Non-small cell lung cancer: current treatment and future advances
Introduction
Lung cancer is among the most deadly cancers for both men and women (1). Its death rate exceeds that of the three most common cancers (colon, breast, and pancreatic) combined (2). Over half of patients diagnosed with lung cancer die within one year of diagnosis and the 5-year survival is around 17.8% (3).
There are two main subtypes of lung cancer, small-cell lung carcinoma and non-small-cell lung carcinoma (NSCLC), accounting for 15% and 85% of all lung cancer, respectively (4). NSCLC is further classified into three types: squamous-cell carcinoma, adenocarcinoma, and large-cell carcinoma.
Squamous-cell carcinoma comprises 25–30% of all lung cancer cases. It arises from early versions of squamous cells in the airway epithelial cells in the bronchial tubes in the center of the lungs. This subtype of NSCLC is strongly correlated with cigarette smoking (5).
The most common type of lung cancer is adenocarcinoma; it comprises around 40% of all lung cancer. It arises from small airway epithelial, type II alveolar cells, which secrete mucus and other substances (6). Adenocarcinoma is the most common type of lung cancer in smokers and nonsmokers in men and women regardless of their age (7). It tends to occur in the periphery of the lung (8), which might be due to the addition of filters in cigarettes preventing large particles from entering the lungs. This results in deeper inhalation of cigarette smoke, leading to peripheral lesions (9). Compared to other types of lung cancer, adenocarcinoma tends to grow slower and has a greater chance of being found before it has spread outside of the lungs.
Large cell (undifferentiated) carcinoma accounts for 5–10% of lung cancers. This type of carcinoma shows no evidence of squamous or glandular maturation and as a result is often diagnosed by default through exclusion of other possibilities. Large cell carcinoma often begins in the central part of the lungs, sometimes into nearby lymph nodes and into the chest wall as well as distant organs (10). Large cell carcinoma tumors are strongly associated with smoking (11).
Risk factors
Smoking is the leading risk factor for lung cancer (2). When cigarettes became the major tobacco product manufactured in the 1900s, lung cancer became more common. Increases in the number of years or the number of packs smoked per day increases the degree of risk for lung cancer (12). Smoking causes at least 80% of lung cancer deaths.
A relative risk of developing lung cancer from passive smoking was found to be 1.14 to 5.20 in people who had never smoked but lived with a smoker based on a meta-analysis and comprehensive review (13). According to the U.S. Surgeon General, living with a smoker can increase a nonsmoker’s chance of developing lung cancer by about 20–30% (14).
Radon, a naturally occurring carcinogen, is among the risk factors linked with lung cancer, and approximately 21,000 lung cancer deaths in the United States have been linked to radon exposure (15). Although radon was initially linked with mine workers, there has been increasing concern attributed to indoor radon exposure from natural uranium deposits that are commonly found in basements. A collection of case-controlled studies from North American, Europe, and China has demonstrated increased incidence rates of lung cancer linked to residential radon exposure at levels of 2.7 picocuries per liter (pCi/L) (16-18).
Lung cancer is considered one of the most common cancers caused by occupational exposures. The use of asbestos in industry or manufacturing has been linked to increased incidence of mesothelioma and lung cancer (19,20). An association between asbestos fiber sizes as a strong predictor of lung cancer mortality has been found (21). Consequently, the U.S. government has taken steps to reduce asbestos use in commercial and industrial projects. Other occupational exposures linked to lung cancer include the use of arsenic and arsenic compounds (antifungal, outdoor wood preservatives, insecticides, herbicides, etc.), exposure to beryllium and beryllium oxide (X-ray and radiation technology, etc.), inhaled chemicals including cadmium, silica, vinyl chloride, nickel compounds, chromium compounds, coal products, mustard gas, and chloromethyl esters and diesel exhaust (22,23).
In big cities and other areas with traffic congestion, long-term and accumulated exposure to air pollution, including emissions composed of polycyclic aromatic hydrocarbons, is identified as a lung cancer risk factor (24). Air pollution has been associated with an 8% increased risk of all-cause lung cancer mortality (25).
Personal or family history of lung cancer serves as a risk factor for a person to develop lung cancer (26). There are certain genes and chromosomes that have been linked to an increased risk of lung cancer. Carriers of TP53 germline sequence variations who also smoke are more than 3 times more likely to develop lung cancer than nonsmokers (27). There are also reports of a marker on chromosome 15 associated with lung cancer, which was explored in three independent genetic studies (28-30). The marker contains three genes for subunits of the nicotine acetylcholine receptor. Cell change can occur when nicotine latches on to this protein, located on the cell surface. Based on results from these three independent studies, people with one copy of the marker have a 30% increased risk of developing lung cancer, while people with two copies have an increased risk of 70–80%.
Current treatment options
Surgery
Patients who have stage I, II, and IIIA NSCLC typically have surgery to remove the tumor if the tumor is found to be resectable and the patient is able to tolerate surgery. Surgeons may remove a lobe or section of the lung containing the tumor. To determine if the tumor is resectable, imaging studies and biopsies are completed as well as an evaluation of patient factors to determine operability. Currently, many surgeons utilize video-assisted thorascopic surgery (VATS), where a small incision is made in the chest and a thorascope is inserted. A lobe can be removed via the scope through this small incision so that a larger incision does not have to be made (31).
Adjuvant
Some patients who have undergone a resection surgery may benefit from adjuvant therapy in reducing the risk of lung cancer relapse. Adjuvant therapy may include radiation, chemotherapy, and targeted therapy. Patients with stage IIA, IIB, and IIIA NSCLC usually receive chemotherapy after surgery to kill any remaining cancer cells in order to prolong survival (32).
