This article has an erratum available at: http://dx.doi.org/10.21037/tlcr-2024-1 the article has been update on 2024-09-13 at here.
EGFR exon 20 insertion mutations and ERBB2 mutations in lung cancer: a narrative review on approved targeted therapies from oral kinase inhibitors to antibody-drug conjugates
Introduction
The last 20 years have seen the explosion of technological advances that have allowed for translation of biomedical knowledge into routine clinical practice within the field of medical oncology. The culmination of these factors within the field of thoracic oncology has heralded the era of precision oncology for lung cancer care (1-6). Thoracic tumors are a heterogeneous group of malignancies—and even among lung cancers specifically, there are multiple histological variants that represent the epithelial, mesenchymal, and other cells of origin of the neoplastic process (Figure 1). Non-small-cell lung cancers (NSCLCs) are the most prevalent of these, comprising of mostly lung adenocarcinoma and squamous cell carcinoma (Figure 1A). Both larger entities can then be further subdivided into clinically relevant subgroups based on tumor biomarkers (7-12). Of these, identification of actionable oncogenic driver alterations (mutations or gene rearrangements) and tumor programmed death ligand 1 (PD-L1) cell surface expression are critical for optimal treatment selection and outcomes in these cancers (13-16). Lung adenocarcinomas are far more robustly enriched with these mostly non-overlapping actionable driver oncogene aberrations that have common intracellular properties leading to constitutive activation of proliferative signaling cascades and induce the anti-apoptotic cellular machinery (32-35). The majority of clinically-relevant de novo driver oncogenes are truncal evolutionary events that are retained by NSCLCs (36,37). Presently, the list of driver oncogenic alterations tied to precision therapies approved by international regulatory bodies include: EGFR, ALK, ROS1, BRAF, MET, RET, KRAS, ERBB2, and NTRK (Figure 1A)—with many others rapidly emerging (13,38-40).
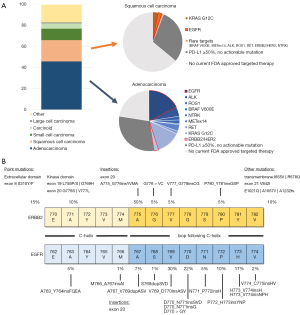
Activating events of ErbB family members are common across all cancer types and represent some of the most frequent driver oncogenes in NSCLC (24-26,41-43). ErbB-1 (also known as epidermal growth factor receptor, EGFR) and ErbB-2 (also known as Her2) are two of the most successful examples of actionable driver oncogenes. Mutations of the EGFR gene are present in more than 15–20% of all NSCLC, particularly in lung adenocarcinomas occurring in younger patients, those of Asian descent, and in the presence of limited/no tobacco exposure as compared to NSCLCs occurring in other patient cohorts (10,17,44-47). Mutations of the ERBB2 gene are present in less than 5% of all NSCLCs and with similar enrichment amongst the populations previously noted (26-31). In both EGFR- and ERBB2-affected NSCLCs, the hotbed of oncogenic driver alterations is within the exon 20 kinase domain. Moreover, these are almost exclusively somatic events—very few cases of EGFR- or ERBB2-mutated NSCLC are associated with germline genetic susceptibility (48).
This review will provide an overview of EGFR and ERBB2 mutations in NSCLC, with a focus on recent clinical approvals of kinase inhibitors and antibodies that have added to the armamentarium of precision oncology within this cohort of cancers with previously unmet need. We present this article in accordance with the Narrative Review reporting checklist (available at https://tlcr.amegroups.com/article/view/10.21037/tlcr-23-98/rc).
Methods
Information used to write this review article was collected from a PubMed.gov search from years 2000 to 2023 using key words EGFR, ERBB2, and exon 20 insertion; hand searches of the references of retrieved literature; personal searches for texts on literature reviews of lung cancers with different ErbB mutations; discussions with co-authors and experts in the field; and personal experience of the senior authors participating in and writing expert reviews of the literature on similar topics. Both preclinical and clinical studies were evaluated. Table 1 summarizes the search strategy for this narrative review.
Table 1
Items | Specification |
---|---|
Date of search | 11/01/2022 to 02/01/2023 |
Databases and other sources searched | PubMed.gov, ASCO annual meeting abstracts, AACR annual meeting abstracts, ESMO annual meeting abstracts, IASLC annual meeting abstracts |
Search terms used | EGFR, ERBB2, and exon 20 insertion |
Timeframe | 06/04/2004 to 01/31/2023 |
Inclusion and exclusion criteria | No exclusion was made to maximize source identification. Manual searches of the references of retrieved literature, personal searches for texts on literature reviews, discussions with co-authors and experts in the field, personal experience of the senior authors (DC, PV, SK) participating in and writing expert reviews of the literature on similar topics were used to maximize inclusion |
Selection process | Four authors (DS, EA, HV, DBC) conducted the initial selection and then consensus was obtained from all authors on literature, meeting abstracts and online references to be used |
Preclinical models of EGFR and ERBB2-directed therapies in NSCLC
The biomedical community has performed extensive characterization of ErbB mutant proteins, with the majority of focus on EGFR and ERBB2 wild-type (WT) proteins and kinase domain mutations (Figure 2). Many of these preclinical models of isolated proteins or rudimentary cell lines have been quite informative of patterns of response and resistance to EGFR/ERBB2 tyrosine kinase inhibitors (TKIs) with close relationship to clinically-observed activity of the same compounds.
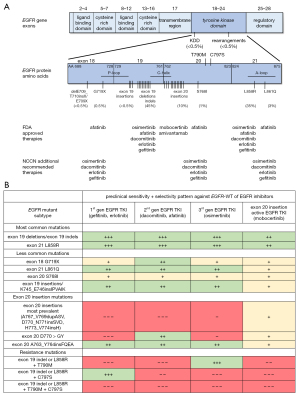
There is significant heterogeneity in the prevalence of EGFR kinase domain aberrations noted, with most mutations occurring within exons 18 to 21 of the kinase domain of the EGFR gene (10,17,44-47) (Figure 2A). The most common mutations center on in-frame deletions or indels of exon 19 (EGFR-delE746_A750 the typical example of LREA-type amino-acid deletions) and the exon 21 L858R mutation (Figure 2A). These common canonical mutants lead to structural changes that change ATP kinetics, enhance EGFR activation (promoting an “on” instead of “off” switch), and generate a favorable therapeutic window to almost all EGFR TKIs that have completed clinical development, including: 1st generation reversible inhibitors (gefitinib, erlotinib), 2nd generation irreversible inhibitors (afatinib, dacomitinib), 3rd generation mutation-selective covalent inhibitors (osimertinib), and EGFR exon 20 insertion active inhibitors (poziotinib, dacomitinib) (33,63-69). This mutation-to-drug response phenotype translates well to the clinical realm, where tumors driven by common EGFR mutations are highly responsive to EGFR TKIs and experience clinical outcomes that far exceed what is typically seen with cytotoxic chemotherapy or immunotherapy (70-77). Hence, these EGFR TKIs have gained regulatory approval for use as frontline treatment options for those with advanced stage disease and are now entering the adjuvant arena (osimertinib) for those with earlier stage disease (78).
However, not all driver alterations in EGFR have proven to be equally actionable, and those with canonical exon 19 deletions or L858R point mutations have experienced the greatest progress with application of available targeted therapies to date. Head-to-head clinical trials have been able to show that for patients with tumors harboring EGFR-exon 19 deletions/indels or L858R, the 3rd generation EGFR TKI osimertinib outperforms others with higher and more durable response rates both systemically and in the central nervous system (CNS) along with lower rates of mucocutaneous/gastrointestinal toxicities and better overall tolerability (79). Osimertinib is therefore the preferred option for palliative first line therapy in cases with advanced disease and following surgery and chemotherapy in the adjuvant setting (78,79).
Other less common EGFR mutations (Figure 2A) display similar structural, drug inhibition, and clinical translational characteristics—but with far greater heterogeneity in their overall efficacy (Figure 2B). Some investigators have proposed using a structure-function subgrouping to better classify in vitro sensitivity of these different structurally-defined categories of EGFR mutants: (I) classical-like (exon 19 deletions/indels, L858R, L861Q) with broad sensitivity to multiple generations of EGFR-directed TKIs; (II) P-loop αC-helix compressing (PACC) (G719X, S768I) with highest sensitivity to 2nd generation irreversible EGFR TKIs (Figure 2B); (III) T790M-like hydrophobic core mutants (such as compound EGFR-T790M), and (IV) exon 20 loop insertions (80). Case series and non-randomized trials (49,81,82) have confirmed activity of afatinib and led to the approval of this drug for tumors with EGFR-G719X, -S768I or -L861Q mutations (Figure 2). Single arm studies have also confirmed the activity of osimertinib (83,84); use of this agent for these less common mutations is thus also endorsed by expert consensus groups due to its more favorable toxicity profile the National Comprehensive Cancer Network (NCCN) (39) (Figure 2). Other less common but also variably EGFR TKI-responsive mutations in preclinical models and patients with NSCLC include: EGFR exon 19 insertions (17,50), EGFR exon 18 indel delE709_T710insX or E709X (51-53), EGFR kinase domain duplications (54) and EGFR fusion genes (55) (Figure 2).
EGFR exon 20 insertion mutations as a group account for close to 10% of all EGFR mutations (Figure 2A) and the most heterogeneous cohort with multiple types on insertions/indels occurring along the span of the exon (Figure 1B) along with highly variable patterns of response to EGFR-directed therapies (18-22,56) (Figure 2B). The most prevalent mutants cluster around insertions spanning amino-acids EGFR-A767, -V769, -D770, -P772 and -N773 (Figure 1B), which localize to the loop following the C-helix of the kinase domain. The crystal structure of one of these EGFR exon 20 insertion mutations reveals an unaltered ATP binding pocket in relation to EGFR WT; the inserted amino acid residues form a wedge at the end of the C-helix that promotes the active kinase conformation and related kinetic changes (19). Quite unlike the mechanism of activation seen with other types of EGFR-affecting alterations, this conformal change renders an unfavorable therapeutic window for 1st, 2nd, and most 3rd generation EGFR TKIs (19,20,56,85). Preclinical models have confirmed primary insensitivity of these exon 20 mutants to these prior EGFR TKIs (Figure 2B).
Multiple academic and pharmaceutical groups have used the growing structural and biochemical knowledge of these mutants to screen for or design EGFR TKIs that could exploit the non-ATP binding pocket structural changes and yield modest therapeutic windows in relation to EGFR WT (23,57-59,86). Most of the small molecules that fit this profile are covalent EGFR inhibitors and include poziotinib, mobocertinib, sunvozertinib, and zipalertinib among others. Preclinical models of the most frequent EGFR exon 20 insertion mutants consistently show only modest sensitivity and selectivity of mobocertinib and similar drugs (Figure 2B). The clinical development of such agents is outlined further below.
However, not all EGFR exon 20 insertion mutations are insensitive to previously existing EGFR TKIs. Notably, the EGFR-A763_Y764insFQEA mutation (and the identical EGFR-D761_E762insEAFQ)—accounting for 5% of exon 20 insertion mutations—stands at the transition between exons 19 and 20 and alters the register of the C-helix toward its N-terminus resulting in structural and kinetic alterations that resemble those seen with other EGFR TKI-sensitizing mutations (such as exon 21 L858R and exon 21 L861Q). This leads to favorable preclinical therapeutic windows for all approved classes of EGFR TKIs (19,60) (Figure 2B). When translated into the clinic, patients whose tumors harbor this mutation have had prolonged responses to aforementioned EGFR TKIs. It is thus reasonable to offer off-label gefitinib, erlotinib, afatinib, dacomitinib, or osimertinib in these cases.
