Augmented reality navigation-guided intraoperative pulmonary nodule localization: a pilot study
Highlight box
Key findings
• The augmented reality navigation-guided pulmonary nodule localization is a safe and feasible technique for the intraoperative localization of small lung nodules.
What is known and what is new?
• Preoperative CT-guided hookwire placement is the most used guiding method.
• We reported the safety and feasibility of a novel intraoperative pulmonary nodule localization method by using the augmented reality technology. This novel technique is promising for minimizing discomfort brought about during transportation of patient, and potential loss or displacement of the localizer.
What is the implication, and what should change now?
• The augmented reality navigation-guided localization technique is promising for localizing subpleural small lung nodules.
Introduction
With the widespread implementation of lung cancer screening programs and increased awareness of this cancer, an increasing number of people are benefiting from low-dose computed tomography (CT) screening. Several randomized trials have reported that low-dose CT screening can significantly reduce lung cancer mortality among high-risk persons (1-4). This screening also increases the detection of small lung nodules.
Traditionally, pulmonary nodules are recognized by palpation during surgery. However, small lesions located deep below the pleural surface or containing a high percentage of non-solid components are difficult to palpate, especially during video-assisted thoracoscopic surgery (VATS) (5). These difficulties emphasize the need for advanced techniques to localize small pulmonary nodules.
Stereotactic navigation is a widely used method in neurosurgery, spine surgery or bronchoscopic exam. By using computer vision (infra-red or visible light camera) or magnetic field to navigate surgical tools, and by registering pre-existing medical image (e.g., CT or magnetic resonance imaging) of the same patient who is under surgery, surgeon can operate with such surgical tool without the necessity to expose the lesion target. Unfortunately, although helpful, most navigation system is expensive and complex. A simple and convenient navigation system is expected.
Preoperative CT (POCT) and intraoperative CT (IOCT) are the two main categories of localizing small pulmonary nodules in clinical practice (6). POCT is represented by methods including dye marking, hookwire placement and coil labeling. CT-guided hookwire placement is the most commonly used POCT guiding method. However, it is limited by the risk of pneumothorax and prolonged patient’s discomfort. Compared with conventional POCT, the ability to localize lesions intraoperatively eliminates the discomfort brought about during transportation of patient to and from radiology department and the operating room. In addition, IOCT minimizes potential loss or displacement of the localizer (7,8). However, the need for a hybrid operating room and expensive medical equipment, such as 3-dimensional (3D) fluoroscopy, has limited the use of IOCT because this equipment is not available in most hospitals (9).
To overcome with these drawbacks and with the collaboration with Jedicare Medical Co., Ltd., we have developed a novel approach for the intraoperative localization of small lung nodules (10). With an augmented reality (AR) navigation system and a specialized lung localization marker, the benefits of IOCT were preserved while avoiding the need for complicated equipment and infrastructure. The feasibility of this novel AR navigation-guided localization has been preliminarily proved in animal experiment (10). This report further describes our system and provides early data regarding its feasibility and safety in patients with pulmonary nodules. We present this article in accordance with the TREND reporting checklist (available at https://tlcr.amegroups.com/article/view/10.21037/tlcr-23-201/rc).
Methods
Ethical statement
The Ethics Committee of Ruijin Hospital, Shanghai Jiao Tong University School of Medicine approved this study (No. 2019-206). The study was conducted in accordance with the Declaration of Helsinki (as revised in 2013). All patients provided written informed consent to perform all study-related procedures and for the publication of the medical data and relevant medical images. The study was registered at ClinicalTrials.gov (NCT04211051).
Patient enrollment
The study included ten patients who underwent AR-navigated localization of a pulmonary nodule at Ruijin Hospital, Shanghai Jiao Tong University School of Medicine between July 2020 and October 2020 (Figure 1). The inclusion criteria were as follows: (I) planned single nodule operation for a diagnosed pulmonary nodule with localization using our new technique, based on the decision of the chief surgeon; (II) nodule located in the outer third of the lung; (III) patient commitment to following the research procedures, cooperating with implementation of the entire research process and withdrawing from the clinical trial at any stage based on their own free will; and (IV) patient completion of a signed and dated informed consent form. The exclusion criteria were as follows: (I) target lesion near the hilum or large blood vessels; (II) forced expiratory volume in one second less than 1.2 L; (III) New York Association Heart functional class III or IV; (IV) presence of an uncontrolled acute pleura infection; and (V) history of thoracic surgery (thoracotomy), pleural infection, or pleural thickening and adhesions on the affected side.
