PFKP confers chemoresistance by upregulating ABCC2 transporter in non-small cell lung cancer
Highlight box
Key findings
• Phosphofructokinase, platelet (PFKP) overexpression correlated with poorer survival rates in non-small cell lung cancer (NSCLC) patients receiving platinum-based chemotherapy, as it promoted chemoresistance through increased ABCC2 expression, while inhibition of PFKP enhanced sensitivity to cisplatin in both in vitro and in vivo settings.
What is known and what is new?
• PFKP is highly expressed in NSCLC and associated with poor prognosis.
• This study unveils the mechanism wherein PFKP regulates chemoresistance by upregulating ABCC2 in NSCLC.
What is the implication, and what should change now?
• Targeting PFKP as a potential personalized therapy and developing strategies to inhibit PFKP and ABCC2 could improve treatment outcomes in NSCLC patients with chemoresistance.
Introduction
Lung cancer has the second-highest incidence and the first-highest mortality rate among all cancers (1). Lung cancer can be classified into two main pathological types: non-small cell lung cancer (NSCLC) and small-cell lung cancer. NSCLC is the predominant type, accounting for approximately 80–85% of all cases (2). Patients with early-stage NSCLC have achieved a good prognosis due to advancements in surgical techniques in recent years (3,4). Despite the different treatment options available for advanced NSCLC patients, such as concurrent chemoradiation, immune-chemotherapy combination, and targeted therapy (5), the 5-year relative survival rate for regional advanced cases is 33%, while it is only 6% for distant metastasis (2). Chemotherapy, including platinum-based agents, is still an important treatment option for patients with advanced NSCLC (6). Chemoresistance is becoming more common in clinical practice, which has dramatically affected the prognosis of such patients and posed a barrier to treatment (7). Therefore, it will be very important for elucidating the molecular mechanisms underlying chemoresistance in NSCLC and identifying crucial therapeutic targets for NSCLC chemoresistance.
Phosphofructokinase-1 (PFK-1), an allosteric enzyme that regulates glucose oxidation in cellular respiration, plays a vital role in glycolysis. The cancer-specific isoform of PFK-1, phosphofructokinase, platelet (PFKP), is highly expressed in various tumors and often promotes tumorigenesis and metastasis (8,9). PFKP also interacts with cyclic adenosine monophosphate (AMP)-activated protein kinase to maintain cellular energy and redox homeostasis, allowing cells to survive under oxidative stress conditions (10). Previous study verified that PFKP is highly expressed in lung cancer by regulating the process of glycolysis, which promotes tumor cell proliferation and is connected to a poorer prognosis of lung cancer patients (8). However, the specific molecular mechanism underlying the potential involvement of PFKP in chemoresistance in NSCLC, as well as its regulatory mechanisms is still unclear. Therefore, a comprehensive understanding of these questions is necessary to provide insights into the developing effective therapies for NSCLC treatment.
The ATP-binding cassette (ABC) superfamily members play an essential role in tumor multi-drug resistance (MDR) in various types of cancer, including lung cancer (11-13). As a member of the ABC subfamily, ABCC2 transports chemotherapeutic agents, such as erythromycin, methotrexate, and etoposide, which in turn affect the drug response of patients with advanced NSCLC (14). ABCC2 enhances the drug resistance of tumor cells by pumping intracellular drugs (e.g., CDDP and vincristine) out of the cells (15). Accordingly, the expression of ABCC2 has a significant impact on the prognosis of patients with NSCLC (16). NF-κB, an inducible transcription factor, regulates ABCC2 expression in lung cancer cells, thereby attenuating their drug resistance (17). Thus, exploring the regulatory mechanism of ABC family proteins may help to solve the challenges arising from to chemoresistance.
In this study, we provide evidence to demonstrate a novel role of PFKP in mediating chemoresistance in NSCLC. Mechanistically, PFKP decreases the content of CDDP by upregulating ABCC2in NSCLC cells, thereby changing their sensitivity to CDDP. Furthermore, PFKP activates the activity of NF-κB through promoting the phosphorylation IκBα, thereby enhancing the expression of ABCC2 and drug resistance of NSCLC cells. These findings may provide a new framework for understanding the mechanism underlying chemoresistance in NSCLC. We present this article in accordance with the ARRIVE and MDAR reporting checklists (available at https://tlcr.amegroups.com/article/view/10.21037/tlcr-23-567/rc).
Methods
Reagents and antibodies
Cisplatin (purity =99.70%), paclitaxel (purity =99.96%) and DMSO were purchased from MCE (Shanghai, China). Advanced DMEM/F-12 (Cat 12634028), HEPES (Cat #15630080), GlutaMAXTM (Cat 35050061), penicillin-streptomycin (Cat 15140122), Tumor Tissue Digestion Solution (Cat 9522), fetal bovine serum (FBS) (Cat 10100147) and RPMI-1640 (Cat 11875093) were from Thermo Fisher Scientific (Waltham, MA, USA). MatrigengeL Matrix (Cat 0827555) was from ABW (Shanghai, China). γ-H2AX (Ser139) (Cat 2577), p-IκBα (Cat 5209), IκBα (Cat 4814), p-p65 (Cat 3033) and p65 (Cat 8242T) were brought from Cell Signaling Technology (Beverly, MA, USA). ABCC2 (Cat ab172630), and Survivin (Cat ab76424) were from Abcam (Shanghai, China). PFKP (Cat 13389-1-AP), GAPDH (Cat 60004-1-Ig) and α-Tubulin (Cat 11224-1-AP) were from Proteintech (Chicago, IL, USA).