Chemotherapy
Approximately 40% of newly diagnosed lung cancer patients are stage IV. The goal for treating these patients is to improve survival and reduce disease-related adverse events. For stage IV NSCLC, cytotoxic combination chemotherapy is the first-line therapy, which might be influenced by histology, age vs. comorbidity, and performance status (PS) (33). The American Society of Clinical Oncology states that treatment for a patient with a PS of 0 or 1 is a regimen of a platinum (cisplatin or carboplatin) plus paclitaxel, gemcitabine, docetaxel, vinorelbine, irinotecan, or pemetrexed (34). Results from four large multicenter randomized clinical trials studying the above agents with either platinum or carboplatin have yielded similar results. From these studies, results have shown that no single regimen demonstrated a significant superiority over any other combination. Median overall survival for patients in these studies was approximately 8–10 months (35-38). The specific combination depends on types and frequencies of toxic effects and should be decided on an individual basis. However, adenocarcinoma patients may benefit from pemetrexed. Cisplatin is the slightly more effective platinum, however it has been associated with more side effects. For patients with a PS of 2, evidence suggests that they may need only one drug, which is typically not platinum (39). For chemotherapy treatment, serious adverse events should prompt a change in agents. Therapy should also be stopped if the cancer grows or if, after four treatment cycles, the disease is stable but the treatment is not shrinking the tumors (40,41). Patients with a PS of 3 do not typically benefit from receiving cytotoxic chemotherapy because the risk of adverse events could worsen their quality of life significantly. For these patients basic supportive care is generally recommended.
Radiotherapy
Radiotherapy uses high-energy beams to damage DNA within cancer cells, thereby destroying them. This therapy can help control or eliminate tumors at specific sites in the body. Patients with NSCLC that is localized to the chest and who are not candidates for surgical resection may benefit from radiotherapy. Radiotherapy also can be part of palliative care to improve quality of life in NSCLC patients who do not respond to surgery or chemotherapy (42).
A technique called stereotactic body radiation therapy (SBRT) is used for early-stage NSCLC patients who have a single small nodule in the lung without any metastases to nearby lymph nodes. This technique uses an advanced coordinate system to precisely locate the tumor and ensure precise placement of the tracking device. This enables delivery of concentrated and highly focused radiation treatment. In a meta-analysis comparing the effectiveness of radiotherapy with photons, protons, and carbon-ions for NSCLC, it was found that SBRT offered greater 2-year overall survival rates, lower costs, and greater patient convenience (43). In a prospective phase II study, 50-month results of 70 medically inoperable patients receiving SBRT showed that receiving SBRT resulted in high rates of local control in medically inoperable patients with Stage 1 NSCLC (44). In a phase III multicenter study of patients with early stage but medically inoperable NSCLC, toxicity and efficacy of SBRT was studied. Of the 55 patients evaluated, it was found that patients who received SBRT had a survival rate of 55.8% at three years along with moderate treatment-related morbidity (45). As a result of these studies and some others, SBRT has been found to offer local control and outcomes approaching surgical resection with lower rates of treatment-related morbidity (46,47).
Biomarker testing
Personalized medicine by targeting appropriate molecular targets in tumors has helped improve survival in patients with NSCLC (48). There are targeted agents that have been successful against epidermal growth factor receptor (EGFR) mutations and anaplastic lymphoma kinase (ALK) rearrangements. Through genomic testing, other molecular changes have been found including gene rearrangements of ROS1 and RET, amplification of MET and activating mutations in BRAF, HER2 and KRAS genes, which might be potential targets for future therapies.
Epidermal growth factor receptor (EGFR) gene
EGFR is a cell-surface tyrosine kinase receptor that can activate pathways associated with cell growth and proliferation when activated. In cancers, mutations of EGFR produce uncontrolled cell division through constant activation. EGFR gene mutations are present in 10–15% of lung cancer adenocarcinomas patients who are of European and Asian descent, in those who have never smoked, and female (49-51). While these characteristics are predominant, mutation testing is integral to finding those patients who would benefit from targeted tyrosine kinase inhibitor therapy. Exons 18–21 are where mutations in EGFR commonly occur, which confers sensitivity to EGFR tyrosine kinase inhibitors; these exons encode a portion of the EGFR kinase domain. Approximately 90% of these mutations are exon 19 deletions and L858R point mutation on exon 21 and are correlated with a response rate of 70% in patients receiving erlotinib or gefitinib treatment (52).
KRAS
KRAS is a common mutated oncogene associated with NSCLC due to missense mutations that substitute an amino acid at position, 12, 13 or 61. Single amino acid mutations at residues G12 and G13 are predominantly recognized. KRAS mutations are identified more frequently in adenocarcinomas, Caucasians, and individuals with a smoking history (53). Approximately 10–25% of patients with adenocarcinoma have KRAS mutation-associated tumors (54). In terms of overlapping with other oncogenic mutations, KRAS has been predominantly found in tumor types that are wild type for EGFR and ALK, meaning these mutations are a new molecular subset of NSCLC. Emerging data suggests that there could be a possible prognostic value of KRAS mutations but a limited role as a predictor for EGFR tyrosine kinase inhibitors or cytotoxic chemotherapy (48,52). One study has suggested that it is possible to directly target a KRAS mutational subset with small-molecule inhibitors directed towards the common G12C lung cancer mutation, a common mutation in smokers vs. non-smokers. These possible new agents rely on mutant cysteine for binding and do not affect the wild-type KRAS protein, thereby showing selectivity for a specific subtype (55).
