First-line versus second-line use of pralsetinib in treatment of rearranged during transfection (RET) fusion-positive advanced non-small cell lung cancer: a cost-effectiveness analysis
Highlight box
Key findings
• Compared to chemotherapy, the use of pralsetinib (PRL) in the first-line therapy resulted in an additional 0.07 quality-adjusted life years (QALYs) at a cost of $133,561, with an incremental cost-effectiveness ratio (ICER) of $1,353,849.65 per QALY.
• In the second-line setting, PRL led to an additional 0.09 QALYs at a cost of $92,797, with an ICER of $559,232.70 per QALY.
What is known and what is new?
• The monthly price of the PRL is nearly $22,371.60, which is quite high.
• This study represents the first attempt to evaluate the cost-effectiveness of PRL for treating patients with rearranged during transfection (RET) fusion-positive advanced NSCLC.
What is the implication, and what should change now?
• Neither PRL as first-line nor second-line therapy was found to be cost-effective for patients with RET fusion-positive advanced NSCLC compared to chemotherapy. Reserving PRL until second-line therapy may be a compromise approach to maintaining control over healthcare expenses while still achieving favorable clinical outcomes.
Introduction
Lung cancer has remained the most fatal type of malignant tumor in the US for many years (1). Non-small cell lung cancer (NSCLC) is responsible for up to 85% of all cases of malignancy associated with the lungs (2); unfortunately, it is often diagnosed at an advanced or metastatic stage and with a poor prognosis, with the 5-year survival rate ranging from 22.7% for newly diagnosed NSCLC to only 5.5% for those with distant metastasis (3,4). About 1–2% of NSCLC individuals have rearranged during transfection (RET) fusions, which activate the RET pathway and result in heightened oncogenic signaling that is independent of ligand activation (5-8).
Pralsetinib (PRL) is a highly potent small molecule inhibitor of RET that is used for the treatment of different solid tumors. In September 2020, PRL was approved in the US for the treatment of metastatic RET fusion-positive NSCLC as detected by a Food and Drug Administration (FDA) approved test (7,9). PRL has demonstrated impressive and long-lasting efficacy, making it the novel standard of care for individuals with RET fusion-positive NSCLC, particularly in the multi-cohort, open-label phase I/II registrational trial ARROW (10,11). However, given its high cost of nearly $22,371.60 per month, information regarding the costs and effectiveness of PRL can aid in determining clinical practices and coverage decisions (12,13). An evaluation of its economic efficiency within the high-cost US healthcare system amidst competitive demands for novel services has yet to be conducted.
The aim of the present investigation was to assess the health and economic consequences of 3 unique treatment strategies for advanced NSCLC with RET fusion-positive from the perspective of US payers. These strategies comprise administration of PRL as first-line therapy, reserving it until the second-line following chemotherapy progression, or chemotherapy alone without PRL. We present this article in accordance with the CHEERS reporting checklist (available at https://tlcr.amegroups.com/article/view/10.21037/tlcr-23-469/rc).
Methods
Patients and treatment
Our study involved a hypothetical cohort of individuals with advanced RET fusion-positive NSCLC, mirroring those enrolled in the ARROW study (10). We evaluated 3 distinct treatment strategies: utilizing PRL as a first-line treatment, a second-line treatment, or opting for chemotherapy alone. We included individuals with a median age of 60 years, body weight of 70 kg, body surface area (BSA) of 1.82 m2 (14,15) who were diagnosed with RET fusion-positive NSCLC. The treatment regimen was determined in accordance with the National Comprehensive Cancer Network (NCCN) Clinical Practice Guidelines (16), and detailed treatment plans are presented in Figure 1. The individuals were divided into 3 groups: those who received PRL either before, or after, pemetrexed-based chemotherapy, and those who did not receive PRL. The chemotherapy regimen consisted of cisplatin plus pemetrexed followed by maintenance treatment with pemetrexed. Docetaxel was used in case of disease progression and best supportive care (BSC) was given in the event of further progression.
