Thyroid transcription factor-1 (TTF-1) expression and the efficacy of combination therapy with immune checkpoint inhibitors and cytotoxic chemotherapy in non-squamous non-small cell lung cancer
Highlight box
Key findings
• Thyroid transcription factor-1 (TTF-1)-negative non-squamous non-small cell lung cancer (NS-NSCLC) patients treated with immune checkpoint inhibitor (ICI) plus chemotherapy tended to have better PFS and similar overall survival (OS) compared with TTF-1-positive patients. In particular, atezolizumab plus bevacizumab plus carboplatin plus paclitaxel (ABCP) showed long-term efficacy compared with other regimens.
What is known and what is new?
• TTF-1-negative NS-NSCLC is associated with poor efficacy of ICI monotherapy and pemetrexed plus platinum cytotoxic chemotherapy.
• TTF-1-negative NS-NSCLC patients treated with ICI plus chemotherapy tended to have better PFS and similar OS compared with TTF-1-positive patients.
What is the implication, and what should change now?
• ICI monotherapy was generally not efficacious for patients with TTF-1-negative NS-NSCLC, thus clinicians should consider ICI plus chemotherapy. ABCP would be an optimal regimen for TTF-1-negative NS-NSCLC.
Introduction
Immune checkpoint inhibitor (ICI) monotherapy or a combination of cytotoxic chemotherapy and ICI is the standard therapy for advanced non-squamous non-small cell lung cancer (NS-NSCLC) (1). Expression of programmed death ligand-1 (PD-L1) in tumor cells is the most widely used therapeutic indicator for ICIs, although the association between the efficacy of ICIs and PD-L1 expression has remained controversial (2). Furthermore, long-term survival is not achieved in all patients with high PD-L1 expression. Therefore, there is a need for biomarkers beyond PD-L1 that can be implemented in clinical practice to determine the optimal ICI and chemotherapy regimen.
Thyroid transcription factor-1 (TTF-1) is mainly expressed in type 2 alveolar epithelial cells; it has essential roles in morphogenesis and differentiation of the lung, thyroid, and forebrain (3,4). TTF-1 is expressed in approximately 70% of lung adenocarcinomas (5). Due to its restricted tissue expression, TTF-1 is one of the most reliable markers for distinguishing primary lung adenocarcinoma from metastatic lung tumors in clinical practice (6). The role of TTF-1 in lung cancer is poorly understood and, in some cases paradoxical. While it reportedly promotes carcinogenesis in lung cancer, some studies indicate it is a tumor suppressor in other tumor types (7-9). Before ICIs were widely used, TTF-1-negative status was reported as a poor prognosis factor in both the early and advanced stages of NS-NSCLC (10). TTF-1-negative status in lung adenocarcinoma is associated with poor efficacy of cytotoxic chemotherapies such as docetaxel and pemetrexed plus platinum (11,12). Even treatment with ICI monotherapy, one of the standard-of-care treatments in NSCLC, is poorly efficacious in TTF-1-negative cases (13,14). Due to these reports, TTF-1-negative status is considered a predictive factor of low therapeutic efficacy. However, the relationship between TTF-1 expression and the efficacy of ICI plus chemotherapy is still unclear. We conducted a retrospective study to elucidate the relationship between TTF-1 expression status and the efficacy of ICI-containing chemotherapy. We present this article in accordance with the STROBE reporting checklist (available at https://tlcr.amegroups.com/article/view/10.21037/tlcr-23-331/rc).
Methods
Patients and methods
We included patients who were administered ICI monotherapy or a combination of cytotoxic chemotherapy and ICI for advanced or recurrent NS-NSCLC at Nippon Medical School Tamanagayama Hospital between January 2016 and December 2021. We grouped patients into two treatment groups: ICI monotherapy or a combination of cytotoxic chemotherapy and ICI, and examined the association between the efficacy of each treatment and tumor TTF-1 expression. We retrieved clinical data including pathological diagnosis, TTF-1 expression, and tumor proportion score (TPS) of PD-L1 as evaluated w the 22C3 assay. In cases with where TTF-1 expression or PD-L1 TPS was unknown, we re-examined the archived pathological specimens by performing immunohistochemistry (IHC) staining for TTF-1 and PD-L1. The PD-L1 TPS was evaluated by IHC using a Dako PD-L1 22C3 pharmDx kit (Dako, Carpinteria, CA, USA). TTF-1 expression was evaluated using TTF-1 monoclonal antibody SPT24 (Nichirei, Tokyo, Japan). Oncogenic drivers including epidermal growth factor receptor (EGFR) and anaplastic lymphoma kinase (ALK) were examined using the Cobas®EGFR mutation test v2 (Roche, Basel, Switzerland) and FISH (Abbott Molecular, Des Plaines, IL, USA) or IHC (Roche, Basel, Switzerland) for ALK, and/or next-generation sequencing via the OncomineTM DX target test (Thermo Fisher, Waltham, MA, USA). The decision of which test to use was made at the physician’s discretion. Response and progression of the tumors were evaluated based on the Response Evaluation Criteria in Solid Tumors (RECIST) version 1.1 (15). This study was approved by the Ethics Committee of Nippon Medical School Tamanagayama Hospital (No. F-2022-010), and conducted according to the Declaration of Helsinki (as revised in 2013). Individual consent for this retrospective analysis was waived.