Clinical specimen selection
The human samples were taken from lung cancer patients who had undergone radical surgery at Taizhou Hospital, Zhejiang Province from December 2021 to March 2022. Informed consent was obtained prior to tissue collection from each patient. The patients did not receive chemotherapy or radiotherapy prior to the surgery. Patient data including clinicopathological information and follow-up records were collected (Table S1). Cells were isolated from the tumor tissue of seven NSCLC surgery patients for the organoid assays and proteins were extracted for further Western blot analyses. The study has obtained approval from the Ethics Committee of Taizhou Hospital of Zhejiang Province affiliated to Wenzhou Medical University (No. 2021-07-138-01). The study was conducted in accordance with the Declaration of Helsinki (as revised in 2013).
Construction of lung cancer organoids
The culture medium comprised Advanced DMEM/F-12, HEPES 10 mM, GlutaMAXTM 2 mM, penicillin-streptomycin and 5% FBS. First, the tissue sample was imaged, weighed, and the volume was measured. The sample was washed with PBS 2–3 times until clear, and the tissue was cut into 1–3 mm3 pieces using scissors. The tissue was then resuspended in 3 mL digestion solution and transferred to a centrifuge tube. The tube was inverted on a single-cell suspension preparation system, with a temperature set at 38.5 ℃ and speed set at 100 ×g, and it was rotated clockwise and counterclockwise for 5 minutes. After centrifugation, the mixture was passed through a 70 µm cell filter, and the remaining sample was washed with 5 mL of basic culture medium. The collected cells were then counted using a cell counter and resuspended in a complete culture medium with the addition of matrix gel. Cells (1×105/10 µL) were added to each well of a 24-well plate, which was then inverted and incubated in a cell culture incubator for 30 minutes to allow the matrix gel to solidify. Afterwards, 1.5 mL of culture medium was added to each well.
In vitro drug sensitivity screening
After 2 days of inoculation of lung cancer organoids, the spheroid state was observed, photographed, and ATP activity was measured in 2–3 randomly selected wells using the ATP-TCA kit (Thermo Cat# A22066). The following concentrations of cisplatin and paclitaxel solutions 50, 25, 12.50, 6.25, 3.13, 1.56, and 0.78 µM were configured using culture medium. After 3 days of inoculation, the organoids were administered by aspirating the medium and supplementing each well with 130 µL of the appropriate solution. The ATP activity was measured after 24 hours using the kit and the IC50 value was calculated.
Cell lines and cell culture
Human NSCLC cell lines A549 and A549/CDDP were obtained from Shanghai Cell Bank (Shanghai, China). The cells were cultured routinely in RPMI-1640 medium supplemented with 10% FBS and 1% penicillin-streptomycin, and maintained at 37 ℃ in a 5% CO2 incubator. The concentration of CDDP in the medium was maintained at 5 µg/mL for the A549/CDDP cells.
Colony formation assay
A549-vector, A549-PFKP, A549/CDDP sh-control, A549/CDDP sh#1, A549/CDDP sh#2, and A549-PFKP ABCC2-siRNA cells [1,000] were inoculated into 35 mm cell culture dishes using complete culture medium incubated for 7 days. After fixation using 4% paraformaldehyde (PFA) and staining with a 0.1% crystalline violet solution, the cells were photographed and counted.
Preparation of plasmids and viral constructs
PFKP-coding sequences were cloned into the pCDH vector by polymerase chain reaction (PCR) amplification and insertion, using the cytomegalovirus (CMV) promoter and a 3Flag tag, as well as an EF1 promoter, CopGFP reporter gene, T2A element, and Puromycin resistance gene. ShRNA oligonucleotide sequences specific to human PFKP were cloned into PLKO-U6-EGFP-P2A-PURO vector to generate PFKP-shRNA. PFKP plasmids and shRNA were transfected using Lipofectamine 3000 reagent (Invitrogen, Carlsbad, CA, USA), following the manufacturer’s protocol. After infection, stable cell lines were selected for 10 days using 0.5 µg/mL puromycin to obtain the desired gene expression. The pBabe-Puro-IκBα-mut (plasmid 15291) expressing mutant IκBα was from Addgene (Cambridge, MA, USA). IkBalpha-mutant A549 cells was obtained by transfection of IκBα-mut plasmids was performed using the Lipofectamine 3000 reagent (Invitrogen) according to the manufacturer’s instruction.