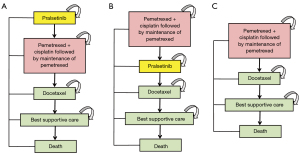
Model structure
This Markov model comprised 3 distinct, mutually independent health states: progression-free survival (PFS), disease progression, and death. Our primary measures included quality-adjusted life years (QALYs), overall expenses, and incremental cost-effectiveness ratios (ICERs). Treatment cost-effectiveness was assessed by utilizing a willingness-to-pay (WTP) threshold of $150,000 per QALY, in accordance with Neumann et al.’s recommendation (17). Our study employed a 3-week cycle length and incorporated a lifetime horizon to evaluate the costs and efficacy of various therapeutic regimens.
Clinical data inputs
The progressed risks of every treatment line were evaluated by analyzing the clinical trial data and the GetData Graph Digitizer software package (version 2.22) was used to extrapolate transition probabilities over a lifetime horizon based on the PFS Kaplan-Meier curves obtained from the ARROW, PARAMOUNT, and IFCT-1103 ULTIMATE trials (10,18,19), and the survival curve of BSC (20). In order to improve the accuracy of our evaluations of average survival rates, the algorithms developed by previously published articles were used when we generated pseudo individual patient data (21,22). The resulting distributions were fitted to the pseudo individual patient data, and the functions of survival analysis included Weibull, Gamma, Log-logistic, Exponential, Gompertz, and Log-normal, among which the optimal fits were determined by the Akaike and Bayesian information criteria. Details regarding the model’s fit are provided in Figure S1 and Table S1, and the model parameters were utilized to evaluate transition probabilities between the primary Markov health statuses. Background death rates for each age group were determined using US life tables (Table S2) (23).
Cost estimates
In our analysis, we considered the utilization of health resources and direct medical expenses such as costs for acquiring and administering drugs, expenses for managing diseases, and any adverse events (AEs) that are linked to the treatment administered (Table S3). We focused solely on severe AEs (grade ≥3) that had an incidence rate higher than 4% in the model.
The drug costs of PRL, pemetrexed, cisplatin, and docetaxel were obtained from the pharmaceuticals public database for March 2023 (24,25). The cost of administration and AEs expenses were extracted from previously published literature (15,26). We made the assumption that patients only received treatment in the initial cycle following the occurrence of an AE, and that the cost of AEs was incurred as a one-time expense. As we assumed that expenses were incurred at the beginning of each cycle, cost adjustments were not made for half of the cycle (27). Physician visit charges were determined based on the codes outlined in the Current Procedure Terminology (28). Our cost calculations adhered to the methodology described by Tumeh et al. (29). The administration costs were tracked for each cycle of chemotherapy, excluding the oral PRL treatment (15), whereas tumor imaging expenses were recorded once every 2 cycles during treatment (14). The costs associated with laboratory testing and physician visits were recorded on a per-cycle basis throughout the treatment period (14,30) (Table S3). We utilized the US Consumer Price Index to adjust for inflation and expressed all costs in terms of 2023 US dollars.
Utility estimates
To evaluate the effectiveness of the treatment, QALYs were utilized as a measure. QALYs are a discounted sum of health status utility values that are specific to American patients. These values range from 0 (which represents mortality) to 1 (which represents complete health) (31). Health utility values were obtained for various states of NSCLC treatment (PFS, disease progression after first-line therapy, second-line therapy, third-line therapy, and death status) by referring to previously published studies on American NSCLC individuals. The utility values for these states were determined to be 0.71, 0.67, 0.59, 0.46, and 0, respectively (32). To assess the reduction in QALYs caused by AEs, we took into account both the incidences and associated disutilities of AEs (33-35). In the first cycle of the model, the overall decline in QALYs resulting from all AEs was considered (36). A list of all parameters related to these utilities is provided in Table S3.
Univariable sensitivity analyses & Monte Carlo simulations
Sensitivity analyses were conducted in order to examine the potential impact of parameters’ uncertainty on results. Clinical parameters in the univariate sensitivity analyses were varied within reasonable bounds based on confidence intervals or assumptions, with a 20% deviation from the baseline value, as demonstrated in the tornado diagram (Figure 2). The upper and lower limits listed in Table S3 were utilized to adjust each parameter. Probability sensitivity analyses (PSA) were conducted through a set of 1,000 Monte Carlo simulations, which incorporated random and simultaneous preset parameter variations, according to specific distribution patterns (Table S3). Due to the unlikely of a price increase for PRL in real-world settings, we sought to investigate the impact of price adjustments on the ICER by solely evaluating the effects of PRL price reductions. The cost-effectiveness of each strategy was analyzed using scatter plots and cost-effectiveness acceptability curves (CEACs), with a WTP threshold of $150,000/QALY (15,37).
