HSP90 expression is associated with outcome in pulmonary carcinoid tumor patients
Highlight box
Key findings
• HSP90AB1 expression was significantly elevated in metastatic pulmonary carcinoid (PC) tumors.
• Increased HSP90 protein expression was associated with shorter disease-specific survival and increased risk of disease-specific death.
What is known and what is new?
• HSP90 proteins are molecular chaperones, and their main function is to inhibit the aggregation and facilitate the proper folding of the polypeptide chain of newly synthesized proteins. They are involved in the genesis and progression of cancer.
• HSP90AB1 expression was higher in metastatic PC tumors than in nonmetastatic or adjacent lung tissue. HSP90 immunohistochemical expression was associated with disease-specific death.
What is the implication, and what should change now?
• HSP90 expression could be used as a prognostic biomarker for PC tumors.
• In the future, inhibition of HSP90 may possess therapeutic potential in management of PC tumor patients.
Introduction
Pulmonary neuroendocrine tumors (NETs) account for approximately 20% of all cases of lung malignancy. They are a heterogeneous group of neoplasms ranging from low- and intermediate-grade carcinoid tumors to high-grade large-cell neuroendocrine carcinoma (LCNEC) and small-cell carcinoma (1). Pulmonary carcinoid (PC) tumors are rare tumors with an incidence of 0.2–2 persons per 100,000 population per year, but both the incidence and prevalence of PCs have markedly increased in recent years (1,2).
PCs are divided into typical carcinoids (TCs) and atypical carcinoids (ACs) based on histological criteria (1). Currently, the only curative treatment is complete surgical excision; however, there is no curative treatment for metastatic disease (2). Despite this, PC tumor patients generally have an indolent clinical course and favorable prognosis, especially when they undergo resection, and exhibit a 5-year survival rate of 90% (3,4). However, both TC and AC are malignant tumors with the potential to metastasize. The main limitation of the current tools is the inability to distinguish between indolent and aggressive tumors to better tailor the follow-up of patients. Additionally, new treatment options for inoperable disease are needed.
Heat shock proteins (HSPs) consist of several families of cytoplasmic proteins. Most of them belong to the group of molecular chaperones, and the main function of these proteins is to inhibit the aggregation and to facilitate the proper folding of the polypeptide chain of newly synthesized and stress-denatured proteins (5). The HSP90 family of proteins includes five main members, of which the two most abundant are cytosolic protein isoforms HSPC1 (also called HSP90AA1 or HSP90-alpha) and HSPC3 (HSP90AB1 or HSP90-beta). Stress-inducible HSCP1 and constitutively expressed HSPC3 are paralogs of the HSP90A gene, sharing 86% identity and approximately 93% sequence similarity (6).
HSP90 proteins have a role in chaperone-specific functions but also facilitate signaling through several pathways that are also essential for tumor growth and contribute to RNA synthesis and processing (7). They promote tumor progression since several cancer proteins need HSP90 machinery and chaperones for their folding and maturation (7,8). Indeed, HSP90 proteins are involved in the genesis and progression of cancer in several ways, due to which they can be linked with various hallmarks of cancer (9-11).
Increased expression of HSP90 is associated with tumor progression and poor prognosis in breast, thyroid and lung cancers (12-14). NETs are less studied, but there is evidence that high cytoplasmic expression of HSP90 is linked to early disease recurrence in patients with gastroenteropancreatic neuroendocrine tumors (GEP-NETs) (15). Since the inhibition of HSP90 has more significant effects on cancer cells than on normal cells, this approach has high potential in the treatment of cancers (8,15,16). Thus, the utility of HSP90 as a drug target in NETs is a more studied subject, and reports investigating PCs, GEP-NETs and small-intestinal NETs (SI-NETs) are available (16-19).
The objective of this study was to identify biomarkers for PC tumors using RNA sequencing and immunohistochemistry. RNA sequencing was first used to detect genes with higher expression in (I) PC tumors than in adjacent lung tissue, (II) AC than in TC, and (III) metastasized than in nonmetastasized tumors. The diagnostic potential of the biomarkers was assessed using immunohistochemistry. We present this article in accordance with the REMARK reporting checklist (available at https://tlcr.amegroups.com/article/view/10.21037/tlcr-23-304/rc).