Western blot analysis
To extract proteins, cells and tissues were lysed with RIPA buffer containing PMSF and phosphatase inhibitors on ice, followed by centrifugation at 12,000 rpm and 4 ℃ for 15 minutes. The protein concentration was measured using the BCA Protein Assay Kit (Beyoncé, Shanghai, China) following the manufacturer’s instructions; 40 µg of protein samples were separated on 8–12% SDS-PAGE and then transferred onto a polyvinylidene fluoride (PVDF) membrane (0.22 µm pore size, Millipore, CA, USA). After blocking with 5% skimmed milk for 2 hours, the membranes were incubated with PFKP (1:1,000), ABCC2 (1:1,000), IκBα (1:1,000), p-IκBα (1:1,000), p-p65 (1:1,000), p65 (1:1,000), α-Tubulin (1:5,000), and GAPDH (1:5,000) antibodies at 4 ℃ overnight. Then, after washing, the appropriate secondary antibodies were used based on the primary antibodies. Horseradish peroxidase (HRP)-conjugated rabbit anti-goat IgG (1:3,000) and HRP-conjugated goat anti-mouse IgG (1:5,000) were applied and incubated for 1 hour at room temperature. After washing with Tris Buffered Brine and Tween20 (TBST), a chemiluminescent reagent (Millipore, Burlington, USA) was used to observe the bands. ImageQuant LAS 500 (GE Health Care, CA, USA) was used to visualize the signal, and ImageJ software (Bio-Rad, CA, USA) was utilized to quantify and analyze the bands.
Quantitative real-time PCR
The Trizol method was used to isolate total RNA from the cultured cells. The PrimeScript ™ RT reagent Kit (Takara, Japan, No. RR047A) and PCR equipment (Bio-Rad, USA) were used for cDNA synthesis. The cDNA was amplified using an ABI 7500 real-time PCR instrument ( Applied, CA, USA) and TB Green® Premix Ex Taq™ II (Takara, Japan, No. RR820L). Normalized cycling threshold (Ct) values using the housekeeping gene GAPDH were employed to evaluate the expression of each group of target genes with the 2−ΔΔCt method.
The primer sequences used are as follows:
PFKP, 5'-GACCTTCGTTCTGGAGGTGAT-3' (forward) and 5'-CACGGTTCTCCGAGAGTTTG-3' (reverse); GAPDH 5'-GGACCTGACCTGCCGTCTAG-3' (forward) and 5'-CACGGTTCTCCGAGAGTTTG-3' (reverse); ABCC2, 5'-CCCTGCTGTTCGATATACCAATC (forward) and 5'-TCGAGAGAATCCAGAATAGGGAC-3' (reverse).
siRNA transfection
Prior to siRNA transfection, A549-PFKP stable cell lines, which had been pre-screened with puromycin, were treated with trypsin and incubated overnight to reach a fusion rate of 60–70%. ABCC2-siRNA was then transfected into the A549-PFKP cells using Lipofectamine 3000 (Thermo Fisher, L3000015).
Animal experiments
Female BALB/c nude mice (5–6 weeks old) were obtained from Charles River (Beijing, China). Mice (6 mice per group) were implanted subcutaneously with 1×106 A549 (vector and PFKP high expression) and A549/CDDP (sh-control and shRNA#1) cells mixed with Matrix (purchased from ABW). When the maximum tumor volume reached 100 mm3, CDDP (2 mg/kg, CDDP/animal weight) was injected intraperitoneally every three days. On day 42, the tumors in mice were removed, measured for weight, and photographed after sacrifice. We formulated a protocol prior to the commencement of the study and all operations involving experimental animals were approved by the Animal Ethics Committee of Taizhou Hospital, Zhejiang Province (No. tzy-2022001), and were carried out in compliance with Taizhou Hospital, Zhejiang Province institutional guidelines for the care and use of animals.
Immunohistochemistry (IHC) and immunofluorescence analysis
The paraffin-embedded tissue sections were deparaffinized, rehydrated, and subjected to antigen retrieval using sodium citrate buffer (pH =6.0). Immunohistochemical staining was conducted using the streptavidin-peroxidase (SP) method as per the instructions provided in the kit. Blocking was performed using 10% FBS. The appropriate primary antibodies were added individually in drops according to the dilution ratios in the antibody instructions and being kept at 4 ℃ overnight, appropriate secondary antibodies were applied. The following concentrations were used for primary antibodies: PFKP (1:800), survivin (1:800), ABCC2 (1:500), p65 (1:2,000), and 1:500 for secondary antibodies. Prepared Diaminobenzidine (DAB) chromogenic solution working solution was added dropwise to the tissue sections and the staining was developed for 10 min and observed under the microscope. ImageJ was used to calculate the area of the stained region.
The experimental procedure involved treating the cells with 4% PFA for 15 minutes, followed by blocking with 5% FBS for 1 hour at 37 ℃. Later, primary antibody γ-H2AX (1:400) was added and incubated overnight at 4 ℃. Further, Alexa Fluor 488 or Alexa Fluor 594 was used as secondary antibody for 1 hour at room temperature. DAPI was then used for a final 5-minute incubation at 37 ℃. A Nikon microscope (Nikon, Tokyo, Japan) was used to randomly capture images of a field of view of cells, which were then stored for computational analysis of fluorescence intensity using ImageJ.
TUNEL (TdT-mediated dUTP nick end labeling)
Cells (5×104) were inoculated and cultured overnight on cover slip and were subject to a TUNEL assay, and an in-situ cell death detection kit was used for this purpose. Images of the samples were taken through AxioVision Rel.4.6 computerized image analysis system (Carl Zeiss, Oberkochen, Germany).
