiated tumors. It was found that the number of recruited CD8+ T cells reached a peak at 60 hours after RT (34). A picture showing the experimental procedures is presented in Figure 1A. Tumor sizes in the RT-treated mice were smaller than those of the control group (Figure 1B). HE staining revealed that the therapeutic effect of RT was accompanied by increased T cells in the tumor space (data not shown). This phenomenon was further confirmed in IF staining and flow cytometry (Figure 1C,1D). The infiltration of recruited CD8+ T cells was increased after treatment of RT.
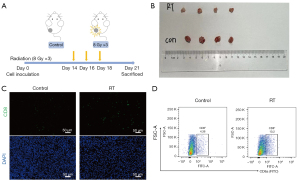
For further exploration, we applied RNA-seq analysis to screen differentially expressed genes (DEGs) in RT-recruited CD8+ T cells (Figure 2A). RNA-esq analysis exhibited that 444 genes were upregulated and 69 genes were downregulated in RT groups compared to control groups (Figure 2B,2C). Then, Gene Ontology (GO) enrichment analysis of the upregulated DEGs in RT-treated CD8+ T cells found that they were mainly enriched in cellular inflammatory and immune pathways, such as leukocyte migration, myeloid leukocyte migration, cell chemotaxis, neutrophil migration, positive regulation of response to external stimulus, and positive regulation of cytokine production (Figure 2D). An important issue for our finding was that these functional pathways exist C5aR1 gene enrichment. It was also found that expression of the C5aR1 gene in CD8+ T cells increased significantly after RT (Figure 2E). Thus, we guessed that expression of C5aR1 on CD8+ T cells mediated both inflammatory and immune responses to RT. These results implied that RT upregulated the expression of C5aR1 on CD8+ T cells to mediate anti-tumor immunity.
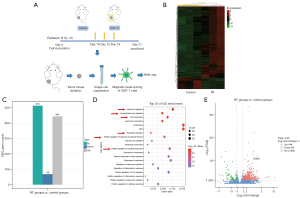
Blocking C5aR signaling in combination with RT can produce synergistic effect in anti-tumor efficacy
Next, we tested whether C5aR1 was an important component in our RT system. We assumed that blocking C5aR signaling would improve response to RT by modulating the TME (i.e., CD8+ T cells). In order to test this hypothesis, we established an LLC mice model treated with RT alone (8 Gy ×3 times, once every other day), C5aR1 inhibitor (W-54011) alone (5.0 mg/kg/day post RT, once every other day), and RT plus C5aR1 inhibitor (W-54011) (Figure 3A). Each group maintained the same weight in the mice (Figure 3B). The representative images of tumors from mice of all groups collected on day 24 are displayed in Figure 3C. Compared with the control group, RT alone (P<0.0001) and W-54011 alone (P=0.0004) had significant impacts on tumor growth, but there was no significant difference between these 2 groups. Notably, the rate of tumor growth in the RT+W-54011 group mice was significantly impeded when compared with RT alone (P=0.0114) and W54011 alone (P=0.0114) (Figure 3D,3E). The combined treatment also led to a significant efficacy in tumor weight (Figure 3F).
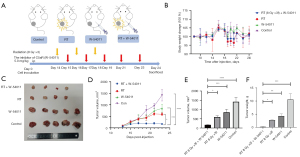
RT promotes the release of C5a on tumor cells and leads to up-regulation of C5aR expression
Considering that loss of C5aR1 potently promoted sensitivity of RT, we next explored the concrete mechanism of RT to C5aR expression in CD8+ T cells. Han et al. suggested that C5a stimulation significantly upregulates the expression of C5aR1 gene in mouse γδT cells (35). Cancer cells have been shown to be capable of releasing C5a generally cleaved from C5 to combine with C5aR and promote cancer invasiveness, which usually occurs at the inflammatory phase of acute inflammation (36).