Methods
Patient cohort and tumor samples
This retrospective study included the majority of previously described patients who were treated surgically between January 1990 and August 2013 in the Helsinki University Hospital (HUH, Helsinki, Finland) (20). Of the 128 patients with sufficient amounts of formalin-fixed paraffin-embedded (FFPE) primary tumor tissue sample left for either RNA sequencing, immunohistochemistry or both, 95 had a TC tumor, and 33 had an AC tumor (Table 1). Twelve metastasis samples were also included in RNA sequencing, and 10 tumor-adjacent lung tissue samples served as reference. This study was conducted in accordance with the Declaration of Helsinki (as revised in 2013). The study was approved by the Ethics Committee IV of HUH (HUS/1258/2020) and individual consent for this retrospective analysis was waived.
Table 1
Variables | TC (n=95) | AC (n=33) | All (n=128) |
---|---|---|---|
Sex, n [%] | |||
Male | 29 [31] | 17 [52] | 46 [36] |
Female | 66 [69] | 16 [48] | 82 [64] |
Age (years) | |||
Mean | 52 | 55 | 53 |
Median | 53 | 55 | 55 |
Range | 19–84 | 23–77 | 19–84 |
Tumor size (cm), n [%] | |||
≤1 | 29 [31] | 10 [30] | 39 [31] |
1.1–2.9 | 56 [59] | 15 [46] | 71 [55] |
≥3 | 10 [10] | 8 [24] | 18 [14] |
Nodal involvement at diagnosis, n [%] | |||
Yes | 3 [3] | 6 [18] | 9 [7] |
No | 67 [71] | 24 [73] | 91 [71] |
Not examined | 25 [26] | 3 [9] | 28 [22] |
Distant metastasis, n [%] | |||
At diagnosis | 0 [0] | 2 [6] | 2 [2] |
During follow-up | 6 [6] | 5 [15] | 11 [9] |
Surgical procedure, n [%] | |||
Lobectomy | 40 [49] | 17 [55] | 57 [51] |
Sleeve resection | 14 [17] | 8 [26] | 22 [19] |
Segmentectomy | 14 [17] | 1 [3] | 15 [13] |
Wedge resection | 9 [11] | 1 [3] | 10 [9] |
Bilobectomy | 1 [1] | 2 [6] | 3 [3] |
Enucleation | 3 [4] | 0 [0] | 3 [3] |
Pneumoectomy | 0 [0] | 2 [6] | 2 [2] |
Not available | 14 | 2 | 16 |
Neoadjuvant treatment, n | |||
Chemotherapy | 0 | 0 | 0 |
Radiotherapy | 0 | 1 | 1 |
Both | 0 | 1 | 1 |
Adjuvant treatment†, n | |||
Chemo/radio therapy only | 1 | 3 | 4 |
SSAs only | 1 | 1 | 2 |
SSAs + chemotherapy | 0 | 1 | 1 |
SSAs + PRRT | 1 | 1 | 2 |
SSAs + PRRT + chemo/radiotherapy | 1 | 1 | 2 |
Ki-67 labeling index, n [%] | |||
<1% | 43 [46] | 10 [30] | 53 [42] |
1–2% | 43 [46] | 18 [54] | 61 [48] |
>2% | 7 [8] | 5 [16] | 12 [10] |
Not available | 2 | 2 |
†, adjuvant treatments were given only for metastatic disease. TC, typical carcinoid tumor; AC, atypical carcinoid tumor; SSA, somatostatin analog; PRRT, peptide receptor radionuclide therapy.
RNA extraction
Total RNA was extracted from FFPE tissue samples (4×1 mm cores in diameter) by using a QIAsymphony method (Qiagen Gmbh, Hilden, Germany). Paraffin was removed by pretreating the cores with Qiagen deparaffinization solution (Qiagen, ref. 939018), after which the cores were lysed in buffer PKD (Qiagen, ref. 1034963) with protease K (Qiagen, ref. 19133). RNA extraction was performed in a QIAsymphony SP instrument (Qiagen) with a QIAsymphony RNA kit (Qiagen, ref. 931636) according to the manufacturer’s protocol. DNAse and DNase booster buffer (Qiagen, ref. 1064143) were used.