Other rare EGFR exon 20 mutations with unique patterns of responsiveness to available therapies include the EGFR-D770 to -G770 alteration that permits response to the irreversible 2nd generation EGFR-TKIs afatinib and dacomitinib (61,62) (Figure 2B). These variants also have modest sensitivity to mobocertinib (Figure 2B) and other similar EGFR TKIs and have been included in completed and ongoing clinical studies investigating novel agents for exon 20 insertion mutations.
In contrast, previously existing EGFR-directed monoclonal antibodies like cetuximab have almost no preclinical or clinical activity as monotherapy for EGFR-mutated NSCLCs (87,88). Novel classes of bivalent EGFR antibodies have had better success in both preclinical and clinical realms. Amivantamab (formerly called JNJ-61186372) is a bispecific antibody targeting extracellular domain of EGFR-MET that shows preclinical activity in TKI-sensitive and TKI-resistant EGFR-mutated NSCLC models. Its activity may be dictated by direct signal inhibition of EGFR plus MET and/or induction of immune-directed antitumor activity (89-92). Antibody-drug conjugates (ADCs) that target other ErB family members that heterodimerize with EGFR also have activity against TKI-sensitive and TKI-resistant EGFR-mutated NSCLC models, and the ErbB3-directed ADC patritumab deruxtecan (HER3-Dxd) is one of the lead drugs undergoing clinical development (93).
ERBB2 (Her2) mutations are also heterogeneous (Figure 1B) and span aberrations including point mutations and exon 20 insertions closely resembling those previously discussed for EGFR. Unlike in other malignancies, ERBB2 mutation—rather than gene amplification or overexpression—appears most predictive of clinical benefit with leverage of ERBB2-directed therapies in NSCLC. The most prevalent ERBB2 exon 20 insertion mutation in lung cancers is ERBB2-A775_G776insYVMA (28,94-100). Preclinical characterization has disclosed that this mutant does not lead to favorable therapeutic windows in relation to EGFR or ERBB2 WT proteins, and clinically-available pan-EGFR/ERBB2 TKIs (such as afatinib, dacomitinib, neratinib) have been tested and have not proven to be effective (101-104). Some pan-EGFR/ERBB2 TKIs narrow therapeutic windows in preclinical models include pyrotinib, poziotinib, and mobocertinib (105,106). However, other ERBB2 exon 20 insertion mutations are less recalcitrant in preclinical models. The ERBB2-P780_Y781insGSP (Figure 1B) and other rare ERBB2 insertions that lead to glycine insertions at positions similar to EGFR-D770>GY are most sensitive to irreversible EGFR/ERBB2 TKIs such as afatinib, dacomitinib and poziotinib (62,107,108). Some patients with these less common ERBB2 exon 20 insertion mutations have had more robust clinical responses to afatinib or dacomitinib (62) and have been amongst those with greatest benefit from poziotinib in clinical studies (109,110). ERBB2 TKIs are minimally active in models with ERBB2 point mutations (27). Novel pan-ERBB TKIs with improved efficacy against ERBB2 exon 20 insertion mutations and CNS penetration, such as TAS2940, continue to undergo preclinical development (111).
Irrespective of location/type of mutation within ERBB2 (Figure 1B), activating mutants lead to enhanced expression of ERBB2 protein that can be exploited to target the extracellular domain of this receptor tyrosine kinase with antibodies and ADCs. Preclinical analyses of both ERBB2-mutated and ERBB2-amplified cancers demonstrate that ERBB2 ubiquitination and internalization are the main events underlying receptor endocytosis and the efficacy of the anti-ERBB2 ADCs ado-trastuzumab emtansine (T-DM1) and trastuzumab deruxtecan (T-DXd) in lung cancer models (112,113).
Mechanisms of resistance to EGFR/ERBB2 TKIs and other oncogene-directed therapies fall into four main categories: (I) on-target resistance mutations [e.g., EGFR-T790M and EGFR-C797S (Figure 2B)]; (II) off-target activation of co-occurring bypass pathways usually through genomic events (alternative oncogene mutation, amplification or rearrangement); (III) epigenetically-mediated histological transformation (usually from adenocarcinoma to high grade neuroendocrine tumors that no longer express/depend on the truncal oncogene); (IV) or as yet unidentified mechanisms that may include changes other than in the genomic landscape alone and that alter the tumor microenvironment and/or pharmacokinetic/pharmacodynamic effects of the drug (47,114-122). In the case of EGFR-mutated NSCLC, these events are present in different frequencies depending on the line of therapy and class of EGFR TKI used. On-target resistance seems to be more frequent when 1st or 2nd generation EGFR TKIs are used in preclinical models and clinical care. EGFR-T790M is the single most common mechanism of resistance with early generation EGFR TKIs, while far more heterogeneous mechanisms of acquired resistance (including EGFR-C797S and others) are more commonly seen with mutation-selective advanced generation covalent TKIs like osimertinib. With the latter, the EGFR-C797S mutation and other definable mechanisms of on-target resistance are identified in less than 15% of rebiopsy series (123). Osimertinib resistance is instead defined by a spectrum of off-target aberrations in parallel signaling pathways involving MET, BRAF, ALK, and RET (123,124).
The mechanisms of acquired resistance to novel exon 20-directed therapies are still being elucidated in evolving post-approval analyses and routine clinical practice. It is likely that both on-target and off-target aberrations similar to those seen with other EGFR-mutated NSCLCs will be identified with time. Our group and others have described in preclinical models that EGFR-C797S is a pan-resistance mutation when occurring in compound with typical EGFR exon 20 insertions in cell lines treated with mobocertinib, poziotinib, and zipalertinib (58,125). EGFR-T790M in compound with exon 20 insertions appears to render resistance to mobocertinib and poziotinib (58,109,126). Less is known about mechanisms of resistance to antibodies and ADCs where resistance may not be solely from biological effects on the target, but may reflect more complex pharmacokinetic and immune changes in the tumor or host.
From bench to bedside: use of EGFR and ERBB2 exon 20-directed therapies in the clinic
Building upon evolving preclinical knowledge amassed by our group and others over the past decade, clinical approvals of several EGFR and ERBB2 exon 20-directed therapies in NSLC have been forthcoming in recent years: mobocertinib [2021], amivantamab [2021], and trastuzumab deruxtecan [2022]. Each will be discussed below.
Mobocertinib
Mobocertinib (formerly named TAK-788 or AP-32788) is a novel EGFR TKI that forms a covalent bond to EGFR (at EGFR-C797) and ERBB2 (at ERBB2-C805). As most exon 20 insertion mutations result in preservation of the typical ATP binding pocket relied upon for activity of 1st, 2nd, and 3rd generation EGFR TKIs, its development hinged on identifying structural changes that would create conformational selectivity for the mutated receptor so as to afford efficacy with manageable toxicity (57). To block oncogenic kinase activation, mobocertinib forms a covalent bond with EGFR-C797 and interacts with the gatekeeper residue through its isopropyl ester moiety which further selects for activity against EGFR exon 20 mutants (57). The drug is a derivate of osimertinib that was modified to achieve its properties in structural modeling and systems (127,128). As previously discussed, mobocertinib has demonstrated in vitro activity against models with EGFR or ERBB2 exon 20 insertion-mutated NSCLC (57,58,105).
Mobocertinib was first clinically studied in a phase I/II multicenter clinical trial NCT02716116 (129). Eligible patients had previously treated metastatic disease with exon 20 insertion mutations in either EGFR or ERBB2. The suitable (and maximum tolerated) dose was found to be 160 mg daily in the dose escalation phase (130). Across all patients, there were 96% treatment-related adverse events (TRAEs), 40% of which were grade ≥3. The most common TRAEs were: diarrhea (83%, 21% ≥ grade 3), nausea (43%), and rash (33%); 54% of patients required dose interruption, 17% dose reduction, and 16% discontinued the medication (130). In terms of pharmacokinetics, maximum plasma concentration was achieved at 4 hours, with half-life in the range of 11–17 hours. At the target dose of 160 mg (n=28) in patients with EGFR-mutated NSCLC, the investigator assessed objective response rate (ORR) was 43% (95% CI: 24–63%), median duration of response (DoR) 13.9 months [5.0–not reached (NR)], and median progression-free survival (PFS) 7.3 months (4.4–15.6 months). Response was independent of the specific EGFR exon 20 mutant variant in this small cohort (130). However, a lower response rate in patients with baseline brain metastasis (intracranial ORR 25%, with a range of 5–57%), suggests limited CNS activity in this small (n=12) subset of patients (130).
Building upon these findings, the trial was expanded (131): newly recruited patients joined the EXCLAIM extension cohort (n=96); of those, patients who had previously received platinum-based chemotherapy (n=86) joined the initial group of patients involved in the dose escalation phase (n=28) to form the platinum-pretreated patients (PPPs) cohort (n=114). Importantly, recruitment for the expansion portion of the trial was international, and over half of the patients were recruited from Asian centers. In the PPP cohort, the independently reviewed ORR was 28% (95% CI: 20–37%), median DoR 17.5 months (7.4–20.3 months), median PFS remained 7.3 months (5.5–9.2 months), and median overall survival (OS) was 24.0 months (14.6–28.8 months). In turn, the EXCLAIM cohort had similar ORR at 25% (17–35%), median DoR was NR (5.6–NR), median PFS was also 7.3 months (5.5–9.1 months), and median OS was NR (13.1–NR). No subgroups were noted to derive specific benefit in either cohort, including when stratified by type of EGFR exon 20 insertion mutation, prior therapy, or baseline brain metastases (131). Most sites of progression were in the brain (22/58), again hinting at reduced CNS efficacy. There were no new safety signals observed, with a predominance of diarrhea (91%, 21% ≥ grade 3) and similar rates of dose reduction (25%) and discontinuation (17%) as seen in the initial phase of the trial (131).
Patient-reported outcomes (PROs) were also captured using the EORTC QLQ-C30 [general quality of life (QoL)] and QLQ-LC13 (lung cancer module). Improvements in dyspnea, cough, and chest pain were noted. Nevertheless, there were no positive or negative changes on overall QoL, although there was a transient reduction in diarrhea scores that improved by study completion (131). A more detailed analysis of PROs with mobocertinib 160 mg daily detailed the frequent detriments in QoL scores related to diarrhea and appetite loss, while improvements were observed for dyspnea, insomnia, and constipation (132). Investigators have since published proposals for proactive management of both dermatologic and gastrointestinal toxicities to minimize the need for mobocertinib dose reductions/interruptions (133). Real-world experience with mobocertinib has mirrored that seen in these initial clinical studies (134).
The findings from NCT02716116 and its expansion cohorts (such as EXCLAIM) are limited by the lack of a control arm (phase I/II design). However, as the outcomes with use of this agent meet an important unmet clinical need, mobocertinib was granted accelerated approval September 2021 by the United States Food and Drug Administration (FDA) for EGFR exon 20 insertion-mutated advanced NSCLC for treatment following disease progression on platinum-based chemotherapy. The use of mobocertinib in the first line setting for metastatic disease is being evaluated in the ongoing phase III trial EXCLAIM-2 (NCT04129502) (135), which is nearing accrual completion and will hopefully determine if mobocertinib should overtake platinum-based chemotherapy as the de facto evidence-based frontline therapy for patients with advanced NSCLC harboring an EGFR exon 20 insertion mutation (135).
To date, there are no published clinical studies specifically studying the effects of mobocertinib in ERBB2 exon 20 insertion-mutated NSCLC. While there were some patients (n=21) in the NCT02716116 trial, the published outcomes reference the EGFR-mutated cohort exclusively (130). Only one preclinical study thus far has studied mobocertinib in two models of ERBB2-mutated NSCLC, driven by ERBB2-A775_G776insYVMA and ERBB2-G776>VC, and with an eye towards identifying activity, dosing, and resistance mechanisms (105). Although mobocertinib has preclinical activity against other EGFR mutations (Figure 2B), there are no ongoing clinical studies targeting common and uncommon mutations that have already been paired with other FDA-approved targeted agents (Figure 2A).