Statistical analysis
The categorical variables of each treatment were analyzed with Fisher’s exact test and Student’s t-test. We analyzed objective response rate (ORR), disease control rate (DCR), progression-free survival (PFS), and overall survival (OS) in each treatment group. PFS was defined as the period from the date of treatment initiation to the date of disease progression or last follow-up. OS was defined as the period from the date of treatment initiation to the date of death from any cause or last follow-up. Both OS and PFS were estimated with the Kaplan-Meier method and patient group differences were compared with the log-rank test. Hazard ratio (HR) and corresponding 95% confidence intervals (CIs) were calculated from a Cox proportional hazard model. We analyzed data with IBM SPSS Statistics program (version 28.0.1.0, IBM, Armonk, NY, USA).
Results
Patient characteristics
A total of 130 patients had been treated with regimens that included ICI. One patient was excluded because they suffered from a double cancer (lung adenocarcinoma and squamous cell carcinoma). We re-examined the pathological tissue samples from 17 of 129 patients to determine PD-L1 and TTF-1 expression status. The last follow-up date was March 31, 2023. The median follow-up time was 17.9 months (range, 0.73–67.2 months).
The characteristics of the entire patient population are shown in Table 1, and characteristics of each treatment group are shown in Tables 2,3. Thirty-three out of 129 patients (25.6%) were TTF-1-negative (Table 1). In the ICI plus chemotherapy group, the proportion of females was slightly higher in TTF-1-positive compared to TTF-1-negative cases (Table 3). The proportion of worse performance status (PS) (≥2) was higher in TTF-1-negative than in TTF-1-positive patients treated with ICI monotherapy (47.4% vs. 25.5%, P=0.08, Table 2). EGFR mutations tended to be more frequent in the TTF-1-positive group (Tables 2,3). The proportion of adenocarcinomas was higher in TTF-1-positive patients in both treatment groups (Tables 2,3). There was no significant correlation between PD-L1 and TTF-1 expression, but a higher proportion of the TTF-1-negative cases were PD-L1 negative when compared with TTF-1-positive cases (Tables 2,3). Other baseline characteristics did not statistically differ between the treatment groups (Tables 2,3).
Table 1
Characteristics | Subtypes | All patients (N=129) |
---|---|---|
Age, median [range], year | 72 [43–86] | |
Sex, n (%) | Male | 91 (70.5) |
Female | 38 (29.5) | |
Smoking, n (%) | Ever | 96 (74.4) |
Never | 33 (25.6) | |
PS, n (%) | 0–1 | 98 (76.0) |
≥2 | 31 (24.0) | |
Stage, n (%) | III | 3 (2.3) |
IV | 95 (73.6) | |
Recurrent | 31 (24.0) | |
Pathology, n (%) | Adenocarcinoma | 113 (87.6) |
Other | 16 (12.4) | |
TTF-1, n (%) | Positive | 96 (74.4) |
Negative | 33 (25.6) | |
PD-L1, n (%) | <1% | 46 (35.7) |
1–49% | 39 (30.2) | |
≥50% | 44 (34.1) | |
EGFR, n (%) | Positive | 20 (15.5) |
Negative | 109 (84.5) | |
ALK, n (%) | Positive | 3 (2.3) |
Negative | 126 (97.7) | |
Regimen, n (%) | ICI | 70 (54.3) |
ICI + chemo | 59 (45.7) |
PS, performance status; TTF-1, thyroid transcription factor-1; PD-L1, programmed death ligand-1; EGFR, epidermal growth factor receptor; ALK, anaplastic lymphoma kinase; chemo, chemotherapy; ICI, immune checkpoint inhibitor.