In the in vivo experiment, C5a and C5aR mRNA expression was found in tumor tissues by qRT-PCR, and was further verified by IHC, IF, and flow cytometry. We profiled the levels of C5a in tumor tissue homogenates and blood from mice with or without RT treatment. Data showed that RT remarkably increased local C5a levels in TME (Figure 4A). Next, RT-qPCR and IHC staining were performed on tumor tissues undergoing RT. The results of RT-qPCR suggested that significant upregulation had occurred in the RT-treated group (Figure 4B), whereas, we did not find any change in C5a and C5aR expression in the spleen (Figure 4C). Abundant deposition of C5 in RT-treated mice was detected in the cytoplasm rather than on the cell membrane compared with the control group (Figure 4D). The significant increases in complement cleavage (C5a) products revealed that RT induced comprehensive complement activation. The expression of C5aR was significantly higher in the RT group (Figure 4E) and high infiltration of C5aR+ cells in TME were seen (Figure 4F). However, when we conducted the same analysis in spleen tissues, no significant differences were observed (Figure 4F). In order to evaluate the response of tumor cells to RT, an in vitro experiment was conducted to detect C5a expression of LLC cells underlying different time points 24 and 48 hours after delivery of RT (4 and 8 Gy radiation). We observed that the mRNA levels of C5a were increased at 24 hours after 4 Gy radiation (Figure 4G).
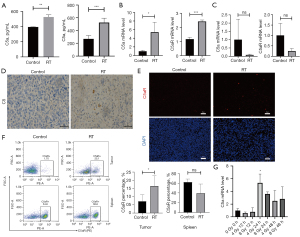
Together, these results indicated that the activation of C5a/C5aR pathway mediated by RT was occurred in TME, which was attributed by tumor cells and infiltrating immune cells.
RT may upregulate C5aR through the AKT/NF-κB pathway
As RT induced substantial production of C5a, it was important to discover the signaling pathway that induced the release of C5a on tumor cells. The role of nuclear factor κB (NF-κB) activation in regulation of C5a in carcinoma-associated fibroblasts (CAFs) had already been characterized (37). Interestingly, a study had shown that the activation of the PI3K/AKT pathway might be a latent mechanism of therapeutic resistance of small cell lung cancer (SCLC) (38). Consequently, we hypothesized that RT might mediate overexpression of C5a and C5aR via activation of oncogenic drivers.
Notably, the expressions of C5aR, p-AKT, and p-IκBα were upregulated in RT groups, yet that of IκBα was decreased (Figure 5A,5B). NF-κB remained inactive through binding to inhibitor κB (IκB) protein. As the “classical” activation of NF-κB, phosphorylation initiates ubiquitination of IκB proteins, thereby resulting in NF-κB translocation to the nucleus for transcription (39). The results of western blotting suggested that sustained NF-kB activation was maintained via IκB phosphorylation. In addition, we found that RT promoted the release of serious of pro-inflammatory factors such as TNF-α (P=0.043), IL-6 (P=0.042), and IL-12 (P=0.018) (Figure 5C), which were all downstream of the NF-κB signaling pathway (40,41). These results revealed that RT might upregulate C5a and C5aR expression via the AKT/NF-κB pathway. Therefore, we concluded that C5a/C5aR1 signaling regulated the antitumor effect of RT through the AKT/NF-κB pathway (Figure 6).
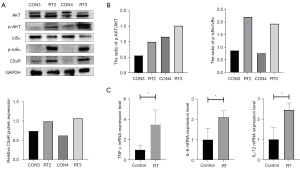
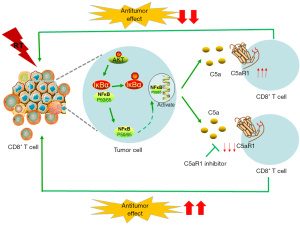
Discussion
Fractionated RT might lead to adaptive alterations in the TME to confine the intensity and durability of the anti-tumor immunotherapy (42). Radioresistance limits the efficacy of RT, which has been attributed to microenvironment alteration or acquired resistance (43). Adequate adaptive immunity has been identified as a key component of antitumor immune response (44). Understanding patterns of immunomodulatory effects of RT would be useful for designing novel combinational therapeutic protocols. In the present study, we found that RT induced the increased infiltration of CD8+ T cells and local activation of complement C5a/C5aR. Concurrent administration of RT and blocking of C5aR improved radiosensitivity and tumor-specific immune response, which was related with highly C5aR expression in CD8+ T cells. The AKT/NF-κB pathway is an important signaling pathway in C5a/C5aR axis mediated by RT.
