Characteristics and prognostic analysis of patients with detected KRAS mutations in resected lung adenocarcinomas by peptide nucleic acid-locked nucleic acid polymerase chain reaction (PNA-LNA PCR) clamp method
Highlight box
Key findings
• Kirsten rat sarcoma virus (KRAS) mutations were detectable with high sensitivity by the peptide nucleic acid-locked nucleic acid polymerase chain reaction (PNA-LNA PCR) clamp method.
What is known and what is new?
• KRAS mutations are frequently present in lung adenocarcinoma. The presence of KRAS mutations in adenocarcinoma is the most common driver mutation for Caucasians (about 20–30%), whereas the rate is about 10–15% in East Asians.
• The frequency of KRAS mutations and their subtypes in surgically resected tumors in Japan was determined.
• The prognosis of patients with surgically resected tumors with KRAS mutations was comparable to that of patients with epidermal growth factor receptor (EGFR) and without KRAS mutations.
• KRAS mutations were detectable with high sensitivity by the PNA-LNA PCR clamp method.
What is the implication, and what should change now?
• The PNA-LNA PCR clamp method was more sensitive and may apply to multiple situations in detecting KRAS mutations in the future.
Introduction
The discovery of driver genes and the development of molecular targeted drugs against them have gradually improved the course of poor prognosis lung cancer (1-7). Kirsten rat sarcoma virus (KRAS) mutations were first reported in 1985 as driver mutations on the human chromosome (8,9). Various drugs targeting KRAS mutations have been developed over the past few decades but have failed to achieve sufficient efficacy (10).
Sotorasib has recently been launched as a molecular targeted therapy for KRAS G12C mutations. This development of an effective molecular targeted therapy for KRAS mutations has enhanced the importance of the frequency, distribution characteristics and detection methods of KRAS mutations.
RAS is a guanosine triphosphate (GTP) binding protein including v-Ha-ras Harvey rat sarcoma viral oncogene homolog (HRAS), KRAS, and neuroblastoma RAS viral oncogene homolog (NRAS). In humans, KRAS mutations account for 85% of RAS mutations (11) and are most common in pancreatic cancer, colorectal cancer, and lung cancer, in that order (12). The frequency of KRAS subtypes varies by organ cancer, with G12D being more common in pancreatic cancer (13) and G12C being more common in lung cancer (14).
The mechanism of carcinogenesis by KRAS mutations is as follows. KRAS binding to guanosine diphosphate (GDP) results in an inactivated state, while binding to GTP results in an activated state. By upstream signals such epidermal growth factor receptor (EGFR), GDP is exchanged for GTP through guanine nucleotide exchange factors (GEF). Mutations in the KRAS gene cause constant activation by sustained GTP binding to mutant KRAS and lead to signal transduction to the intracellular phosphoinositide 3-kinases (PI3K), mitogen-activated protein kinase (MAPK), and Ral guanine nucleotide exchange factors (RAL-GEFs) pathways, leading to tumor formation. This sustainability of GTP binding to mutated KRAS varies among KRAS mutation subtypes (15,16).
KRAS mutations are frequently present in lung adenocarcinoma. The presence of KRAS mutations in adenocarcinoma is the most common driver mutation for Caucasians (about 20–30%) (17-19), whereas the rate is about 10–15% in East Asians (20-22). Recently, a Japanese report showed 14% of Japanese patients to have KRAS mutations. Analysis of subtypes of KRAS mutations in the Japanese data showed that G12C, G12D, G12V, and G12A were the most common, in that order. KRAS G12C mutations are found in 4.0% of all lung adenocarcinoma patients (23). There have been few recent publications on the frequency and distribution of KRAS, but its background factors are becoming more important with the launch of molecular targeted drugs for KRAS G12C.
In phase I trials of sotorasib in non-small cell lung cancer (NSCLC) cases and phase II trials in previously treated NSCLC patients, objective response rate (ORR) was 32.2% and 37.1%, respectively, disease control rate (DCR) was 88.1% and 80.6%, and median progression-free survival (PFS) was 6.3 and 6.8 months (24,25). With these results, sotorasib has been approved by the Food and Drug Administration (FDA) and the Japanese Pharmaceuticals and Medical Devices Agency (PMDA) for patients with KRAS G12C.
The QIAGEN therascreen KRAS RGQ PCR kit (for tissue) and Guardant360 CDX (for plasma) are approved as companion diagnostics for sotorasib (26). However, Guardant360 CDX is not covered by health insurance in many countries, including Japan, especially due to its cost and long turnaround time. On the other hand, although the therascreen PCR kit has the potential to overcome the two drawbacks of the Guardant360 CDX, a more sensitive test method is needed to detect KRAS gene mutations in plasma in the future. In this regard, the peptide nucleic acid-locked nucleic acid polymerase chain reaction (PNA-LNA PCR) clamp has been used to measure EGFR mutations in plasma as well as tumor tissue with high sensitivity (27). A comparison between the QIAGEN therascreen KRAS RGQ PCR kit and the PNA-LNA clamp method for KRAS, which is considered more sensitive, is needed.
In the present study, KRAS mutations in resected tumor tissue were evaluated by both the PNA-LNA PCR clamp method and the therascreen kit. We expected that the PNA-LNA PCR clamp method was superior to the therascreen in detection of KRAS mutations. The frequency of KRAS mutation subtypes and characteristics of patients with KRAS mutations were analyzed. We present this article in accordance with the STROBE reporting checklist (available at https://tlcr.amegroups.com/article/view/10.21037/tlcr-23-15/rc).
Methods
Study design
This was a single-institution retrospective study. Surgically resected tumors from lung adenocarcinoma patients were collected. The PNA-LNA PCR clamp analysis of KRAS G12X mutations was compared with analysis by the therascreen KRAS RGQ kit. The association between KRAS mutation status and patient characteristics and prognosis was evaluated.