Table 2
Characteristics | Subtypes | TTF-1-negative (N=19) | TTF-1-positive (N=51) | P |
---|---|---|---|---|
Age, median [range], year | 76 [50–86] | 73 [43–85] | 0.61 | |
Sex, n (%) | Male | 12 (63.2) | 35 (68.6) | 0.78 |
Female | 7 (36.8) | 16 (31.4) | ||
Smoking, n (%) | Ever | 14 (73.7) | 35 (68.6) | 0.78 |
Never | 5 (26.3) | 16 (31.4) | ||
PS, n (%) | 0–1 | 10 (52.6) | 38 (74.5) | 0.08 |
≥2 | 9 (47.4) | 13 (25.5) | ||
Stage, n (%) | III | 1 (5.3) | 0 | 0.13 |
IV | 14 (73.7) | 32 (62.7) | ||
Recurrent | 4 (21.0) | 19 (37.3) | ||
EGFR, n (%) | Positive | 0 | 9 (17.6) | 0.10 |
Negative | 19 (100.0) | 42 (82.4) | ||
ALK, n (%) | Positive | 1 (5.3) | 2 (3.9) | 1.00 |
Negative | 18 (94.7) | 49 (96.1) | ||
Pathology, n (%) | Adenocarcinoma | 14 (73.7) | 47 (92.2) | 0.05 |
Other | 5 (26.3) | 4 (7.8) | ||
PD-L1, n (%) | <1% | 6 (31.6) | 12 (23.5) | 0.17 |
1–49% | 8 (42.1) | 13 (25.5) | ||
≥50% | 5 (26.3) | 26 (51.0) | ||
Regimen, n (%) | Pembrolizumab | 10 (52.6) | 32 (62.7) | 0.58 |
Atezolizumab | 5 (26.3) | 8 (15.7) | ||
Nivolumab | 4 (21.1) | 11 (21.6) |
TTF-1, thyroid transcription factor-1; PS, performance status; EGFR, epidermal growth factor receptor; ALK, anaplastic lymphoma kinase; PD-L1, programmed death ligand-1.
Table 3
Characteristics | Subtypes | TTF-1-negative (N=14) | TTF-1-positive (N=45) | P |
---|---|---|---|---|
Age, median [range], year | 68.5 [55–76] | 70.0 [45–82] | 0.74 | |
Sex, n (%) | Male | 13 (92.9) | 31 (68.9) | 0.09 |
Female | 1 (7.1) | 14 (31.1) | ||
Smoking, n (%) | Ever | 12 (85.7) | 35 (77.8) | 0.71 |
Never | 2 (14.3) | 10 (22.2) | ||
PS, n (%) | 0–1 | 11 (78.6) | 39 (86.7) | 0.43 |
≥2 | 3 (21.4) | 6 (13.3) | ||
Stage, n (%) | III | 1 (7.1) | 1 (2.2) | 0.51 |
IV | 12 (85.8) | 37 (82.2) | ||
Recurrent | 1 (7.1) | 7 (15.6) | ||
EGFR, n (%) | Positive | 0 | 11 (24.4) | 0.051 |
Negative | 14 (100.0) | 34 (75.6) | ||
ALK, n (%) | Positive | 0 | 0 | 1.00 |
Negative | 14 (100.0) | 45 (100.0) | ||
Pathology, n (%) | Adenocarcinoma | 9 (64.3) | 43 (95.6) | 0.006 |
Other | 5 (35.7) | 2 (4.4) | ||
PD-L1, n (%) | <1% | 9 (64.3) | 19 (42.2) | 0.26 |
1–49% | 2 (14.3) | 16 (35.6) | ||
≥50% | 3 (21.4) | 10 (22.2) | ||
Regimen, n (%) | CBDCA + PTX + BEV + ATZ | 6 (42.9) | 18 (40.0) | 0.22 |
CBDCA + nab-PTX + ATZ/Pembro | 4 (28.6) | 4 (8.9) | ||
Platinum + PEM + Pembro | 4 (28.6) | 22 (48.9) | ||
CBDCA + PEM + Nivo+Ipi | 0 | 1 (2.2) |
TTF-1, thyroid transcription factor-1; PS, performance status; EGFR, epidermal growth factor receptor; ALK, anaplastic lymphoma kinase; PD-L1, programmed death ligand-1; CBDCA, carboplatin; PTX, paclitaxel; BEV, bevacizumab; ATZ, atezolizumab; nab-PTX, nanoparticle albumin-bound paclitaxel; Pembro, pembrolizumab; PEM, pemetrexed; Nivo, nivolumab; Ipi, ipilimumab.
Treatment regimen
Of the 129 patients, 70 were treated with ICI monotherapy and 59 were treated with ICI plus chemotherapy. We separated the ICI monotherapy group across three main regimens: pembrolizumab (200 mg/body every 3 weeks), nivolumab (240 mg/body every 2 weeks or 480 mg/body every 4 weeks), and atezolizumab (1,200 mg/body every 3 weeks); the frequency at which these regimens were used was similar between the TTF-1-negative and -positive groups (Table 2). The ICI plus chemotherapy group was also separated according to three main regimens: atezolizumab (1,200 mg/body) plus bevacizumab (15 mg/kg) plus carboplatin (CBDCA) [area under the curve (AUC) 5] plus paclitaxel (175 mg/m2) (ABCP), atezolizumab (1,200 mg/body) or pembrolizumab (200 mg/body) plus CBDCA (AUC 6) plus nab-paclitaxel (100 mg/m2), and pembrolizumab (200 mg/body) plus platinum [cis-diamminedichloroplatinum (CDDP) (75 mg/m2) or CBDCA (AUC5)] plus pemetrexed (500 mg/m2) every 3–4 weeks up to 4 cycles. After four cycles, the following maintenance therapies were administered. The attending physician made appropriate decisions regarding the dose reduction of chemotherapy according to the patient’s condition. The frequency with which these regimens was chosen did not differ between groups (Table 3).