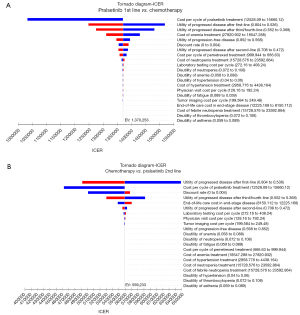
Statistical analysis
We used TreeAge Pro 2022 software (TreeAge, Williamstown, MA, USA) to construct a Markov model that assessed both costs and effectiveness, with a 3% discount rate applied to both utilities and expenses (14). R software (version 4.2.1; The R Foundation for Statistical Computing, Vienna, Austria) was utilized for statistical analyses.
Results
Model validation
The simulated clinical outcomes yielded by our model basically exhibited conformity with the results of corresponding clinical investigations in relation to PFS (Figure S1). The median PFS values obtained from our model did not exhibit significant deviation from the PFS data reported in clinical trials (Table S4).
Base case results
Our study findings revealed that individuals who received PRL as a first-line treatment gained 0.45 QALYs, resulting in an improvement of 0.07 QALYs, whereas those who did not receive PRL until the second-line treatment gained 0.47 QALYs, resulting in an improvement of 0.09 QALYs compared to chemotherapy (Table 1). The cost-effectiveness analysis indicated that the use of first-line PRL incurred an additional cost of $93,275 with an ICER of $1,353,849.65 per QALY, whereas the use of second-line PRL incurred an additional cost of $52,512 with an ICER of $559,232.70 per QALY compared to chemotherapy. Since both treatment strategies yielded QALY values surpassing the predefined WTP threshold of $150,000/QALY, neither alternative was deemed cost-effective relative to chemotherapy (Table 1). Our threshold analyses suggested that reducing the cost of PRL by approximately 71.34% for first-line treatment or 84.49% for second-line treatment would be necessary to make it cost-effective at a WTP threshold of $150,000 per QALY.
Table 1
Arm | Costs, US $ | △Costs, US $ | QALYs | △QALYs | ICER, US $/QALY |
---|---|---|---|---|---|
Chemotherapy | 40,286 | – | 0.38 | – | – |
PRL 1st line | 133,561 | 93,275 | 0.45 | 0.07 | 1,353,849.65 |
PRL 2nd line | 92,797 | 52,512 | 0.47 | 0.09 | 559,232.70 |
ICER, incremental cost-effectiveness ratio; QALYs, quality-adjusted life years; PRL, pralsetinib.
Sensitivity analyses
Tornado diagrams (Figure 2) were created to present the results of univariate sensitivity analyses. The crucial factors impacting the ICERs were congruent across both models, which included the cost of PRL and the utility values for RET fusion-positive NSCLC. Despite notable variations in each parameter’s values, the ICER consistently exceeded $400,000 per QALY.
A scatter plot (Figure 3) was used in the PSA to illustrate the ICERs for 1,000 samples. The 4 quadrants of the plot represented the variations in costs and effectiveness, with a $150,000 per QALY level delineated by a line. Results from all models indicated that their respective points were positioned above this threshold. Furthermore, Figure 4 presents the CEAC for different WTP per QALY values, which revealed that neither first- nor second-line PRL had a probability of being cost-effective when considering a WTP threshold of $150,000 per QALY in competition with chemotherapy. This was consistent across all PSA outcomes.