Eligible patients
Patients who underwent surgical procedures between June 1, 2016 and September 30, 2019 at the Department of Respiratory Surgery, Iwate Medical University, were eligible for KRAS gene mutation testing if they satisfied the following conditions. (I) Stage I–III disease according to Tumor-Node-Metastasis (TNM) classification 8th edition and histopathological diagnosis of lung adenocarcinoma. (II) It was possible to prepare 5 µm × 5 unstained thin section specimens from paraffin block specimens. Patients with stage IV disease were excluded due to its negative impact on disease-free survival (DFS) and overall survival (OS), and patients with squamous cell lung cancer were excluded due to the extremely small number of KRAS mutations (28,29). KRAS mutations are typically present as a single-driver mutation (30-33). Cases in which driver mutations such as EGFR mutations and anaplastic lymphoma kinase (ALK) mutations were confirmed to be mutually exclusive with KRAS mutations were excluded. However, patients with EGFR mutations who underwent surgery at the same time were used as comparators for KRAS patients for characteristics, DFS, and OS.
Testing methods
Five unstained thin sections of 5 µm × 5 µm were prepared from paraffin block specimens of surgical specimens of the subject patients, and DNA was extracted from sections with QIAamp DNA formalin fixed paraffin embedded (FFPE) Tissue (QIAGEN, Hilden, Germany). The PNA-LNA PCR clamp method was used to measure the KRAS exon G12X point mutation. Although this PCR system is as well established as the method used for EGFR gene mutations (34). This PCR system achieved a detection rate of less than 0.1% by using smaller PCR products and by increasing the number of cycles from 45 to 50. The therascreen®KRAS mutation detection kit was also used to evaluate the concordance with the companion diagnostic agent. DNA extraction and gene mutation testing were performed by LSI Medience Corporation (Tokyo, Japan). This method is also able to detect only the G12X point mutation. All discordant samples required confirmation by Sanger sequencing.
Collection of clinical data
Clinical information on patients who underwent KRAS gene testing, including age, gender, underlying disease, smoking history, programmed death-ligand 1 (PD-L1) expression, clinical stage, postoperative chemotherapy, relapse date, and death date, was collected from electronic medical records. PD-L1 expression was assessed in formalin-fixed, paraffin-embedded specimens at the department of pathology in Iwate Medical University School of Medicine, using the commercially available PD-L1 IHC 22C3 pharmDx assay (Dako North America). The last day of the data collection period was September 30, 2022. As a control group, information was also collected on patients with EGFR mutations who had undergone surgery and were in stages I-III during the period of study. In the investigation of prognosis among KRAS, EGFR, and KRAS wild groups, data on the patients’ sex, age, smoking status, pathological stage, complications, and adjuvant chemotherapies to check imbalance in them.
Endpoints
Endpoints included the frequency of KRAS mutation-positive patients, background factors of KRAS mutation-positive patients, impact of KRAS mutation positivity on DFS and OS, and comparison of KRAS mutation detection between the PNA-LNA PCR clamp method and the therascreen method.
Statistical analysis
All statistical analyses were performed using EZR, a statistical software program that extends the capabilities of R and R Commander. For DFS and OS, Kaplan-Meier curves were created and analyzed using the log-rank test and Cox proportional hazards model, with a two-sided 5% significance level. Bonferroni correction was used when statistical analysis required multigroup comparisons.
Ethical statement
The study was conducted in accordance with the Declaration of Helsinki (as revised in 2013) and was approved by the institutional ethics committee of Iwate Medical University (No. HG2021-002). The study is a retrospective observational study, with no new invasive procedures, and consent was obtained in an opt-out fashion.
Results
From June 1, 2016 to September 30, 2020, 630 lung cancer patients were treated at our institution (Figure 1). There were 499 patients with lung adenocarcinoma, including 219 patients with EGFR mutation, 12 with ALK fusion gene, 33 with stage IV disease, and 38 whose specimens could not be secured, all of whom were excluded from KRAS measurement. Excluding these patients, 197 patients were tested for KRAS mutations.
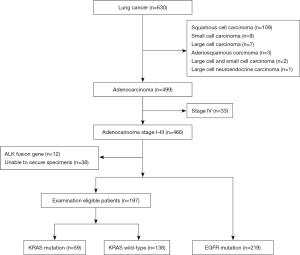
Among the 197 stage I–III adenocarcinomas, excluding those with EGFR mutations and ALK fusion, KRAS G12X mutations were detected in 59 cases (29.9%). Among 466 cases including those with EGFR and ALK mutations, the frequency of KRAS mutations was 12.7%. A rare oncogenic mutation, KRAS A11V, was detected only by the whole adenocarcinoma PNA-LNA PCR clamp method. The KRAS G12C mutation, an indication for treatment with molecular-targeted drugs, was detected in 16 (8.1%) of the adenocarcinomas tested, and in 3.4% of the adenocarcinomas including other driver gene mutations. The highest frequency by gene mutation subtype was 23 (39.0%) cases of G12V, followed by 16 (27.1%) cases of G12C, 12 (20.3%) cases of G12D, 4 (6.8%) cases of G12S, and 2 cases of G12A (Figure 2).
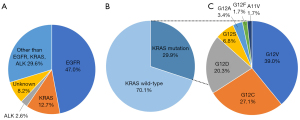
Both PNA-LNA PCR clamp and therascreen were performed, and the concordance rate was 95.9% (189/197) (Table 1). All discordant cases were confirmed by Sanger sequencing (Table 2). For the G12C, G12V, and G12A mutations, both the PNA-LNA PCR clamp and therascreen methods were consistent, but for the G12D and G12S mutations, the PNA-LNA PCR clamp method showed higher detection rates. There was one specimen with discrepant results between G12V by therascreen kit and G12F by PNA-LNA PCR clamp method, and the sequencing result was G12F. The sensitivity and specificity of the PNA-LNA PCR clamp method were 1.00 [95% confidence interval (CI): 1.00–1.00] and 0.95 (95% CI: 0.91–0.98), respectively. The negative predictive value was 1.00 (95% CI: 1.00–1.00).
Table 1
Therascreen | PNA-LNA PCR clamp | |||||||
---|---|---|---|---|---|---|---|---|
Wild-type | A11V | G12A | G12C | G12D | G12F | G12S | G12V | |
Wild-type | 138 | 1† | 5‡ | 1‡ | ||||
A11V | ||||||||
G12A | 2 | |||||||
G12C | 16 | |||||||
G12D | 7 | |||||||
G12F | ||||||||
G12S | 3 | |||||||
G12V | 1† | 23 |
†, genetic mutation testing not covered by therascreen; ‡, positive signal confirmed by therascreen, but cutoff not met and test is negative. Concordance rate: 95.9% (189/197). KRAS, Kirsten rat sarcoma virus; PNA-LNA PCR, peptide nucleic acid-locked nucleic acid polymerase chain reaction.