Efficacy of ICI monotherapy and the relationship with TTF-1 expression
In Table S1, ORR and DCR were not statistically different between the TTF-1-positive and -negative groups (23.5% vs. 15.8%, P=0.74; 52.9% vs. 42.1%, P=0.59). In the ICI monotherapy group (N=70), PFS was not significantly different between TTF-1-negative and -positive patients (median 3.8 vs. 3.6 months, P=0.27; Figure 1A,1B). However, the long-term efficacy of ICI monotherapy (>2 years) was not observed in TTF-1-negative patients. TTF-1-negative patients tended to have worse OS than TTF-1-positive patients (median 15.6 vs. 19.5 months, P=0.13). EGFR mutations and ALK translocations are poor predictive factors for ICI monotherapy (16). Since such patients were included in our initial analysis and could confound our findings, we decided to perform a sub-analysis where we examined the impact of TTF-1 status in patients who had only wild-type EGFR and ALK. These patients had poorer PFS and OS when they had TTF-1-negative tumors (Figure 1C,1D). A trend for worse PFS was observed in TTF-1-negative cases compared with those that were TTF-1-positive (median PFS: 3.8 vs. 4.5 months, P=0.088; HR: 1.75, 95% CI: 0.91–3.35); this trend was therefore more obvious than the differences we observed in our initial analysis of all patients, including those with EGFR mutations and ALK translocations (Figure 1A,1B). Indeed, the shape of the Kaplan-Meier curve for OS was not significantly different between wild-type EGFR and ALK cases, and the corresponding curve based on all cases.
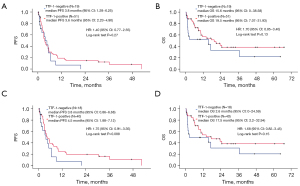
Efficacy of ICI and chemotherapy, and the relationship with TTF-1 expression
Table S2 shows that ORR was higher in the TTF-1-negative group compared with TTF-1-positive patients (78.6% vs. 46.7%, P=0.063), whereas DCR was similar in the two groups (92.9% vs. 82.2%, P=0.67). As shown in Figure 2A,2B, there were no significant differences in PFS and OS; however, TTF-1 negative patients tended to have better PFS and a similar OS when compared with TTF-1-positive patients (median PFS: 9.9 vs. 9.6 months, P=0.14; median OS: 32.3 vs. 18.9 months, P=0.78). To elucidate which regimens contributed to prolonged PFS and OS in TTF-1-negative patients, we plotted Kaplan-Meier curves by each regimen (Figure 3A,3B). ABCP was the only regimen to elicit a long-term response. The efficacies of each regimen in TTF-1-positive patients are shown in Figure S1A,S1B. In the TTF-1 positive population, OS and PFS were similar for all regimens. The efficacy of ABCP and the relationship with TTF-1 expression is shown in Figure 4A,4B. The TTF-1-negative group tended to have longer PFS and OS compared with the TTF-1-positive group (median PFS: 22.5 vs. 9.7 months, P=0.078; median OS: not reached vs. 10.9 months, P=0.11). For all the other treatment regimens, PFS and OS in the TTF-1-negative group were not superior to those of TTF-1-positive patients (Figure S2A,S2B). The relationships between TTF-1 expression and the efficacy of all regimens except for ABCP are shown in Figures S3,S4.
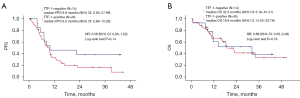
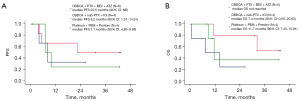
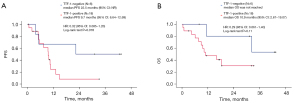
Treatment efficacy by TTF-1 expression according to PD-L1 expression status
The relationship between TTF-1 expression status and treatment efficacy according to PD-L1 expression status is shown in Figures 5,6. In the ICI monotherapy group excluding EGFR and ALK mutations/translocations with PD-L1 ≥50% expression, TTF-1-negative patients had statistically worse PFS compared with TTF-1-positive patients (median PFS 2.1 vs. 9.4 months, P=0.019). There were no significant differences in OS (median OS 2.6 vs. 24.7 months, P=0.27), but mortality in the early stage of initiating treatment tended to be higher in the TTF-1-negative group. In the ICI + chemotherapy group, TTF-1-negative patients tended to have better PFS and OS than TTF-1-positive patients in the PD-L1 <1% expression group (median PFS 22.5 vs. 8.0 months, P=0.056, median OS 32.3 vs. 16.1 months, P=0.15).