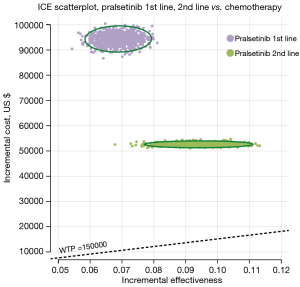
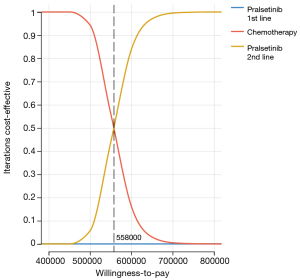
Discussion
The field of oncology has been characterized by dynamic changes, with the introduction of costly interventions such as targeted agents, and immunotherapies. Although technological advancements in cancer treatment have the potential to enhance survival rates (10,38), they may also result in a significant rise in healthcare expenses. This escalation in costs has a detrimental effect on the entire US healthcare system. Moreover, it is imperative to bear in mind that patients are increasingly being burdened with treatment expenses through co-pays, deductibles, and co-insurance, as well as other potential out-of-pocket costs (39,40). The high prices linked with cancer therapy necessitate an evaluation of their value in terms of cost-effectiveness, which is becoming progressively more crucial.
To the best of our knowledge, this is the first investigation into the cost-effectiveness of PRL for patients with RET fusion-positive advanced NSCLC. The IUPAC name for PRL is N-{(1S)-1-[6-(4-fluoropyrazol-1-yl)pyridin-3-yl]ethyl}-1-methoxy-4-{4-methyl-6-[(5-methyl-1H-pyrazol-3-yl)amino]pyrimidin-2-yl}cyclohexane-1-carboxamide. The atomic formula of PRL is C27H32FN9O2, with a molecular weight of 533.61 g/mol. The solubility of PRL declines in hydrophilic media exceeding the pH range of 1.99–7.64 from 0.880 to <0.001 mg/mL. PRL has shown potential as an anticancer agent in vitro using cultured cells and in vivo through animal tumor implantation models carrying oncogenic RET mutations including KIF5B-RET, CCDC6-RET, RET M918T, RET C634W, RET V804E, RET V804L, and RET V804M (41).
Our analysis revealed that although the use of PRL both in the first-line and second-line treatments were unlikely to be cost-effective due to high cost, PRL may be a relatively more cost-effective second-line treatment option compared to first-line treatments due to its lower ICERs and lack of a significant impact on efficacy. However, the PSA analysis revealed that PRL did not meet the cost-effectiveness threshold of $150,000 per QALY for first-line and second-line treatments, with a 0% probability of being cost-effective. Our discovery underscores the significance of the timing of initiation of PRL usage in routine clinical settings, which may have a substantially impact on its cost-effectiveness.
In our model, the cost of PRL was identified as the main influential factor. In recent years, the scientific community has increasingly recognized the significance of financial toxicity. The high price of some targeted therapies has posed a significant barrier to their widespread utilization globally, particularly in regions with limited or inequitable healthcare resources. Patients are confronted with life-changing decisions regarding their health. According to the simulation model, reducing the cost of PRL by approximately 71.34% for first-line treatment or 84.49% for second-line treatment would be necessary to make it cost-effective at a WTP threshold of $150,000 per QALY. Hence, it is crucial to consider the decreased drug costs linked with the integration of novel precision therapies, and to also explore the potential for augmenting the drug’s cost-effectiveness through the enactment of a patient support program and measures aimed at price reduction.
The utility was identified as another significant determinant in our model. The health utility values for patients with disease progression status and PFS status were obtained from published data on health-related quality of life in NSCLC individuals (32). In order to mitigate the potential over-reliance on utility values in impacting outcome stability, we conducted a comprehensive review of existing research to expand the scope of utility values considered in our sensitivity analyses. Our analysis indicated that the impact of utility values on outcomes was minimal and irrespective of whether PRL was utilized as first or second-line treatment; it was deemed not cost-effective based on both upper and lower bounds of utility values.