Table 2
PNA-LNA PCR clamp | Therascreen KRAS mutation detection kit | Sanger sequencing |
---|---|---|
KRAS G12D | KRAS wild-type | KRAS G12D |
KRAS G12D | KRAS wild-type | KRAS G12D |
KRAS G12D | KRAS wild-type | KRAS G12D |
KRAS A11V | KRAS wild-type | KRAS A11V† |
KRAS G12S | KRAS wild-type | KRAS G12S |
KRAS G12D | KRAS wild-type | KRAS G12D |
KRAS G12D | KRAS wild-type | KRAS G12D |
KRAS G12F | KRAS G12V | KRAS G12F† |
†, gene mutation not analyzed by therascreen. PNA-LNA PCR, peptide nucleic acid-locked nucleic acid polymerase chain reaction; KRAS, Kirsten rat sarcoma virus.
We evaluated the background factors among the KRAS mutation-positive group and KRAS mutation-negative group and the EGFR mutation group without KRAS gene testing (Table 3). KRAS G12C-positive patients were more often male, smokers, and had higher expression rates of PD-L1. In terms of smoking status, there was a trend toward more KRAS G12C mutations among heavy smokers. By stage, KRAS mutations were equally frequent in both early and advanced stages of disease. There was also a tendency for KRAS mutations to be more prevalent in patients with high PD-L1 expression, which may be associated with smoking status. In terms of smoking status, KRAS G12C mutations were frequently found in heavy smokers. By staging, KRAS mutations were equally frequent in both early and advanced stages of disease. A tendency for KRAS mutations to be more prevalent in patients with high PD-L1 expression might be associated with smoking status.
Table 3
Characteristics | KRAS wild-type | KRAS mutation | EGFR mutation | ||||||||||
---|---|---|---|---|---|---|---|---|---|---|---|---|---|
All (n=138) |
All (n=59) |
G12C (n=16) | G12V (n=23) | G12D (n=12) | G12A (n=2) | Others (n=6) | All (n=219) | L858R (n=120) | del19 (n=64) | Others (n=35) | |||
Median age, years [range] | 70 [36–84] |
72 [44–85] | 71 [44–85] |
71 [58–84] |
78 [49–85] |
69 [65–73] |
71.5 [69–84] | 70 [43–86] | 70 [51–86] |
71 [43–85] |
72 [48–84] |
||
Age group, n (%) | |||||||||||||
≤60 years | 27 (19.6) | 5 (8.5) | 3 (18.8) | 1 (4.3) | 1 (8.3) | 0 (0) | 0 (0) | 29 (13.2) | 12 (10.0) | 14 (21.9) | 3 (8.6) | ||
61–74 years | 68 (49.3) | 28 (47.5) | 10 (62.5) | 17 (73.9) | 3 (25.0) | 2 (100.0) | 1 (16.7) | 122 (55.7) | 69 (57.5) | 33 (51.6) | 20 (57.1) | ||
≥75 years | 43 (31.2) | 20 (33.9) | 3 (18.8) | 5 (21.7) | 8 (66.7) | 0 (0) | 5 (83.3) | 68 (31.1) | 39 (32.5) | 17 (26.6) | 12 (34.3) | ||
Sex, n (%) | |||||||||||||
Male | 79 (57.2) | 46 (78.0) | 14 (87.5) | 17 (73.9) | 7 (58.3) | 2 (100.0) | 6 (100.0) | 83 (37.9) | 41 (34.2) | 28 (43.8) | 14 (40.0) | ||
Female | 59 (42.8) | 13 (22.0) | 2 (12.5) | 6 (26.1) | 5 (41.7) | 0 (0) | 0 (0) | 136 (62.1) | 79 (65.8) | 36 (56.3) | 21 (60.0) | ||
Smoking history, n (%) | |||||||||||||
Never | 57 (41.3) | 12 (20.3) | 1 (6.3) | 5 (21.7) | 5 (41.7) | 0 (0) | 1 (16.7) | 145 (66.2) | 86 (71.7) | 35 (54.7) | 24 (68.6) | ||
Previous | 50 (36.2) | 28 (47.5) | 8 (50.0) | 9 (39.1) | 6 (50.0) | 2 (100.0) | 3 (50.0) | 55 (25.1) | 28 (23.3) | 17 (26.6) | 10 (28.6) | ||
Current | 31 (22.5) | 19 (32.2) | 7 (43.8) | 9 (39.1) | 1 (8.3) | 0 (0) | 2 (33.3) | 19 (8.7) | 6 (5.0) | 12 (18.8) | 1 (2.9) | ||
Brinkman index, n (%) | |||||||||||||
<600 | 81 (58.7) | 26 (44.1) | 3 (18.8) | 13 (56.5) | 8 (66.7) | 1 (50.0) | 1 (16.7) | 192 (87.7) | 109 (90.8) | 53 (82.8) | 30 (85.7) | ||