Intracellular cisplatin and DNA-bound cisplatin analysis
In order to detect intracellular CDDP content, we collect the indicated cells in the log phase of growth were suspended in medium at a density of 1×106/mL and incubated for 4 h in the presence of CDDP at 37 ℃ in a humidified atmosphere containing 5% CO2. The intracellular platinum level was determined by atomic absorption spectrophotometry. At the same time, the total cell protein and DNA levels was measured and the CDDP content in the total protein and DNA levels was analyzed.
Dual-luciferase analysis
The activity of NF-κB signaling was assessed via a dual-luciferase reporter gene assay kit (Promega, USA). The transfected cells were lysed and the supernatant was collected. Data were read using BioTek Synergy (Bio-Rad, USA).
Bioinformatics analysis
Data from microarray experiments were obtained through The Cancer Genome Atlas (TCGA) (http://www.tcga.org/) and Gene Expression Omnibus (GEO) databases (https://www.ebi.ac.uk/arrayexpress/).
The Venn diagram was created using http://bioinformatics.psb.ugent.be/webtools/Venn/. Gene Set Enrichment Analysis (GSEA) was performed with GSEA 2.0.9 from http://www.broadinstitute.org/gsea/.
Statistical analysis
The results are presented as the mean ± standard deviation of independent experiments. The statistical analyses and graphing were done using GraphPad Prism 8.0. For intergroup comparisons, we used analysis of variance (ANOVA) and a Student’s t-test. Results with a P value less than 0.05 were considered statistically significant. All statistical data were obtained from at least three independent biological replicates, and each experiment included at least two technical replicates.
Results
High PFKP expression correlates with poorer prognosis and chemoresistance in NSCLC
To explore the role of PFKP in NSCLC, we analyzed the relationship between the expression levels of PFKP in lung tumors and survival of lung cancer patients using the online Kaplan-Meier survival analysis tool available at (http://kmplot.com/analysis/index.php?p=service&cancer=lung). The results showed that among 478 patients with NSCLC, those with high PFKP expression had lower overall survival (OS) and disease-free survival (DFS) rates compared to those with baseline expression (Figure 1A). For lung cancer patients receiving chemotherapy across CaArray dataset, high expression of PFKP was associated with poorer OS and post-progression survival (PPS) compared to those with low expression. Similarly, in the GSE14814 database, high PFKP expression was significantly correlated with poorer OS. The combined analysis of more than 17 cohorts’ data also revealed a significant correlation between high PFKP expression and poorer OS and first-progression survival (FPS) (Figure 1B).

Cisplatin (CDDP) was used as a first-line chemotherapy for NSCLC. The GSEA analysis revealed that PFKP overexpression was closely associated with CDDP chemotherapy markers not found in methotrexate and fluorouracil, indicating that PFKP expression may contribute to CDDP resistance in NSCLC (Figure 1C).
We next established organoids using specimens from clinical NSCLC patients to investigate the role of PFKP in chemoresistance (Figure S1). The results showed that the expression of PFKP was significantly elevated in chemotherapy-resistant organoids compared with the chemotherapy-sensitive samples (Figure 1D). Correspondingly, the immunohistochemical results yielded the same results (Figure 1E,1F). These results suggest that PFKP expression could be closely associated with chemotherapy resistance.
PFKP confers chemoresistance in NSCLC cells
To further investigate the direct role PFKP plays in chemoresistance, we established a cisplatin-resistant A549 cell line (A549/CDDP). In the cytotoxicity assay, the cisplatin-resistant A549 cells better resisted the toxicity of CDDP (CDDP concentration of 5 µg/mL), P<0.05 (Figure 2A). Also, PFKP expression in A549/CDDP cells was higher than that in A549 cells at both mRNA and protein levels (Figure 2B,2C).
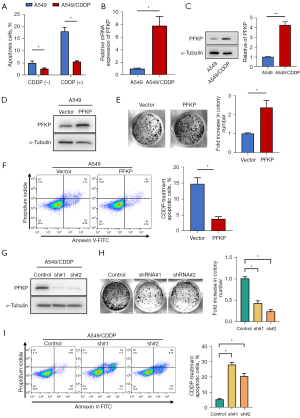
Next, we used lentivirus to knock down PFKP in A549/CDDP cells or overexpress PFKP in A549 cells (Figure 2D). The results demonstrated that overexpression of PFKP increased cell surviving fractions (Figure 2E) and decreased CDDP-induced cell apoptosis (Figure 2F) in A549 cells. In contrast, knockdown of PFKP inhibited the cell proliferation (Figure 2G,2H) and increased CDDP-induced cell apoptosis (Figure 2I) in A549/CDDP cells. These findings suggest that high expression of PFKP protects chemo-resistant cells from the pro-apoptotic effect of CDDP.
PFKP promotes chemoresistance of NSCLC tumors in vivo
To determine whether the expression profile of PFKP influences the killing effect of CDDP on tumor cells in vivo, we injected A549 and A549/CDDP cells subcutaneously into BALB/c nude mice to establish xenograft models. When the average volume of tumors had reached approximately 100 mm3, CDDP (2 mg/kg) was injected intraperitoneally every three days up to 42 days when mice were sacrificed, and the subcutaneous tumors were excised (Figure 3A). The tumor weights of PFKP-overexpressing A549 tumors were obviously larger than those in the vector control vector-expressing tumors, while the tumor weights of PFKP-knockdown A549/CDDP tumors were smaller than the control group (Figure 3B). Survivin was highly expressed in tumor tissues and played important role in preventing CDDP-induced cell death (18,19). Immunohistochemical staining of xenograft sections showed that the expression of survivin was increased in the PFKP-overexpressing tumors compared to the control group (Figure 3C). In contrast, knockdown of PFKP in A549/CDDP tumors resulted in a decrease in the expression of survivin (Figure 3C). The above results suggest that PFKP significantly enhances drug resistance in NSCLC tumor cells in vivo.