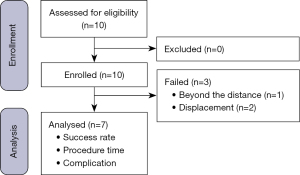
AR navigation system
The AR navigation system (JediVision; Jedicare Medical Co., Ltd, Shanghai, China) was run on both a Windows 10-based personal computer and a Hololens computer (Microsoft, Redmond, Washington) (Figure 2A). Optical video data were acquired by the imbedded camera of the Hololens computer and used for navigation. Objects with a specific 2D or 3D quick-response (QR) code (Figure 2B) pattern were located and tracked through these video data, and a 1.8-mm maximum position error was achieved in the lab when simulating the entire navigation procedure with a moving headset. During animal experiments, a 1.9-mm mean position error and 4.4-mm maximum position error were recorded from CT data of twelve navigated puncture attempts (10).
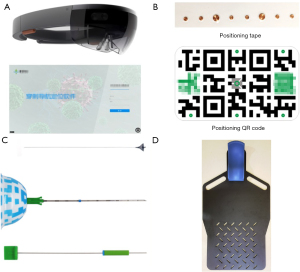
Lung localization marker
The marker (LungBrella; Jedicare Medical Co., Ltd, Shanghai, China) used for lung localization was a 6×8-mm umbrella-shaped braided nitinol marker with a tail, which was compressed and pre-loaded in an 18-G needle with a 3D patterned and video-trackable handle. After deployed inside the lung parenchyma, this marker would self-expand and lock within the lung tissue. The marker tail would remain outside the surface of the lung and could, therefore, be used to locate the marker by applying traction to the tail with forceps or clamps (Figure 2C). Given the metal material of the marker, it can be identified on CT or X-ray examination. The localization marker has been introduced in our preliminary animal experiment (10).
Localization and surgical procedure
Preoperatively, all patients underwent plain chest CT while lying on a CT table with the chest skin fully exposed. A position board was used to ensure consistency between the patient’s position during the CT scanning and the time of the navigational puncture (Figure 2D). Positioning tape was attached in the middle and on the lesion side of the chest, and the CT scan was performed during the exhalation stage. After the scan was completed, each metal marker was marked with a pen (skin mark point), the positioning tape was removed, and the patient returned to the ward. The CT data were uploaded into JediVision software to formulate the puncture plan. The expected anchor position of the marker and the skin entry point for the transthoracic puncture were determined manually on the personal computer. The navigation plan was subsequently transferred to the Hololens computer through a USB cable before the start of surgery.
The intraoperative localization procedure was performed as follows, with the patient receiving general anesthesia. After wearing the Hololens properly, the position of the QR code and the LungBrella needle were identified for quick position calibration. This process (identification and recording the position) was accomplished in just one second. Next, four software preselected registration points were recorded sequentially by the LungBrella needle tip touching the skin mark point. After this registration process was complete, the 3D virtual organs and targets were precisely matched with the patient’s body. Finally, the puncture was performed using AR guidance, and the marker was released after the needle reached the expected anchor position. After localization, the operation was performed as planned (Figures 3,4, Video 1).
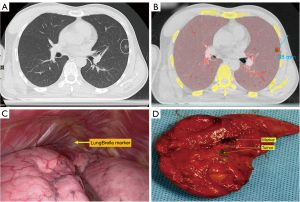
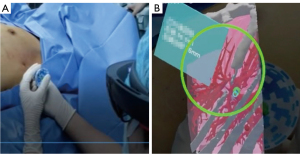
Evaluation
The primary endpoint of the study was the success rate of the localization procedure. A successful procedure was defined as localization meeting all these criteria: (I) distance between the LungBrella marker and pulmonary nodule less than 10 mm; (II) no displacement of the marker from the localization procedure to the operation; and (III) no errors related to the device during the procedure. The secondary endpoints were the procedure times (duration of localization and duration of operation) and the occurrence of perioperative complications.
Statistical analyses
Continuous data were expressed as median (range), and categorical data were described as number (percentage). The two-sided Student’s t-test was used for continuous variables, and chi-squared or Fisher exact tests were used for categorical variables. When the P value was less than 0.05, we considered the result to be statistically significant. All analyses were performed using SPSS 23 software (IBM Corp, Armonk, NY).