600–1,200 | 46 (33.3 | 21 (35.6) | 8 (50.0) | 7 (30.4) | 1 (8.3) | 1 (50.0) | 4 (66.7) | 23 (10.5) | 9 (7.5) | 9 (14.1) | 5 (14.3) | ||
>1,200 | 11 (8.0) | 12 (20.3) | 5 (31.3) | 3 (13.0) | 3 (35.0) | 0 (0) | 1 (16.7) | 4 (1.8) | 2 (1.7) | 2 (3.1) | 0 (0) | ||
Stage, n (%) | |||||||||||||
I | 102 (73.9) | 38 (64.4) | 11 (68.8) | 14 (60.9) | 8 (66.7) | 1 (50.0) | 4 (66.7) | 182 (83.1) | 105 (87.5) | 48 (68.6) | 29 (82.9) | ||
II | 20 (14.5) | 15 (25.4) | 3 (18.8) | 8 (34.8) | 3 (25.0) | 0 (0) | 1 (16.7) | 13 (5.9) | 7 (5.8) | 5 (7.1) | 1 (2.9) | ||
III | 16 (11.6) | 6 (10.2) | 2 (12.5) | 1 (4.3) | 1 (8.3) | 1 (50.0) | 1 (16.7) | 24 (11.0) | 8 (6.7) | 11 (15.7) | 5 (14.3) | ||
PD-L1 status, n (%) | |||||||||||||
Unknown | 19 (13.8) | 4 (6.8) | 0 (0) | 1 (4.3) | 1 (8.3) | 0 (0) | 2 (33.3) | 22 (10.0) | 11 (9.2) | 7 (10.9) | 4 (11.4) | ||
<1% | 70 (50.7) | 33 (55.9) | 6 (37.5) | 14 (60.9) | 9 (75.0) | 2 (100.0) | 2 (33.3) | 132 (60.3) | 78 (65.0) | 32 (50.0) | 22 (62.9) | ||
1–49% | 39 (28.3) | 8 (13.6) | 3 (18.8) | 3 (13.0) | 1 (8.3) | 0 (0) | 1 (16.7) | 50 (22.8) | 26 (21.7) | 18 (28.1) | 6 (17.1) | ||
≥50% | 10 (7.2) | 14 (23.7) | 7 (43.8) | 5 (21.7) | 1 (8.3) | 0 (0) | 1 (16.7) | 15 (6.8) | 5 (4.2) | 7 (10.9) | 3 (8.6) | ||
Adjuvant chemotherapy, n (%) | 51 (37.0) | 22 (37.2) | 5 (31.3) | 11 (47.8) | 5 (41.7) | 0 (0) | 1 (16.7) | 59 (26.9) | 30 (25.0) | 19 (29.7) | 10 (28.6) | ||
Medical history, n (%) | |||||||||||||
COPD | 23 (16.7) | 18 (30.5) | 6 (37.5) | 6 (26.1) | 1 (8.3) | 1 (50.0) | 4 (66.7) | 19 (8.7) | 9 (7.5) | 9 (14.1) | 1 (2.9) | ||
Hypertension | 68 (49.3) | 36 (61.0) | 9 (56.2) | 13 (56.5) | 9 (75.0) | 0 (0) | 5 (83.3) | 107 (48.9) | 53 (44.2) | 33 (51.6) | 21 (60.0) | ||
Diabetes mellitus | 33 (23.9) | 15 (25.4) | 7 (43.8) | 8 (34.8) | 3 (25.0) | 0 (0) | 0 (0) | 35 (16.0) | 18 (15.0) | 9 (14.1) | 8 (22.9) | ||
Dyslipidemia | 31 (22.5) | 13 (22.0) | 4 (25.0) | 4 (17.4) | 3 (25.0) | 0 (0) | 2 (33.3) | 48 (21.9) | 26 (21.7) | 12 (18.8) | 10 (28.6) | ||
Heart failure | 0 (0) | 4 (6.8) | 1 (6.3) | 0 (0) | 2 (16.7) | 1 (50.0) | 0 (0) | 3 (1.4) | 0 (0) | 2 (3.1) | 1 (2.9) | ||
Ischemic heart disease | 0 (0) | 4 (6.8) | 2 (12.5) | 0 (0) | 1 (8.3) | 0 (0) | 1 (16.7) | 6 (2.7) | 6 (5.0) | 0 (0) | 0 (0) | ||
Arrhythmia | 11 (8.0) | 10 (16.9) | 0 (0) | 5 (21.7) | 2 (16.7) | 1 (50.0) | 2 (33.3) | 22 (10.0) | 10 (8.3) | 7 (10.9) | 5 (14.3) | ||
Cerebrovascular disease | 11 (8.0) | 7 (11.9) | 1 (6.3) | 2 (8.7) | 2 (16.7) | 1 (50.0) | 1 (16.7) | 19 (8.7) | 11 (9.2) | 6 (9.4) | 2 (5.7) | ||
Autoimmune disorder | 4 (2.9) | 1 (1.7) | 0 (0) | 0 (0) | 1 (8.3) | 0 (0) | 0 (0) | 12 (5.5) | 9 (7.5) | 0 (0) | 3 (8.6) | ||
Malignant tumor | 31 (22.5) | 15 (25.4) | 6 (37.5) | 8 (34.8) | 1 (8.3) | 0 (0) | 0 (0) | 56 (25.6) | 34 (28.3) | 14 (21.9) | 8 (22.9) |
KRAS, Kirsten rat sarcoma virus; EGFR, epidermal growth factor receptor; PD-L1, programmed death ligand 1; COPD, chronic obstructive pulmonary disease.
In this study, there were no statistically significant differences in DFS or OS between patients with and without genetic mutations (Figure 3, Table 4, Table 5). However, when divided by each stage, patients with stage II EGFR mutations tended to have shorter DFS (Figures S1-S3), possibly due to the small number of cases. There were no significant differences in DFS and OS between patients with KRAS G12 and other subtypes of KRAS mutations (Figure S4).