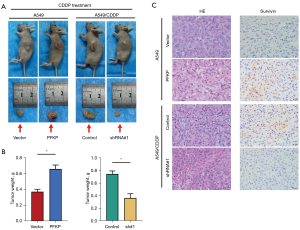
PFKP promotes cellular drug resistance through upregulating the expression of ABCC2
Next, we tested whether PFKP promotes cellular drug resistance through affecting intracellular CDDP content. Following treatment with 5 µg/mL CDDP, the intracellular amount of CDDP in PFKP-overexpressing cells was notably lower than that in the control A549 cells (Figure 4A). Correspondingly, the knockdown of PFKP resulted in a significant increase in intracellular CDDP (Figure 4A). Also, we observed a similar trend in the amount of DNA-bound CDDP (Figure 4B). To further investigate the effect of PFKP on CDDP-induced DNA double-strand breaks (DSBs), immunocytochemical analyses were performed with the γ-H2AX antibody (20). We observed that the level of γ-H2AX was significantly decreased in the PFKP-overexpressing cells and increased in the PFKP-knockdown cells (Figure 4C). These results suggest that PFKP expression may influence the therapeutic effect of CDDP by decreasing intracellular CDDP levels and CDDP-mediated DNA-damage.
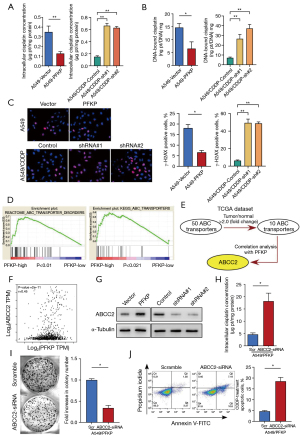
To investigate how PFKP affects intracellular CDDP content, we performed a classical enrichment analysis using GSEA analysis to investigate the correlation between PFKP expression and the ABC drug exporters (Figure 4D). Approximately 50+ members of the ABC family are known to exist (21), and further analysis using the TCGA database identified 10 ABC family proteins that were significantly elevated in lung cancer. Among these ten transporter proteins, only ABCC2 was closely associated with PFKP (Figure 4E,4F, Figure S2). The results demonstrated that overexpression of PFKP increased the expression of ABCC2, whereas knockdown of PFKP decreased the expression of ABCC2 (Figure 4G). As expected, knockdown of ABCC2 increased the CDDP content in the A549/PFKP cells (Figure 4H). The knockdown of ABCC2 reduced the capacity of monoclonal formation (Figure 4I) and enhanced the cell-killing effect (Figure 4J) of CDDP in PFKP-overexpressing A549 cells. The above data suggest that ABCC2 upregulation is required for PFKP-mediated cellular drug resistance.
PFKP upregulates ABCC2 through activating NF-κB
Previous study demonstrated that NF-κB transcription factor acts as a positive regulator of ABCC2 transcription (22). Thus, we wondered whether PFKP may upregulating ABCC2 by activating the NF-κB pathway. The GSEA analysis revealed that PFKP was closely associated with NF-κB signaling (Figure 5A). To explore whether PFKP is essential for activating NF-κB signaling, we compared the luciferase activity in cells with each PFKP expression profile. The overexpression of PFKP in A549 cells induced a significant increase in NF-κB activity, while PFKP knockdown A549/CDDP cells showed a significant decrease in NF-κB activity (Figure 5B). To further investigate how PFKP regulates ABCC2 expression through NF-κB pathway, we examined the phosphorylation of IκBα, a negative regulator of NF-κB, and the expression of NF-κB subunit p65 in the nucleus. Western blot results showed that p-IκBα and nuclear p65 expression were elevated in PFKP-overexpressing A549 cells, and decreased in PFKP-knockdown A549-CDDP cells (Figure 5C).
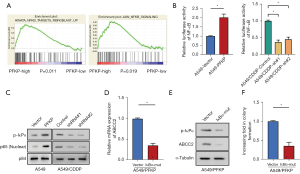
To elucidate whether an increase in IκBα leads to a rise in ABCC2 protein levels, we introduced mutant form of IκBα (IκBα-mut) in A549 cells and then examined the levels of ABCC2 mRNA in IκBα-mut A549 and control cells. Compared with control A549 cells, the IκBα-mut A549cells showed a significant decrease in both mRNA and protein levels of ABCC2 (Figure 5D,5E). The IκBα-mut A549 cells also showed reduced monoclonal formation compared to control A549 cells (Figure 5F). The above results suggest that PFKP upregulate ABCC2 expression probably through activating NF-κB pathway.