The scope of our analysis is subject to certain limitations inherent in modeling. First, to estimate the rates of progression, survival data were integrated from diverse clinical trial patients. Nonetheless, this methodology resulted in a certain degree of uncertainty, as none of the trial populations followed the treatment protocols outlined in our model precisely. As a result, it may not fully capture the real-world scenario. Second, in each clinical trial, the total time span for patient follow-up observation for overall survival and PFS varies across the treatments due to the availability of data, potentially influencing curve parameter estimates. Nevertheless, lifetime horizon analyses were performed along with relevant sensitivity analyses to mitigate the potential impact of follow-up time on the findings. Third, our analysis only considered the most frequently occurring AEs. However, based on the findings from our univariate sensitivity analyses, this is unlikely to significantly impact the results. Fourth, in instances where the extent of variability for specific variables is uncertain, a uniform deviation of 20% above and below baseline values was adopted for sensitivity analyses. Despite being a frequently utilized technique in economic evaluations, this range could potentially be imprecise for certain variables. Fifth, as the ARROW trial is a phase I/II trial with a restricted patient pool, it may have introduced some degree of uncertainty regarding the clinical result of the study. To solve this concern, a sensitivity analysis was conducted. However, even with this consideration, the cost-effectiveness analysis suggests that PRL should still not be considered cost-effective. The ongoing AcceleRET Lung (NCT04222972) study is an international, randomized phase III trial that involves open-label evaluation of PRL’s efficacy and safety as a first-line treatment option against standard of care treatment for advanced/metastatic NSCLC cases with RET fusion-positive status (42). Therefore, it is necessary to re-examine the results after the completion of future phase III trials for validation.
Conclusions
Viewed through the perspective of US payers, our study found that for patients with RET fusion-positive NSCLC, neither PRL as first-line nor second-line therapy was found to be cost-effective compared to chemotherapy at WTP thresholds of $150,000 per QALYs. Reserving PRL until second-line therapy may be a compromise approach to maintaining control over healthcare expenses while still achieving favorable clinical outcomes. Therefore, it is essential to take into account both clinical outcomes and economic impact before making a determination regarding the use of PRL.
Acknowledgments
Funding: This study was supported by the China Anti-Cancer Association HER2 Target Chinese Research Fund (No. CETSDSSCORP239018) and the Key Project of Science & Technology Development Fund of Tianjin Education Commission for Higher Education, China (No. 2022ZD064).
Footnote
Reporting Checklist: The authors have completed the CHEERS reporting checklist. Available at https://tlcr.amegroups.com/article/view/10.21037/tlcr-23-469/rc
Peer Review File: Available at https://tlcr.amegroups.com/article/view/10.21037/tlcr-23-469/prf
Conflicts of Interest: All authors have completed the ICMJE uniform disclosure form (available at https://tlcr.amegroups.com/article/view/10.21037/tlcr-23-469/coif). The authors have no conflicts of interest to declare.
Ethical Statement: The authors are accountable for all aspects of the work in ensuring that questions related to the accuracy or integrity of any part of the work are appropriately investigated and resolved.
Open Access Statement: This is an Open Access article distributed in accordance with the Creative Commons Attribution-NonCommercial-NoDerivs 4.0 International License (CC BY-NC-ND 4.0), which permits the non-commercial replication and distribution of the article with the strict proviso that no changes or edits are made and the original work is properly cited (including links to both the formal publication through the relevant DOI and the license). See: https://creativecommons.org/licenses/by-nc-nd/4.0/.
References
- Siegel RL, Miller KD, Fuchs HE, et al. Cancer statistics, 2022. CA Cancer J Clin 2022;72:7-33. [Crossref] [PubMed]
- de Groot PM, Wu CC, Carter BW, et al. The epidemiology of lung cancer. Transl Lung Cancer Res 2018;7:220-33. [Crossref] [PubMed]
- Reade CA, Ganti AK. EGFR targeted therapy in non-small cell lung cancer: potential role of cetuximab. Biologics 2009;3:215-24.
- National-Cancer-Institute. SEER Cancer Statistics Review (CSR) 1975–2015. [updated April 2018; cited December 2022]. Available online: https://seer.cancer.gov/csr/1975_2015/
- Lipson D, Capelletti M, Yelensky R, et al. Identification of new ALK and RET gene fusions from colorectal and lung cancer biopsies. Nat Med 2012;18:382-4. [Crossref] [PubMed]
- Desai A, Menon SP, Dy GK. Alterations in genes other than EGFR/ALK/ROS1 in non-small cell lung cancer: trials and treatment options. Cancer Biol Med 2016;13:77-86. [Crossref] [PubMed]
- Novello S, Califano R, Reinmuth N, et al. RET Fusion-Positive Non-small Cell Lung Cancer: The Evolving Treatment Landscape. Oncologist 2023;28:402-13. [Crossref] [PubMed]
- Rocco D, Sapio L, Della Gravara L, et al. Treatment of Advanced Non-Small Cell Lung Cancer with RET Fusions: Reality and Hopes. Int J Mol Sci 2023;24:2433. [Crossref] [PubMed]
- US Food and Drug Administration. GAVRETO™ (pralsetinib) capsules, for oral use: US prescribing information. 2020. Available online: https://www.blueprintmedicines.com/uspi/GAVRETO.pdf (Accessed March 12 2023).