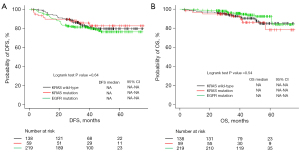
Table 4
Characteristics | Univariate analysis | Multivariate analysis | |||||
---|---|---|---|---|---|---|---|
HR | 95% CI | P value | HR | 95% CI | P value | ||
Age, years | |||||||
61–74 vs. ≤60 | 1.56 | 0.69–3.50 | 0.282 | ||||
≥75 vs. ≤60 | 1.60 | 0.68–3.75 | 0.279 | ||||
≥75 vs. 61–74 | 1.03 | 0.61–1.74 | 0.921 | ||||
Sex | |||||||
Male vs. female | 1.62 | 0.99–2.65 | 0.054 | ||||
Stage | |||||||
II vs. I | 3.13 | 1.65–5.95 | <0.001 | 2.07 | 1.01–4.24 | 0.047 | |
III vs. I | 7.16 | 4.10–12.5 | <0.001 | 4.47 | 2.30–8.69 | <0.001 | |
III vs. II | 2.29 | 1.14–4.60 | 0.021 | 2.16 | 1.07–4.37 | 0.032 | |
PD-L1 status | |||||||
1–49% vs. <1% | 2.12 | 1.23–3.66 | 0.007 | 1.55 | 0.88–2.72 | 0.131 | |
≥50% vs. <1% | 3.48 | 1.84–6.58 | <0.001 | 2.06 | 1.06–4.02 | 0.033 | |
≥50% vs. 1–49% | 1.64 | 0.84–3.19 | 0.144 | ||||
Smoking history | |||||||
Previous vs. never | 0.97 | 0.53–1.75 | 0.910 | ||||
Current vs. never | 2.23 | 1.25–3.96 | 0.006 | 1.40 | 0.77–2.55 | 0.227 | |
Current vs. previous | 2.31 | 1.20–4.43 | 0.012 | 1.82 | 0.94–3.54 | 0.076 | |
Brinkman index | |||||||
600–1,200 vs. <600 | 1.47 | 0.85–2.52 | 0.165 | ||||
>1,200 vs. <600 | 1.23 | 0.44–3.42 | 0.695 | ||||
>1,200 vs. 600–1,200 | 0.84 | 0.28–2.46 | 0.746 | ||||
Mutation | |||||||
KRAS wild-type vs. EGFR mutation | 1.02 | 0.60–1.74 | 0.936 | ||||
KRAS wild-type vs. KRAS mutation | 1.08 | 0.50–2.34 | 0.849 | ||||
KRAS mutation vs. EGFR mutation | 0.95 | 0.46–1.97 | 0.887 | ||||
Medical history | |||||||
Adjuvant chemotherapy | 3.34 | 2.05–5.44 | <0.001 | 1.68 | 0.93–3.04 | 0.084 | |
COPD | 1.98 | 1.11–3.52 | 0.020 | 1.20 | 0.64–2.25 | 0.574 | |
Hypertension | 0.80 | 0.49–1.30 | 0.376 | ||||
Diabetes mellitus | 0.89 | 0.48–1.67 | 0.719 | ||||
Dyslipidemia | 0.83 | 0.45–1.52 | 0.543 | ||||
Heart failure | 0.78 | 0.11–5.62 | 0.805 | ||||
Ischemic heart disease | 0.35 | 0.05–2.52 | 0.296 | ||||
Arrhythmia | 1.08 | 0.49–2.36 | 0.855 | ||||
Cerebrovascular disease | 0.98 | 0.40–2.45 | 0.974 | ||||
Autoimmune disorder | 0.32 | 0.04–2.31 | 0.260 | ||||
Malignant tumor | 0.80 | 0.45–1.45 | 0.468 |
PD-L1, programmed death ligand 1; KRAS, Kirsten rat sarcoma virus; EGFR, epidermal growth factor receptor; COPD, chronic obstructive pulmonary disease; HR, hazard ratio; CI, confidence interval.
Table 5
Characteristics | Univariate analysis | Multivariate analysis | |||||
---|---|---|---|---|---|---|---|
HR | 95% CI | P value | HR | 95% CI | P value | ||
Age, years | |||||||
61–74 vs. ≤60 | 1.48 | 0.43–5.17 | 0.535 | ||||
≥75 vs. ≤60 | 1.90 | 0.53–6.81 | 0.325 | ||||
≥75 vs. 61–74 | 1.28 | 0.58–2.82 | 0.543 | ||||
Sex | |||||||
Male vs. female | 1.55 | 0.73–3.29 | 0.249 | ||||
Stage | |||||||
II vs. I | 2.15 | 0.70–6.61 | 0.180 | ||||
III vs. I | 8.20 | 3.67–18.3 | <0.001 | 7.31 | 3.18–16.8 | <0.001 | |
III vs. II | 3.80 | 3.67–18.3 | 0.022 | 3.73 | 1.18–11.7 | 0.025 | |
PD-L1 status | |||||||
1–49% vs. <1% | 1.79 | 0.76–4.19 | 0.180 | ||||
≥50% vs. <1% | 2.77 | 1.05–7.31 | 0.039 | 1.44 | 0.51–4.06 | 0.493 | |
≥50% vs. 1–49% | 1.55 | 0.55–4.36 | 0.406 | ||||
Smoking history | |||||||
Previous vs. never | 1.54 | 0.65–3.63 | 0.323 | ||||
Current vs. never | 2.21 | 0.86–5.71 | 0.101 | ||||
Current vs. previous | 1.44 | 0.55–3.78 | 0.463 | ||||
Brinkman index | |||||||
600–1,200 vs. <600 | 1.34 | 0.59–3.07 | 0.485 | ||||
>1,200 vs. <600 | 0.86 | 0.11–6.44 | 0.883 | ||||
>1,200 vs. 600–1,200 | 0.64 | 0.08–5.14 | 0.675 | ||||
Mutation | |||||||
KRAS wild-type vs. EGFR mutation | 1.46 | 0.62–3.43 | 0.389 | ||||
KRAS wild-type vs. KRAS mutation | 0.61 | 0.23–1.60 | 0.311 | ||||
KRAS mutation vs. EGFR mutation | 2.40 | 0.93–6.20 | 0.070 | ||||
Medical history | |||||||
Adjuvant chemotherapy | 1.35 | 0.62–2.92 | 0.451 | ||||
COPD | 1.96 | 0.79–4.85 | 0.145 | ||||
Hypertension | 1.26 | 0.59–2.66 | 0.552 | ||||
Diabetes mellitus | 2.32 | 1.07–5.03 | 0.033 | 2.36 | 1.08–5.16 | 0.031 | |
Dyslipidemia | 1.63 | 0.74–3.61 | 0.227 | ||||
Heart failure | Inf | ||||||
Ischemic heart disease | Inf | ||||||
Arrhythmia | 0.34 | 0.05–2.49 | 0.287 | ||||
Cerebrovascular disease | 2.27 | 0.78–6.59 | 0.130 | ||||
Autoimmune disorder | Inf | ||||||
Malignant tumor | 0.65 | 0.25–1.70 | 0.378 |
PD-L1, programmed death ligand 1; KRAS, Kirsten rat sarcoma virus; EGFR, epidermal growth factor receptor; COPD, chronic obstructive pulmonary disease; HR, hazard ratio; Cl, confidence interval; Inf, infinity.
DFS showed significant differences in univariate analysis for stage, PD-L1 (1–49 vs. <1, ≥50 vs. <1), smoking history (current vs. never, current vs. previous), adjuvant chemotherapy, and history of chronic obstructive pulmonary disease (COPD), but only stage II vs. I [hazard ratios (HR) =2.07, 95% CI: 1.01–4.24, P value =0.047], III vs. I (HR =4.47, 95% CI: 2.30–8.69, P value <0.001), III vs. II (HR =2.16, 95% CI: 1.07–4.37, P value =0.032) and PD-L1 ≥50 vs. <1 (HR =2.06, 95% CI: 1.06–4.02, P value =0.033) in multivariate analysis (Table 4).