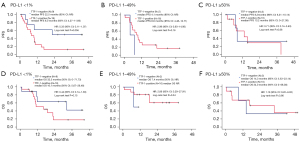
Discussion
In our study, TTF-1-negative patients treated with ICI monotherapy tended to have worse PFS and OS than TTF-1-positive patients. Additionally, long-term efficacy (>2 years) and therapeutic efficacy for high PD-L1 expression of ICI monotherapy were not observed in TTF-1-negative patients. On the other hand, TTF-1-negative patients treated with ICI plus chemotherapy tended to have better PFS and a similar OS when compared with TTF-1-positive patients. As a side note that ICI plus chemotherapy showed better PFS and OS compared with ICI monotherapy in TTF-1-negative patients (Figure S5). Thus, clinicians should consider ICI plus chemotherapy in TTF-1-negative patients. To our knowledge, this is the first report of a favorable therapeutic efficacy in TTF-1-negative NS-NSCLC. TTF-1-negative status has been recognized as a poor predictive factor in various chemotherapy regimens, including platinum plus pemetrexed, docetaxel and EGFR-tyrosine kinase inhibitors (TKIs) (11,12,17). Our study showed that ICI/chemotherapy regimens (and especially ABCP) can have long-term efficacy in TTF-1-negative lung cancer patients.
In the ICI monotherapy group TTF-1-negative status tended to be associated with worse PFS and OS compared with TTF-1-positive status, and that PFS tended to be worse in the TTF-1-negative group once EGFR and ALK mutations/translocations were removed from the analysis. This finding is consistent with those of previous studies (13,14). Neither EGFR nor ALK status had a significant impact on OS. We suggest this is because our study included patients who had had a long-term response to EGFR-TKIs after progression on ICI monotherapy. Especially in the PD-L1 ≥50% group, TTF-1 negative patients had significantly worse PFS than positive patients. PD-L1 high expression state is generally considered to have a favorable therapeutic efficacy of ICI. Even with high PD-L1 expression, TTF-1-negative patients may not respond to ICI monotherapy as well as TTF-1-positive patients. We also note the fraction of patients with poor PS was higher in TTF-1-negative compared to TTF-1-positive individuals. TTF-1-negative status is related to a higher frequency of serine-threonine kinase 11 (STK11) and Kelch-like epichlorohydrin-associated protein 1 (KEAP1) mutations (18,19). These mutations are associated with a tumor microenvironment that has a lower density of tumor-infiltrating leukocytes and cytotoxic CD8+ T lymphocytes, and lower PD-L1 expression (20-24). In our study, the TTF-1-negative group also had a higher proportion of PD-L1 negative tumors. TTF-1-negative status is therefore a poor predictive factor for ICI monotherapy, as it is associated with an ‘immune cold’ phenotype.
In the ICI plus chemotherapy group, there was no significant difference in PFS and OS between TTF-1-negative and -positive patients, although the Kaplan-Meier curves in both cases for TTF-1-negative patients were slightly above those of TTF-1-positive patients (Figure 2). To elucidate which regimens contributed to prolonged PFS and OS in TTF-1-negative cases, we analyzed Kaplan-Meier according to the individual treatment regimens. There was a notably improved trend with the ABCP regimen (Figure 3A,3B). Median PFS with ABCP was longer in the TTF-1-negative group than in the TTF-1-positive group (22.5 vs. 9.7 months, respectively, Figure 4A). In the IMpower 150 trial, median PFS in the ABCP group was 8.3 months, suggesting that this treatment is by no means less effective in TTF-1-positive patients (25). We consider these differences in therapeutic efficacy are related to the specificity of the TTF-1-negative tumor microenvironment. TTF-1-negative status is associated with serglycin (SRGN) overexpression, and leads to secretion of chemokines and cytokines including interleukin (IL)-6, IL-8, C-X-C motif chemokine 1. These agents reprogram the tumor microenvironment to a more state that is more conducive to malignancy; the tumor cells induce angiogenesis and suppress immune recognition by interacting with fibroblasts and endothelial cells (26). In addition, chronic inflammation in the tumor microenvironment stimulates nuclear factor kappa B (NF-κB) activity. High NF-κB activity induces angiogenesis, suppresses the immune response, and stimulates cancer stem cell formation; together these processes engender a microenvironment that facilitates tumor cell survival and proliferation (27). ABCP is efficacious in NS-NSCLC with STK-11 or KEAP1 mutations which are associated with TTF-1-negative status and have a poor prognosis (28). Inhibiting the VEGF pathway improves vascular permeability in tumor tissue, suppresses regulatory T cells, decreases the recruitment of myeloid derived suppressor cells, and reduces the expression of programmed cell death protein 1 (PD-1) on CD8-positive cytotoxic T lymphocytes and regulatory T cells (29-32). We suggest that bevacizumab converts the immunosuppressive tumor microenvironment in TTF-1-negative patients to an immune stimulatory environment via inhibition of VEGF, thereby enhancing the efficacy of atezolizumab. This hypothesis is supported by our observation that atezolizumab plus bevacizumab exhibited long-term efficacy in our study, whereas bevacizumab plus chemotherapy is not efficacious compared with chemotherapy (33). On the other hand, the regimens of ICI plus CBDCA plus nab-paclitaxel and pembrolizumab plus platinum plus pemetrexed were not more effective in TTF-1-negative compared to TTF-1-positive patients (Figures S3,S4). A recent study demonstrated that PFS was significantly longer in TTF-1-positive than TTF-1-negative patients treated with ICI plus platinum plus pemetrexed (34). Our institution used ABCP after EGFR or ALK-TKI failure, in patients with massive pleural effusions, multiple liver metastases, and/or rapidly progressing tumors. ABCP therapy may also be promising for TTF-1-negative NS-NSCLC patients.