Results
Patient and lesion characteristics
A total of ten patients (with one nodule each) were enrolled in the study. Their characteristics are shown in Tables 1,2. The median nodule size was 10.0 mm (range, 7.2–16.2 mm), and the nodules were classified as ground-glass opacity (70%) or solid (30%) (Table 3). All target nodules were surgically removed after localization. Nine patients underwent segmentectomy, while the remaining patient underwent wedge resection. Three patients underwent robotic-assisted thoracic surgery and seven patients underwent VATS.
Table 1
Case No. | Time of operation (min) | Approach | Resection | Frozen pathology | Pathologic diagnosis | LN positive | Perioperative complications | Distance (mm) | Successful localization |
---|---|---|---|---|---|---|---|---|---|
1 | 160 | VATS | Segmentectomy | MIA | MIA | 0 | None | 5 | Yes |
2 | 150 | VATS | Segmentectomy | AAH | AAH | 0 | None | 5 | Yes |
3 | 150 | VATS | Segmentectomy | AAH | MIA | 0 | None | 3 | Yes |
4 | 200 | VATS | Segmentectomy | Hamartoma | Hamartoma | 0 | None | 12 | No |
5 | 140 | RATS | Segmentectomy | MIA | MIA | 0 | None | 10 | Yes |
6 | 165 | VATS | Segmentectomy | MIA | IAC | 0 | None | / | No |
7 | 105 | RATS | Segmentectomy | Adenocarcinoma | IAC | 0 | None | 10 | Yes |
8 | 105 | RATS | Segmentectomy | AIS | AIS | 0 | None | 0 | Yes |
9 | 200 | VATS | Segmentectomy | IAC | IAC | 0 | None | 8 | Yes |
10 | 60 | VATS | Wedge resection | MIA | MIA | 0 | None | / | No |
LN, lymph node; VATS, video-assisted thoracoscopic surgery; MIA, minimally invasive adenocarcinoma; AAH, atypical adenomatous hyperplasia; IAC, invasive adenocarcinoma; RATS, robotic-assisted thoracic surgery; AIS, adenocarcinoma in situ.
Table 2
Characteristics | Value |
---|---|
Sex, n [%] | |
Male | 5 [50] |
Female | 5 [50] |
Age, years, median [range] | 50 [39–64] |
Body mass index, kg/m2, median (range) | 22.86 (18.79–28.64) |
Smoking history, n [%] | |
Yes | 1 [10] |
No | 9 [90] |
Table 3
Characteristics | Value |
---|---|
Diameter, mm, median (range) | 10.0 (7.2–16.2) |
Nodule type, n [%] | |
Ground-glass opacity | 7 [70] |
Solid | 3 [30] |
Location, n [%] | |
Right upper lobe | 4 [40] |
Right middle lobe | 1 [10] |
Right lower lobe | 1 [10] |
Left upper lobe | 4 [40] |
Left lower lobe | 0 |
Resection, n [%] | |
Wedge resection | 1 [10] |
Segmentectomy | 9 [90] |
Lobectomy | 0 |
Approach, n [%] | |
VATS | 7 [70] |
RATS | 3 [30] |
Pathologic diagnosis, n [%] | |
Adenocarcinoma in situ | 1 [10] |
Minimally invasive adenocarcinoma | 4 [40] |
Invasive adenocarcinoma | 3 [30] |
Atypical adenomatous hyperplasia | 1 [10] |
Hamartoma | 1 [10] |
Stage, n [%] | |
Benign | 1 [10] |
0 | 1 [10] |
IA1 | 6 [60] |
IA2 | 2 [20] |
RATS, robotic-assisted thoracic surgery; VATS, video-assisted thoracoscopic surgery.
Procedure outcomes
All LungBrella markers were retrieved entirely from the resected specimens. Localization was successful in seven patients (Table 4). In these cases, the LungBrella marker was placed at a median distance of 5.8 mm (range, 0–10 mm) from the edge of the nodule. The median duration of the localization procedure was 9.4 min (range, 5–19 min), and the median duration of surgery was 172.9 min (range, 105–200 min).
Table 4
Characteristics | Value |
---|---|
Time of localization procedure, min, median [range] | 9.4 [5–19] |
Time of operation, min, median [range]† | 172.9 [105–200] |
Distance between LungBrella marker and nodule, mm, median [range] | 5.8 [0–10] |
Successful localization, n [%] | 7 [70] |
Unsuccessful localization, n [%] | 3 [30] |
Excessive distance between marker and nodule | 1 [10] |
Marker displacement | 2 [20] |
Perioperative complications, n [%] | |
Pneumothorax | 0 |
Intrapulmonary focal hemorrhage | 0 |
Pneumonia | 0 |
Atrial fibrillation | 0 |
†, only the 7 successful cases are included.