The complement system plays a role in the immune system and represents the link between innate and adaptive immunity. Complement C5a has been linked to poorer cancer prognosis and advanced tumor stage (45). In both CP and AP of complement activation, C3 deficiency would be unable to support proteolytic cleavage of C5 to C5a. However, C5a might not be produced in the tumors via C3 activation (26). Fractionated RT has been shown to improve local complement activation (46,47), which is in line with our observation that tumor cells produced C5a and upregulated C5aR1 upon RT. In our study, we observed an increase in C5aR1 expression on CD8+ T cells after RT by RNA sequencing (RNA-seq), so we hypothesized that RT may promote the release of C5a to regulate anti-tumor immunity, and inhibition of this process can promote the sensitivity of RT.
Mounting evidence suggests that RT induces the release of C3a and C5a in immune and tumor cells, with a contribution of dendritic cell (DC) activation and obvious infiltration of CD8+ T-cells (46). Interestingly, some studies have found that elevated levels of C5a could recruit more MDSCs in the spleen to tumors, followed with interfering with the antitumor CD8+ T-cells response (48,49). It has been suggested that C5a probably triggers the formation of neutrophil extracellular traps (NETs) by MDSCs to promote metastasis and cancer progression (50). Studies have also revealed that C5a stimulates neutrophils to produce reactive oxygen species, which is involved in oxidative damage and clearance of T lymphocytes in numerous cancers (51). Furthermore, Medler et al. observed that PMX-53, a C5aR1 inhibitor, promoted anti-tumor efficacy of chemotherapy associated with affecting MDSCs in the TME and reversing the depletion state of CD8+ T cells (26). The activation of complement C5a could prevent side effects of chemotherapy (52). In fact, numerous studies have identified synergistic combinations of blocking with C5aR signal with chemotherapy, checkpoint inhibitors, and other antitumor therapies (25,53). The predominant effect of C5aR1 blockade on antitumor affect appears to agree with an observation from our research. In the preclinical LLC models of our study, anti-C5aR also showed the effect of reverse radioresistance. The specific mechanism by which complement mediates radiosensitivity needed to be further explored.
Many studies have indicated that complement component might downregulate immunosuppressive cell populations to impair T-cell trafficking. Some novel ideals were highlighted in our investigation. Firstly, tumor infiltrating CD8+ T cells had already been clarified to express complement receptors C5aR (54). Next, based on our above finding, it was shown that that C5aR1 expression in CD8+ T cells in the TME, but not in the spleen, was highly inversely correlated with antitumor effect in RT-treated mice. In fact, complement components could also perform a direct role on T cells. C3a and C5a combined with C3aR and C5aR have been clarified to mediate T-cell induction and inhibit Treg function (55,56). We believed that complement produced by RT might regulate anti-tumor immunity by directly binding to and promoting the up-regulation of receptors on the surface of immune cells. In addition, Gunn et al. reported that the C5a effect in T-cell responses occurred in a concentration dependent manner (57). As shown in our study, RT induced the release of C5a in concentrations up to 600 pg/mL in the TME. High C5a levels within the TME stimulated tumor progression accompanied by impacting the infiltration of CD8+ T cells (57), which was similar to findings from our research. Together, this study was the first to confirm that C5a-C5aR mediated by RT suppressed acquired antitumor immunity to result in development of radioresistance.
The PI3K/AKT pathway is one of the main pathways to maintain the survival of tumor cells, and it is mostly upregulated in human tumors. Studies have shown that RT could significantly activate the PI3K/AKT pathway, enhance the repair of DNA damage caused by RT, promote epithelial-mesenchymal transformation (EMT), and generate RT resistance (42,58-60). These findings suggested that the PI3K/AKT signaling pathway played a complex role as a mediator, affecting the effect of RT by mediating the biological effects induced by RT. The effects of C5a on the enhancement of cancer invasion were associated with activation of the PI3K/AKT pathway (61). Similarly, our data also showed that RT strengthened the C5a-C5aR system by impressing the AKT signaling pathway. Blocking with the transfer of p65 into the cell nucleus decreased the activity of the C5aR1 promoter (45). Alongside the inhibition of NF-κB signaling pathway, C5a secretion is dampened (37). Western blotting revealed the phosphorylation and degradation of IκBα in mice treated RT. The complement system could secrete proinflammatory cytokines including IL-1β, IL-6, and TNF-α in human monocytes/macrophages (62). Antagonism to C3aR and C5aR1 have been shown to inhibit IL-10 production in CD8+ T cells to represent a novel mechanism of complement-mediated immunosuppression (63). We discovered that RT upregulated tumor inflammation and mRNA level of TNFα, IL-6, IL-12, and C5aR. Whether the blockade of these proinflammatory cytokines could generate complement-mediated radioresistance requires further research.