Our retrospective study contained several major limitations. First, it was a single center study and the sample size was relatively small, especially once we had subdivided the patients treated with ICI plus chemotherapy into each regimen. This limitation weakened our power to detect meaningful differences in efficacy between regimens. However, we did not exclude patients due to uncertain PD-L1 or TTF-1 status, as we re-examined such cases to confirm the relative status. To the best of our knowledge, previous studies evaluating therapeutic efficacy and TTF-1 expression in NSCLC only included cases where TTF-1 expression status was known, thus leading to selection bias. In contrast, we suggest that our study provides less biased and more clinically practical data. A second limitation of our study was our inability to determine SRGN expression or the mutational status of KEAP1/STK11 and other druggable mutations (with the exception of EGFR and ALK). However, neither SRGN expression nor KEAP1/STK11 mutation routinely screened for in clinical practice, and the status of these mutations is difficult to obtain in all cases. In addition, the status of druggable mutations is not available in all cases, as it is highly dependent on the volume of tumor specimens and the clinical course. The third limitation relates to the examination for TTF-1. Our institution has been using SPT24 antibody for examining tumor TTF-1 status. However, false positive signals with the SPT24 antibody have been reported in squamous cell carcinoma, and the 8G7G3 antibody has better specificity (35). Although we did not diagnose squamous cell carcinoma solely based on TTF-1 expression status, the use of different TTF-1 antibodies could impact the interpretation of our results.
Our study supports previous findings that ICI monotherapy for TTF-1-negative NS-NSCLC has poor efficacy compared with the same treatment in TTF-1-positive cases, and suggests the combination of ICI plus chemotherapy can be efficacious regardless of TTF-1 status. This appears to be the case especially for the ABCP regimen. For NS-NSCLC with poor prognostic factors including TTF-1-negative status, addition of a VEGF inhibitor enhances the efficacy of ICI, and this combination might be an optimal treatment option. Further prospective research with larger cohorts will be required to address this clinical hypothesis.
Conclusions
ICI monotherapy tended to have poor efficacy for TTF-1-negative NS-NSCLC; thus, clinicians should consider ICI plus chemotherapy. Based on our study, we suggest ABCP is an optimal regimen for TTF-1-negative NS-NSCLC.
Acknowledgments
Funding: None.
Footnote
Reporting Checklist: The authors have completed the STROBE reporting checklist. Available at https://tlcr.amegroups.com/article/view/10.21037/tlcr-23-331/rc
Data Sharing Statement: Available at https://tlcr.amegroups.com/article/view/10.21037/tlcr-23-331/dss
Peer Review File: Available at https://tlcr.amegroups.com/article/view/10.21037/tlcr-23-331/prf
Conflicts of Interest: All authors have completed the ICMJE uniform disclosure form (available at https://tlcr.amegroups.com/article/view/10.21037/tlcr-23-331/coif). MS has received funding from Chugai Pharmaceutical, MSD, and Taiho Pharmaceutical; and honoraria for lectures from Bristol-Meyers Squibb, Chugai Pharmaceutical, MSD, Ono Pharmaceutical, and Taiho Pharmaceutical. TH has received funding from Chugai Pharmaceutical, and Taiho Pharmaceutica; and honoraria for lectures from Bristol-Meyers Squibb, Chugai Pharmaceutical, MSD, and, Taiho Pharmaceutical. The other authors have no conflicts of interest to declare.
Ethical Statement: The authors are accountable for all aspects of the work in ensuring that questions related to the accuracy or integrity of any part of the work are appropriately investigated and resolved. The study was conducted in accordance with the Declaration of Helsinki (as revised in 2013). The study was approved by Nippon Medical School Tamanagayama Hospital, Tokyo, Japan ethics committee (No. F-2022-010) and individual consent for this retrospective analysis was waived.