Anaplastic lymphoma kinase (ALK)
Approximately 3–7% of all lung tumors contain ALK mutations (56-58) where these mutations are commonly seen in patients who are of a younger age. In a study by Koh et al., patients with ALK mutations had a median age of 49 compared to a median age of 61 in patients who were ALK negative (P<0.001; n=221) (59). ALK mutations are also more common in adenocarcinoma patients with acinar histology or signet ring cells or those who have never smoked (60,61). Rearrangement in EML-4-ALK is the most common ALK rearrangement seen in NSCLC patients. These rearrangements arise on chromosome 2p23 due to the fusion between the 5’ and of the EML-4 gene and the 3’ end of the ALK gene, of which there are at least nine different fusion variants. EML-4 mutations are common in adenocarcinomas of patients who had never smoked or had been light smokers whose tumors lack both EGFR and KRAS mutations (56,61). ALK mutations are non-overlapping with other oncogenic mutations associated with NSCLC such as EGFR or RAS (61,62). Other ALK mutations not involving EML-4 have been found, including KIF5B-ALK and TFG-ALK. In terms of treatment, patients with EML4-ALK fusions or ALK rearrangements do not benefit from EGFR-specific tyrosine kinase inhibitor therapy (63).
Currently there is an FDA-approved agent, crizotinib (Xalkori®, Pfizer), that targets constitutively activated receptor tyrosine kinases that result from EML4-ALK and other ALK fusions. In a single arm study of ALK-positive metastatic NSCLC (64), patients exhibited objective response rates of 50–61%. In a trial with previously untreated advanced non-squamous ALK-positive NSCLC, patients were randomized to receive crizotinib 250 mg by mouth twice a day (n=172) or intravenous chemotherapy (pemetrexed 500 mg/m2 plus either cisplatin 75 mg/m2 or carboplatin target area under the curve 5–6 mg/mL/min (PPC group); all administered intravenously every three weeks for ≤6 cycles, n=171). The primary endpoint of the study was progression-free survival while the secondary endpoints included overall response rate, overall survival, safety, and patient-reported outcomes. They found that crizotinib prolonged progression-free survival 10.9 vs. 7 months in patients receiving PPC. The overall response rate was also higher in patients receiving crizotinib at 74% vs. 45% in patients receiving PPC. Overall crizotinib showed significant improvements in progression-free survival and overall response rate compared with standard chemotherapy and its safety profile was acceptable (64). This pivotal trial established crizotinib as the standard of care for patients with previously untreated, advanced ALK-positive non-squamous NSCLC.
BRAF
BRAF is a proto-oncogene, which is a regulated signal transduction serine/threonine protein kinase that is able to promote cell proliferation and survival (65). BRAF somatic mutations have been found in 1–4% of all NSCLC, most commonly in patients with adenocarcinomas (54,66-70). These mutations are more commonly linked with former/current smokers (69,70). The kinase domain locations of BRAF mutations in lung cancer patients differ from BRAF mutations in breast cancer patients. In a study of 697 patients with lung adenocarcinoma, BRAF mutations were present in 18 (3%), of which the BRAF mutations identified were V600E (50%), G469A (39%) and D594G (11%) (69). A great majority of BRAF mutations have been found to be non-overlapping with other oncogenic mutations in NSCLC (EGFR mutations, ALK rearrangements, etc.).
Role of immunotherapy in non-small cell lung cancer
Immunotherapy is a breakthrough treatment in oncology that uses the body’s own natural defense system to fight off cancer. Some cancer cells share characteristics with healthy cells and thus the immune system cannot differentiate between the body’s normal and abnormal (cancer) cells (71). It is believed that immunotherapy works by boosting the immune system so that it can target cancer cells and stop or slow the growth of cancer cells, by preventing cancer cells from spreading to other parts of the body, or by helping the immune system increase its effectiveness (72). Data has shown that improved survival is associated with a strong antitumor immune response. Higher numbers of CD4+ T cells, CD8+ T cells, natural killer cells, and/or dendritic cells are associated with better patient survival (73-75).
New strategies in immunotherapy are targeting immune-modulating mechanisms that help tumor cells defend themselves against the immune system (Table 1). This approach targets immune checkpoint pathways, which includes the blockade of the inhibitory receptors cytotoxic T-lymphocyte-associated antigen 4 (CTLA-4) and programmed cell death-1 (PD-1) and its ligand, PD-L1. These immune checkpoints are used by the immune system to maintain self-tolerance and regulate the immune response in the body to protect tissues from damage as the immune system launches a response to a pathogen (82). Immune checkpoint pathways can be dysregulated by tumor resistance mechanisms.
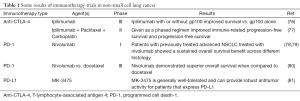
Full table
Cytotoxic T-lymphocyte-associated antigen 4 (anti-CTLA-4) therapy
CTLA-4 has an important role in down-regulating T cell activation, proliferation, and effector functions (83). Ipilimumab therapy in patients previously treated for metastatic melanoma demonstrated improved overall survival (84). Key analyses from a phase III trial showed that in the ipilimumab-alone group, 1-year survival was estimated to be 45.6% vs. 21.6–38% in patients receiving the other treatment regimens in the study. After two years, approximately 24% of the patients who received ipilimumab were still alive (76).
Ipilimumab in combination with paclitaxel and carboplatin in two different treatment schedules (phased or concurrent) was evaluated in chemotherapy-naïve patients who had stage IIIb or IV NSCLC. This was a phase II trial in which the primary endpoint was an improvement of the immune-related progression-free survival (77). Patients in the concurrent treatment arm received ipilimumab concurrently for four doses followed by placebo, while the patients in the phase arm received two doses of placebo and then four doses of ipilimumab. Following six cycles of the combination, patients received a maintenance regimen of placebo or ipilimumab every 12 weeks or until disease progression. When comparing the phased schedule versus chemotherapy alone, immune-related overall response rate was nearly doubled (32% vs. 18%, respectively). Progression free survival improvements in patients with squamous cell histology has prompted a larger trial using the same schedule in patients with squamous cell carcinoma
Programmed cell death-1 (PD-1) and PD-L1
The PD-1 immune checkpoint pathway is an additional target for NSCLC therapy. It has a role in preventing T cell activation through down-regulation of the immune system, which promotes self-tolerance and reduces autoimmunity. Ultimately, the blocking of this pathway augments the T cell response in the body (85,86). There have been some promising results in early clinical trials with two agents, nivolumab and MK-3475, discussed next.