Clinical relevance of PFKP and ABCC2 in NSCLC
The immunohistochemical results of tumor sections from NSCLC patients showed increased ABCC2 expression and p65 nuclear localization in the tumor tissues with high PFKP expression compared to those with low PFKP expression (Figure 6A). To investigate whether ABCC2 and the p65 (also called RELA) subunit of NF-κB are relevant to patients’ survival prognosis, we analyzed the relationship between ABCC2 or p65 expression and survival in lung cancer patients using the online Kaplan-Meier survival analysis (www.kmplot.com). Among 1,925 NSCLC patients, OS and first-progression (FP) of the patients with high ABCC2 expression was lower than those with low ABCC2 expression (P=0.014) (Figure 6B). Similarly, OS and FP of the patients with high p65 expression was lower than those with low p65 expression (P=0.0075) (Figure 6B). Collectively, high expression of PFKP could be associated with activation of NF-κB-dependent ABCC2 upregulation in NSCLC.
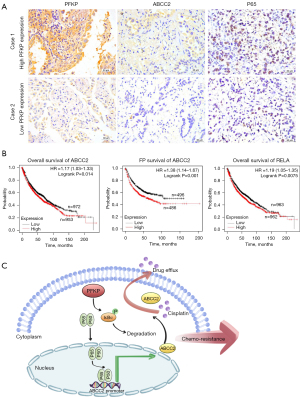
Discussion
In this study, we unveiled that PFKP caused an increase in ABCC2 expression, which, thanks to the effect of the efflux pump, enabled NSCLC cells to acquire the ability to resist chemotherapeutic drugs This was accompanied by PFKP-induced phosphorylation of IκBα to activate the NF-κB signaling pathway, which may upregulate the expression of ABCC2 (Figure 6C).
PFKP is one of the three isomers of phosphofructokinase 1 (PFK1), the other two are phosphofructokinase liver type (PFKL) and phosphofructokinase muscle type (PFKM) (23). PFKP was previously reported to be upregulated in most tumor types, including breast cancer, hepatoma, and kidney cancers, and be strongly associated with prognosis. PFKP expression was higher in oral squamous cell carcinoma tissues than in paraneoplastic non-cancerous tissues and was linked with tumor cell differentiation and metastasis (24). In contrast to normal brain tissue, PFKP is upregulated in glioblastoma and functions as a pro-oncogene (25). Interestingly, recent studies have shown that PFKP is a nuclear shuttle protein with active nuclear transport and nuclear localization sequences (NLSs) (26). Although PFKP is a critical enzyme in glycolysis, PFKP may promote tumor cell proliferation through its non-glycolytic-related function by regulating PI3K and YAP/TAZ activity (27). PFKP also promotes long-chain fatty acid oxidation in the context of tumor cell glucose starvation, maintains cellular homeostasis of redox and energy metabolism, and enhances tumor cell resistance to oxidative stress (10). Overexpression of PFKP promoted β-catenin (S552) phosphorylation and epithelial-to-mesenchymal transition in NSCLC cells under hypoxia, thereby enhancing cell survival and proliferation (28). These findings suggest that PFKP may not only function as a key enzyme in glycolysis but also contributes significantly to the survival of tumor cells through non-enzyme functions. Our organoid experiments have demonstrated that NSCLC samples with high PFKP expression exhibit variable degrees of resistance to conventional chemotherapeutic agents such as CDDP and paclitaxel. Additionally, our observations of lower intracellular drug concentrations of CDDP induced by ABCC2 upregulation induced by PFKP further support the potential significance of PFKP in NSCLC progression and chemoresistance.
In previous studies, the ABC superfamily has been shown to play an essential role in the development of MDR in tumors, with the primary mechanism of action being the efflux pump, which reduces intracellular drug concentrations and protects them from the effects of drugs, thereby avoiding cell death (11,12,15). This phenomenon was also observed in our experiments, which showed that PFKP induced a significant decrease in intracellular cisplatin drug concentration in NSCLC cells, and this process was reversed by knockdown of ABCC2. Thus, the altered intracellular drug concentration may be due to the pumping activity of ABCC2, which to some extent explains the tolerance to chemotherapeutic drugs in NSCLC cells with high PFKP expression. These results provide significant insights into the underlying molecular mechanisms involved in PFKP-mediated chemoresistance.
NF-κB, a well-known transcription factor, has been shown to have a regulatory role in tumor MDR (29). IκBα is a critical negative regulator of the NF-κB signaling pathway. When IκBα is phosphorylated, IκBα is quickly degraded by proteasome, thus promoting the activation of p65/p50, which enters the nucleus to regulates the expression of related genes, thus exerting a corresponding effect (30). Existing studies demonstrate that transcription factor Nrf2 binds to the promoter region of ABCC2 and thereby regulates its expression (31). Further evidence reveals that NF-κB activates Nrf2 expression by binding to a specific promoter region (32,33). Accordingly, inhibition of NF-κB downregulates Nrf2 and therefore triggering the downregulation of ABCC2 expression (22). Our data further suggest that nuclear activation of p65 is observed in tumor tissues with high PFKP expression. Additionally, the luciferase experiments show that PFKP potentially activates NF-κB via phosphorylating IκBα. These findings indicate that NF-κB may upregulate ABCC2 by activating NF-κB pathway in NSCLC, which could have important implications for the development of targeted therapeutic strategies.
In conclusion, we elucidated the underlying mechanism by which PFKP mediates chemoresistance in tumor tissues. Specifically, PFKP enhanced the expression of ABCC2 through increasing the activity of NF-κB. The findings of this study enhance our comprehension of the molecular mechanisms associated with chemoresistance in NSCLC and may have significant implications for the development of more efficacious therapeutic interventions for this pathology.