Amivantamab-vmjw
Amivantamab is a humanized, bispecific antibody that binds EGFR and MET extracellularly to target activating mutations, including EGFR exon 20 insertion mutations, as well as MET mutations and amplification (89). Three distinct mechanisms inhibit tumor growth in the setting of aberrant EGFR and MET signaling: (I) immune effector cells (such as NK cells and macrophages) target mutated tumor cells for destruction through antibody-dependent cellular cytotoxicity, trogocytosis and phagocytosis (89); (II) inhibition of ligand binding prevents ligand-induced activation, phosphorylation, and downstream signaling that promote cellular proliferation; and (III) downmodulation or degradation of EGFR and MET receptors, thereby decreasing tumor volume (89).
The antibody amivantamab was first studied in a phase I/II dose-escalation and dose-expansion study (NCT02609776/CHRYSALIS) (136). Eligible patients were previously treated with, ineligible for, or declined standard chemotherapy for metastatic NSCLC harboring EGFR exon 20 insertion mutations. The maximum tolerated dose identified was 1,050 mg (1,400 mg for patients ≥80 kg) once weekly administered intravenously for four weeks, followed by once every 2 weeks starting on week 5. Safety and efficacy were evaluated in the recommended phase two dose cohort (n=258), which included all patients given the maximum tolerated dose (137). Within this cohort, patients who had previously received platinum-based chemotherapy were included in the safety population (n=114), while those who had at least 3 disease assessments at the clinical cutoff were included in the efficacy population (n=81). In the efficacy population, the investigator-assessed ORR was 36% (95% CI: 25–47%), median PFS 8.3 months (5.5–10.6 months), and median OS 22.8 months (14.6–NR), although this endpoint was immature (137). Responses were seen irrespective of EGFR exon 20 insertion mutation type (137). In the safety population, 99% of patients experienced a TRAE (35% ≥ grade 3), most commonly rash (86%, 4% ≥ grade 3), infusion-related reaction (66%), paronychia (45%), and constipation (24%). Infusion-related reactions were observed primarily during the first dose, split over two days, with most occurring on day 1 (93%) as compared to day 2 (4%) of the infusion (137). TRAEs led to dose reductions (13%) and discontinuations (10%), most commonly for rash at 10% and 1.8%, respectively (137).
Though data are limited by the early phase nature of the trial, the FDA granted accelerated approval May 2021 to amivantamab for the treatment of advanced EGFR exon 20 insertion-mutated advanced NSCLC following prior platinum-based chemotherapy. The CHRYSALIS trial is ongoing and analyses of activity in other cohorts of EGFR-mutated advanced NSCLC are expected shortly (136). Use of amivantamab in the second-line setting with/without the third-generation EGFR inhibitor, lazertinib, is being evaluated in the ongoing phase I trial, CHRYSALIS-2 (NCT04077463) (138). In the first-line setting, amivantamab in combination with carboplatin and pemetrexed is ongoing in the phase III PAPILLON trial (NCT04538664) in patients with advanced EGFR exon 20 insertion-mutated NSCLC (139). A subcutaneous formulation is also currently being evaluated in the phase I PALOMA trial (NCT04606381) (140). There is no data studying the use of amivantamab in ERBB2-mutated NSCLC, and the absence of direct targeting of ERBB2 likely limits applications of this agent to tumors with EGFR or MET activation.
Fam-trastuzumab deruxtecan-nxki (T-DXd)
T-DXd is an ADC that consists of a humanized monoclonal antibody directed at ERBB2 with a cleavable tetrapeptide-based linker attached to a potent topoisomerase I inhibitor (a derivative of the camptothecin analog exatecan: DXd; DX-8951 derivative) as its cytotoxic drug payload (141). Preclinical models have shown T-DXd has a higher drug-to-antibody ratio and shorter payload half-life than other ERBB2-directed ADCs (mainly T-DM1) (142).
T-DXd initially received FDA approval as a breakthrough therapy in December 2019 for use in ERBB2-overexpressing, unresectable/metastatic breast cancer based on the results of the DESTINY-Breast01 trial (141). A phase I dose-expansion trial in multiple advanced solid tumors (n=60) with ERBB2 overexpression (immunohistochemistry of at least 1+) or ERBB2 mutations included 18 patients with advanced NSCLC (143), 11 of which had tumors with ERBB2 mutations (almost all of which were exon 20 insertions). ORR and median PFS in the overall cohort vs. ERBB2-mutated NSCLCs were 28.3% vs. 72.7% and 7.2 months (95% CI: 4.8–11.1 months) vs. 11.3 months (95% CI: 8.1–14.3 months), respectively (143).
DESTINY-Lung01 next specifically explored use of T-DXd exclusively in ERBB2-mutated NSCLC (144). The trial was conducted as a phase II, single arm trial of patients with previously treated, refractory metastatic ERBB2-mutant NSCLC (n=91). The trial reported an ORR of 55% (95% CI: 44–65%) with median DoR 9.3 months (95% CI: 5.7–14.7 months), median PFS 8.2 months (95% CI: 6.0–11.9 months), and median OS 17.8 months (95% CI: 13.8–22.1 months). Responses were seen across all ERBB2 mutation types and regardless of type/extent of prior cancer therapy, including prior pan-EGFR/ERBB2-directed therapies in 14% with some responses to T-DXd (144). Our group has similarly published our clinical experience with poziotinib following T-DXd (145). Though limited by small numbers, these outcomes suggest possible non-overlapping mechanisms of drug resistance between TKIs and ADCs directed against ERBB2. DESTINY-Lung02 study next endeavored to assess the safest and most effective dose of T-DXd in previously treated, ERBB2-mutated advanced NSCLC. T-DXd was evaluated at a dose of 6.4 vs. 5.4 mg/kg in a randomized dose-finding trial (146,147). The ORRs were similar across the two doses. Of note, the efficacy cohort of 5.4 mg/kg (n=52) disclosed ORR of 58% (95% CI: 43–71%) and the median DOR of 8.7 months (95% CI: 7.1–not estimable) (146,147).
Cytopenias are amongst the most prevalent TRAEs with use of T-DXd in the published studies to date. The most common TRAEs in DESTINY-Lung01 trial were neutropenia (15% grade 3) and anemia (10% grade 3) (144). Of particular importance is the identification of drug-induced interstitial lung disease (ILD) in both initial and sequent trials of T-DXd across cancer types, including treatment-related respiratory failure and death in 1 patient in the initial phase I trial. Drug-related ILD was reported in 26% of participants in DESTINY-Lung01 and resulted in death in 2% of all patients included in the trial (144). In DESTINY-Lung02, higher rates of drug-induced ILD at the 6.4 vs. 5.4 mg/kg dose (14.0% vs. 5.9%, respectively) led to selection of the 5.4 mg/kg dose administered intravenously every 3 weeks for further study and approval (146,147). In this study, any grade drug-related ILD event occurred in 5.9% and 14.0% of cases receiving T-DXd 5.4 or 6.4 mg/kg, respectively (147). This has resulted in a black box warning for ILD. As use of these agents evolves in real-world settings, it will be necessary to assess whether the risk of ILD is exacerbated by prior chest radiotherapy and/or immune checkpoint inhibitor (ICI) use as are commonly the case in the care of patients with NSCLC. Given poor tolerability of respiratory toxicities in patients with significant baseline pulmonary comorbidities, a high index of clinical suspicion and low threshold to identify, evaluate, and manage patients experiencing such toxicities will be necessary in this patient population (144). Clinical guidance on best practice for diagnosis and management of T-DXd-induced pneumonitis has been published (148).
On the basis of these results, T-DXd was granted accelerated approval in August 2022 by the FDA for use in metastatic or unresectable ERBB2-mutated NSCLC with disease progression following prior therapy. While the current approval is for patients with previously treated, ERBB2-mutated advanced NSCLC, important questions still remain regarding optimal combinations and sequencing of T-DXd with other biologically active systemic therapies in NSCLC (145). The ongoing DESTINY-Lung03 trial (NCT04686305) is investigating the safety of T-DXd in combination with platinum doublet chemotherapy and durvalumab (149). Also ongoing, DESTINY-Lung04 (NCT05048797) will investigate the safety and efficacy of T-DXd versus standard-of-care first-line treatments (platinum-doublet +/− pembrolizumab) in advanced NSCLC with ERBB2 exon 19 or 20 kinase domain mutations (150).
Therapies in development: poziotinib, sunvozertinib, zipalertinib
Poziotinib is an irreversible pan-ErbB inhibitor with activity against EGFR, ERBB2, and ERBB4. In vitro, poziotinib has demonstrated its abilities as a potent inhibitor of cells with EGFR or ERBB2 exon 20 insertions (23). However, concerns regarding toxicity have limited its use in the clinical setting. A phase II trial of poziotinib in EGFR-mutated advanced lung adenocarcinoma with acquired resistance to erlotinib/gefitinib (n=37) showed limited clinical efficacy with ORR of 8% and median PFS 2.7 months (151). Of note, this trial included predominantly patients with acquired EGFR-T790M; no patients with EGFR exon 20 insertions were included.
Subsequent preclinical studies further characterized the potential utility and clinical activity of poziotinib in EGFR exon 20 insertion mutations and ERBB2 mutations specifically, ultimately leading to clinical studies yielding more favorable results (23). A phase II trial was published in which patients with ERBB2 exon 20 insertion-mutated advanced NSCLC (n=30) received poziotinib 16mg daily; both previously treated and treatment-naïve patients were included (109). Investigators reported an ORR of 43% (95% CI: 25–63%), disease control rate (DCR) of 73%, and median PFS of 5.5 months (95% CI: 4.9–7.0 months). Another trial by the same group included patients with advanced NSCLC harboring EGFR exon 20 mutations (n=50) and yielded similar findings: ORR of 32% (95% CI: 20.7–45.8%), DCR of 84%, and median PFS again 5.5 months (95% CI: 5.0–9.4 months) (152). Patients in this latter trial were heavily pretreated (94%), 34% of whom had received prior EGFR TKI therapy. Acquired mechanisms of resistance to poziotinib were also explored in this study, with identification of EGFR-T790M, MET amplification, and epithelial to mesenchymal transition as has been seen with other EGFR-directed agents (152).
ZENITH20-2 is a phase II, single arm trial of poziotinib in previously treated advanced NSCLC with ERBB2 exon 20 insertion mutations (110,153). The trial enrolled 90 patients, all of whom were treated with poziotinib 16 mg daily. Similar to prior studies, the ORR was 27.8% (95% CI: 18.9–38.2%), DCR 70% (95% CI: 59.4–79.2%), and median PFS 5.5 months (95% CI: 3.9–5.8 months). Response rates were noted to be similar regardless of prior therapy, including 27.8% of patients who had received prior anti-EGFR/ERBB2 targeted therapy. Additionally, there was no difference in response based on the type of ERBB2 mutation present; ERBB2-A775_G776insYVMA was present in 65 out of 90 patients. ZENITH20-4 subsequently investigated poziotinib in treatment-naïve ERBB2-mutated advanced NSCLC (153,154). The results of this trial were presented in abstract form at the 2022 ESMO Targeted Anticancer Therapies Congress (154). The trial included 70 patients and reported a combined ORR of 41% (95% CI: 30–54%) with a median PFS of 5.6 months (range, 0–20 months) (154).
These phase II trial results have been further corroborated in a real-world study (n=30) describing clinical outcomes in an expanded access program that provided poziotinib for compassionate use to patients with advanced NSCLC with EGFR and ERBB2 exon 20 insertion mutations (155). In keeping with prior studies, an ORR of 30% with median PFS of 5.6 months (95% CI: 3.6–6.7 months) was reported (155).