Nivolumab was recently approved by the FDA in October, 2015, based on the CheckMate 057 trial that showed improvement in overall survival in an open-label and randomized multicenter trial comparing nivolumab to docetaxel in metastatic non-squamous NSCLC patients (78,79). CheckMate 017 was an open-label, international, and randomized trial comparing nivolumab to docetaxel in previously treated patients with advanced, squamous cell NSCLC, and it was stopped early by Bristol-Myers Squibb after meeting its end point (80). Overall survival and an objective response rate and progression-free survival were assessed in the trial, which randomized 272 patients with advanced or metastatic squamous cell NSCLC to receive nivolumab or docetaxel. According to the study’s Data Monitoring Committee, nivolumab demonstrated superior overall survival when compared to docetaxel.
MK-3475 is a humanized monoclonal antibody that targets the ligand of PD-1, PD-L1, thereby removing the inhibition of T cell activation against tumor cells. One study looked at 84 NSCLC patients with no prior systemic therapy for metastatic disease; these patents were then tested to see if the tumors in their tissue expressed PD-L1 (81). Of these, 57 patients had tumors that expressed PD-L1, however only 45 were eligible to be randomized to receive 2 mg/kg of MK-3475 every three weeks (n=6), 10 mg/kg every three weeks (n=23), or 10 mg/kg every two weeks (n=16). Overall response rate was 36% across all groups (67% in the 2 mg/kg every three weeks group, 27% in the 10 mg/kg every three weeks group, and 35% in the 10 mg/kg every two weeks group). Common side effects included fatigue, pruritus, diarrhea, and dyspnea and dermatitis acneiform. Data from this study suggests that MK-3475 is generally well tolerated and effective in patients with locally advanced or metastatic NSCLC that expresses PD-L1.
Immunotherapy through vaccine development
The goal of vaccine therapy in NSCLC is to shift the immune balance in favor of activation so that the host may launch a response to tumor-associated antigens (87,88). Currently there are two developing strategies to use vaccines in the treatment of NSCLC: tumor vaccines and antigen-specific immunotherapy (Table 2). Tumor (whole-cell) vaccines are developed from autologous or allogenic tumor cells. These vaccines work by exposing the host’s immune system to various tumor-associated antigens (99). Antigen-specific immunotherapy incorporates specific antitumor immunity against antigens expressed on tumor cells. Since these vaccines target a specific antigen, they may not be able to be used in all patients. Currently there are some ongoing phase III trials involving potential new vaccine therapies in NSCLC (Table 2).

Full table
Tumor cell vaccines
Belagenpumatucel-L (Lucanix®, NovaRx Corp.) is an allogenic tumor cell vaccine using genetically modified whole tumor cells. It is composed of 4 irradiated NSCLC lines gene-modified with transforming-growth factor (TGF)-β2, of which 2 are adenocarcinoma lines, 1 is a squamous line, and one is a large-cell carcinoma line (H460, H520, SKLU-1, and RH2, respectively) (100). Higher levels of (TGF)-β2 are associated with suppression of the immune system, which leads to neutralization of natural killers cells and suppression of dendritic cells (101). Another role of belagenpumatucel-L is that it has immune-stimulating effects through activation of a specific T cell response against NSCLC cells. The plasmid (TGF)-β2 transgene portion of the vaccine may suppress tumor production through its expressed antisense RNA (102).
Belagenpumatucel-L was evaluated (phase II clinical trial) in 75 patients with NSCLC stages II-IV and was well tolerated with positive responses in patients who showed elevated antibody levels (89). In a phase III trial of belagenpumatucel-L, patients without progression after completion of frontline chemotherapy (phase IIIA, n=42; phase IIIB/IV, n=490) were randomized 1:1 (belagenpumatucel-L or placebo) between 4 and 17.4 weeks from the end of frontline chemotherapy and were treated until disease progression or withdrawal (90). The study evaluated the utility of belagenpumatucel-L in improving overall survival. Secondary endpoints of the study included progression-free survival, response rate, and safety. Of the enrolled 532 patients (270 vaccine and 262 placebo), 57% had adenocarcinoma and 27% had squamous cell carcinoma. This study did not achieve its predefined primary endpoint; median overall survival in the vaccine group was 20.3 vs. 17.8 months in the placebo group [hazard ratio (HR) 0.94; P=0.594]. A predefined COX regression helped identify prognostic factors for improved outcome and showed a significant and clinically meaningful greater overall survival in the vaccine group over placebo. The overall survival was improved by 7.3 months in the vaccine group for patients who were randomized within 12 weeks of chemotherapy completion. The median overall survival in the group was 20.7 months with belagenpumatucel-L compared to 13.7 months with placebo (HR 0.75; P=0.083). In patients who had previously received pretreatment radiation, improved median overall survival was shown (90).
Antigen-specific vaccines
Melanoma-associated antigen-A3 (MAGE-A3)
MAGE-A3 is primarily expressed on tumor cells (35% of NSCLCs express it) but not on normal cells except testicular germ cells and placental trophoblast (88), where its increased expression is associated with advanced disease and poor prognosis (103).