Conclusions
In conclusion, our study reveals a potential mechanism underlying chemoresistance in NSCLC. We found that overexpression of PFKP is associated with poor prognosis and reduced sensitivity to platinum-based chemotherapy in NSCLC patients. Through in vitro and in vivo experiments, we demonstrated that PFKP regulates chemosensitivity by upregulating ABCC2 via NF-κB activation. These findings highlight PFKP as a potential personalized therapeutic target for overcoming chemoresistance in NSCLC. Further investigation into the PFKP-NF-κB-ABCC2 signaling pathway could offer new therapeutic strategies to improve the diagnosis and treatment of NSCLC.
Acknowledgments
The authors would like to thank the research facilities provided by Taizhou Hospital in Zhejiang Province.
Funding: The present study was funded by
Footnote
Reporting Checklist: The authors have completed the ARRIVE and MDAR reporting checklists. Available at https://tlcr.amegroups.com/article/view/10.21037/tlcr-23-567/rc
Data Sharing Statement: Available at https://tlcr.amegroups.com/article/view/10.21037/tlcr-23-567/dss
Peer Review File: Available at https://tlcr.amegroups.com/article/view/10.21037/tlcr-23-567/prf
Conflicts of Interest: All authors have completed the ICMJE uniform disclosure form (available at https://tlcr.amegroups.com/article/view/10.21037/tlcr-23-567/coif). The authors have no conflicts of interest to declare.
Ethical Statement: The authors are accountable for all aspects of the work in ensuring that questions related to the accuracy or integrity of any part of the work are appropriately investigated and resolved. The study was conducted in accordance with the Declaration of Helsinki (as revised in 2013). The Institutional Review Board of Taizhou Hospital of Zhejiang Province affiliated to Wenzhou Medical University approved this study (No. 2021-07-138-01). Informed consent was obtained prior to tissue collection from each patient. All operations involving experimental animals were approved by the Animal Ethics Committee of Taizhou Hospital, Zhejiang Province (No. tzy-2022001), and were carried out in compliance with Taizhou Hospital, Zhejiang Province institutional guidelines for the care and use of animals.
Open Access Statement: This is an Open Access article distributed in accordance with the Creative Commons Attribution-NonCommercial-NoDerivs 4.0 International License (CC BY-NC-ND 4.0), which permits the non-commercial replication and distribution of the article with the strict proviso that no changes or edits are made and the original work is properly cited (including links to both the formal publication through the relevant DOI and the license). See: https://creativecommons.org/licenses/by-nc-nd/4.0/.
References
- Sung H, Ferlay J, Siegel RL, et al. Global Cancer Statistics 2020: GLOBOCAN Estimates of Incidence and Mortality Worldwide for 36 Cancers in 185 Countries. CA Cancer J Clin 2021;71:209-49. [Crossref] [PubMed]
- Siegel RL, Miller KD, Fuchs HE, et al. Cancer statistics, 2022. CA Cancer J Clin 2022;72:7-33. [Crossref] [PubMed]
- Jasper K, Stiles B, McDonald F, et al. Practical Management of Oligometastatic Non-Small-Cell Lung Cancer. J Clin Oncol 2022;40:635-41. [Crossref] [PubMed]
- Miller KD, Nogueira L, Devasia T, et al. Cancer treatment and survivorship statistics, 2022. CA Cancer J Clin 2022;72:409-36. [Crossref] [PubMed]
- Chen P, Liu Y, Wen Y, et al. Non-small cell lung cancer in China. Cancer Commun (Lond) 2022;42:937-70. [Crossref] [PubMed]
- Herbst RS, Morgensztern D, Boshoff C. The biology and management of non-small cell lung cancer. Nature 2018;553:446-54. [Crossref] [PubMed]
- Kryczka J, Kryczka J, Czarnecka-Chrebelska KH, et al. Molecular Mechanisms of Chemoresistance Induced by Cisplatin in NSCLC Cancer Therapy. Int J Mol Sci 2021;22:8885. [Crossref] [PubMed]
- Shen J, Jin Z, Lv H, et al. PFKP is highly expressed in lung cancer and regulates glucose metabolism. Cell Oncol (Dordr) 2020;43:617-29. [Crossref] [PubMed]
- Wang G, Xu Z, Wang C, et al. Differential phosphofructokinase-1 isoenzyme patterns associated with glycolytic efficiency in human breast cancer and paracancer tissues. Oncol Lett 2013;6:1701-6. [Crossref] [PubMed]
- Chen J, Zou L, Lu G, et al. PFKP alleviates glucose starvation-induced metabolic stress in lung cancer cells via AMPK-ACC2 dependent fatty acid oxidation. Cell Discov 2022;8:52. [Crossref] [PubMed]