Our study had certain limitations. We recognize the limitations of using animal models to simulate human clinical diseases. Conclusions based on animal experiments needed to be validated by further clinical studies. In addition, in this study, we only found the phenomena of complement C5a reversing radioresistance, the specific underlying mechanisms and changes in the TME need to be further explored.
Conclusions
In conclusion, RT promotes the release of C5a from tumor cells and leads to up-regulation of C5aR1 expression on CD8+ T cells via the AKT/NF-κB pathway. Inhibition of the combination of complement C5a and C5aR can modulate antitumor immune responses and improve RT sensitivity. Our work provides evidence that the combination of RT and C5aR blockade open a new window of opportunity and multiple therapeutic targets to promote anti-tumor therapeutic effect in lung cancer. C5a can effectively improve long-term survival of lung cancer patients treated with radiotherapy as a radiotherapy sensitizer.
Acknowledgments
The authors appreciate the academic support from the AME Lung Cancer Collaborative Group. The authors also appreciate the Ethics Committee of Shandong Provincial Qianfoshan Hospital.
Funding: This study was funded by the Shandong Natural Science Foundation (Nos. ZR2021LSW023, ZR2022MH111, ZR2021QH356 and ZR2022MH103) and Shandong Province Medicine and Health Science and Technology Development Plan Project (No. 202009031334).
Footnote
Reporting Checklist: The authors have completed the ARRIVE reporting checklist. Available at https://tlcr.amegroups.com/article/view/10.21037/tlcr-23-258/rc
Data Sharing Statement: Available at https://tlcr.amegroups.com/article/view/10.21037/tlcr-23-258/dss
Peer Review File: Available at https://tlcr.amegroups.com/article/view/10.21037/tlcr-23-258/prf
Conflicts of Interest: All authors have completed the ICMJE uniform disclosure form (available at https://tlcr.amegroups.com/article/view/10.21037/tlcr-23-258/coif). The authors have no conflicts of interest to declare.
Ethical Statement: The authors are accountable for all aspects of the work in ensuring that questions related to the accuracy or integrity of any part of the work are appropriately investigated and resolved. All animal protocols were approved by The First Affiliated Hospital of Shandong First Medical University & Shandong Provincial Qianfoshan Hospital Animal Ethics Committee (No. 2022-S303), in accordance with the Guide for the Care and Use of Laboratory Animals published by the US National Institute of Health (NIH; Bethesda, MD, USA; publication No. 96-01).
Open Access Statement: This is an Open Access article distributed in accordance with the Creative Commons Attribution-NonCommercial-NoDerivs 4.0 International License (CC BY-NC-ND 4.0), which permits the non-commercial replication and distribution of the article with the strict proviso that no changes or edits are made and the original work is properly cited (including links to both the formal publication through the relevant DOI and the license). See: https://creativecommons.org/licenses/by-nc-nd/4.0/.
References
- Siegel RL, Miller KD, Fuchs HE, et al. Cancer statistics, 2022. CA Cancer J Clin 2022;72:7-33. [Crossref] [PubMed]
- Thai AA, Solomon BJ, Sequist LV, et al. Lung cancer. Lancet 2021;398:535-54. [Crossref] [PubMed]
- Vinod SK, Hau E. Radiotherapy treatment for lung cancer: Current status and future directions. Respirology 2020;25:61-71. [Crossref] [PubMed]
- Césaire M, Montanari J, Curcio H, et al. Radioresistance of Non-Small Cell Lung Cancers and Therapeutic Perspectives. Cancers (Basel) 2022;14:2829. [Crossref] [PubMed]