In a multicenter, double-blinded phase II clinical trial, the efficacy of MAGE-A3 as a tumor-specific vaccine target in NSCLC was evaluated for tolerability and efficacy (91). One hundred eighty-two patients with completely resected MAGE-A3 (+) stage pib OR PII were assigned in a 2:1 ratio to receive postoperative recombinant MAGE-A3 protein plus adjuvant or placebo. Patients were vaccinated for a total of five cycles every three weeks followed by 8 vaccinations every three months. The primary endpoint of this study was disease-free interval and other endpoints included safety, disease-free survival, and overall survival. After a median follow-up of 28 months, group comparisons of disease-free survival and overall survival were 0.73 (95% CI: 0.45–1.16) and 0.66 (95% CI: 0.36–1.20), respectively. The results of the study showed a positive trend for activity of MAGE-A3 in the treatment of NSCLC with improvement of disease-free interval and disease-free survival of 27%. Although this improvement was not statistically significant, the promise of the results prompted the development of a phase III trial named MAGRIT (MAGE-A3 as Adjuvant Non-Small Cell Lung Cancer Immunotherapy).
The MAGRIT trial evaluated disease-free survival and overall survival, yearly disease-free survival from 2–5 years, lung cancer specific survival, disease-free specific survival, and adverse events in curatively resected patients whose lung cancers were MAGE-A3 positive. There were 2,270 patients who were stage IB, II, and IIIA and assigned to either the MAGE-A3 or placebo group. MAGE-A3 as an adjuvant treatment did not increase disease-free survival compared to placebo in the overall population or in the patients who did not receive chemotherapy (92). While the trial did not meet its primary endpoint, the MAGRIT trial helps confirm that vaccines are well tolerated with mild effects and no apparent increase in immune-mediated disorders.
Liposome BLP25 vaccine
Liposomal BLP25 (L-BLP25) is a peptide-based vaccine that targets the exposed core peptide of a membrane-associated glycoprotein (MUC1), which is commonly found on the apical surface of most epithelial cells of the respiratory, genitourinary, and digestive system. When MUC1 becomes aberrantly glycosylated, it becomes immunologically different from the MUC1 on normal cells. Overexpression of MUC1 is associated with approximately 60% of lung cancers and is also associated with greater immunosuppression and a poorer prognosis in patients with adenocarcinoma (104).
The effects of the L-BLP25 vaccine on survival and toxicity were evaluated in a randomized phase IIb study in patients with stage IIIB and IV NSCLC. Patients were randomized into the L-BLP25 plus best supportive care (BSC) or BSC alone group, with the groups having 88 and 83 patients, respectively. Patients in the L-BLP25 plus BSC group had a median survival time of 4.4 months longer than the patients in the BSC alone group (93). Further results from updated survival analysis indicated a median survival time of 17.2 months and a 31% 3-year survival rate in patients in the L-BLP25 plus BSC arm compared to 13.0 months and 17% 3-year survival rate in the group of patients receiving BSC alone (94).
A phase III trial (START)was undertaken based on the results of the phase IIb study. Patients (n=1,513) with unresectable stage III NSCLC who did not progress after primary chemo-radiotherapy were randomized 2:1 to receive either L-BLP25 or placebo. Despite L-BLP25 being well tolerated, the START trial failed to meet its primary efficacy endpoint. However, there was a significant survival advantage in those patients treated with L-BLP25 and concurrent chemotherapy and radiation (95).
CIMAVax EGF
This vaccine was developed for the treatment of advanced stages IIIB and IV NSCLC after first-line chemotherapy. It was developed and is currently approved for use in Cuba, Peru, and Venezuela. It was developed from human recombinant EGF conjugated to the P64K Neisseria meningitides recombinant protein (96).
Prevaccination treatment with cyclophosphamide was evaluated in a pilot trial with the role of cyclophosphamide being to reduce inhibition of T-suppressor cells. Throughout the trials, serial antibody measurements of EGF were done through an enzyme-linked immunosorbent assay (ELISA) and were stratified based on their measurement; a good antibody responder produced an antibody response to a titer greater than 1:4,000 and a poor antibody responder had a titer less than 1:4,000. The results of the pooled trials showed that there was no significant difference in antibody responses with the pretreatment of cyclophosphamide prior to EGF administration. The use of ISA 51 rather than aluminum hydroxide promoted a significant difference in the number of antibody responders. Thus, there appears to be a survival time relationship with anti-EGF antibody titers and immune response duration (105).
A phase II trial evaluating the immunogenicity safety and effect on survival of an EGF-based cancer vaccine included 80 patients with stage IIIB/IV NSCLC after completing first-line chemotherapy (96). Patients were randomly assigned to receive best supportive care or EGF vaccinations. Good antibody responders who were vaccinated had a longer median overall survival of 11.7 months compared to 3.6 months in vaccinated, poor antibody responders. A longer median overall survival of 13 months was found in vaccinated patients with serum EGF levels below 168 pg/mL versus 5.6 months in vaccinated patients whose serum EGF levels were greater than 168 pg/mL. The results of the trial showed a trend toward increased overall survival for vaccinated patients; this was found to be statistically significant in the subgroup of patients younger than the age of 60 versus patients over the age of 60 (11.57 and 5.33 months respectively, P=0.124). There was a direct correlation between a decrease in serum EGF levels and survival and a correlation between antibody response and survival.
TG4010 (MVA-MUC1-IL-2) vaccine
Like the L-BLP25 vaccine, TG4010 targets the MUC1 antigen on malignant cells but uses a recombinant vaccine virus (modified virus of Ankara or MVA) that encodes human MUC1 and IL-2. There was induced expression of MUC1 on the cell surface after the transduction of peripheral blood mononuclear cells obtained from healthy donors with MVA-MUC1-IL-2 (106).