Open Access Statement: This is an Open Access article distributed in accordance with the Creative Commons Attribution-NonCommercial-NoDerivs 4.0 International License (CC BY-NC-ND 4.0), which permits the non-commercial replication and distribution of the article with the strict proviso that no changes or edits are made and the original work is properly cited (including links to both the formal publication through the relevant DOI and the license). See: https://creativecommons.org/licenses/by-nc-nd/4.0/.
References
- Singh N, Temin S, Baker S Jr, et al. Therapy for Stage IV Non-Small-Cell Lung Cancer Without Driver Alterations: ASCO Living Guideline. J Clin Oncol 2022;40:3323-43. [Crossref] [PubMed]
- Doroshow DB, Bhalla S, Beasley MB, et al. PD-L1 as a biomarker of response to immune-checkpoint inhibitors. Nat Rev Clin Oncol 2021;18:345-62. [Crossref] [PubMed]
- Ikeda K, Clark JC, Shaw-White JR, et al. Gene structure and expression of human thyroid transcription factor-1 in respiratory epithelial cells. J Biol Chem 1995;270:8108-14. [Crossref] [PubMed]
- Kimura S, Hara Y, Pineau T, et al. The T/ebp null mouse: thyroid-specific enhancer-binding protein is essential for the organogenesis of the thyroid, lung, ventral forebrain, and pituitary. Genes Dev 1996;10:60-9. [Crossref] [PubMed]
- Yatabe Y, Mitsudomi T, Takahashi T. TTF-1 expression in pulmonary adenocarcinomas. Am J Surg Pathol 2002;26:767-73. [Crossref] [PubMed]
- Stenhouse G, Fyfe N, King G, et al. Thyroid transcription factor 1 in pulmonary adenocarcinoma. J Clin Pathol 2004;57:383-7. [Crossref] [PubMed]
- Kwei KA, Kim YH, Girard L, et al. Genomic profiling identifies TITF1 as a lineage-specific oncogene amplified in lung cancer. Oncogene 2008;27:3635-40. [Crossref] [PubMed]
- Tanaka H, Yanagisawa K, Shinjo K, et al. Lineage-specific dependency of lung adenocarcinomas on the lung development regulator TTF-1. Cancer Res 2007;67:6007-11. [Crossref] [PubMed]
- Winslow MM, Dayton TL, Verhaak RG, et al. Suppression of lung adenocarcinoma progression by Nkx2-1. Nature 2011;473:101-4. [Crossref] [PubMed]
- Kim JH, Kim HS, Kim BJ, et al. Prognostic Impact of TTF-1 Expression in Non-Squamous Non-Small-Cell Lung Cancer: A Meta-Analysis. J Cancer 2018;9:4279-86. [Crossref] [PubMed]
- Takeuchi A, Oguri T, Yamashita Y, et al. Value of TTF-1 expression in non-squamous non-small-cell lung cancer for assessing docetaxel monotherapy after chemotherapy failure. Mol Clin Oncol 2020;13:9. [Crossref] [PubMed]
- Frost N, Zhamurashvili T, von Laffert M, et al. Pemetrexed-Based Chemotherapy Is Inferior to Pemetrexed-Free Regimens in Thyroid Transcription Factor 1 (TTF-1)-Negative, EGFR/ALK-Negative Lung Adenocarcinoma: A Propensity Score Matched Pairs Analysis. Clin Lung Cancer 2020;21:e607-21. [Crossref] [PubMed]
- Galland L, Le Page AL, Lecuelle J, et al. Prognostic value of Thyroid Transcription Factor-1 expression in lung adenocarcinoma in patients treated with anti PD-1/PD-L1. Oncoimmunology 2021;10:1957603. [Crossref] [PubMed]
- Nakahama K, Kaneda H, Osawa M, et al. Association of thyroid transcription factor-1 with the efficacy of immune-checkpoint inhibitors in patients with advanced lung adenocarcinoma. Thorac Cancer 2022;13:2309-17. [Crossref] [PubMed]
- Eisenhauer EA, Therasse P, Bogaerts J, et al. New response evaluation criteria in solid tumours: revised RECIST guideline (version 1.1). Eur J Cancer 2009;45:228-47. [Crossref] [PubMed]
- Oya Y, Kuroda H, Nakada T, et al. Efficacy of Immune Checkpoint Inhibitor Monotherapy for Advanced Non-Small-Cell Lung Cancer with ALK Rearrangement. Int J Mol Sci 2020;21:2623. [Crossref] [PubMed]
- Park JY, Jang SH, Kim HI, et al. Thyroid transcription factor-1 as a prognostic indicator for stage IV lung adenocarcinoma with and without EGFR-sensitizing mutations. BMC Cancer 2019;19:574. [Crossref] [PubMed]