Localization was not successful in three cases (Case No. 4, No. 6 and No. 10, Table 1). Case No. 4 was due to a CT DICOM header parsing error after the CT scan switching to a different CT scanner. Failure of case No. 6 was caused by inaccurate 2D QR code registration in the complex operating room environment, and the third failure was the result of incorrect manipulation of the puncture needle. Marker of case No. 4 was found more than 10 mm superficial than the target lesion, which did not meet criteria 1. Markers of case No. 6 and No. 10 were all found at the surface of lung pleura, which were classified as failure of criteria 2. But all these three cases did not result in the removal of an excessive amount of lung tissue.
No complications occurred during the localization procedure or perioperatively. Specifically, no deaths, significant complications (pneumothorax, intrapulmonary hemorrhage, pneumonia, or atrial fibrillation), or device problems (fractures or faults) were observed in any patient. No postoperative recurrence or metastasis occurred during 2 years of follow-up.
Discussion
With the rapid development of low-dose spiral CT and extensive promotion of lung cancer screening programs, small pulmonary nodules are more easily and commonly detected in clinical practice, leading to increasing concerns among patients and physicians (2,11). Minimally invasive surgery is now the gold standard for removing these small nodules (12,13). Generally, VATS is applied on the resectable nodule, with subsequent pathologic examination performed to determine whether further lymph node dissection is needed. Intraoperatively, it is difficult and challenging to localize small pulmonary nodules by direct vision, especially when the nodules do not produce pleural indentation or discoloration. Localizing nodules by palpation of the pulmonary parenchyma is widely recognized as time-consuming and frustrating. Most importantly, failure to localize nodules may necessitate conversion to open thoracotomy. Suzuki’s team (14) reported that if a target nodule diameter is less than 10 mm and the distance from the pleura is more than 10 mm, the failure rate of nodule localization during VATS is nearly 100%, which is also believed to be the most common reason for conversion to thoracotomy.
Therefore, preoperative or intraoperative localization of small pulmonary nodules is important. Various image-guided methods have been used to localize these nodules, including dye injection, hookwire placement, and coil labeling, with each method exhibiting specific advantages and disadvantages (6,15-18). CT-guided hookwire placement is considered the most easy and efficient method, with an approximately 95% accuracy and an acceptable rate of complications (16). Nevertheless, it has several limitations. One of the main disadvantages is the excessive reliance on CT, resulting in the need to transport patients between the radiology department and operating room. It is also accompanied by substantial radiation exposure, and the need for multiple repeated CT scans or attempts to detect the locator when placing the hookwire, especially on occasions with inexperienced operators.
To simplify the localization of small pulmonary nodules and reduce the amount of radiation exposure, a novel AR navigation-guided pulmonary nodule localization was recently established by our team to guide percutaneous nodule localization. Use of AR navigation-guided technology aids surgery by providing additional visualization projected onto the patient’s body. Combined with advanced computer algorithms, such as deep learning or artificial intelligence and depth estimation, AR navigation-guided technology ensures the precision and safety of this application and accelerates the learning curve for difficult new procedures (19). Our AR navigation-guided localization procedure simply requires patients to undergo a standard CT scan with attached skin markers before surgery. A single thoracic surgeon performs the transthoracic marker implantation procedure while the patient receives general anesthesia in a standard operating room, after which resection of the pulmonary nodule is performed. Localization of pulmonary nodules is greatly simplified by AR navigation, with a mean registration and marker implantation time of 9.4 min, which is substantially shorter than the mean time required for intraoperative CT-guided procedures (a mean time of 16.8 min) (19).
When using our novel AR navigation-guided pulmonary nodule localization technique, intraoperative frozen section pathology revealed that all nodules were resected with an oncologically safe margin. Localization was successful in seven patients, with a median distance from the implanted marker to the planned target of 5.8 mm. We acknowledge that three cases were unsuccessful because of software defect (case No. 4, No. 6) and operative (case No. 10) problems. The causes of these failed cases were subsequently corrected. No perioperative complication nor excessive removal of lung tissue occurred, supporting the safety, feasibility, and utility of our technique for localizing subpleural small lung nodules.