In a phase II randomized, open-label study, two schedules of TG4010 combined with first-line chemotherapy were compared in patients with stage IIIB/IV NSCLC. In arm 1 (n=44) TG4010 was combined with cisplatin (100 mg/m2 day 1) and vinorelbine (25 mg/m2 days 1 and 8) while in arm 2 (n=21) patients were treated with TG4010 monotherapy until disease progression, followed by TG4010 plus the same chemotherapy as in arm 1. Median survivals for arms 1 and 2 were 12.7 and 14.9 months, respectively. Patients with a detectable CD8 T cell response also showed detectable immune responses against MUC1 antigen and showed increased time to tumor progression and longer median survival when compared to patients with no detectable CD8 T cell response. This study showed that the vaccine had a MUC1-specific cellular immune response (97).
A larger phase IIb trial was developed to evaluate 6-month progression-free survival with a target rate of 40% or higher in the experimental group (98). One hundred and forty-eight patients with stage IIIB/IV NSCLC expressing MUC1 immunohistochemistry were enrolled in the study. Seventy-four patients were allocated to the combination therapy group and received TG4010 plus cisplatin and gemcitabine for up to six cycles, and another 74 patients received only chemotherapy. Comparing the progression-free survival at 6 months between patients in the TG4010 with chemotherapy arm and those in the chemotherapy-alone arm, the results did not differ significantly [43.2% (95% CI: 33.4–53.5%) and 35.1% (95% CI: 25.9–45.3%), respectively, P=0.307]. Median overall survival for patients in the TG4010 was 10.7 months (95% CI: 8.8–18.0) and 10.3 months in the chemotherapy-alone group (95% CI: 8.3–12.5); these results failed to show statistical significance. Longer median overall survival was found in patients who had an objective response to TG4010 based on the World Health Organization (WHO) imaging criteria. Common side effects in the TG4010 group included fever, abdominal pain, and injection-site pain of any grade, following the National Cancer Institute Common Toxicity Criteria. Using these criteria, the rates of serious adverse events did not differ significantly between the TG4010 group and chemotherapy-alone group (52.1% and 47.2%, respectively). A shorter median overall survival and increased rates of adverse events were found in patients with increased numbers of activated CD16+, CD56+, CD69+, and natural killer cells measured before treatments when compared to patients with normal natural killer cell populations.
Conclusions
Significant advances have been made toward the reduction of occupational health hazards associated with lung cancer, especially smoking, and for the prevention of various disorders. In recent decades, targeted therapy and immunotherapy have made noticeable contributions to the improved management of lung cancer. Additionally, genetic and biomarker testing are helping in the personalized management of the various forms of lung cancer. Through personalized management of NSCLC, treatments are individualized and can target specific mutations with greater precision with the goal of lengthening progression-free survival. Immunotherapy presents the idea of boosting and guiding the body’s own immune defenses to target cancers cells. There are current clinical trials investigating the utilization of vaccines to treat NSCLC. Because lung cancer causes more deaths in the United States than any other cancer, research is constantly being pursued to develop novel treatments.
Acknowledgements
None.
Footnote
Conflicts of Interest: The authors have no conflicts of interest to declare.
References
- Siegel R, Ma J, Zou Z, et al. Cancer statistics, 2014. CA Cancer J Clin 2014;64:9-29. [Crossref] [PubMed]
- American Cancer Society. Cancer Facts and Figures 2015. Available online: http://www.cancer.org/acs/groups/content/@editorial/documents/document/acspc-044552.pdf
- National Cancer Institute. SEER Cancer Statistics Review, 1975-2011. Available online: http://seer.cancer.gov/csr/1975_2011/
- Sher T, Dy GK, Adjei AA. Small cell lung cancer. Mayo Clin Proc 2008;83:355-67. [Crossref] [PubMed]
- Kenfield SA, Wei EK, Stampfer MJ, et al. Comparison of aspects of smoking among the four histological types of lung cancer. Tob Control 2008;17:198-204. [Crossref] [PubMed]
- Noguchi M, Morikawa A, Kawasaki M, et al. Small adenocarcinoma of the lung. Histologic characteristics and prognosis. Cancer 1995;75:2844-52. [Crossref] [PubMed]
- Couraud S, Zalcman G, Milleron B, et al. Lung cancer in never smokers--a review. Eur J Cancer 2012;48:1299-311. [Crossref] [PubMed]
- Travis WD, Travis LB, Devesa SS. Lung cancer. Cancer 1995;75:191-202. [Crossref] [PubMed]
- Stellman SD, Muscat JE, Hoffmann D, et al. Impact of filter cigarette smoking on lung cancer histology. Prev Med 1997;26:451-6. [Crossref] [PubMed]
- Brambilla E, Pugatch B, Geisinger K, et al. Large cell carcinoma. In: Travis W, Brambilla E, Müller-Hermelink H, et al. editors. World Health Organization Classification of Tumours Pathology and Genetics of Tumours of the Lung, Pleura, Thymus and Heart. WHO Press, Geneva, 2004:45-50.