Sequencing library preparation and 3' RNA sequencing
Strand-specific NGS libraries were prepared with a QuantSeq 3' mRNA-Seq Library kit (Lexogen, Vienna, Austria, cat. No. 015.96), of which sequences close to the 3' end of polyadenylated RNAs were targeted. Sequencing was performed as single-end runs with a NovaSeq 6000 instrument (Illumina Inc., San Diego, CA, USA) using the NovaSeq S1 XP NS1-200 protocol (Illumina Inc.). RNA sequencing runs were further processed using BlueBee® (Illumina Inc.) software.
Bioinformatics analysis
Bioinformatics services were purchased from MediSapiens Ltd. (Helsinki, Finland). The RNA sequencing data were analyzed using cluster and differentially expressed genes (DEG) analyses. Analyses were performed on variance stabilizing transformation (vst) data using the default settings of the DESeq() function in the R package DESeq2 version 1.28.1 (21). The scale of the vst data was roughly equivalent to the log2 scale. Using a standard threshold, samples were plotted in dendrograms and heatmaps with the top 50, 100, 150, 200, 250, and 500 genes that exhibited the highest standard deviation in expression. The categorization of high or low expression was made based on the median expression of the gene across all samples.
Immunohistochemical assessment of HSP90 protein expression
Immunohistochemistry was performed on tissue microarray slides that were generated as previously described (20). The anti-HSP90 (AC88) (cat. No. ab13492, Abcam, Cambridge, UK, dilution 1:200) mouse monoclonal primary antibody was used. Clone AC88 targets amino acid residues 604–697 of the human HSP90 sequence, covering both HSPC1 and HSPC3 proteins. Heat-induced antigen retrieval was performed using Thermo Scientific™ Lab Vision™ PT Module™ (Cheshire, UK) with Tris/EDTA (pH 9) buffer. The Dako REAL EnVision Detection System (ref. K5007, Agilent, Santa Clara, CA, USA) was used for visualization. Appropriate positive and negative controls were applied.
Stained slides were digitized with a Pannoramic 250 Flash III (3DHISTECH, Budapest, Hungary) microscope slide scanner using a calibrated linear color model, brightfield scanning mode, and a 20× objective. Digitized slides were manually scored in CaseViewer 2.4 (3DHISTECH) slide viewing software by two independent researchers blinded to the patients’ clinical data. Based on the intensity of cytoplasmic staining, a value from 0 to 3 was determined for each sample (0= negative, 1= weak, 2= moderate, and 3= strong) with a threshold of 5% positivity of malignant cells.
Statistical analyses
Statistical analyses involved cross-tabulation, Mann-Whitney U test, Kaplan-Meier method in addition to the log-rank test and Cox regression analysis. During cross-tabulation, Fisher’s exact test was used for dichotomous variables, and the chi-square test was used for other variables. The Mann-Whitney U test was utilized to compare protein expression with continuous variables. The Kaplan-Meier method and log-rank test were used to estimate survival probabilities and compare differences in disease-specific survival (DSS). The association of HSP90 expression with DSS was determined using the time elapsed between the day of the primary tumor surgery and the time of death from PC tumor. A univariable Cox regression model was used to calculate hazard ratios (HRs) and 95% confidence intervals (CIs) for HSP90 status and other factors associated with the risk of disease-specific death. Both Kaplan-Meier and Cox regression analyses were performed using dichotomous variables by which staining results were classified as either negative (score 0–1) or positive (score 2–3). The results were considered statistically significant when the P value was less than 0.05. Two-tailed tests were used. Calculations were performed using IBM SPSS Statistics for Windows, Version 25.0. (IBM Corp., Armonk, NY, USA).
Results
Gene expression analyses
Gene expression analysis included 118 PC tumor samples. Of these, 87 were TCs and 31 ACs. Fifteen tumors presented lymph node or distant metastasis (MET), while the remaining 103 tumors did not (non-MET). In addition, 12 metastasis samples and 10 adjacent lung tissue samples were included in the analysis.
In hierarchical unsupervised clustering analysis of the top 50, 100, 150, 200, 250 and 500 genes that showed the highest standard deviation in expression, nonneoplastic lung tissue samples were segregated together. Similar clustering was also observed between some AC tumors and metastatic tumors, and the majority of metastatic AC tumors segregated into the same main cluster (Figure 1). However, no major differences were observed between the sample categories (AC vs. TC vs. adjacent lung tissue or MET vs. non-MET vs. adjacent lung tissue). Thus, the results were examined using median-based high–low categorized vst data from the top 500 genes yielded from the analyses.