Compared with traditional preoperative CT-guided hookwire localization, this novel AR navigation-guided method can be performed in a standard operating room after general anesthesia, therefore, reduces the interval between localization procedure and surgery, and eliminates the discomfort and potential risk of loss or displacement of the localizer brought about during transportation of patient to and from radiology department and the operating room. When facing complications including pneumothorax and hemorrhage, surgery could also be carried out immediately to minimize the impact of complications. In recent years, other localization methods have also been reported, for example IOCT-guided localization, intraoperative dye injection or bronchoscopic localization. Compared with these methods, which need specific equipment (near-infrared fluorescence camera, electromagnetic navigation bronchoscopy, etc.), the AR navigation procedures can be adapted with commercial head-mounted AR devices, which are more readily available. This technology may eventually reduce costs because of the need for fewer medical professionals and less specialized equipment (20,21). Also, localization of pulmonary nodules is greatly simplified with AR navigation-guided method, with a lot shorter time of localization.
This study and the technique have some limitations. For example, the small number of participants and the lack of comparative group do not allow us to make definitive statements about the efficacy and safety of the technique. And most of the cases enrolled underwent segmentectomy. The precision and safety should be further evaluated in future studies with large sample size and different surgical type. A limitation of the technique is that the patient position must be identical during the CT procedure and the operation. In this study, we used the supine position, which limited the lesion locations suitable for our localization procedure. Methods to match the patient’s respiration level during the CT scanning and intraoperative AR navigation procedure must also be developed. Furthermore, like translational CT-guided hookwire localization, some complications, for example fatal air embolism, still may occur during further application of the AR-guided localization even though the incidence of air embolism should be very low (22,23). Nevertheless, this study provides highly encouraging results regarding the feasibility and potential benefits of AR for localizing small lung nodules.
Conclusions
In conclusion, according to this prospective exploratory study, the augmented reality navigation-guided pulmonary nodule localization could be a safe and feasible technique for localizing subpleural small lung nodules.
Acknowledgments
The authors thank Fei Sun and Jiatao Ma from Jedicare Medical (Shanghai) Co., Ltd., who designed the LungBrella markers and the associated software. We also thank all the patients and their families.
Funding: This work was supported by the grant from
Footnote
Reporting Checklist: The authors have completed the TREND reporting checklist. Available at https://tlcr.amegroups.com/article/view/10.21037/tlcr-23-201/rc
Data Sharing Statement: Available at https://tlcr.amegroups.com/article/view/10.21037/tlcr-23-201/dss
Peer Review File: Available at https://tlcr.amegroups.com/article/view/10.21037/tlcr-23-201/prf
Conflicts of Interest: All authors have completed the ICMJE uniform disclosure form (available at https://tlcr.amegroups.com/article/view/10.21037/tlcr-23-201/coif). JL declared collaboration with Jedicare Medical Co., Ltd. The other authors have no conflicts of interest to declare.
Ethical Statement: The authors are accountable for all aspects of the work in ensuring that questions related to the accuracy or integrity of any part of the work are appropriately investigated and resolved. The study was conducted in accordance with the Declaration of Helsinki (as revised in 2013). The study was approved by the Ethics Committee of Ruijin Hospital, Shanghai Jiao Tong University School of Medicine (No. 2019-206) and informed consent was obtained from all individual participants.
Open Access Statement: This is an Open Access article distributed in accordance with the Creative Commons Attribution-NonCommercial-NoDerivs 4.0 International License (CC BY-NC-ND 4.0), which permits the non-commercial replication and distribution of the article with the strict proviso that no changes or edits are made and the original work is properly cited (including links to both the formal publication through the relevant DOI and the license). See: https://creativecommons.org/licenses/by-nc-nd/4.0/.