High-grade gastrointestinal and mucocutaneous toxicities have been of significant concern with poziotinib and have limited the prospect for its use in routine clinical practice. In the ZENITH20-2 trial, the most common grade 3 TRAEs included rash and diarrhea in 78.9% of all patients, with 13% ultimately discontinuing poziotinib as a result (152). Other studies have shown similarly high rates of drug-induced rash, diarrhea, mucositis, paronychia, and xerosis in up to one-third of all patients and resulting in dose reduction in the majority (72%) of patients (109). Due to concerns regarding low overall response rates with minimal DoR and poor tolerability, the FDA elected not to approve poziotinib for use in EGFR- or ERBB2-mutated NSCLC (156), and enrollment in a phase 2 study of poziotinib in advanced NSCLC harboring EGFR or ERBB2 exon 20 insertion mutations was halted in early 2023 as a result (153).
Sunvozertinib (formerly DZD9008) is a newly developed EGFR exon 20 insertion mutation-active TKI which also irreversibly binds EGFR-C797 like mobocertinib, instead using an anilino-phenyl moiety to interact with the C-helix of EGFR (86). Sunvozertinib is currently being studied in an international phase I study in advanced NSCLC (157). With median follow-up thus far under 6 months, early clinical data suggests an ORR around 40% in heavily pretreated patients (86). Similar to other TKIs in this class, the primary toxicities appear to again be mostly gastrointestinal and dermatologic (86). There is additionally evidence that sunvozertinib may have anti-tumor activity against other EGFR-sensitizing mutations as well as ERBB2 exon 20 insertion mutants (86).
Zipalertinib (formerly TAS6417/CLN-081) is a pan-mutation-selective EGFR TKI with potent activity against exon 20 insertion mutations in preclinical studies, however with limited efficacy in ERBB2-mutated lung cancer (59). Zipalertinib fits into the ATP binding site of the EGFR hinge region, conferring its antitumor activity (158). Currently, the drug is being evaluated in an international phase I/II trial (159), with interim results suggestive of an ORR around 40% (159). Rash was present in more than half of the study participants; conversely, diarrhea appears less common than with other EGFR TKIs (159). Additionally, a phase II trial for EGFR exon 20 insertion-mutated NSCLC and phase III trial against standard-of-care chemotherapy in the first line setting are planned.
Other EGFR exon 20 insertion mutant active TKIs undergoing initial clinical trial development include BLU-451 and BAY2927088.
Platinum doublet chemotherapy and ICIs
Even despite the ongoing advances in targeted therapies outlined above, the evidence-based standard of care for first line therapy in advanced NSCLC harboring EGFR or ERBB2 exon 20 insertion mutations remains platinum-based chemotherapy, with or without an ICI even as outcomes have remained stagnant and generally modest (39). In a large retrospective review of 105 patients receiving first line platinum doublet chemotherapy, an ORR 19.2% and median PFS of 6.5 months were noted (160-165). Another retrospective study of 77 patients receiving first line pemetrexed (alone or in combination with a platinum agent) reported a better ORR of 41.5%, however again with limited median PFS of 7.6 months and median OS of 25 months (161). In the second line setting, responses to single agent chemotherapy have been reported with similarly limited efficacy and median PFS of 4.0 months (95% CI: 3.2–4.8 months) (160).
In a recent retrospective cohort of advanced NSCLC with EGFR exon 20 insertion mutations receiving chemotherapy as either first or second line treatment was reported (162). Amongst 41 patients receiving first line platinum-based treatment, median PFS was 5.7 months (95% CI: 3.0–10.9 months); for those receiving ICI in addition to chemotherapy, a median PFS of 4.5 months (1.2–10.3 months) was reported, though the subset was small (n=16). Of the 50 patients who received chemotherapy in the second line, median PFS was 3.3 months (95% CI: 2.3–5.9 months) (162). Notably, reported outcomes with chemotherapy-based regimens have superseded those seen with 1st and 2nd generation EGFR TKIs in this molecularly-defined subgroup, where median PFS for this earlier generation targeted agents have not exceeded 3 months (160).
The largest study was a multi-center retrospective evaluation of 93 patients who received platinum-based chemotherapy. The ORR was 43.5% and median PFS was 6.0 months (95% CI: 5.0–7.1 months). Outcomes of second line chemotherapy were not specifically shown in this cohort (163). A second smaller study included 25 patients that received platinum/pemetrexed in the first line, with an ORR of 36% and median PFS of 5.1 months (95% CI: 4.9–5.3 months) (164). A retrospective study of NSCLC patients with either EGFR or ERBB2 exon 20 insertion mutations reported a median PFS of 6 months (95% CI: 5.7–7.0 months) with first-line platinum-containing chemotherapies (162).
Outcomes with contemporary regimens including the addition of ICI to platinum doublet chemotherapy are still being vetted, and there have been very few case series evaluating the efficacy of ICIs advanced NSCLC with either EGFR or ERBB2 exon 20 insertion mutations (162-165). The largest study is a retrospective evaluation of 15 patients with EGFR exon 20-mutated advanced NSCLC who received ICI (either as first or second line treatment). Limited efficacy was noted with ORR 6.7%, median PFS of 2.0 months (95% CI: 0.6–2.7 months), and median OS of 5.3 months (95% CI: 1.8–12.5 months). There was a trend towards decreased OS (12.9 vs. 25.2 months, P=0.08) in those receiving vs. not receiving ICI therapy compared to EGFR exon 20 insertion-mutated NSCLCs that never received immunotherapy. In this limited sample as has been true elsewhere in the literature surrounding ICI use in EGFR-mutated NSCLC, tumor PD-L1 expression does not appear predictive of treatment response (165). In a retrospective, single-center cohort study of 122 ERBB2-mutated advanced outcomes of 26 patients receiving ICI therapy were evaluated. In the ICI-treated cohort, the ORR with was 12% (95% CI: 3–30%), median PFS 1.9 months (95% CI: 1.5–4.0 months) and median OS 10.4 months (95% CI: 5.9–NR) (166).
Additional data can be extrapolated from larger retrospective studies evaluating ICIs in a pooled analysis of oncogene-driven NSCLCs. A multi-center study included two groups of ERBB2-mutated NSCLC reported dismal responses to single agent ICI, with median PFS ranging from 1.9 months (95% CI: 1.6–2.1 months; n=15 patients from one center) to 3.0 months (95% CI: 1.8–NR; n=21 patients from a different center) (167). In another study, 29 patients who had received ICI therapy at any point in their treatment course were evaluated; ICI-associated ORR of 7% and median PFS of 2.5 months (95% CI: 1.8–3.5 months) were reported (168). In yet another retrospective study of 23 patients that receiving ICI therapy in the second line and beyond, showed reported improved ORR of 27.3%, though with still poor median PFS of 2.2 months (1.7–15.2 months) (169).
Similar to what has been previously described in other EGFR-mutated NSCLC subgroups (170,171), these findings raise important questions regarding the relevance of ICI-based approaches in patients whose tumors harbor exon 20 alterations in EGFR and ERBB2. It is postulated that limited/absent tobacco exposure in these molecularly defined subgroups may render an immune microenvironment that is not favorable for engagement of the immune system. ICI use (particularly as a sole agent) in these patients should be used with caution, possibly deferring until other targeted and/or chemotherapeutic strategies have been exhausted first.
Conclusions
Evolving insights into the biologic importance of oncogenic driver pathways and opportunities for novel drug development have led to brisk advances in the identification and management of oncogene-driven NSCLCs, thus linking scientific discovery in the lab to innovations in the clinic that have transformed the care of many patients. However, due to unique challenges stemming from protein structure and biology, leveraging the power of targeted therapeutics for an important molecularly-defined subset—those with EGFR and ERBB2 exon 20 insertion mutations—has lagged beyond others of its kind and represents an ongoing albeit evolving area of unmet need. Identifying agents that possess the hallmark characteristics of other targeted therapies already available for use in advanced NSCLC—deep, durable, and tolerable efficacy—has proven challenging in this molecularly-defined subset and has necessitated diversification of the targeted therapeutics armamentarium, including development of novel EGFR-directed TKIs, bivalent antibodies, and ADCs.
This review provides an overview of EGFR and ERBB2 mutations in NSCLC with a focus on the recent clinical approvals of mobocertinib, amivantamab, and T-DXd that have added to the armamentarium of precision oncology. Table 2 summarizes the major clinical studies of the EGFR TKI mobocertinib, EGFR-MET antibody amivantamab, and anti-ERBB2 ADC T-DXd, with a focus on efficacy and toxicity. Though promising efficacy (even in treatment-refractory disease) and manageable toxicities have been demonstrated in studies to date, currently ongoing and future studies will hopefully shed light on ways to optimize treatment selection, dosing, and sequencing so as to maximize outcomes for these important subsets of patients with advanced NSCLCs harboring EGFR exon 20 insertion mutations and ERBB2 mutations.
Table 2
Treatment (type) | Mutation | Ref. | Type of study | No. of patients | ORR (%) [95% CI] | mPFS (months) [95% CI] | mOS (months) [95% CI] | Common adverse events (CTCAE) | CTCAE grade ≥3 adverse events |
---|---|---|---|---|---|---|---|---|---|
Mobocertinib (covalent EGFR ex20ins active TKI) | EGFR ex20ins, ERBB2 ex20ins | (130) | Phase I/II | 28 | 43 [24–63] | 7.3 [4.4–15.6] | – | Diarrhea, nausea/vomiting, rash | Diarrhea, nausea, stomatitis |
EGFR ex20ins | (131) | Phase I/II | Platinum-pretreated patients: 114 | 28 [20–37] | 7.3 [5.5–9.2] | 24 [14.6–28.8] | Diarrhea, rash, paronychia, anorexia, nausea/vomiting | Diarrhea, stomatitis | |
Amivantamab (bi-specific EGFR-MET antibody) | EGFR ex20ins | (137) | Phase I | Efficacy: 81; safety: 114 | 36 [25–47] | 8.3 [5.5–10.6] | 22.8 [14.6–NR] | Rash, infusion-related reaction, paronychia, constipation | Hypokalemia, diarrhea, rash |
Trastuzumab deruxtecan (ERBB2 antibody-drug conjugate) | ERBB2 mutations | (143) | Phase I | Ex20ins: 8; other: 3 | 73 [39–94] | 11.3 [8.1–14.3] | 17.3 [17.3–NR] | Cytopenias, nausea/vomiting, diarrhea, anorexia, fatigue, alopecia | Anemia, neutropenia, thrombocytopenia, nausea, pneumonitis |
ERBB2 mutations | (112) | Phase II | Total: 91; Ex20ins: 78 | 55 [44–65] | 8.2 [6–11.9] | 17.8 [13.8–22.1] | Cytopenias, nausea/vomiting, fatigue, alopecia, diarrhea | Anemia, neutropenia, nausea, pneumonitis |
EGFR, epidermal growth factor receptor; ERBB2, erb-b2 receptor tyrosine kinase 2 (also known as HER2, human epidermal growth factor receptor-2); ORR, objective response rate; CI, confidence interval; mPFS, median progression-free survival; mOS, median overall survival; CTCAE, Common Terminology Criteria for Adverse Events; ex20ins, exon 20 insertion mutations; TKI, tyrosine kinase inhibitor; MET, MET proto-oncogene, receptor tyrosine kinase; ref., reference; NR, not reached.
Acknowledgments
Funding: This work was funded in part through National Institutes of Health (NIH)/National Cancer Institute (NCI) grants R37 CA218707 (to DB Costa) and R01 CA240257 (to SS Kobayashi).