- Griesinger F, Curigliano G, Thomas M, et al. Safety and efficacy of pralsetinib in RET fusion-positive non-small-cell lung cancer including as first-line therapy: update from the ARROW trial. Ann Oncol 2022;33:1168-78. [Crossref] [PubMed]
- Zhou Q, Zhao J, Chang J, et al. Efficacy and safety of pralsetinib in patients with advanced RET fusion-positive non-small cell lung cancer. Cancer 2023; Epub ahead of print. [Crossref]
- Wickwire EM, Shaya FT, Scharf SM. Health economics of insomnia treatments: The return on investment for a good night’s sleep. Sleep Med Rev 2016;30:72-82. [Crossref] [PubMed]
- Al Khayat MNMT, Armstrong N, Howick J, et al. Pralsetinib for RET Fusion-Positive Advanced Non-small-Cell Lung Cancer: An Evidence Review Group Perspective of a NICE Single Technology Appraisal. Pharmacoeconomics 2023;41:353-61. [Crossref] [PubMed]
- Ding D, Hu H, Li S, et al. Cost-Effectiveness Analysis of Durvalumab Plus Chemotherapy in the First-Line Treatment of Extensive-Stage Small Cell Lung Cancer. J Natl Compr Canc Netw 2021;19:1141-7. [Crossref] [PubMed]
- Lin S, Luo S, Zhong L, et al. Cost-effectiveness of atezolizumab plus chemotherapy for advanced non-small-cell lung cancer. Int J Clin Pharm 2020;42:1175-83. [Crossref] [PubMed]
- National Comprehensive Cancer Network. NCCN Clinical Practice Guidelines in Oncology: Non-Small Cell Lung Cancer. Version 1. 2023. Available from: https://www.nccn.org/guidelines/ (Accessed March 12, 2023)
- Neumann PJ, Cohen JT, Weinstein MC. Updating cost-effectiveness--the curious resilience of the $50,000-per-QALY threshold. N Engl J Med 2014;371:796-7. [Crossref] [PubMed]
- Paz-Ares L, de Marinis F, Dediu M, et al. Maintenance therapy with pemetrexed plus best supportive care versus placebo plus best supportive care after induction therapy with pemetrexed plus cisplatin for advanced non-squamous non-small-cell lung cancer (PARAMOUNT): a double-blind, phase 3, randomised controlled trial. Lancet Oncol 2012;13:247-55. [Crossref] [PubMed]
- Cortot AB, Audigier-Valette C, Molinier O, et al. Weekly paclitaxel plus bevacizumab versus docetaxel as second- or third-line treatment in advanced non-squamous non-small-cell lung cancer: Results of the IFCT-1103 ULTIMATE study. Eur J Cancer 2020;131:27-36. [Crossref] [PubMed]
- Shepherd FA, Dancey J, Ramlau R, et al. Prospective randomized trial of docetaxel versus best supportive care in patients with non-small-cell lung cancer previously treated with platinum-based chemotherapy. J Clin Oncol 2000;18:2095-103. [Crossref] [PubMed]
- Hoyle MW, Henley W. Improved curve fits to summary survival data: application to economic evaluation of health technologies. BMC Med Res Methodol 2011;11:139. [Crossref] [PubMed]
- Wan X, Peng L, Li Y. A review and comparison of methods for recreating individual patient data from published Kaplan-Meier survival curves for economic evaluations: a simulation study. PLoS One 2015;10:e0121353. [Crossref] [PubMed]
- Arias E, Heron M, Xu J. United States Life Tables, 2014. Natl Vital Stat Rep 2017;66:1-64.
- Drug Information Database. Available online: https://www.drugs.com/price-guide/ (Accessed March 12, 2023).