- Karthikeyan SK, Gimbrone NT, Percy TR, et al. Loss of cellular identity in common pre-clinical models of serine threonine kinase 11 (Liver kinase B1) loss. Cancer Treat Res Commun 2021;26:100286. [Crossref] [PubMed]
- Cardnell RJ, Behrens C, Diao L, et al. An Integrated Molecular Analysis of Lung Adenocarcinomas Identifies Potential Therapeutic Targets among TTF1-Negative Tumors, Including DNA Repair Proteins and Nrf2. Clin Cancer Res 2015;21:3480-91. [Crossref] [PubMed]
- Cheng W, Xu B, Zhang H, et al. Lung adenocarcinoma patients with KEAP1 mutation harboring low immune cell infiltration and low activity of immune environment. Thorac Cancer 2021;12:2458-67. [Crossref] [PubMed]
- Koyama S, Akbay EA, Li YY, et al. STK11/LKB1 Deficiency Promotes Neutrophil Recruitment and Proinflammatory Cytokine Production to Suppress T-cell Activity in the Lung Tumor Microenvironment. Cancer Res 2016;76:999-1008. [Crossref] [PubMed]
- Kadara H, Choi M, Zhang J, et al. Whole-exome sequencing and immune profiling of early-stage lung adenocarcinoma with fully annotated clinical follow-up. Ann Oncol 2017;28:75-82. [Crossref] [PubMed]
- Biton J, Mansuet-Lupo A, Pécuchet N, et al. TP53, STK11, and EGFR Mutations Predict Tumor Immune Profile and the Response to Anti-PD-1 in Lung Adenocarcinoma. Clin Cancer Res 2018;24:5710-23. [Crossref] [PubMed]
- Papillon-Cavanagh S, Doshi P, Dobrin R, et al. STK11 and KEAP1 mutations as prognostic biomarkers in an observational real-world lung adenocarcinoma cohort. ESMO Open 2020;5:e000706. [Crossref] [PubMed]
- Socinski MA, Jotte RM, Cappuzzo F, et al. Atezolizumab for First-Line Treatment of Metastatic Nonsquamous NSCLC. N Engl J Med 2018;378:2288-301. [Crossref] [PubMed]
- Tanaka I, Dayde D, Tai MC, et al. SRGN-Triggered Aggressive and Immunosuppressive Phenotype in a Subset of TTF-1-Negative Lung Adenocarcinomas. J Natl Cancer Inst 2022;114:290-301. [Crossref] [PubMed]
- Taniguchi K, Karin M. NF-κB, inflammation, immunity and cancer: coming of age. Nat Rev Immunol 2018;18:309-24. [Crossref] [PubMed]
- West HJ, McCleland M, Cappuzzo F, et al. Clinical efficacy of atezolizumab plus bevacizumab and chemotherapy in KRAS-mutated non-small cell lung cancer with STK11, KEAP1, or TP53 comutations: subgroup results from the phase III IMpower150 trial. J Immunother Cancer 2022;10:e003027. [Crossref] [PubMed]
- Terme M, Pernot S, Marcheteau E, et al. VEGFA-VEGFR pathway blockade inhibits tumor-induced regulatory T-cell proliferation in colorectal cancer. Cancer Res 2013;73:539-49. [Crossref] [PubMed]
- Lindau D, Gielen P, Kroesen M, et al. The immunosuppressive tumour network: myeloid-derived suppressor cells, regulatory T cells and natural killer T cells. Immunology 2013;138:105-15. [Crossref] [PubMed]
- Voron T, Colussi O, Marcheteau E, et al. VEGF-A modulates expression of inhibitory checkpoints on CD8+ T cells in tumors. J Exp Med 2015;212:139-48. [Crossref] [PubMed]
- Shaked Y, Henke E, Roodhart JM, et al. Rapid chemotherapy-induced acute endothelial progenitor cell mobilization: implications for antiangiogenic drugs as chemosensitizing agents. Cancer Cell 2008;14:263-73. [Crossref] [PubMed]
- Takeuchi A, Oguri T, Yamashita Y, et al. TTF-1 Expression Predicts the Merit of Additional Antiangiogenic Treatment in Non-squamous Non-small Cell Lung Cancer. Anticancer Res 2018;38:5489-95. [Crossref] [PubMed]
- Ibusuki R, Yoneshima Y, Hashisako M, et al. Association of thyroid transcription factor-1 (TTF-1) expression with efficacy of PD-1/PD-L1 inhibitors plus pemetrexed and platinum chemotherapy in advanced non-squamous non-small cell lung cancer. Transl Lung Cancer Res 2022;11:2208-15. [Crossref] [PubMed]
- Vidarsdottir H, Tran L, Nodin B, et al. Comparison of Three Different TTF-1 Clones in Resected Primary Lung Cancer and Epithelial Pulmonary Metastases. Am J Clin Pathol 2018;150:533-44. [Crossref] [PubMed]