Footnote
Reporting Checklist: The authors have completed the Narrative Review reporting checklist. Available at https://tlcr.amegroups.com/article/view/10.21037/tlcr-23-98/rc
Peer Review File: Available at https://tlcr.amegroups.com/article/view/10.21037/tlcr-23-98/prf
Conflicts of Interest: All authors have completed the ICMJE uniform disclosure form (available at https://tlcr.amegroups.com/article/view/10.21037/tlcr-23-98/coif). PVL serves as an unpaid editorial board member of Translational Lung Cancer Research from October 2019 to September 2023. DR reports receiving personal fees (consulting fees and honoraria) from TelaDoc Health, DynaMed, and Astra Zeneca; nonfinancial support (institutional research support) from Bristol-Myers Squibb, Novocure, and Abbvie/Stemcentrx; all outside the submitted work. SSK reports research support from Boehringer Ingelheim, MiRXES, Johnson&Johnson, and Taiho Therapeutics, as well as personal fees (honoraria) from AstraZeneca, Boehringer Ingelheim, Bristol Meyers Squibb, Chugai Pharmaceutical, and Takeda Pharmaceuticals, plus royalties from Life Technologies; all outside the submitted work. SSK was funded in part through NIH/NCI grant R01CA240257. PVL has received consulting fees for pathology services for Gala Therapeutics, Galvanize Therapeutics, Intuitive Surgical, and Ruby Robotics; all unrelated to the content of the current manuscript. PVL is the Editor-in-Chief of the Journal of the American Society of Cytopathology. DBC reports receiving consulting fees and honoraria from Takeda/Millennium Pharmaceuticals, AstraZeneca, Pfizer, Blueprint Medicines, and Janssen; institutional research support from Takeda/Millennium Pharmaceuticals, AstraZeneca, Pfizer, Merck Sharp and Dohme, Merrimack Pharmaceuticals, Bristol Myers Squibb, Clovis Oncology, Spectrum Pharmaceuticals, Tesaro and Daiichi Sankyo, and consulting fees from Teladoc and Grand Rounds by Included Health plus royalties from Life Technologies; all outside the submitted work. DBC was funded in part through NIH/NCI grant R37CA218707. The other authors have no conflicts of interest to declare.
Ethical Statement: The authors are accountable for all aspects of the work in ensuring that questions related to the accuracy or integrity of any part of the work are appropriately investigated and resolved.
Open Access Statement: This is an Open Access article distributed in accordance with the Creative Commons Attribution-NonCommercial-NoDerivs 4.0 International License (CC BY-NC-ND 4.0), which permits the non-commercial replication and distribution of the article with the strict proviso that no changes or edits are made and the original work is properly cited (including links to both the formal publication through the relevant DOI and the license). See: https://creativecommons.org/licenses/by-nc-nd/4.0/.
References
- Howlader N, Forjaz G, Mooradian MJ, et al. The Effect of Advances in Lung-Cancer Treatment on Population Mortality. N Engl J Med 2020;383:640-9. [Crossref] [PubMed]
- Swanton C, Govindan R. Clinical Implications of Genomic Discoveries in Lung Cancer. N Engl J Med 2016;374:1864-73. [Crossref] [PubMed]
- Reck M, Rabe KF. Precision Diagnosis and Treatment for Advanced Non-Small-Cell Lung Cancer. N Engl J Med 2017;377:849-61. [Crossref] [PubMed]
- Kwak EL, Bang YJ, Camidge DR, et al. Anaplastic lymphoma kinase inhibition in non-small-cell lung cancer. N Engl J Med 2010;363:1693-703. [Crossref] [PubMed]
- Shaw AT, Ou SH, Bang YJ, et al. Crizotinib in ROS1-rearranged non-small-cell lung cancer. N Engl J Med 2014;371:1963-71. [Crossref] [PubMed]
- VanderLaan PA, Rangachari D, Costa DB. Lung Cancer with a High Tumor Mutational Burden. N Engl J Med 2018;379:1093. [Crossref] [PubMed]
- Nicholson AG, Tsao MS, Beasley MB, et al. The 2021 WHO Classification of Lung Tumors: Impact of Advances Since 2015. J Thorac Oncol 2022;17:362-87. [Crossref] [PubMed]
- Travis WD, Brambilla E, Noguchi M, et al. International association for the study of lung cancer/american thoracic society/european respiratory society international multidisciplinary classification of lung adenocarcinoma. J Thorac Oncol 2011;6:244-85. [Crossref] [PubMed]
- Rami-Porta R, Bolejack V, Giroux DJ, et al. The IASLC lung cancer staging project: the new database to inform the eighth edition of the TNM classification of lung cancer. J Thorac Oncol 2014;9:1618-24.
- VanderLaan PA, Rangachari D, Majid A, et al. Tumor biomarker testing in non-small-cell lung cancer: A decade of change. Lung Cancer 2018;116:90-5. [Crossref] [PubMed]
- Kris MG, Johnson BE, Berry LD, et al. Using multiplexed assays of oncogenic drivers in lung cancers to select targeted drugs. JAMA 2014;311:1998-2006. [Crossref] [PubMed]
- ICGC/TCGA Pan-Cancer Analysis of Whole Genomes Consortium. Pan-cancer analysis of whole genomes. Nature 2020;578:82-93. [Crossref] [PubMed]
- VanderLaan PA, Rangachari D, Costa DB. The rapidly evolving landscape of biomarker testing in non-small cell lung cancer. Cancer Cytopathol 2021;129:179-81. [Crossref] [PubMed]
- Rangachari D, VanderLaan PA, Shea M, et al. Correlation between Classic Driver Oncogene Mutations in EGFR, ALK, or ROS1 and 22C3-PD-L1 ≥50% Expression in Lung Adenocarcinoma. J Thorac Oncol 2017;12:878-83. [Crossref] [PubMed]
- Torous VF, Rangachari D, Gallant BP, et al. PD-L1 testing using the clone 22C3 pharmDx kit for selection of patients with non-small cell lung cancer to receive immune checkpoint inhibitor therapy: are cytology cell blocks a viable option? J Am Soc Cytopathol 2018;7:133-41. [Crossref] [PubMed]
- Chen Z, Fillmore CM, Hammerman PS, et al. Non-small-cell lung cancers: a heterogeneous set of diseases. Nat Rev Cancer 2014;14:535-46. [Crossref] [PubMed]
- Costa DB. Kinase inhibitor-responsive genotypes in EGFR mutated lung adenocarcinomas: moving past common point mutations or indels into uncommon kinase domain duplications and rearrangements. Transl Lung Cancer Res 2016;5:331-7. [Crossref] [PubMed]
- Arcila ME, Nafa K, Chaft JE, et al. EGFR exon 20 insertion mutations in lung adenocarcinomas: prevalence, molecular heterogeneity, and clinicopathologic characteristics. Mol Cancer Ther 2013;12:220-9. [Crossref] [PubMed]
- Yasuda H, Park E, Yun CH, et al. Structural, biochemical, and clinical characterization of epidermal growth factor receptor (EGFR) exon 20 insertion mutations in lung cancer. Sci Transl Med 2013;5:216ra177. [Crossref] [PubMed]
- Yasuda H, Kobayashi S, Costa DB. EGFR exon 20 insertion mutations in non-small-cell lung cancer: preclinical data and clinical implications. Lancet Oncol 2012;13:e23-31. [Crossref] [PubMed]
- Riess JW, Gandara DR, Frampton GM, et al. Diverse EGFR Exon 20 Insertions and Co-Occurring Molecular Alterations Identified by Comprehensive Genomic Profiling of NSCLC. J Thorac Oncol 2018;13:1560-8. [Crossref] [PubMed]
- Vyse S, Huang PH. Targeting EGFR exon 20 insertion mutations in non-small cell lung cancer. Signal Transduct Target Ther 2019;4:5. [Crossref] [PubMed]
- Robichaux JP, Elamin YY, Tan Z, et al. Mechanisms and clinical activity of an EGFR and HER2 exon 20-selective kinase inhibitor in non-small cell lung cancer. Nat Med 2018;24:638-46. [Crossref] [PubMed]
- Mishra R, Hanker AB, Garrett JT. Genomic alterations of ERBB receptors in cancer: clinical implications. Oncotarget 2017;8:114371-92. [Crossref] [PubMed]
- Stephens P, Hunter C, Bignell G, et al. Lung cancer: intragenic ERBB2 kinase mutations in tumours. Nature 2004;431:525-6. [Crossref] [PubMed]
- Shigematsu H, Takahashi T, Nomura M, et al. Somatic mutations of the HER2 kinase domain in lung adenocarcinomas. Cancer Res 2005;65:1642-6. [Crossref] [PubMed]
- Greulich H, Kaplan B, Mertins P, et al. Functional analysis of receptor tyrosine kinase mutations in lung cancer identifies oncogenic extracellular domain mutations of ERBB2. Proc Natl Acad Sci U S A 2012;109:14476-81. [Crossref] [PubMed]
- Chmielecki J, Ross JS, Wang K, et al. Oncogenic alterations in ERBB2/HER2 represent potential therapeutic targets across tumors from diverse anatomic sites of origin. Oncologist 2015;20:7-12. [Crossref] [PubMed]
- Arcila ME, Chaft JE, Nafa K, et al. Prevalence, clinicopathologic associations, and molecular spectrum of ERBB2 (HER2) tyrosine kinase mutations in lung adenocarcinomas. Clin Cancer Res 2012;18:4910-8. [Crossref] [PubMed]
- Zeng J, Ma W, Young RB, et al. Targeting HER2 genomic alterations in non-small cell lung cancer. J Natl Cancer Cent 2021;1:58-73. [Crossref]
- Mazières J, Peters S, Lepage B, et al. Lung cancer that harbors an HER2 mutation: epidemiologic characteristics and therapeutic perspectives. J Clin Oncol 2013;31:1997-2003. [Crossref] [PubMed]
- Hanahan D. Hallmarks of Cancer: New Dimensions. Cancer Discov 2022;12:31-46. [Crossref] [PubMed]
- Costa DB, Halmos B, Kumar A, et al. BIM mediates EGFR tyrosine kinase inhibitor-induced apoptosis in lung cancers with oncogenic EGFR mutations. PLoS Med 2007;4:1669-79; discussion 1680. [Crossref] [PubMed]
- Wahida A, Buschhorn L, Fröhling S, et al. The coming decade in precision oncology: six riddles. Nat Rev Cancer 2023;23:43-54. [Crossref] [PubMed]
- Cohen P, Cross D, Jänne PA. Kinase drug discovery 20 years after imatinib: progress and future directions. Nat Rev Drug Discov 2021;20:551-69. [Crossref] [PubMed]
- Jamal-Hanjani M, Wilson GA, McGranahan N, et al. Tracking the Evolution of Non-Small-Cell Lung Cancer. N Engl J Med 2017;376:2109-21. [Crossref] [PubMed]
- Bailey C, Black JRM, Reading JL, et al. Tracking Cancer Evolution through the Disease Course. Cancer Discov 2021;11:916-32. [Crossref] [PubMed]
- Singh N, Temin S, Baker S Jr, et al. Therapy for Stage IV Non-Small-Cell Lung Cancer With Driver Alterations: ASCO Living Guideline. J Clin Oncol 2022;40:3310-22. [Crossref] [PubMed]
- Ettinger DS, Wood DE, Aisner DL, et al. Non-Small Cell Lung Cancer, Version 3.2022, NCCN Clinical Practice Guidelines in Oncology. J Natl Compr Canc Netw 2022;20:497-530. [Crossref] [PubMed]