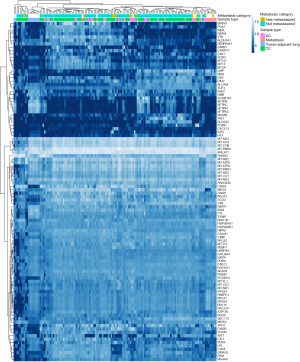
In this analysis, we observed that the median-based high expression of HSP90AB1 differed between MET, non-MET, and adjacent lung tissue samples (87%, 45%, and 0%, respectively, P<0.0001). In addition, the expression of HSP90AA1 was categorized as high in 73% of the METs, while only 46% of non-METs and 30% of adjacent lung tissue samples showed high expression (P=0.069). Therefore, the levels of the proteins HSPC1 and HSPC3, encoded by these two genes, were selected to be tested as prognostic markers, even though the difference in HSP90AA1 expression was not statistically significant.
Immunohistochemical expression of HSP90 and association with clinicopathological variables
Immunohistochemistry was performed for 128 PC tumors, of which 95 were TC tumors and 33 were AC tumors. Seventeen of these primary PC tumors had metastasized and twelve of these metastasis samples were included in the analysis. Tumor samples were scored from 0 to 3 based on their staining intensity (Figure 2). The staining patterns were highly homogeneous in the cytoplasm of the tumor cells. Most of the tumors (71%) showed weak HSP90 expression (Figure 3), and there was no difference in HSP90 expression between TC and AC (P=0.835) or between metastatic and nonmetastatic tumors (P=0.344). Moreover, HSP90 expression was not associated with tumor size, the Ki-67 proliferation index [previously analyzed from the same TMA cores with the QuPath software (20)] or patient sex or age.
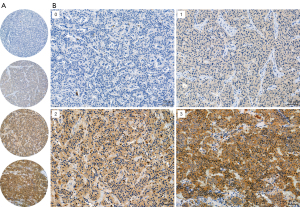
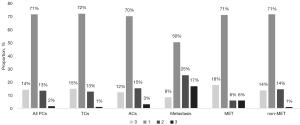
Association of HSP90 expression with patient outcome
Of the 128 patients, six died due to PC. Five of these had an AC tumor with metastatic disease, and one had a TC tumor. The average DSS time was 3.6 years (range, 1.1–8.7 years; median 3.0 years). The average follow-up time for all patients was 12.3 years (range, 1.1–27.8 years; median 10.2 years).
HSP90 expression was associated with shorter DSS in Kaplan-Meier analysis (Figure 4A). In addition, Kaplan-Meier survival analysis was performed between PC subtypes, metastasized and nonmetastasized disease, and high and low Ki-67 labeling index to demonstrate the effect of well-known indicators of poor prognosis on the outcome of patients with PC disease (Figure 4B-4D).
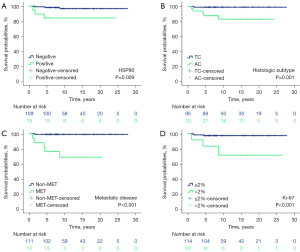
Based on univariable Cox survival regression analysis, high HSP90 expression was associated with an increased risk of shorter survival (HR 6.4, 95% CI: 1.3–31.8, P=0.023; Table 2). In addition, AC subtype, metastatic disease and high Ki-67 labeling index were associated with an increased risk of disease-specific death (all P values <0.05). Other potential risk factors, such as sex, age, or tumor size, were not associated with DSS.
Table 2
Variables | HR | 95% CI | P value |
---|---|---|---|
HSP90 (positive vs. negative) | 6.4 | 1.3–31.8 | 0.023 |
Histologic subtype (AC vs. TC) | 15.2 | 1.8–130.0 | 0.013 |
Metastatic disease (yes vs. no) | 34.9 | 4.1–298.7 | 0.001 |
Ki-67 labeling index† (>2% vs. ≤2%) | 9.9 | 2.0–49.5 | 0.005 |
Sex (female vs. male) | 0.6 | 0.1–2.8 | 0.485 |
Age (years) | 1.0 | 1.0–1.1 | 0.481 |
Tumor size (cm) | 1.1 | 1.0–1.1 | 0.092 |
†, information missing from 2 patients. HR, hazard ratio; CI, confidence interval; HSP90, heat-shock protein 90; AC, atypical carcinoid; TC, typical carcinoid.