References
- de Koning HJ, van der Aalst CM, de Jong PA, et al. Reduced Lung-Cancer Mortality with Volume CT Screening in a Randomized Trial. N Engl J Med 2020;382:503-13. [Crossref] [PubMed]
- National Lung Screening Trial Research Team. Reduced lung-cancer mortality with low-dose computed tomographic screening. N Engl J Med 2011;365:395-409. [Crossref] [PubMed]
- Gould MK, Tang T, Liu IL, et al. Recent Trends in the Identification of Incidental Pulmonary Nodules. Am J Respir Crit Care Med 2015;192:1208-14. [Crossref] [PubMed]
- Li N, Tan F, Chen W, et al. One-off low-dose CT for lung cancer screening in China: a multicentre, population-based, prospective cohort study. Lancet Respir Med 2022;10:378-91. [Crossref] [PubMed]
- Tamura M, Oda M, Fujimori H, et al. New indication for preoperative marking of small peripheral pulmonary nodules in thoracoscopic surgery. Interact Cardiovasc Thorac Surg 2010;11:590-3. [Crossref] [PubMed]
- Wang L, Sun D, Gao M, et al. Computed tomography-guided localization of pulmonary nodules prior to thoracoscopic surgery. Thorac Cancer 2023;14:119-26. [Crossref] [PubMed]
- Narayanam S, Gerstle T, Amaral J, et al. Lung tattooing combined with immediate video-assisted thoracoscopic resection (IVATR) as a single procedure in a hybrid room: our institutional experience in a pediatric population. Pediatr Radiol 2013;43:1144-51. [Crossref] [PubMed]
- Chen PH, Hsu HH, Yang SM, et al. Preoperative Dye Localization for Thoracoscopic Lung Surgery: Hybrid Versus Computed Tomography Room. Ann Thorac Surg 2018;106:1661-7. [Crossref] [PubMed]
- Grogan EL, Jones DR, Kozower BD, et al. Identification of small lung nodules: technique of radiotracer-guided thoracoscopic biopsy. Ann Thorac Surg 2008;85:S772-7. [Crossref] [PubMed]
- Li C, Zheng Y, Yuan Y, et al. Augmented reality navigation-guided pulmonary nodule localization in a canine model. Transl Lung Cancer Res 2021;10:4152-60. [Crossref] [PubMed]
- MacMahon H, Naidich DP, Goo JM, et al. Guidelines for Management of Incidental Pulmonary Nodules Detected on CT Images: From the Fleischner Society 2017. Radiology 2017;284:228-43. [Crossref] [PubMed]
- Bertolaccini L, Prisciandaro E, Bardoni C, et al. Minimally Invasive Anatomical Segmentectomy versus Lobectomy in Stage IA Non-Small Cell Lung Cancer: A Systematic Review and Meta-Analysis. Cancers (Basel) 2022;14:6157. [Crossref] [PubMed]
- Ettinger DS, Wood DE, Aisner DL, et al. NCCN Guidelines® Insights: Non-Small Cell Lung Cancer, Version 2.2023. J Natl Compr Canc Netw 2023;21:340-50. [Crossref] [PubMed]
- Suzuki K, Nagai K, Yoshida J, et al. Video-assisted thoracoscopic surgery for small indeterminate pulmonary nodules: indications for preoperative marking. Chest 1999;115:563-8. [Crossref] [PubMed]
- Lin MW, Chen JS. Image-guided techniques for localizing pulmonary nodules in thoracoscopic surgery. J Thorac Dis 2016;8:S749-55. [Crossref] [PubMed]
- Tseng YH, Lee YF, Hsieh MS, et al. Preoperative computed tomography-guided dye injection to localize multiple lung nodules for video-assisted thoracoscopic surgery. J Thorac Dis 2016;8:S666-71. [Crossref] [PubMed]
- Lin MW, Tseng YH, Lee YF, et al. Computed tomography-guided patent blue vital dye localization of pulmonary nodules in uniportal thoracoscopy. J Thorac Cardiovasc Surg 2016;152:535-544.e2. [Crossref] [PubMed]
- Tang L, Zhang Y, Wang Y. Intraoperative identification of pulmonary nodules during minimally invasive thoracic surgery: a narrative review. Quant Imaging Med Surg 2022;12:5271-87. [Crossref] [PubMed]
- Chao YK, Wen CT, Fang HY, et al. A single-center experience of 100 image-guided video-assisted thoracoscopic surgery procedures. J Thorac Dis 2018;10:S1624-30. [Crossref] [PubMed]
- Jiang T, Yu D, Wang Y, et al. HoloLens-Based Vascular Localization System: Precision Evaluation Study With a Three-Dimensional Printed Model. J Med Internet Res 2020;22:e16852. [Crossref] [PubMed]
- Lee D, Yu HW, Kim S, et al. Vision-based tracking system for augmented reality to localize recurrent laryngeal nerve during robotic thyroid surgery. Sci Rep 2020;10:8437. [Crossref] [PubMed]
- Ichinose J, Kohno T, Fujimori S, et al. Efficacy and complications of computed tomography-guided hook wire localization. Ann Thorac Surg 2013;96:1203-8. [Crossref] [PubMed]
- Yi JH, Choi PJ, Bang JH, et al. Systemic air embolism after computed tomography-guided hook wire localization: two case reports and literature review. J Thorac Dis 2018;10:E59-64. [Crossref] [PubMed]