For OS, univariate analysis showed significant differences in stage III vs. I, III vs. II, PD-L1 ≥50 vs. <1, and diabetes mellitus, while multivariate analysis showed significant differences in stage III vs. I (HR =7.31, 95% CI: 3.18–16.8, P value <0.001), III vs. II (HR =3.73, 95% CI: 1.18–11.7, P value =0.025), and diabetes mellitus (HR =2.36, 95% CI: 1.08–5.16, P value =0.031) (Table 5).
Discussion
In this study, KRAS mutations in resected tumor specimens (n=197) were evaluated by both the PNA-LNA PCR clamp method and the therascreen PCR kit. Discrepant cases were confirmed by sequencing. The overall frequency of KRAS in adenocarcinoma without stage IV was 12.7%, with KRAS G12C at 3.4%. G12V was the most common subtype, followed by G12C and G12D.
In the two PCRs used in this study, G12C was matched in all cases, but other subtypes were detected more frequently by the PNA-LNA PCR clamp method compared to the therascreen PCR kit. This result may be related to the fact that the minimum detection sensitivity of the PNA-LNA PCR clamp method is about 0.1%. In comparison, the detection sensitivity of the therascreen PCR kit is only about 1%.
KRAS mutations are found in approximately 30% of lung adenocarcinomas and 4% of lung squamous cell carcinomas according to Western databases (12). In Japanese data, KRAS mutations are found in 9.7% of lung adenocarcinomas (22). This difference was attributed to the mutually exclusive nature of KRAS mutations with other driver mutations (30-33). KRAS mutations are less frequent in East Asia, where EGFR mutations are more common, and KRAS mutations are more common in Europe and the United States, where EGFR mutations are less frequent. In the present study, KRAS mutations were found in 12.7% of tumors, which is consistent with the frequency in Japanese patients.
Regarding KRAS mutation subtypes, a German prospective cohort study of advanced non-small cell lung cancer reported that among patients with KRAS mutations, 38.9% were positive for KRAS G12C mutation, 21.2% for G12V mutation, and 13.9% for G12D (35). In the present study, the frequency of KRAS mutations was consistent with that in previous Japanese reports (22,23). However, the frequency of subtypes was different. In previous reports, KRAS G12C was the most common, but in this study, G12V was the most common, followed by G12C.
In previous reports, KRAS G12C was the most common, but in this study, G12V was the most common. The reason for this was unclear, and might be related to the fact that this study was conducted in an area with a high rate of smoking. KRAS mutations, in contrast to EGFR mutations, were more frequent in smokers (36,37). Several studies have shown an association between smoking and KRAS mutations: the guanine to thymine transversions resulting in KRAS G12C and KRAS G12V are more commonly found in past or current smokers; the guanine to adenine transition resulting in KRAS G12D is more common in non-smokers (36,38,39). In this study, the G12C and G12V mutations were more prevalent in heavy smokers, while the G12D mutation was not.
In this study, DFS was affected by PD-L1 in multivariate analysis, but OS was not affected by PD-L1. In a previously reported meta-analysis of 15 studies and 3,790 patients on prognosis in early resected NSCLC, PD-L1 expression was associated with significantly shorter DFS (HR =1.56, 95% CI: 1.18–2.05, P value <0.01), and significantly worse OS (HR =1.68, 95% CI: 1.29–2.18, P value <0.01) (40). These results differed from those of the present study. Also, there were no statistically significant differences in DFS and OS by genetic variants in this study. However, previously reported meta-analyses have shown that surgically treated NSCLC patients with EGFR mutations tend to have prolonged DFS and OS. Others have shown that KRAS mutations predict worse DFS and OS in resected NSCLC patients (41). This difference could be attributed to the shorter observation period in this study, fewer patients who relapsed, or fewer patients who received chemotherapy after relapse.
The prognosis of patients with KRAS mutations has been the subject of various studies. There are several reports of KRAS mutations having poor prognosis (42,43). On the other hand, a number of reports have found that KRAS mutations are not a poor prognostic factor (35,44). Many of these studies are retrospective cohort studies or were conducted before the advent of immune checkpoint inhibitors (ICI). In this study, lung cancer patients with KRAS mutations had a high frequency of PD-L1 expression. Similarly, several studies have shown that patients with KRAS mutation-positive tumors have higher PD-L1 expression and tumor mutation burden compared to KRAS wild-type patients (23,35,45). KRAS mutated tumors co-mutated with TP53 or cyclin dependent kinase inhibitor 2B (CDKN2a/B) mutations are more responsive to treatment with immune checkpoint inhibitors (46). Treatment strategies including ICI are promising candidates for KRAS mutated lung cancer. ICI and KRAS G12C inhibitors such as sotorasib may alter the prognosis of KRAS-positive patients in the future.
In this study, KRAS gene mutations were detected using the PNA-LNA PCR clamp method, a highly sensitive detection method, together with the companion diagnostic therascreen kit. The two tests were all consistent for the G12C mutation, which is an indication for molecular targeted therapy. For mutations other than G12C mutation, the PNA-LNA PCR clamp had a higher detection rate. The PNA-LNA PCR clamp is more sensitive than the therascreen kit and is currently being considered for application to plasma gene mutation analysis. We have utilized the high sensitivity of the PNA-LNA PCR clamp method to monitor plasma EGFR gene mutations as a biomarker search in several clinical trials (27,47). We are now investigating the use of this sensitive and inexpensive method for plasma analysis of KRAS as well.
A limitation of this study is that it is a single-center surgical specimen study and has a short observation period. Although novel molecular-targeted agents are indicated for stage IV NSCLC, the target population in this study had earlier stage cancer. In addition, postoperative and recurrence-free survival was used as one of the outcomes, rather than PFS. Further studies will be needed for advanced stage lung cancer.
Conclusions
This study examined KRAS frequency and its background factors in Japan using surgical specimens, and found a trend toward higher frequency in smokers and males; KRAS mutations did not affect DFS and OS.
Both the PNA-LNA PCR clamp and therascreen kit were consistent in detecting G12C mutations; the PNA-LNA PCR clamp method was more sensitive and may be applicable to multiple situations in the detection of KRAS mutations in the future. Further studies in advanced lung cancer are warranted.
Acknowledgments
Funding: None.