Discussion
Using gene expression analysis, we observed that the expression of HSP90A was higher in primary tumors that had metastasized than in nonmetastatic primary tumors or adjacent lung tissue. However, this difference could not be seen at the protein level with immunohistochemistry. Nevertheless, HSP90 immunohistochemical expression was associated with DSS. To our knowledge, this is the first study to evaluate the association between immunohistochemical HSP90 expression and clinical parameters in PC tumor patients.
The clinical course of PC is typically indolent, but the prognosis for individual patients is difficult to predict. The occurrence of PC tumor recurrence or metastasis cannot be predicted with the currently used clinical or molecular markers, e.g., those in the current WHO classification (1). Combined with the knowledge that PC recurrence is possible even after decades, this limitation leads to clinical and radiological surveillance serving as a constant reminder of the disease for the patient, unnecessary exposure to radiation and additional health care costs. Thus, new biomarkers that distinguish cases of PC with favorable outcomes from cases that are more prone to recur or metastasize are needed. Moreover, clinical management of PC tumors relies on surgical resection, and no curative treatment strategy is available for metastatic disease (22). Novel drug candidates are thus urgently needed.
HSP90 is an interesting targetable protein for the management of cancer due to its abundant expression and crucial role in apoptosis, cell cycle control and stabilization of overexpressed oncogenic proteins (7,23). Overexpression of HSP90 has been observed in numerous tumors, including breast, lung, colorectal, ovarian, endometrial, and prostate cancer, and high expression has been linked with poor prognosis (23). Previous studies have also reported HSP90 overexpression in SI-NETs and GEP-NETs (15,18).
Hofving et al. studied the expression of HSP90 in a cohort of 767 SI-NETs from 379 patients with immunohistochemistry (18). They observed that HSP90 expression was higher in SI-NET cells than in the tumor stroma, but they did not find any relationship between HSP90 expression and overall survival. Gamboa et al. studied a large series (n=263) of gastroenteropancreatic NETs and found that immunohistochemical expression of HSP90 was associated with advanced T-stage and early disease recurrence (15).
In our study, HSP90A expression was increased in metastatic PC tumors, but we could not find a difference in the protein level with the monoclonal antibody we used. This may have been due to the altered affinity of the primary antibody to the epitope as a result of translational and posttranslational modifications. HSP90 immunohistochemical expression was associated with DSS. However, there were only a limited number of disease-specific deaths, which may affect the results.
HSP90 inhibitors have demonstrated antiproliferative efficacy in vitro. Andersson et al. noticed a significant inhibition of tumor cell growth in a NET cell line after treatment with HSP90 inhibitors (24). Gilbert et al. and Gloesenkamp et al. studied several NET cell lines and reported anticancer drugs targeting HSP90 to inhibit cell proliferation either alone or in combination with other therapies (16,17). Recently, several studies have shown with neuroendocrine cell lines, xenograft models and patient-derived SI-NET tumor cells that inhibitors of HSP90 can potentiate the tumor cell killing effect of radiotherapy (18,19,25,26). Indeed, several HSP90-targeted drugs have been reported and studied in preclinical and clinical models (23,27). However, none of these HSP90 inhibitors are in clinical use for the treatment of any cancer type due to dose-limited toxicity, drug resistance and poor bioavailability. Currently, the development of HSP90 inhibitors has shifted from paninhibitors to more specific isoform-specific inhibitors that can achieve antitumor effects with reduced toxicity (23).
Our study can be considered representative since it comprised a large number of well-characterized PC tumor patients with up-to-date clinical follow-up and survival data. All tumors were re-evaluated by an expert pathologist according to the latest WHO classification. With our TMA method, adequate amount of tumor tissue was stained using a monoclonal antibody and the staining pattern was highly homogeneous. Scoring was carefully performed by two independent, experienced researchers. Automated image analysis tools were not utilized in this series, but we acknowledge their value in analyzing series of hundreds of tumors. A drawback of our study was the limited number of disease-specific deaths despite a relatively long follow-up. Based on this low number of disease-specific deaths, a reliable multivariable analysis could not be performed, and a larger study is required to obtain more detailed results.