- Izumi H, Matsumoto S, Liu J, et al. The CLIP1-LTK fusion is an oncogenic driver in non-small-cell lung cancer. Nature 2021;600:319-23. [Crossref] [PubMed]
- Pao W, Miller V, Zakowski M, et al. EGF receptor gene mutations are common in lung cancers from "never smokers" and are associated with sensitivity of tumors to gefitinib and erlotinib. Proc Natl Acad Sci U S A 2004;101:13306-11. [Crossref] [PubMed]
- Paez JG, Jänne PA, Lee JC, et al. EGFR mutations in lung cancer: correlation with clinical response to gefitinib therapy. Science 2004;304:1497-500. [Crossref] [PubMed]
- Lynch TJ, Bell DW, Sordella R, et al. Activating mutations in the epidermal growth factor receptor underlying responsiveness of non-small-cell lung cancer to gefitinib. N Engl J Med 2004;350:2129-39. [Crossref] [PubMed]
- Yamaguchi N, Vanderlaan PA, Folch E, et al. Smoking status and self-reported race affect the frequency of clinically relevant oncogenic alterations in non-small-cell lung cancers at a United States-based academic medical practice. Lung Cancer 2013;82:31-7. [Crossref] [PubMed]
- Carrot-Zhang J, Soca-Chafre G, Patterson N, et al. Genetic Ancestry Contributes to Somatic Mutations in Lung Cancers from Admixed Latin American Populations. Cancer Discov 2021;11:591-8. [Crossref] [PubMed]
- Imielinski M, Berger AH, Hammerman PS, et al. Mapping the hallmarks of lung adenocarcinoma with massively parallel sequencing. Cell 2012;150:1107-20. [Crossref] [PubMed]
- Jorge SE, Kobayashi SS, Costa DB. Epidermal growth factor receptor (EGFR) mutations in lung cancer: preclinical and clinical data. Braz J Med Biol Res 2014;47:929-39. [Crossref] [PubMed]
- Oxnard GR, Nguyen KS, Costa DB. Germline mutations in driver oncogenes and inherited lung cancer risk independent of smoking history. J Natl Cancer Inst 2014;106:djt361. [Crossref] [PubMed]
- Sehgal K, Rangachari D, VanderLaan PA, et al. Clinical Benefit of Tyrosine Kinase Inhibitors in Advanced Lung Cancer with EGFR-G719A and Other Uncommon EGFR Mutations. Oncologist 2021;26:281-7. [Crossref] [PubMed]
- He M, Capelletti M, Nafa K, et al. EGFR exon 19 insertions: a new family of sensitizing EGFR mutations in lung adenocarcinoma. Clin Cancer Res 2012;18:1790-7. [Crossref] [PubMed]
- Ackerman A, Goldstein MA, Kobayashi S, et al. EGFR delE709_T710insD: a rare but potentially EGFR inhibitor responsive mutation in non-small-cell lung cancer. J Thorac Oncol 2012;7:e19-20. [Crossref] [PubMed]
- Kobayashi Y, Togashi Y, Yatabe Y, et al. EGFR Exon 18 Mutations in Lung Cancer: Molecular Predictors of Augmented Sensitivity to Afatinib or Neratinib as Compared with First- or Third-Generation TKIs. Clin Cancer Res 2015;21:5305-13. [Crossref] [PubMed]
- Xu H, Yang G, Li W, et al. EGFR Exon 18 Mutations in Advanced Non-Small Cell Lung Cancer: A Real-World Study on Diverse Treatment Patterns and Clinical Outcomes. Front Oncol 2021;11:713483. [Crossref] [PubMed]
- Du Z, Brown BP, Kim S, et al. Structure-function analysis of oncogenic EGFR Kinase Domain Duplication reveals insights into activation and a potential approach for therapeutic targeting. Nat Commun 2021;12:1382. [Crossref] [PubMed]
- Konduri K, Gallant JN, Chae YK, et al. EGFR Fusions as Novel Therapeutic Targets in Lung Cancer. Cancer Discov 2016;6:601-11. [Crossref] [PubMed]
- Jorge SE, Lucena-Araujo AR, Yasuda H, et al. EGFR Exon 20 Insertion Mutations Display Sensitivity to Hsp90 Inhibition in Preclinical Models and Lung Adenocarcinomas. Clin Cancer Res 2018;24:6548-55. [Crossref] [PubMed]
- Gonzalvez F, Vincent S, Baker TE, et al. Mobocertinib (TAK-788): A Targeted Inhibitor of EGFR Exon 20 Insertion Mutants in Non-Small Cell Lung Cancer. Cancer Discov 2021;11:1672-87. [Crossref] [PubMed]
- Vasconcelos PENS, Kobayashi IS, Kobayashi SS, et al. Preclinical characterization of mobocertinib highlights the putative therapeutic window of this novel EGFR inhibitor to EGFR exon 20 insertion mutations. JTO Clin Res Rep 2021;2:100105. [Crossref] [PubMed]
- Udagawa H, Hasako S, Ohashi A, et al. TAS6417/CLN-081 Is a Pan-Mutation-Selective EGFR Tyrosine Kinase Inhibitor with a Broad Spectrum of Preclinical Activity against Clinically Relevant EGFR Mutations. Mol Cancer Res 2019;17:2233-43. [Crossref] [PubMed]
- Vasconcelos PENS, Gergis C, Viray H, et al. EGFR-A763_Y764insFQEA Is a Unique Exon 20 Insertion Mutation That Displays Sensitivity to Approved and In-Development Lung Cancer EGFR Tyrosine Kinase Inhibitors. JTO Clin Res Rep 2020;1:100051. [Crossref] [PubMed]
- Kobayashi IS, Viray H, Rangachari D, et al. EGFR-D770>GY and Other Rare EGFR Exon 20 Insertion Mutations with a G770 Equivalence Are Sensitive to Dacomitinib or Afatinib and Responsive to EGFR Exon 20 Insertion Mutant-Active Inhibitors in Preclinical Models and Clinical Scenarios. Cells 2021;10:3561. [Crossref] [PubMed]
- Kosaka T, Tanizaki J, Paranal RM, et al. Response Heterogeneity of EGFR and HER2 Exon 20 Insertions to Covalent EGFR and HER2 Inhibitors. Cancer Res 2017;77:2712-21. [Crossref] [PubMed]
- Ji H, Li D, Chen L, et al. The impact of human EGFR kinase domain mutations on lung tumorigenesis and in vivo sensitivity to EGFR-targeted therapies. Cancer Cell 2006;9:485-95. [Crossref] [PubMed]
- Kobayashi S, Shimamura T, Monti S, et al. Transcriptional profiling identifies cyclin D1 as a critical downstream effector of mutant epidermal growth factor receptor signaling. Cancer Res 2006;66:11389-98. [Crossref] [PubMed]
- Engelman JA, Zejnullahu K, Gale CM, et al. PF00299804, an irreversible pan-ERBB inhibitor, is effective in lung cancer models with EGFR and ERBB2 mutations that are resistant to gefitinib. Cancer Res 2007;67:11924-32. [Crossref] [PubMed]
- Li D, Ambrogio L, Shimamura T, et al. BIBW2992, an irreversible EGFR/HER2 inhibitor highly effective in preclinical lung cancer models. Oncogene 2008;27:4702-11. [Crossref] [PubMed]
- Yun CH, Mengwasser KE, Toms AV, et al. The T790M mutation in EGFR kinase causes drug resistance by increasing the affinity for ATP. Proc Natl Acad Sci U S A 2008;105:2070-5. [Crossref] [PubMed]
- Zhou W, Ercan D, Chen L, et al. Novel mutant-selective EGFR kinase inhibitors against EGFR T790M. Nature 2009;462:1070-4. [Crossref] [PubMed]
- Yun CH, Boggon TJ, Li Y, et al. Structures of lung cancer-derived EGFR mutants and inhibitor complexes: mechanism of activation and insights into differential inhibitor sensitivity. Cancer Cell 2007;11:217-27. [Crossref] [PubMed]
- Mok TS, Wu Y-L, Ahn M-J, et al. Osimertinib or Platinum-Pemetrexed in EGFR T790M-Positive Lung Cancer. N Engl J Med 2017;376:629-40. [Crossref] [PubMed]
- Mok TS, Wu YL, Thongprasert S, et al. Gefitinib or carboplatin-paclitaxel in pulmonary adenocarcinoma. N Engl J Med 2009;361:947-57. [Crossref] [PubMed]
- Rosell R, Carcereny E, Gervais R, et al. Erlotinib versus standard chemotherapy as first-line treatment for European patients with advanced EGFR mutation-positive non-small-cell lung cancer (EURTAC): a multicentre, open-label, randomised phase 3 trial. Lancet Oncol 2012;13:239-46. [Crossref] [PubMed]
- Sequist LV, Yang JC, Yamamoto N, et al. Phase III study of afatinib or cisplatin plus pemetrexed in patients with metastatic lung adenocarcinoma with EGFR mutations. J Clin Oncol 2013;31:3327-34. [Crossref] [PubMed]
- Wu YL, Cheng Y, Zhou X, et al. Dacomitinib versus gefitinib as first-line treatment for patients with EGFR-mutation-positive non-small-cell lung cancer (ARCHER 1050): a randomised, open-label, phase 3 trial. Lancet Oncol 2017;18:1454-66. [Crossref] [PubMed]
- Jänne PA, Yang JC, Kim DW, et al. AZD9291 in EGFR inhibitor-resistant non-small-cell lung cancer. N Engl J Med 2015;372:1689-99. [Crossref] [PubMed]
- Soria JC, Ohe Y, Vansteenkiste J, et al. Osimertinib in Untreated EGFR-Mutated Advanced Non-Small-Cell Lung Cancer. N Engl J Med 2018;378:113-25. [Crossref] [PubMed]
- Costa DB, Kobayashi S, Tenen DG, et al. Pooled analysis of the prospective trials of gefitinib monotherapy for EGFR-mutant non-small cell lung cancers. Lung Cancer 2007;58:95-103. [Crossref] [PubMed]
- Wu YL, Tsuboi M, He J, et al. Osimertinib in Resected EGFR-Mutated Non-Small-Cell Lung Cancer. N Engl J Med 2020;383:1711-23. [Crossref] [PubMed]
- Ramalingam SS, Vansteenkiste J, Planchard D, et al. Overall Survival with Osimertinib in Untreated, EGFR-Mutated Advanced NSCLC. N Engl J Med 2020;382:41-50. [Crossref] [PubMed]
- Robichaux JP, Le X, Vijayan RSK, et al. Structure-based classification predicts drug response in EGFR-mutant NSCLC. Nature 2021;597:732-7. [Crossref] [PubMed]
- Shi Y, Au JS, Thongprasert S, et al. A prospective, molecular epidemiology study of EGFR mutations in Asian patients with advanced non-small-cell lung cancer of adenocarcinoma histology (PIONEER). J Thorac Oncol 2014;9:154-62. [Crossref] [PubMed]
- Yang JC, Sequist LV, Geater SL, et al. Clinical activity of afatinib in patients with advanced non-small-cell lung cancer harbouring uncommon EGFR mutations: a combined post-hoc analysis of LUX-Lung 2, LUX-Lung 3, and LUX-Lung 6. Lancet Oncol 2015;16:830-8. [Crossref] [PubMed]
- Cho JH, Lim SH, An HJ, et al. Osimertinib for Patients With Non-Small-Cell Lung Cancer Harboring Uncommon EGFR Mutations: A Multicenter, Open-Label, Phase II Trial (KCSG-LU15-09). J Clin Oncol 2020;38:488-95. [Crossref] [PubMed]
- Bar J, Peled N, Schokrpur S, et al. UNcommon EGFR Mutations: International Case Series on Efficacy of Osimertinib in Real-Life Practice in First-LiNe Setting (UNICORN). J Thorac Oncol 2023;18:169-80. [Crossref] [PubMed]
- Hirano T, Yasuda H, Tani T, et al. In vitro modeling to determine mutation specificity of EGFR tyrosine kinase inhibitors against clinically relevant EGFR mutants in non-small-cell lung cancer. Oncotarget 2015;6:38789-803. [Crossref] [PubMed]
- Wang M, Yang JC, Mitchell PL, et al. Sunvozertinib, a Selective EGFR Inhibitor for Previously Treated Non-Small Cell Lung Cancer with EGFR Exon 20 Insertion Mutations. Cancer Discov 2022;12:1676-89. [Crossref] [PubMed]