Footnote
Reporting Checklist: The authors have completed the STROBE reporting checklist. Available at https://tlcr.amegroups.com/article/view/10.21037/tlcr-23-15/rc
Data Sharing Statement: Available at https://tlcr.amegroups.com/article/view/10.21037/tlcr-23-15/dss
Peer Review File: Available at https://tlcr.amegroups.com/article/view/10.21037/tlcr-23-15/prf
Conflicts of Interest: All authors have completed the ICMJE uniform disclosure form (available at https://tlcr.amegroups.com/article/view/10.21037/tlcr-23-15/coif). HT is a current employee of LSI Medience, Inc., which is a for-profit company that deals with clinical testing and research equipment and reagents. The other authors have no conflicts of interest to declare.
Ethical Statement: The authors are accountable for all aspects of the work in ensuring that questions related to the accuracy or integrity of any part of the work are appropriately investigated and resolved. The study was conducted in accordance with the Declaration of Helsinki (as revised in 2013) and was approved by the institutional ethics committee of Iwate Medical University (No. HG2021-002). The study is a retrospective observational study, with no new invasive procedures, and consent was obtained in an opt-out fashion.
Open Access Statement: This is an Open Access article distributed in accordance with the Creative Commons Attribution-NonCommercial-NoDerivs 4.0 International License (CC BY-NC-ND 4.0), which permits the non-commercial replication and distribution of the article with the strict proviso that no changes or edits are made and the original work is properly cited (including links to both the formal publication through the relevant DOI and the license). See: https://creativecommons.org/licenses/by-nc-nd/4.0/.
References
- Denis MG, Bennouna J. Osimertinib for Front-Line Treatment of Locally Advanced or Metastatic EGFR-Mutant NSCLC Patients: Efficacy, Acquired Resistance and Perspectives for Subsequent Treatments. Cancer Manag Res 2020;12:12593-602. [Crossref] [PubMed]
- Tabbò F, Passiglia F, Novello S. Upfront Management of ALK-Rearranged Metastatic Non-small Cell Lung Cancer: One Inhibitor Fits All? Curr Oncol Rep 2021;23:10. [Crossref] [PubMed]
- Drilon A, Jenkins C, Iyer S, et al. ROS1-dependent cancers – biology, diagnostics and therapeutics. Nat Rev Clin Oncol 2021;18:35-55. [Crossref] [PubMed]
- Malapelle U, Rossi G, Pisapia P, et al. BRAF as a positive predictive biomarker: Focus on lung cancer and melanoma patients. Crit Rev Oncol Hematol 2020;156:103118. [Crossref] [PubMed]
- Paik PK, Felip E, Veillon R, et al. Tepotinib in Non-Small-Cell Lung Cancer with MET Exon 14 Skipping Mutations. N Engl J Med 2020;383:931-43. [Crossref] [PubMed]
- Drilon A, Oxnard GR, Tan DSW, et al. Efficacy of Selpercatinib in RET Fusion-Positive Non-Small-Cell Lung Cancer. N Engl J Med 2020;383:813-24. [Crossref] [PubMed]
- Doebele RC, Drilon A, Paz-Ares L, et al. Entrectinib in patients with advanced or metastatic NTRK fusion-positive solid tumours: integrated analysis of three phase 1-2 trials. Lancet Oncol 2020;21:271-82. [Crossref] [PubMed]
- Popescu NC, Amsbaugh SC, DiPaolo JA, et al. Chromosomal localization of three human ras genes by in situ molecular hybridization. Somat Cell Mol Genet 1985;11:149-55. [Crossref] [PubMed]
- Román M, Baraibar I, López I, et al. KRAS oncogene in non-small cell lung cancer: clinical perspectives on the treatment of an old target. Mol Cancer 2018;17:33. [Crossref] [PubMed]
- Uras IZ, Moll HP, Casanova E. Targeting KRAS Mutant Non-Small-Cell Lung Cancer: Past, Present and Future. Int J Mol Sci 2020;21:4325. [Crossref] [PubMed]
- Simanshu DK, Nissley DV, McCormick F. RAS Proteins and Their Regulators in Human Disease. Cell 2017;170:17-33. [Crossref] [PubMed]
- Prior IA, Hood FE, Hartley JL. The Frequency of Ras Mutations in Cancer. Cancer Res 2020;80:2969-74. [Crossref] [PubMed]
- Luo J. KRAS mutation in pancreatic cancer. Semin Oncol 2021;48:10-8. [Crossref] [PubMed]
- Seo KY, Jelinsky SA, Loechler EL. Factors that influence the mutagenic patterns of DNA adducts from chemical carcinogens. Mutat Res 2000;463:215-46. [Crossref] [PubMed]
- Muñoz-Maldonado C, Zimmer Y, Medová M. A Comparative Analysis of Individual RAS Mutations in Cancer Biology. Front Oncol 2019;9:1088. [Crossref] [PubMed]
- Takács T, Kudlik G, Kurilla A, et al. The effects of mutant Ras proteins on the cell signalome. Cancer Metastasis Rev 2020;39:1051-65. [Crossref] [PubMed]
- Griesinger F, Eberhardt W, Nusch A, et al. Biomarker testing in non-small cell lung cancer in routine care: Analysis of the first 3,717 patients in the German prospective, observational, nation-wide CRISP Registry (AIO-TRK-0315). Lung Cancer 2021;152:174-84. [Crossref] [PubMed]
- Barlesi F, Mazieres J, Merlio JP, et al. Routine molecular profiling of patients with advanced non-small-cell lung cancer: results of a 1-year nationwide programme of the French Cooperative Thoracic Intergroup (IFCT). Lancet 2016;387:1415-26. [Crossref] [PubMed]
- Roberts PJ, Stinchcombe TE, Der CJ, et al. Personalized medicine in non-small-cell lung cancer: is KRAS a useful marker in selecting patients for epidermal growth factor receptor-targeted therapy? J Clin Oncol 2010;28:4769-77. [Crossref] [PubMed]