Conclusions
This study confirms that HSP90 plays a role in PC tumors and emphasizes ideas indicated by recent literature that the inhibition of HSP90 may have therapeutic potential in the management of PC tumor patients in the future. Future translational research is required to further improve the understanding of the role of HSP90 in NET biology and clinical treatment strategies.
Acknowledgments
We acknowledge the Helsinki Biobank (Helsinki, Finland) as the origin of the patient material. We thank Nanna Merikoski, University of Helsinki, for technical assistance and the Sequencing Unit of the Institute for Molecular Medicine Finland FIMM Technology Centre, University of Helsinki, supported by Biocenter Finland for sequencing services.
Funding: This study was funded by the Finnish Cancer Foundation (no grant number), the Helsinki University Hospital Research Fund (No. TYH2021204) and the Jane and Aatos Erkko Foundation (No. 4706174).
Footnote
Reporting Checklist: The authors have completed the REMARK reporting checklist. Available at https://tlcr.amegroups.com/article/view/10.21037/tlcr-23-304/rc
Data Sharing Statement: Available at https://tlcr.amegroups.com/article/view/10.21037/tlcr-23-304/dss
Peer Review File: Available at https://tlcr.amegroups.com/article/view/10.21037/tlcr-23-304/prf
Conflicts of Interest: All authors have completed the ICMJE uniform disclosure form (available at https://tlcr.amegroups.com/article/view/10.21037/tlcr-23-304/coif). The authors have no conflicts of interest to declare.
Ethical Statement: The authors are accountable for all aspects of the work in ensuring that questions related to the accuracy or integrity of any part of the work are appropriately investigated and resolved. This study was conducted in accordance with the Declaration of Helsinki (as revise in 2013). The study protocol was approved by the Ethics Committee IV of Helsinki University Hospital (No. HUS/1258/2020) and individual consent for this retrospective analysis was waived.
Open Access Statement: This is an Open Access article distributed in accordance with the Creative Commons Attribution-NonCommercial-NoDerivs 4.0 International License (CC BY-NC-ND 4.0), which permits the non-commercial replication and distribution of the article with the strict proviso that no changes or edits are made and the original work is properly cited (including links to both the formal publication through the relevant DOI and the license). See: https://creativecommons.org/licenses/by-nc-nd/4.0/.
References
- Borczuk AC, Chan JKC, Cooper WA, et al. Tumours of the lung. In: WHO Classification of Thoracic Tumours, 5th ed., edited by the WHO Classification of Tumors Editorial Board. Lyon (France): International Agency for Research on Cancer; 2021:19-191.
- Singh S, Bergsland EK, Card CM, et al. Commonwealth Neuroendocrine Tumour Research Collaboration and the North American Neuroendocrine Tumor Society Guidelines for the Diagnosis and Management of Patients With Lung Neuroendocrine Tumors: An International Collaborative Endorsement and Update of the 2015 European Neuroendocrine Tumor Society Expert Consensus Guidelines. J Thorac Oncol 2020;15:1577-98. [Crossref] [PubMed]
- Cusumano G, Fournel L, Strano S, et al. Surgical Resection for Pulmonary Carcinoid: Long-Term Results of Multicentric Study-The Importance of Pathological N Status, More Than We Thought. Lung 2017;195:789-98. [Crossref] [PubMed]
- Kneuertz PJ, Kamel MK, Stiles BM, et al. Incidence and Prognostic Significance of Carcinoid Lymph Node Metastases. Ann Thorac Surg 2018;106:981-8. [Crossref] [PubMed]
- Ellis RJ. Molecular chaperones: assisting assembly in addition to folding. Trends Biochem Sci 2006;31:395-401. [Crossref] [PubMed]
- Chen B, Piel WH, Gui L, et al. The HSP90 family of genes in the human genome: insights into their divergence and evolution. Genomics 2005;86:627-37. [Crossref] [PubMed]