- Muscat JE, Stellman SD, Zhang ZF, et al. Cigarette smoking and large cell carcinoma of the lung. Cancer Epidemiol Biomarkers Prev 1997;6:477-80. [PubMed]
- Hecht SS. Tobacco smoke carcinogens and lung cancer. J Natl Cancer Inst 1999;91:1194-210. [Crossref] [PubMed]
- Whitrow MJ, Smith BJ, Pilotto LS, et al. Environmental exposure to carcinogens causing lung cancer: epidemiological evidence from the medical literature. Respirology 2003;8:513-21. [Crossref] [PubMed]
- U.S. Dept. of Health and Human Services. The Health Consequences of Smoking-50 Years of Progress. A Report of the Surgeon General, 2014. Available online: http://www.surgeongeneral.gov/library/reports/50-years-of-progress/
- United States Environmental Protection Agency. Health Risk of Radon. Available online: https://www.epa.gov/radon/health-risk-radon
- Krewski D, Lubin JH, Zielinski JM, et al. Residential radon and risk of lung cancer: a combined analysis of 7 North American case-control studies. Epidemiology 2005;16:137-45. [Crossref] [PubMed]
- Krewski D, Lubin JH, Zielinski JM, et al. A combined analysis of North American case-control studies of residential radon and lung cancer. J Toxicol Environ Health A 2006;69:533-97. [Crossref] [PubMed]
- Darby S, Hill D, Auvinen A, et al. Radon in homes and risk of lung cancer: collaborative analysis of individual data from 13 European case-control studies. BMJ 2005;330:223. [Crossref] [PubMed]
- Hodgson JT, Darnton A. The quantitative risks of mesothelioma and lung cancer in relation to asbestos exposure. Ann Occup Hyg 2000;44:565-601. [Crossref] [PubMed]
- Berman DW, Crump KS. A meta-analysis of asbestos-related cancer risk that addresses fiber size and mineral type. Crit Rev Toxicol 2008;38 Suppl 1:49-73. [Crossref] [PubMed]
- Stayner L, Kuempel E, Gilbert S, et al. An epidemiological study of the role of chrysotile asbestos fibre dimensions in determining respiratory disease risk in exposed workers. Occup Environ Med 2008;65:613-9. [Crossref] [PubMed]
- van Loon AJ, Kant IJ, Swaen GM, et al. Occupational exposure to carcinogens and risk of lung cancer: results from The Netherlands cohort study. Occup Environ Med 1997;54:817-24. [Crossref] [PubMed]
- Spyratos D, Zarogoulidis P, Porpodis K, et al. Occupational exposure and lung cancer. J Thorac Dis 2013;5 Suppl 4:S440-5. [PubMed]
- Vineis P, Husgafvel-Pursiainen K. Air pollution and cancer: biomarker studies in human populations. Carcinogenesis 2005;26:1846-55. [Crossref] [PubMed]
- Pope CA 3rd, Burnett RT, Thun MJ, et al. Lung cancer, cardiopulmonary mortality, and long-term exposure to fine particulate air pollution. JAMA 2002;287:1132-41. [Crossref] [PubMed]
- Sellers T, Yang P. Familial and genetic influences on risk of lung cancer. In: King R, Rotter J, Motulsky A. editors. The Genetic Basis of Common Diseases. New York: NY, Oxford University, 2002:700-12.
- Hwang SJ, Cheng LS, Lozano G, et al. Lung cancer risk in germline p53 mutation carriers: association between an inherited cancer predisposition, cigarette smoking, and cancer risk. Hum Genet 2003;113:238-43. [Crossref] [PubMed]
- Thorgeirsson TE, Geller F, Sulem P, et al. A variant associated with nicotine dependence, lung cancer and peripheral arterial disease. Nature 2008;452:638-42. [Crossref] [PubMed]
- Hung RJ, McKay JD, Gaborieau V, et al. A susceptibility locus for lung cancer maps to nicotinic acetylcholine receptor subunit genes on 15q25. Nature 2008;452:633-7. [Crossref] [PubMed]
- Amos CI, Wu X, Broderick P, et al. Genome-wide association scan of tag SNPs identifies a susceptibility locus for lung cancer at 15q25.1. Nat Genet 2008;40:616-22. [Crossref] [PubMed]
- Howington JA, Blum MG, Chang AC, et al. Treatment of stage I and II non-small cell lung cancer: Diagnosis and management of lung cancer, 3rd ed: American College of Chest Physicians evidence-based clinical practice guidelines. Chest 2013;143:e278S-313S.
- Chemotherapy in non-small cell lung cancer: a meta-analysis using updated data on individual patients from 52 randomised clinical trials. Non-small Cell Lung Cancer Collaborative Group. BMJ 1995;311:899-909. [Crossref] [PubMed]
- Ramalingam S, Belani C. Systemic chemotherapy for advanced non-small cell lung cancer: recent advances and future directions. Oncologist 2008;13 Suppl 1:5-13. [Crossref] [PubMed]
- Masters GA, Temin S, Azzoli CG, et al. Systemic Therapy for Stage IV Non-Small-Cell Lung Cancer: American Society of Clinical Oncology Clinical Practice Guideline Update. J Clin Oncol 2015;33:3488-515. [Crossref] [PubMed]
- Kelly K, Crowley J, Bunn PA Jr, et al. Randomized phase III trial of paclitaxel plus carboplatin versus vinorelbine plus cisplatin in the treatment of patients with advanced non--small-cell lung cancer: a Southwest Oncology Group trial. J Clin Oncol 2001;19:3210-8. [PubMed]
- Scagliotti GV, De Marinis F, Rinaldi M, et al. Phase III randomized trial comparing three platinum-based doublets in advanced non-small-cell lung cancer. J Clin Oncol 2002;20:4285-91. [Crossref] [PubMed]
- Schiller JH, Harrington D, Belani CP, et al. Comparison of four chemotherapy regimens for advanced non-small-cell lung cancer. N Engl J Med 2002;346:92-8. [Crossref] [PubMed]
- Fossella F, Pereira JR, von Pawel J, et al. Randomized, multinational, phase III study of docetaxel plus platinum combinations versus vinorelbine plus cisplatin for advanced non-small-cell lung cancer: the TAX 326 study group. J Clin Oncol 2003;21:3016-24. [Crossref] [PubMed]
- Gridelli C, Ardizzoni A, Le Chevalier T, et al. Treatment of advanced non-small-cell lung cancer patients with ECOG performance status 2: results of an European Experts Panel. Ann Oncol 2004;15:419-26. [Crossref] [PubMed]
- Pisters KM, Evans WK, Azzoli CG, et al. Cancer Care Ontario and American Society of Clinical Oncology adjuvant chemotherapy and adjuvant radiation therapy for stages I-IIIA resectable non small-cell lung cancer guideline. J Clin Oncol 2007;25:5506-18. [Crossref] [PubMed]
- Scott WJ, Howington J, Feigenberg S, et al. Treatment of non-small cell lung cancer stage I and stage II: ACCP evidence-based clinical practice guidelines (2nd edition). Chest 2007;132:234S-242S.