- Olivares-Urbano MA, Griñán-Lisón C, Marchal JA, et al. CSC Radioresistance: A Therapeutic Challenge to Improve Radiotherapy Effectiveness in Cancer. Cells 2020;9:1651. [Crossref] [PubMed]
- Sheng J, Zhao Q, Zhao J, et al. SRSF1 modulates PTPMT1 alternative splicing to regulate lung cancer cell radioresistance. EBioMedicine 2018;38:113-26. [Crossref] [PubMed]
- McLaughlin M, Patin EC, Pedersen M, et al. Inflammatory microenvironment remodelling by tumour cells after radiotherapy. Nat Rev Cancer 2020;20:203-17. [Crossref] [PubMed]
- Herrera FG, Bourhis J, Coukos G. Radiotherapy combination opportunities leveraging immunity for the next oncology practice. CA Cancer J Clin 2017;67:65-85. [Crossref] [PubMed]
- Deng L, Liang H, Burnette B, et al. Irradiation and anti-PD-L1 treatment synergistically promote antitumor immunity in mice. J Clin Invest 2014;124:687-95. [Crossref] [PubMed]
- Lippitz BE, Harris RA. A translational concept of immuno-radiobiology. Radiother Oncol 2019;140:116-24. [Crossref] [PubMed]
- Wang Y. Advances in Hypofractionated Irradiation-Induced Immunosuppression of Tumor Microenvironment. Front Immunol 2021;11:612072. [Crossref] [PubMed]
- Zhang J, He T, Xue L, et al. Senescent T cells: a potential biomarker and target for cancer therapy. EBioMedicine 2021;68:103409. [Crossref] [PubMed]
- Shinde-Jadhav S, Mansure JJ, Rayes RF, et al. Role of neutrophil extracellular traps in radiation resistance of invasive bladder cancer. Nat Commun 2021;12:2776. [Crossref] [PubMed]
- Krisnawan VE, Stanley JA, Schwarz JK, et al. Tumor Microenvironment as a Regulator of Radiation Therapy: New Insights into Stromal-Mediated Radioresistance. Cancers (Basel) 2020;12:2916. [Crossref] [PubMed]
- Liu J, Jiang M, Guan J, et al. LncRNA KCNQ1OT1 enhances the radioresistance of lung squamous cell carcinoma by targeting the miR-491-5p/TPX2-RNF2 axis. J Thorac Dis 2022;14:4081-95. [Crossref] [PubMed]
- Roumenina LT, Daugan MV, Petitprez F, et al. Context-dependent roles of complement in cancer. Nat Rev Cancer 2019;19:698-715. [Crossref] [PubMed]
- Yuan M, Liu L, Wang C, et al. The Complement System: A Potential Therapeutic Target in Liver Cancer. Life (Basel) 2022;12:1532. [Crossref] [PubMed]
- Cho MS, Vasquez HG, Rupaimoole R, et al. Autocrine effects of tumor-derived complement. Cell Rep 2014;6:1085-95. [Crossref] [PubMed]
- Reis ES, Mastellos DC, Ricklin D, et al. Complement in cancer: untangling an intricate relationship. Nat Rev Immunol 2018;18:5-18. [Crossref] [PubMed]
- Revel M, Sautès-Fridman C, Fridman WH, et al. C1q+ macrophages: passengers or drivers of cancer progression. Trends Cancer 2022;8:517-26. [Crossref] [PubMed]
- Ding P, Xu Y, Li L, et al. Intracellular complement C5a/C5aR1 stabilizes β-catenin to promote colorectal tumorigenesis. Cell Rep 2022;39:110851. [Crossref] [PubMed]
- Senent Y, Tavira B, Pio R, et al. The complement system as a regulator of tumor-promoting activities mediated by myeloid-derived suppressor cells. Cancer Lett 2022;549:215900. [Crossref] [PubMed]
- Gao J, Zhang LX, Ao YQ, et al. Elevated circASCC3 limits antitumor immunity by sponging miR-432-5p to upregulate C5a in non-small cell lung cancer. Cancer Lett 2022;543:215774. [Crossref] [PubMed]
- Dunkelberger J, Zhou L, Miwa T, et al. C5aR expression in a novel GFP reporter gene knockin mouse: implications for the mechanism of action of C5aR signaling in T cell immunity. J Immunol 2012;188:4032-42. [Crossref] [PubMed]
- Ajona D, Ortiz-Espinosa S, Moreno H, et al. A Combined PD-1/C5a Blockade Synergistically Protects against Lung Cancer Growth and Metastasis. Cancer Discov 2017;7:694-703. [Crossref] [PubMed]