- Mukohara T, Engelman JA, Hanna NH, et al. Differential effects of gefitinib and cetuximab on non-small-cell lung cancers bearing epidermal growth factor receptor mutations. J Natl Cancer Inst 2005;97:1185-94. [Crossref] [PubMed]
- Goldberg SB, Redman MW, Lilenbaum R, et al. Randomized Trial of Afatinib Plus Cetuximab Versus Afatinib Alone for First-Line Treatment of EGFR-Mutant Non-Small-Cell Lung Cancer: Final Results From SWOG S1403. J Clin Oncol 2020;38:4076-85. [Crossref] [PubMed]
- Yun J, Lee SH, Kim SY, et al. Antitumor Activity of Amivantamab (JNJ-61186372), an EGFR-MET Bispecific Antibody, in Diverse Models of EGFR Exon 20 Insertion-Driven NSCLC. Cancer Discov 2020;10:1194-209. [Crossref] [PubMed]
- Moores SL, Chiu ML, Bushey BS, et al. A novel bispecific antibody targeting EGFR and cMet is effective against EGFR inhibitor-resistant lung tumors. Cancer Res 2016;76:3942-53. [Crossref] [PubMed]
- Neijssen J, Cardoso RMF, Chevalier KM, et al. Discovery of amivantamab (JNJ-61186372), a bispecific antibody targeting EGFR and MET. J Biol Chem 2021;296:100641. [Crossref] [PubMed]
- Syed YY. Amivantamab: First Approval. Drugs 2021;81:1349-53. [Crossref] [PubMed]
- Jänne PA, Baik C, Su WC, et al. Efficacy and Safety of Patritumab Deruxtecan (HER3-DXd) in EGFR Inhibitor-Resistant, EGFR-Mutated Non-Small Cell Lung Cancer. Cancer Discov 2022;12:74-89. [Crossref] [PubMed]
- Riudavets M, Sullivan I, Abdayem P, et al. Targeting HER2 in non-small-cell lung cancer (NSCLC): a glimpse of hope? An updated review on therapeutic strategies in NSCLC harbouring HER2 alterations. ESMO Open 2021;6:100260. [Crossref] [PubMed]
- Herter-Sprie GS, Greulich H, Wong KK. Activating Mutations in ERBB2 and Their Impact on Diagnostics and Treatment. Front Oncol 2013;3:86. [Crossref] [PubMed]
- Uy NF, Merkhofer CM, Baik CS. HER2 in Non-Small Cell Lung Cancer: A Review of Emerging Therapies. Cancers (Basel) 2022;14:4155. [Crossref] [PubMed]
- Yu X, Ji X, Su C. HER2-Altered Non-Small Cell Lung Cancer: Biology, Clinicopathologic Features, and Emerging Therapies. Front Oncol 2022;12:860313. [Crossref] [PubMed]
- Rolfo C, Russo A. HER2 Mutations in Non-Small Cell Lung Cancer: A Herculean Effort to Hit the Target. Cancer Discov 2020;10:643-5. [Crossref] [PubMed]
- Passaro A, Peters S. Targeting HER2-Mutant NSCLC - The Light Is On. N Engl J Med 2022;386:286-9. [Crossref] [PubMed]
- Li BT, Ross DS, Aisner DL, et al. HER2 Amplification and HER2 Mutation Are Distinct Molecular Targets in Lung Cancers. J Thorac Oncol 2016;11:414-9. [Crossref] [PubMed]
- Costa DB, Jorge SE, Moran JP, et al. Pulse Afatinib for ERBB2 Exon 20 Insertion-Mutated Lung Adenocarcinomas. J Thorac Oncol 2016;11:918-23. [Crossref] [PubMed]
- Shen X, Chen B, Ma Z, et al. A systematic analysis of the resistance and sensitivity of HER2YVMA receptor tyrosine kinase mutant to tyrosine kinase inhibitors in HER2-positive lung cancer. J Recept Signal Transduct Res 2016;36:89-97. [Crossref] [PubMed]
- Perera SA, Li D, Shimamura T, et al. HER2YVMA drives rapid development of adenosquamous lung tumors in mice that are sensitive to BIBW2992 and rapamycin combination therapy. Proc Natl Acad Sci U S A 2009;106:474-9. [Crossref] [PubMed]
- Hyman DM, Piha-Paul SA, Won H, et al. HER kinase inhibition in patients with HER2- and HER3-mutant cancers. Nature 2018;554:189-94. [Crossref] [PubMed]
- Han H, Li S, Chen T, et al. Targeting HER2 exon 20 insertion-mutant lung adenocarcinoma with a novel tyrosine kinase inhibitor mobocertinib. Cancer Res 2021;81:5311-24. [Crossref] [PubMed]
- Zhao J, Xia Y. Targeting HER2 alterations in non-small-cell lung cancer: a comprehensive review. JCO Precis Oncol 2020;4:411-25. [Crossref] [PubMed]
- Lai WV, Lebas L, Barnes TA, et al. Afatinib in patients with metastatic or recurrent HER2-mutant lung cancers: a retrospective international multicentre study. Eur J Cancer 2019;109:28-35. [Crossref] [PubMed]
- Kris MG, Camidge DR, Giaccone G, et al. Targeting HER2 aberrations as actionable drivers in lung cancers: phase II trial of the pan-HER tyrosine kinase inhibitor dacomitinib in patients with HER2-mutant or amplified tumors. Ann Oncol 2015;26:1421-7. [Crossref] [PubMed]
- Elamin YY, Robichaux JP, Carter BW, et al. Poziotinib for Patients With HER2 Exon 20 Mutant Non-Small-Cell Lung Cancer: Results From a Phase II Trial. J Clin Oncol 2022;40:702-9. [Crossref] [PubMed]
- Le X, Cornelissen R, Garassino M, et al. Poziotinib in Non-Small-Cell Lung Cancer Harboring HER2 Exon 20 Insertion Mutations After Prior Therapies: ZENITH20-2 Trial. J Clin Oncol 2022;40:710-8. [Crossref] [PubMed]
- Oguchi K, Araki H, Tsuji S, et al. TAS2940, a novel brain-penetrable pan-ERBB inhibitor, for tumors with HER2 and EGFR aberrations. Cancer Sci 2023;114:654-64. [Crossref] [PubMed]
- Li BT, Shen R, Buonocore D, et al. Ado-Trastuzumab Emtansine for Patients With HER2-Mutant Lung Cancers: Results From a Phase II Basket Trial. J Clin Oncol 2018;36:2532-7. [Crossref] [PubMed]
- Li BT, Michelini F, Misale S, et al. HER2-Mediated Internalization of Cytotoxic Agents in ERBB2 Amplified or Mutant Lung Cancers. Cancer Discov 2020;10:674-87. [Crossref] [PubMed]
- Costa DB, Nguyen KS, Cho BC, et al. Effects of erlotinib in EGFR mutated non-small cell lung cancers with resistance to gefitinib. Clin Cancer Res 2008;14:7060-7. [Crossref] [PubMed]
- Kobayashi S, Boggon TJ, Dayaram T, et al. EGFR mutation and resistance of non-small-cell lung cancer to gefitinib. N Engl J Med 2005;352:786-92. [Crossref] [PubMed]
- Kobayashi S, Ji H, Yuza Y, et al. An alternative inhibitor overcomes resistance caused by a mutation of the epidermal growth factor receptor. Cancer Res 2005;65:7096-101. [Crossref] [PubMed]
- Nguyen KS, Kobayashi S, Costa DB. Acquired resistance to epidermal growth factor receptor tyrosine kinase inhibitors in non-small-cell lung cancers dependent on the epidermal growth factor receptor pathway. Clin Lung Cancer 2009;10:281-9. [Crossref] [PubMed]
- Costa DB, Kobayashi S, Pandya SS, et al. CSF concentration of the anaplastic lymphoma kinase inhibitor crizotinib. J Clin Oncol 2011;29:e443-5. [Crossref] [PubMed]
- Rangachari D, Yamaguchi N, VanderLaan PA, et al. Brain metastases in patients with EGFR-mutated or ALK-rearranged non-small-cell lung cancers. Lung Cancer 2015;88:108-11. [Crossref] [PubMed]
- Costa DB, Shaw AT, Ou SH, et al. Clinical experience with crizotinib in patients with advanced ALK-rearranged non-small-cell lung cancer and brain metastases. J Clin Oncol 2015;33:1881-8. [Crossref] [PubMed]
- Niederst MJ, Sequist LV, Poirier JT, et al. RB loss in resistant EGFR mutant lung adenocarcinomas that transform to small-cell lung cancer. Nat Commun 2015;6:6377. [Crossref] [PubMed]
- Zhang Z, Stiegler AL, Boggon TJ, et al. EGFR-mutated lung cancer: a paradigm of molecular oncology. Oncotarget 2010;1:497-514. [Crossref] [PubMed]
- Oxnard GR, Hu Y, Mileham KF, et al. Assessment of resistance mechanisms and clinical implications in patients with EGFR T790M-positive lung cancer and acquired resistance to osimertinib. JAMA Oncol 2018;4:1527-34. [Crossref] [PubMed]
- Choudhury NJ, Marra A, Sui JSY, et al. Molecular biomarkers of disease outcomes and mechanisms of acquired resistance to first-line osimertinib in advanced EGFR-mutant lung cancers. J Thorac Oncol 2023;18:463-75. [Crossref] [PubMed]
- Kagawa Y, Hayashida T, Liu J, et al. The EGFR C797S Mutation Confers Resistance to a Novel EGFR Inhibitor CLN-081 to EGFR Exon 20 Insertion Mutations. JTO Clin Res Rep 2023;4:100462. [Crossref] [PubMed]
- Koga T, Kobayashi Y, Tomizawa K, et al. Activity of a novel HER2 inhibitor, poziotinib, for HER2 exon 20 mutations in lung cancer and mechanism of acquired resistance: An in vitro study. Lung Cancer 2018;126:72-9. [Crossref] [PubMed]
- Wang J, Lam D, Yang J, et al. Discovery of mobocertinib, a new irreversible tyrosine kinase inhibitor indicated for the treatment of non-small-cell lung cancer harboring EGFR exon 20 insertion mutations. Med Chem Res 2022;31:1647-62. [Crossref] [PubMed]
- Huang WS, Li F, Gong Y, et al. Discovery of mobocertinib, a potent, oral inhibitor of EGFR exon 20 insertion mutations in non-small cell lung cancer. Bioorg Med Chem Lett 2023;80:129084. [Crossref] [PubMed]
- NCT02716116. A Phase 1/2 Study of the Safety, Pharmacokinetics, and Anti-Tumor Activity of the Oral EGFR/HER2 Inhibitor TAK-788 (AP32788) in Non-Small Cell Lung Cancer. clinicaltrials.gov; 2023. Accessed January 22, 2023. Available online: https://clinicaltrials.gov/ct2/show/NCT02716116
- Riely GJ, Neal JW, Camidge DR, et al. Activity and Safety of Mobocertinib (TAK-788) in Previously Treated Non-Small Cell Lung Cancer with EGFR Exon 20 Insertion Mutations from a Phase I/II Trial. Cancer Discov 2021;11:1688-99. [Crossref] [PubMed]
- Zhou C, Ramalingam SS, Kim TM, et al. Treatment Outcomes and Safety of Mobocertinib in Platinum-Pretreated Patients With EGFR Exon 20 Insertion-Positive Metastatic Non-Small Cell Lung Cancer: A Phase 1/2 Open-label Nonrandomized Clinical Trial. JAMA Oncol 2021;7:e214761. Erratum in: JAMA Oncol 2022;8:1359. [Crossref] [PubMed]
- Garcia Campelo MR, Zhou C, Ramalingam SS, et al. Mobocertinib (TAK-788) in EGFR Exon 20 Insertion+ Metastatic NSCLC: Patient-Reported Outcomes from EXCLAIM Extension Cohort. J Clin Med 2022;12:112. [Crossref] [PubMed]
- Yang JC, Zhou C, Jänne PA, et al. Characterization and management of adverse events observed with mobocertinib (TAK-788) treatment for EGFR exon 20 insertion-positive non-small cell lung cancer. Expert Rev Anticancer Ther 2023;23:95-106. [Crossref] [PubMed]
- Kian W, Christopoulos P, Remilah AA, et al. Real-world efficacy and safety of mobocertinib in EGFR exon 20 insertion-mutated lung cancer. Front Oncol 2022;12:1010311. [Crossref] [PubMed]
- NCT04129502. A Randomized Phase 3 Multicenter Open-Label Study to Compare the Efficacy of TAK-788 as First-Line Treatment Versus Platinum-Based Chemotherapy in Patients With Non-Small Cell Lung Cancer With EGFR Exon 20 Insertion Mutations. clinicaltrials.gov; 2022. Accessed January 22, 2023. Available online: https://clinicaltrials.gov/ct2/show/NCT04129502.