- Bruhn O, Cascorbi I. Polymorphisms of the drug transporters ABCB1, ABCG2, ABCC2 and ABCC3 and their impact on drug bioavailability and clinical relevance. Expert Opin Drug Metab Toxicol 2014;10:1337-54. [Crossref] [PubMed]
- Hoffmann U, Kroemer HK. The ABC transporters MDR1 and MRP2: multiple functions in disposition of xenobiotics and drug resistance. Drug Metab Rev 2004;36:669-701. [Crossref] [PubMed]
- Ito K, Oleschuk CJ, Westlake C, et al. Mutation of Trp1254 in the multispecific organic anion transporter, multidrug resistance protein 2 (MRP2) (ABCC2), alters substrate specificity and results in loss of methotrexate transport activity. J Biol Chem 2001;276:38108-14. [Crossref] [PubMed]
- Campa D, Müller P, Edler L, et al. A comprehensive study of polymorphisms in ABCB1, ABCC2 and ABCG2 and lung cancer chemotherapy response and prognosis. Int J Cancer 2012;131:2920-8. [Crossref] [PubMed]
- Chen Y, Zhou H, Yang S, et al. Increased ABCC2 expression predicts cisplatin resistance in non-small cell lung cancer. Cell Biochem Funct 2021;39:277-86. [Crossref] [PubMed]
- Jemnitz K, Heredi-Szabo K, Janossy J, et al. ABCC2/Abcc2: a multispecific transporter with dominant excretory functions. Drug Metab Rev 2010;42:402-36. [Crossref] [PubMed]
- Ke SZ, Ni XY, Zhang YH, et al. Camptothecin and cisplatin upregulate ABCG2 and MRP2 expression by activating the ATM/NF-κB pathway in lung cancer cells. Int J Oncol 2013;42:1289-96. [Crossref] [PubMed]
- Wheatley SP, Altieri DC. Survivin at a glance. J Cell Sci 2019;132:jcs223826. [Crossref] [PubMed]
- Sun XP, Dong X, Lin L, et al. Up-regulation of survivin by AKT and hypoxia-inducible factor 1α contributes to cisplatin resistance in gastric cancer. FEBS J 2014;281:115-28. [Crossref] [PubMed]
- Kinner A, Wu W, Staudt C, et al. Gamma-H2AX in recognition and signaling of DNA double-strand breaks in the context of chromatin. Nucleic Acids Res 2008;36:5678-94. [Crossref] [PubMed]
- Dean M, Moitra K, Allikmets R. The human ATP-binding cassette (ABC) transporter superfamily. Hum Mutat 2022;43:1162-82. [Crossref] [PubMed]
- Wang Z, Sun X, Feng Y, et al. Dihydromyricetin reverses MRP2-induced multidrug resistance by preventing NF-κB-Nrf2 signaling in colorectal cancer cell. Phytomedicine 2021;82:153414. [Crossref] [PubMed]
- Ausina P, Da Silva D, Majerowicz D, et al. Insulin specifically regulates expression of liver and muscle phosphofructokinase isoforms. Biomed Pharmacother 2018;103:228-33. [Crossref] [PubMed]
- Chen G, Liu H, Zhang Y, et al. Silencing PFKP inhibits starvation-induced autophagy, glycolysis, and epithelial mesenchymal transition in oral squamous cell carcinoma. Exp Cell Res 2018;370:46-57. [Crossref] [PubMed]
- Sanzey M, Abdul Rahim SA, Oudin A, et al. Comprehensive analysis of glycolytic enzymes as therapeutic targets in the treatment of glioblastoma. PLoS One 2015;10:e0123544. [Crossref] [PubMed]
- Gao X, Qin S, Wu Y, et al. Nuclear PFKP promotes CXCR4-dependent infiltration by T cell acute lymphoblastic leukemia. J Clin Invest 2021;131:e143119. [Crossref] [PubMed]
- Lee JH, Liu R, Li J, et al. EGFR-Phosphorylated Platelet Isoform of Phosphofructokinase 1 Promotes PI3K Activation. Mol Cell 2018;70:197-210.e7. [Crossref] [PubMed]
- Zhang L, Ke J, Min S, et al. Hyperbaric Oxygen Therapy Represses the Warburg Effect and Epithelial-Mesenchymal Transition in Hypoxic NSCLC Cells via the HIF-1α/PFKP Axis. Front Oncol 2021;11:691762. [Crossref] [PubMed]
- Wang Z, Zhang L, Ni Z, et al. Resveratrol induces AMPK-dependent MDR1 inhibition in colorectal cancer HCT116/L-OHP cells by preventing activation of NF-κB signaling and suppressing cAMP-responsive element transcriptional activity. Tumour Biol 2015;36:9499-510. [Crossref] [PubMed]
- Lin G, Li C, Huang C, et al. Co-expression of NF-κB-p65 and phosphorylated NF-κB-p105 is associated with poor prognosis in surgically resectable non-small cell lung cancer. J Cell Mol Med 2018;22:1923-30. [Crossref] [PubMed]
- Vollrath V, Wielandt AM, Iruretagoyena M, et al. Role of Nrf2 in the regulation of the Mrp2 (ABCC2) gene. Biochem J 2006;395:599-609. [Crossref] [PubMed]
- Rushworth SA, Zaitseva L, Murray MY, et al. The high Nrf2 expression in human acute myeloid leukemia is driven by NF-κB and underlies its chemo-resistance. Blood 2012;120:5188-98. [Crossref] [PubMed]
- Bellezza I, Giambanco I, Minelli A, et al. Nrf2-Keap1 signaling in oxidative and reductive stress. Biochim Biophys Acta Mol Cell Res 2018;1865:721-33. [Crossref] [PubMed]