- CMS. The Centers for Medicare & Medicaid Services. Available online: https://www.cms.gov/ (Accessed March 12, 2023).
- Wan X, Luo X, Tan C, et al. First-line atezolizumab in addition to bevacizumab plus chemotherapy for metastatic, nonsquamous non-small cell lung cancer: A United States-based cost-effectiveness analysis. Cancer 2019;125:3526-34. [Crossref] [PubMed]
- Wu B, Chen H, Shen J, et al. Cost-effectiveness of adding rh-endostatin to first-line chemotherapy in patients with advanced non-small-cell lung cancer in China. Clin Ther 2011;33:1446-55. [Crossref] [PubMed]
- Centers for Disease Control and Prevention: 2023 Medicare physician fee schedule. Available online: http://www.cms.gov/Medicare/Medicare (Accessed March 12, 2023).
- Tumeh JW, Moore SG, Shapiro R, et al. Practical approach for using Medicare data to estimate costs for cost-effectiveness analysis. Expert Rev Pharmacoecon Outcomes Res 2005;5:153-62. [Crossref] [PubMed]
- Liu Q, Luo X, Yi L, et al. First-Line Chemo-Immunotherapy for Extensive-Stage Small-Cell Lung Cancer: A United States-Based Cost-Effectiveness Analysis. Front Oncol 2021;11:699781. [Crossref] [PubMed]
- Whitehead SJ, Ali S. Health outcomes in economic evaluation: the QALY and utilities. Br Med Bull 2010;96:5-21. [Crossref] [PubMed]
- Chouaid C, Agulnik J, Goker E, et al. Health-related quality of life and utility in patients with advanced non-small-cell lung cancer: a prospective cross-sectional patient survey in a real-world setting. J Thorac Oncol 2013;8:997-1003. [Crossref] [PubMed]
- Nafees B, Stafford M, Gavriel S, et al. Health state utilities for non small cell lung cancer. Health Qual Life Outcomes 2008;6:84. [Crossref] [PubMed]
- Westwood M, Joore M, Whiting P, et al. Epidermal growth factor receptor tyrosine kinase (EGFR-TK) mutation testing in adults with locally advanced or metastatic non-small cell lung cancer: a systematic review and cost-effectiveness analysis. Health Technol Assess 2014;18:1-166. [Crossref] [PubMed]
- Nafees B, Lloyd AJ, Dewilde S, et al. Health state utilities in non-small cell lung cancer: An international study. Asia Pac J Clin Oncol 2017;13:e195-203. [Crossref] [PubMed]
- Su D, Wu B, Shi L. Cost-effectiveness of Atezolizumab Plus Bevacizumab vs Sorafenib as First-Line Treatment of Unresectable Hepatocellular Carcinoma. JAMA Netw Open 2021;4:e210037. [Crossref] [PubMed]
- Rabin R, de Charro F. EQ-5D: a measure of health status from the EuroQol Group. Ann Med 2001;33:337-43. [Crossref] [PubMed]
- Jeon Y, Jung HA, Park S, et al. Expanded Access Program Pralsetinib in Advanced Non-Small Cell Lung Cancer with Rearranged during Transfection (RET) Gene Rearrangement. Cancer Res Treat 2023; Epub ahead of print. [Crossref]
- Zafar SY, Peppercorn JM, Schrag D, et al. The financial toxicity of cancer treatment: a pilot study assessing out-of-pocket expenses and the insured cancer patient’s experience. Oncologist 2013;18:381-90. [Crossref] [PubMed]
- Zafar SY. Financial Toxicity of Cancer Care: It’s Time to Intervene. J Natl Cancer Inst 2016;108:djv370. [Crossref] [PubMed]
- Ali F, Neha K, Chauhan G. Pralsetinib: chemical and therapeutic development with FDA authorization for the management of RET fusion-positive non-small-cell lung cancers. Arch Pharm Res 2022;45:309-27. [Crossref] [PubMed]
- Servetto A, Esposito D, Ferrara R, et al. RET rearrangements in non-small cell lung cancer: Evolving treatment landscape and future challenges. Biochim Biophys Acta Rev Cancer 2022;1877:188810. [Crossref] [PubMed]