- Tan AC, Lai GGY, Tan GS, et al. Utility of incorporating next-generation sequencing (NGS) in an Asian non-small cell lung cancer (NSCLC) population: Incremental yield of actionable alterations and cost-effectiveness analysis. Lung Cancer 2020;139:207-15. [Crossref] [PubMed]
- Jia Y, Jiang T, Li X, et al. Characterization of distinct types of KRAS mutation and its impact on first-line platinum-based chemotherapy in Chinese patients with advanced non-small cell lung cancer. Oncol Lett 2017;14:6525-32. [Crossref] [PubMed]
- Saito M, Shiraishi K, Kunitoh H, et al. Gene aberrations for precision medicine against lung adenocarcinoma. Cancer Sci 2016;107:713-20. [Crossref] [PubMed]
- Tamiya Y, Matsumoto S, Zenke Y, et al. Large-scale linic-genomic profile of non-small cell lung cancer with KRAS G12C: Results from LC-SCRUM-Asia study. Lung Cancer 2023;176:103-11. [Crossref] [PubMed]
- Hong DS, Fakih MG, Strickler JH, et al. KRAS(G12C) Inhibition with Sotorasib in Advanced Solid Tumors. N Engl J Med 2020;383:1207-17. [Crossref] [PubMed]
- Skoulidis F, Li BT, Dy GK, et al. Sotorasib for Lung Cancers with KRAS p.G12C Mutation. N Engl J Med 2021;384:2371-81. [Crossref] [PubMed]
- Bauml JM, Li BT, Velcheti V, et al. Clinical validation of Guardant360 CDx as a blood-based companion diagnostic for sotorasib. Lung Cancer 2022;166:270-8. [Crossref] [PubMed]
- Watanabe K, Fukuhara T, Tsukita Y, et al. EGFR Mutation Analysis of Circulating Tumor DNA Using an Improved PNA-LNA PCR Clamp Method. Can Respir J 2016;2016:5297329. [Crossref] [PubMed]
- Clinical Lung Cancer Genome Project (CLCGP). Network Genomic Medicine (NGM). A genomics-based classification of human lung tumors. Sci Transl Med 2013;5:209ra153. [Crossref] [PubMed]
- Comprehensive genomic characterization of squamous cell lung cancers. Nature 2012;489:519-25. [Crossref] [PubMed]
- Imielinski M, Berger AH, Hammerman PS, et al. Mapping the hallmarks of lung adenocarcinoma with massively parallel sequencing. Cell 2012;150:1107-20. [Crossref] [PubMed]
- Lee B, Lee T, Lee SH, et al. Clinicopathologic characteristics of EGFR, KRAS, and ALK alterations in 6,595 lung cancers. Oncotarget 2016;7:23874-84. [Crossref] [PubMed]
- Li S, Li L, Zhu Y, et al. Coexistence of EGFR with KRAS, or BRAF, or PIK3CA somatic mutations in lung cancer: a comprehensive mutation profiling from 5125 Chinese cohorts. Br J Cancer 2014;110:2812-20.
- Jordan EJ, Kim HR, Arcila ME, et al. Prospective Comprehensive Molecular Characterization of Lung Adenocarcinomas for Efficient Patient Matching to Approved and Emerging Therapies. Cancer Discov 2017;7:596-609. [Crossref] [PubMed]
- Nagai Y, Miyazawa H. Genetic heterogeneity of the epidermal growth factor receptor in non-small cell lung cancer cell lines revealed by a rapid and sensitive detection system, the peptide nucleic acid-locked nucleic acid PCR clamp. Cancer Res 2005;65:7276-82. [Crossref] [PubMed]
- Sebastian M, Eberhardt WEE, Hoffknecht P, et al. KRAS G12C-mutated advanced non-small cell lung cancer: A real-world cohort from the German prospective, observational, nation-wide CRISP Registry (AIO-TRK-0315). Lung Cancer 2021;154:51-61. [Crossref] [PubMed]
- Dogan S, Shen R, Ang DC, et al. Molecular epidemiology of EGFR and KRAS mutations in 3,026 lung adenocarcinomas: higher susceptibility of women to smoking-related KRAS-mutant cancers. Clin Cancer Res 2012;18:6169-77. [Crossref] [PubMed]
- El Osta B, Behera M, Kim S, et al. Characteristics and Outcomes of Patients With Metastatic KRAS-Mutant Lung Adenocarcinomas: The Lung Cancer Mutation Consortium Experience. J Thorac Oncol 2019;14:876-89. [Crossref] [PubMed]
- Karachaliou N, Mayo C, Costa C, et al. KRAS mutations in lung cancer. Clin Lung Cancer 2013;14:205-14. [Crossref] [PubMed]
- Riely GJ, Marks J, Pao W. KRAS mutations in non-small cell lung cancer. Proc Am Thorac Soc 2009;6:201-5. [Crossref] [PubMed]
- Shi T, Zhu S, Guo H, et al. The Impact of Programmed Death-Ligand 1 Expression on the Prognosis of Early Stage Resected Non-Small Cell Lung Cancer: A Meta-Analysis of Literatures. Front Oncol 2021;11:567978. [Crossref] [PubMed]
- Zhang SM, Zhu QG, Ding XX, et al. Prognostic value of EGFR and KRAS in resected non-small cell lung cancer: a systematic review and meta-analysis. Cancer Manag Res 2018;10:3393-404. [Crossref] [PubMed]
- Nadal E, Chen G, Prensner JR, et al. KRAS-G12C mutation is associated with poor outcome in surgically resected lung adenocarcinoma. J Thorac Oncol 2014;9:1513-22. [Crossref] [PubMed]
- Liu SY, Sun H, Zhou JY, et al. Clinical characteristics and prognostic value of the KRAS G12C mutation in Chinese non-small cell lung cancer patients. Biomark Res 2020;8:22. [Crossref] [PubMed]
- Villaruz LC, Socinski MA, Cunningham DE, et al. The prognostic and predictive value of KRAS oncogene substitutions in lung adenocarcinoma. Cancer 2013;119:2268-74. [Crossref] [PubMed]
- Arbour KC, Rizvi H, Plodkowski AJ, et al. Treatment Outcomes and Clinical Characteristics of Patients with KRAS-G12C-Mutant Non-Small Cell Lung Cancer. Clin Cancer Res 2021;27:2209-15. [Crossref] [PubMed]
- Skoulidis F, Goldberg ME, Greenawalt DM, et al. STK11/LKB1 Mutations and PD-1 Inhibitor Resistance in KRAS-Mutant Lung Adenocarcinoma. Cancer Discov 2018;8:822-35. [Crossref] [PubMed]
- Fukuhara T, Saito H, Furuya N, et al. Evaluation of plasma EGFR mutation as an early predictor of response of erlotinib plus bevacizumab treatment in the NEJ026 study. EBioMedicine 2020;57:102861. [Crossref] [PubMed]