- NCT02609776. A Phase 1, First-in-Human, Open-Label, Dose Escalation Study of JNJ-61186372, a Human Bispecific EGFR and CMet Antibody, in Subjects With Advanced Non-Small Cell Lung Cancer. clinicaltrials.gov; 2023. Accessed January 22, 2023. Available online: https://clinicaltrials.gov/ct2/show/NCT02609776.
- Park K, Haura EB, Leighl NB, et al. Amivantamab in EGFR Exon 20 Insertion-Mutated Non-Small-Cell Lung Cancer Progressing on Platinum Chemotherapy: Initial Results From the CHRYSALIS Phase I Study. J Clin Oncol 2021;39:3391-402. [Crossref] [PubMed]
- NCT04077463. An Open-Label Phase 1/1b Study to Evaluate the Safety and Pharmacokinetics of JNJ-73841937 (Lazertinib), a Third Generation EGFR-TKI, as Monotherapy or in Combinations With JNJ-61186372, a Human Bispecific EGFR and CMet Antibody in Participants With Advanced Non-Small Cell Lung Cancer. clinicaltrials.gov; 2023. Accessed January 22, 2023. Available online: https://clinicaltrials.gov/ct2/show/NCT04077463
- NCT04538664. A Randomized, Open-Label Phase 3 Study of Combination Amivantamab and Carboplatin-Pemetrexed Therapy, Compared With Carboplatin-Pemetrexed, in Patients With EGFR Exon 20ins Mutated Locally Advanced or Metastatic Non-Small Cell Lung Cancer. clinicaltrials.gov; 2023. Accessed January 22, 2023. Available online: https://clinicaltrials.gov/ct2/show/NCT04538664.
- NCT04606381. An Open-Label, Multicenter, Dose Escalation Phase 1b Study to Assess the Safety and Pharmacokinetics of Subcutaneous Delivery of Amivantamab, a Human Bispecific EGFR and CMet Antibody for the Treatment of Advanced Solid Malignancies. clinicaltrials.gov; 2023. Accessed January 22, 2023. Available online: https://clinicaltrials.gov/ct2/show/NCT04606381
- Modi S, Saura C, Yamashita T, et al. Trastuzumab deruxtecan in previously treated HER2-positive breast cancer. N Engl J Med 2020;382:610-21. [Crossref] [PubMed]
- Ricciuti B, Lamberti G, Andrini E, et al. Antibody-drug conjugates for lung cancer in the era of personalized oncology. Semin Cancer Biol 2021;69:268-78. [Crossref] [PubMed]
- Tsurutani J, Iwata H, Krop I, et al. Targeting HER2 with Trastuzumab Deruxtecan: A Dose-Expansion, Phase I Study in Multiple Advanced Solid Tumors. Cancer Discov 2020;10:688-701. [Crossref] [PubMed]
- Li BT, Smit EF, Goto Y, et al. Trastuzumab Deruxtecan in HER2-Mutant Non-Small-Cell Lung Cancer. N Engl J Med 2022;386:241-51. [Crossref] [PubMed]
- Viray H, Rangachari D, Costa DB. Trastuzumab Deruxtecan in Non-Small-Cell Lung Cancer. N Engl J Med 2022;386:1769-70. [Crossref] [PubMed]
- Smit EFF, Li BT, Mazieres J, et al. 1361TiP Trastuzumab deruxtecan (T-DXd) in patients (pts) with HER2-mutated (HER2m) metastatic non-small cell lung cancer (NSCLC): A phase (ph) II study (DESTINY-Lung02). Ann Oncol 2021;32:S1032-S1033. [Crossref]
- Goto K, Sang-We K, Kubo T, et al. LBA55 Trastuzumab deruxtecan (T-DXd) in patients (Pts) with HER2-mutant metastatic non-small cell lung cancer (NSCLC): Interim results from the phase 2 DESTINY-Lung02 trial. Ann Oncol 2022;33:S1422. [Crossref]
- Swain SM, Nishino M, Lancaster LH, et al. Multidisciplinary clinical guidance on trastuzumab deruxtecan (T-DXd)-related interstitial lung disease/pneumonitis—focus on proactive monitoring, diagnosis, and management. Cancer Treat Rev 2022;106: [Crossref] [PubMed]
- NCT04686305. A Phase Ib Multicenter, Open-Label Dose-Escalation Study to Evaluate the Safety and Tolerability of Trastuzumab Deruxtecan (T-DXd) and Durvalumab in Combination With Cisplatin, Carboplatin or Pemetrexed in First-Line Treatment of Patients With Advanced or Metastatic Non-Squamous Non-Small Cell Lung Cancer (NSCLC) and Human Epidermal Growth Factor Receptor 2 Overexpression (HER2+). (DESTINY-Lung03). clinicaltrials.gov; 2023. Accessed January 26, 2023. Available online: https://clinicaltrials.gov/ct2/show/NCT04686305
- NCT05048797. An Open-Label, Randomized, Multicenter, Phase 3 Study to Assess the Efficacy and Safety of Trastuzumab Deruxtecan as First-Line Treatment of Unresectable, Locally Advanced, or Metastatic NSCLC Harboring HER2 Exon 19 or 20 Mutations (DESTINY-Lung04). clinicaltrials.gov; 2023. Accessed January 26, 2023. https://clinicaltrials.gov/ct2/show/NCT05048797
- Han JY, Lee KH, Kim SW, et al. A Phase II Study of Poziotinib in Patients with Epidermal Growth Factor Receptor (EGFR)-Mutant Lung Adenocarcinoma Who Have Acquired Resistance to EGFR-Tyrosine Kinase Inhibitors. Cancer Res Treat 2017;49:10-9. [Crossref] [PubMed]
- Elamin YY, Robichaux JP, Carter BW, et al. Poziotinib for EGFR exon 20-mutant NSCLC: Clinical efficacy, resistance mechanisms, and impact of insertion location on drug sensitivity. Cancer Cell 2022;40:754-767.e6. [Crossref] [PubMed]
- NCT03318939. A Phase 2 Study of Poziotinib in Patients With Non-Small Cell Lung Cancer (NSCLC), Locally Advanced or Metastatic, With EGFR or HER2 Exon 20 Insertion Mutation (ZENITH20). clinicaltrials.gov; 2022. Accessed January 26, 2023. Available online: https://clinicaltrials.gov/ct2/show/NCT03318939
- Cornelissen R, Sun S, Wollner M, et al. LBA46 Efficacy and safety of poziotinib in treatment-naïve NSCLC harboring HER2 exon 20 mutations: A multinational phase II study (ZENITH20-4). Ann Oncol 2021;32:S1324. [Crossref]
- Prelaj A, Bottiglieri A, Proto C, et al. Poziotinib for EGFR and HER2 exon 20 insertion mutation in advanced NSCLC: Results from the expanded access program. Eur J Cancer 2021;149:235-48. [Crossref] [PubMed]
- Spectrum Pharmaceuticals Receives Complete Response Letter from U.S. Food and Drug Administration for Poziotinib. Drugs.com. Accessed January 30, 2023. Available online: https://www.drugs.com/nda/poziotinib_221125.html
- NCT03974022. A Phase I/II, Open-Label, Multicenter Study to Assess the Safety, Tolerability, Pharmacokinetics and Anti-Tumor Efficacy of DZD9008 in Patients With Advanced Non-Small Cell Lung Cancer (NSCLC) With EGFR or HER2 Mutation. clinicaltrials.gov; 2023. Accessed January 26, 2023. Available online: https://clinicaltrials.gov/ct2/show/NCT03974022
- Hasako S, Terasaka M, Abe N, et al. TAS6417, A Novel EGFR Inhibitor Targeting Exon 20 Insertion Mutations. Mol Cancer Ther 2018;17:1648-58. [Crossref] [PubMed]
- Piotrowska Z, Tan DS, Smit EF, et al. Safety, tolerability, and antitumor activity of zipalertinib among patients with non-small-cell lung cancer harboring epidermal growth factor receptor exon 20 insertions. J Clin Oncol 2023; Epub ahead of print. [Crossref] [PubMed]
- Yang G, Li J, Xu H, et al. EGFR exon 20 insertion mutations in Chinese advanced non-small cell lung cancer patients: Molecular heterogeneity and treatment outcome from nationwide real-world study. Lung Cancer 2020;145:186-94. [Crossref] [PubMed]
- Xu CW, Wang WX, Wang D, et al. Pemetrexed-based chemotherapy for non-small-cell lung cancer patients with EGFR exon 20 insertion mutation: a multicenter study. Transl Lung Cancer Res 2020;9:1853-61. [Crossref] [PubMed]
- Ou SHI, Lin HM, Hong JL, et al. Real-world response and outcomes in NSCLC patients with EGFR exon 20 insertion mutations. J Clin Oncol 2021;39:abstr 9098.
- Mazières J, Barlesi F, Filleron T, et al. Lung cancer patients with HER2 mutations treated with chemotherapy and HER2-targeted drugs: results from the European EUHER2 cohort. Ann Oncol 2016;27:281-6. [Crossref] [PubMed]
- Wang Y, Zhang S, Wu F, et al. Outcomes of Pemetrexed-based chemotherapies in HER2-mutant lung cancers. BMC Cancer 2018;18:326. [Crossref] [PubMed]
- Metro G, Baglivo S, Bellezza G, et al. Sensitivity to Immune Checkpoint Blockade in Advanced Non-Small Cell Lung Cancer Patients with EGFR Exon 20 Insertion Mutations. Genes (Basel) 2021;12:679. [Crossref] [PubMed]
- Lai WCV, Feldman DL, Buonocore DJ, et al. PD-L1 expression, tumor mutation burden and response to immune checkpoint blockade in patients with HER2-mutant lung cancers. J Clin Oncol 2018;36:abstr 9060.
- Negrao MV, Skoulidis F, Montesion M, et al. Oncogene-specific differences in tumor mutational burden, PD-L1 expression, and outcomes from immunotherapy in non-small cell lung cancer. J Immunother Cancer 2021;9:e002891. [Crossref] [PubMed]
- Mazieres J, Drilon A, Lusque A, et al. Immune checkpoint inhibitors for patients with advanced lung cancer and oncogenic driver alterations: results from the IMMUNOTARGET registry. Ann Oncol 2019;30:1321-8. [Crossref] [PubMed]
- Guisier F, Dubos-Arvis C, Viñas F, et al. Efficacy and Safety of Anti-PD-1 Immunotherapy in Patients With Advanced NSCLC With BRAF, HER2, or MET Mutations or RET Translocation: GFPC 01-2018. J Thorac Oncol 2020;15:628-36. [Crossref] [PubMed]
- Gainor JF, Shaw AT, Sequist LV, et al. EGFR Mutations and ALK Rearrangements Are Associated with Low Response Rates to PD-1 Pathway Blockade in Non-Small Cell Lung Cancer: A Retrospective Analysis. Clin Cancer Res 2016;22:4585-93. [Crossref] [PubMed]
- Lee CK, Man J, Lord S, et al. Checkpoint inhibitors in metastatic EGFR-mutated non-small cell lung cancer-a meta-analysis. J Thorac Oncol 2017;12:403-7. [Crossref] [PubMed]