- Medler TR, Murugan D, Horton W, et al. Complement C5a Fosters Squamous Carcinogenesis and Limits T Cell Response to Chemotherapy. Cancer Cell 2018;34:561-578.e6. [Crossref] [PubMed]
- Downs-Canner S, Magge D, Ravindranathan R, et al. Complement Inhibition: A Novel Form of Immunotherapy for Colon Cancer. Ann Surg Oncol 2016;23:655-62. [Crossref] [PubMed]
- Zha H, Han X, Zhu Y, et al. Blocking C5aR signaling promotes the anti-tumor efficacy of PD-1/PD-L1 blockade. Oncoimmunology 2017;6:e1349587. [Crossref] [PubMed]
- Reichhardt MP, Meri S. Intracellular complement activation-An alarm raising mechanism? Semin Immunol 2018;38:54-62. [Crossref] [PubMed]
- Bruni D, Angell HK, Galon J. The immune contexture and Immunoscore in cancer prognosis and therapeutic efficacy. Nat Rev Cancer 2020;20:662-80. [Crossref] [PubMed]
- Parihar VK, Syage A, Flores L, et al. The Cannabinoid Receptor 1 Reverse Agonist AM251 Ameliorates Radiation-Induced Cognitive Decrements. Front Cell Neurosci 2021;15:668286. [Crossref] [PubMed]
- Liljedahl E, Konradsson E, Gustafsson E, et al. Combined anti-C1-INH and radiotherapy against glioblastoma. BMC Cancer 2023;23:106. [Crossref] [PubMed]
- Livak KJ, Schmittgen TD. Analysis of relative gene expression data using real-time quantitative PCR and the 2(-Delta Delta C(T)) Method. Methods 2001;25:402-8. [Crossref] [PubMed]
- Liu Q, Hao Y, Du R, et al. Radiotherapy programs neutrophils to an antitumor phenotype by inducing mesenchymal-epithelial transition. Transl Lung Cancer Res 2021;10:1424-43. [Crossref] [PubMed]
- Han G, Geng S, Li Y, et al. γδT-cell function in sepsis is modulated by C5a receptor signalling. Immunology 2011;133:340-9. [Crossref] [PubMed]
- Nitta H, Murakami Y, Wada Y, et al. Cancer cells release anaphylatoxin C5a from C5 by serine protease to enhance invasiveness. Oncol Rep 2014;32:1715-9. [Crossref] [PubMed]
- Su S, Chen J, Yao H, et al. CD10(+)GPR77(+) Cancer-Associated Fibroblasts Promote Cancer Formation and Chemoresistance by Sustaining Cancer Stemness. Cell 2018;172:841-856.e16. [Crossref] [PubMed]
- Jin Y, Chen Y, Tang H, et al. Activation of PI3K/AKT Pathway Is a Potential Mechanism of Treatment Resistance in Small Cell Lung Cancer. Clin Cancer Res 2022;28:526-39. [Crossref] [PubMed]
- Hayden MS, Ghosh S. NF-κB, the first quarter-century: remarkable progress and outstanding questions. Genes Dev 2012;26:203-34. [Crossref] [PubMed]
- Yao J, Du X, Chen S, et al. Rv2346c enhances mycobacterial survival within macrophages by inhibiting TNF-α and IL-6 production via the p38/miRNA/NF-κB pathway. Emerg Microbes Infect 2018;7:158. [Crossref] [PubMed]
- Nie Y, Wang Z, Chai G, et al. Dehydrocostus Lactone Suppresses LPS-induced Acute Lung Injury and Macrophage Activation through NF-κB Signaling Pathway Mediated by p38 MAPK and Akt. Molecules 2019;24:1510. [Crossref] [PubMed]
- Gong X, Li X, Jiang T, et al. Combined Radiotherapy and Anti-PD-L1 Antibody Synergistically Enhances Antitumor Effect in Non-Small Cell Lung Cancer. J Thorac Oncol 2017;12:1085-97. [Crossref] [PubMed]
- Gu C, Luo J, Lu X, et al. REV7 confers radioresistance of esophagus squamous cell carcinoma by recruiting PRDX2. Cancer Sci 2019;110:962-72. [Crossref] [PubMed]
- Dang N, Waer M, Sprangers B, et al. Improved Anti-Tumour Adaptive Immunity Can Overcome the Melanoma Immunosuppressive Tumour Microenvironment. Cancers (Basel) 2019;11:1694. [Crossref] [PubMed]
- Kong F, Tao Y, Yuan D, et al. Hepatitis B Virus Core Protein Mediates the Upregulation of C5α Receptor 1 via NF-κB Pathway to Facilitate the Growth and Migration of Hepatoma Cells. Cancer Res Treat 2021;53:506-27. [Crossref] [PubMed]