- Hoter A, El-Sabban ME, Naim HY. The HSP90 Family: Structure, Regulation, Function, and Implications in Health and Disease. Int J Mol Sci 2018;19:2560. [Crossref] [PubMed]
- Vartholomaiou E, Echeverría PC, Picard D. Unusual Suspects in the Twilight Zone Between the Hsp90 Interactome and Carcinogenesis. Adv Cancer Res 2016;129:1-30. [Crossref] [PubMed]
- Vahid S, Thaper D, Zoubeidi A. Chaperoning the Cancer: The Proteostatic Functions of the Heat Shock Proteins in Cancer. Recent Pat Anticancer Drug Discov 2017;12:35-47. [Crossref] [PubMed]
- Hanahan D, Weinberg RA. The hallmarks of cancer. Cell 2000;100:57-70. [Crossref] [PubMed]
- Hanahan D, Weinberg RA. Hallmarks of cancer: the next generation. Cell 2011;144:646-74. [Crossref] [PubMed]
- Dimas DT, Perlepe CD, Sergentanis TN, et al. The Prognostic Significance of Hsp70/Hsp90 Expression in Breast Cancer: A Systematic Review and Meta-analysis. Anticancer Res 2018;38:1551-62. [Crossref] [PubMed]
- Mittal S, Rajala MS. Heat shock proteins as biomarkers of lung cancer. Cancer Biol Ther 2020;21:477-85. [Crossref] [PubMed]
- Paladino L, Vitale AM, Santonocito R, et al. Molecular Chaperones and Thyroid Cancer. Int J Mol Sci 2021;22:4196. [Crossref] [PubMed]
- Gamboa AC, Ethun CG, Postlewait LM, et al. HSP90 expression and early recurrence in gastroenteropancreatic neuroendocrine tumors: Potential for a novel therapeutic target. Surg Oncol 2020;35:460-5. [Crossref] [PubMed]
- Gilbert JA, Adhikari LJ, Lloyd RV, et al. Molecular markers for novel therapies in neuroendocrine (carcinoid) tumors. Endocr Relat Cancer 2010;17:623-36. [Crossref] [PubMed]
- Gloesenkamp C, Nitzsche B, Lim AR, et al. Heat shock protein 90 is a promising target for effective growth inhibition of gastrointestinal neuroendocrine tumors. Int J Oncol 2012;40:1659-67. [Crossref] [PubMed]
- Hofving T, Sandblom V, Arvidsson Y, et al. 177Lu-octreotate therapy for neuroendocrine tumours is enhanced by Hsp90 inhibition. Endocr Relat Cancer 2019;26:437-49. [Crossref] [PubMed]
- Lundsten S, Spiegelberg D, Stenerlöw B, et al. The HSP90 inhibitor onalespib potentiates 177Lu-DOTATATE therapy in neuroendocrine tumor cells. Int J Oncol 2019;55:1287-95. [Crossref] [PubMed]
- Vesterinen T, Mononen S, Salmenkivi K, et al. Clinicopathological indicators of survival among patients with pulmonary carcinoid tumor. Acta Oncol 2018;57:1109-16. [Crossref] [PubMed]
- Love MI, Huber W, Anders S. Moderated estimation of fold change and dispersion for RNA-seq data with DESeq2. Genome Biol 2014;15:550. [Crossref] [PubMed]
- Baudin E, Caplin M, Garcia-Carbonero R, et al. Lung and thymic carcinoids: ESMO Clinical Practice Guidelines for diagnosis, treatment and follow-up☆. Ann Oncol 2021;32:439-51. [Crossref] [PubMed]
- Li ZN, Luo Y. HSP90 inhibitors and cancer: Prospects for use in targeted therapies Oncol Rep 2023;49:6. (Review). [Crossref] [PubMed]
- Andersson E, Arvidsson Y, Swärd C, et al. Expression profiling of small intestinal neuroendocrine tumors identifies subgroups with clinical relevance, prognostic markers and therapeutic targets. Mod Pathol 2016;29:616-29. [Crossref] [PubMed]
- Spiegelberg D, Abramenkovs A, Mortensen ACL, et al. The HSP90 inhibitor Onalespib exerts synergistic anti-cancer effects when combined with radiotherapy: an in vitro and in vivo approach. Sci Rep 2020;10:5923. [Crossref] [PubMed]
- Lundsten S, Spiegelberg D, Raval NR, et al. The radiosensitizer Onalespib increases complete remission in (177)Lu-DOTATATE-treated mice bearing neuroendocrine tumor xenografts. Eur J Nucl Med Mol Imaging 2020;47:980-90. [Crossref] [PubMed]
- Zhang J, Li H, Liu Y, et al. Targeting HSP90 as a Novel Therapy for Cancer: Mechanistic Insights and Translational Relevance. Cells 2022;11:2778. [Crossref] [PubMed]