- Surace L, Lysenko V, Fontana AO, et al. Complement is a central mediator of radiotherapy-induced tumor-specific immunity and clinical response. Immunity 2015;42:767-77. [Crossref] [PubMed]
- Elvington M, Scheiber M, Yang X, et al. Complement-dependent modulation of antitumor immunity following radiation therapy. Cell Rep 2014;8:818-30. [Crossref] [PubMed]
- Markiewski MM, DeAngelis RA, Benencia F, et al. Modulation of the antitumor immune response by complement. Nat Immunol 2008;9:1225-35. [Crossref] [PubMed]
- Corrales L, Ajona D, Rafail S, et al. Anaphylatoxin C5a creates a favorable microenvironment for lung cancer progression. J Immunol 2012;189:4674-83. [Crossref] [PubMed]
- Ortiz-Espinosa S, Morales X, Senent Y, et al. Complement C5a induces the formation of neutrophil extracellular traps by myeloid-derived suppressor cells to promote metastasis. Cancer Lett 2022;529:70-84. [Crossref] [PubMed]
- Linde IL, Prestwood TR, Qiu J, et al. Neutrophil-activating therapy for the treatment of cancer. Cancer Cell 2023;41:356-372.e10. [Crossref] [PubMed]
- Brandolini L, d'Angelo M, Novelli R, et al. Paclitaxel binds and activates C5aR1: A new potential therapeutic target for the prevention of chemotherapy-induced peripheral neuropathy and hypersensitivity reactions. Cell Death Dis 2022;13:500. [Crossref] [PubMed]
- Li Z, Meng X, Wu P, et al. Glioblastoma Cell-Derived lncRNA-Containing Exosomes Induce Microglia to Produce Complement C5, Promoting Chemotherapy Resistance. Cancer Immunol Res 2021;9:1383-99. [Crossref] [PubMed]
- Wang Y, Zhang H, He YW. The Complement Receptors C3aR and C5aR Are a New Class of Immune Checkpoint Receptor in Cancer Immunotherapy. Front Immunol 2019;10:1574. [Crossref] [PubMed]
- Kwan WH, van der Touw W, Paz-Artal E, et al. Signaling through C5a receptor and C3a receptor diminishes function of murine natural regulatory T cells. J Exp Med 2013;210:257-68. [Crossref] [PubMed]
- Lalli PN, Strainic MG, Yang M, et al. Locally produced C5a binds to T cell-expressed C5aR to enhance effector T-cell expansion by limiting antigen-induced apoptosis. Blood 2008;112:1759-66. [Crossref] [PubMed]
- Gunn L, Ding C, Liu M, et al. Opposing roles for complement component C5a in tumor progression and the tumor microenvironment. J Immunol 2012;189:2985-94. [Crossref] [PubMed]
- Yuan Y, Liao H, Pu Q, et al. miR-410 induces both epithelial-mesenchymal transition and radioresistance through activation of the PI3K/mTOR pathway in non-small cell lung cancer. Signal Transduct Target Ther 2020;5:85. [Crossref] [PubMed]
- Ferrandon S, DeVecchio J, Duraes L, et al. CoA Synthase (COASY) Mediates Radiation Resistance via PI3K Signaling in Rectal Cancer. Cancer Res 2020;80:334-46. [Crossref] [PubMed]
- Toulany M, Rodemann HP. Phosphatidylinositol 3-kinase/Akt signaling as a key mediator of tumor cell responsiveness to radiation. Semin Cancer Biol 2015;35:180-90. [Crossref] [PubMed]
- Maeda Y, Kawano Y, Wada Y, et al. C5aR is frequently expressed in metastatic renal cell carcinoma and plays a crucial role in cell invasion via the ERK and PI3 kinase pathways. Oncol Rep 2015;33:1844-50. [Crossref] [PubMed]
- Takabayashi T, Vannier E, Clark BD, et al. A new biologic role for C3a and C3a desArg: regulation of TNF-alpha and IL-1 beta synthesis. J Immunol 1996;156:3455-60. [Crossref] [PubMed]
- Peng W, McKenzie JA, Hwu P. Complementing T-cell Function: An Inhibitory Role of the Complement System in T-cell-Mediated Antitumor Immunity. Cancer Discov 2016;6:953-5. [Crossref] [PubMed]
(English Language Editor: J. Jones)