- Amini A, Yeh N, Gaspar LE, et al. Stereotactic body radiation therapy (SBRT) for lung cancer patients previously treated with conventional radiotherapy: a review. Radiat Oncol 2014;9:210. [Crossref] [PubMed]
- Grutters JP, Kessels AG, Pijls-Johannesma M, et al. Comparison of the effectiveness of radiotherapy with photons, protons and carbon-ions for non-small cell lung cancer: a meta-analysis. Radiother Oncol 2010;95:32-40. [Crossref] [PubMed]
- Fakiris AJ, McGarry RC, Yiannoutsos CT, et al. Stereotactic body radiation therapy for early-stage non-small-cell lung carcinoma: four-year results of a prospective phase II study. Int J Radiat Oncol Biol Phys 2009;75:677-82. [Crossref] [PubMed]
- Timmerman R, Paulus R, Galvin J, et al. Stereotactic body radiation therapy for inoperable early stage lung cancer. JAMA 2010;303:1070-6. [Crossref] [PubMed]
- Lagerwaard FJ, Verstegen NE, Haasbeek CJ, et al. Outcomes of stereotactic ablative radiotherapy in patients with potentially operable stage I non-small cell lung cancer. Int J Radiat Oncol Biol Phys 2012;83:348-53. [Crossref] [PubMed]
- Onishi H, Shirato H, Nagata Y, et al. Stereotactic body radiotherapy (SBRT) for operable stage I non-small-cell lung cancer: can SBRT be comparable to surgery? Int J Radiat Oncol Biol Phys 2011;81:1352-8. [Crossref] [PubMed]
- Riely GJ, Marks J, Pao W. KRAS mutations in non-small cell lung cancer. Proc Am Thorac Soc 2009;6:201-5. [Crossref] [PubMed]
- Lynch TJ, Bell DW, Sordella R, et al. Activating mutations in the epidermal growth factor receptor underlying responsiveness of non-small-cell lung cancer to gefitinib. N Engl J Med 2004;350:2129-39. [Crossref] [PubMed]
- Paez JG, Jänne PA, Lee JC, et al. EGFR mutations in lung cancer: correlation with clinical response to gefitinib therapy. Science 2004;304:1497-500. [Crossref] [PubMed]
- Pao W, Miller V, Zakowski M, et al. EGF receptor gene mutations are common in lung cancers from "never smokers" and are associated with sensitivity of tumors to gefitinib and erlotinib. Proc Natl Acad Sci U S A 2004;101:13306-11. [Crossref] [PubMed]
- Riely GJ, Ladanyi M. KRAS mutations: an old oncogene becomes a new predictive biomarker. J Mol Diagn 2008;10:493-5. [Crossref] [PubMed]
- Riely GJ, Kris MG, Rosenbaum D, et al. Frequency and distinctive spectrum of KRAS mutations in never smokers with lung adenocarcinoma. Clin Cancer Res 2008;14:5731-4. [Crossref] [PubMed]
- Brose MS, Volpe P, Feldman M, et al. BRAF and RAS mutations in human lung cancer and melanoma. Cancer Res 2002;62:6997-7000. [PubMed]
- Ostrem JM, Peters U, Sos ML, et al. K-Ras(G12C) inhibitors allosterically control GTP affinity and effector interactions. Nature 2013;503:548-51. [Crossref] [PubMed]
- Soda M, Choi YL, Enomoto M. Identification of the transforming EML4-ALK fusion gene in non-small-cell lung cancer. Nature 2007;448:561-6. [Crossref] [PubMed]
- Takeuchi K, Choi YL, Soda M, et al. Multiplex reverse transcription-PCR screening for EML4-ALK fusion transcripts. Clin Cancer Res 2008;14:6618-24. [Crossref] [PubMed]
- Koivunen JP, Mermel C, Zejnullahu K, et al. EML4-ALK fusion gene and efficacy of an ALK kinase inhibitor in lung cancer. Clin Cancer Res 2008;14:4275-83. [Crossref] [PubMed]
- Koh Y, Kim DW, Kim TM, et al. Clinicopathologic characteristics and outcomes of patients with anaplastic lymphoma kinase-positive advanced pulmonary adenocarcinoma: suggestion for an effective screening strategy for these tumors. J Thorac Oncol 2011;6:905-12. [Crossref] [PubMed]
- Inamura K, Takeuchi K, Togashi Y, et al. EML4-ALK fusion is linked to histological characteristics in a subset of lung cancers. J Thorac Oncol 2008;3:13-7. [Crossref] [PubMed]
- Inamura K, Takeuchi K, Togashi Y, et al. EML4-ALK lung cancers are characterized by rare other mutations, a TTF-1 cell lineage, an acinar histology, and young onset. Mod Pathol 2009;22:508-15. [Crossref] [PubMed]
- Kwak EL, Bang YJ, Camidge DR, et al. Anaplastic lymphoma kinase inhibition in non-small-cell lung cancer. N Engl J Med 2010;363:1693-703. [Crossref] [PubMed]
- Togashi Y, Soda M, Sakata S, et al. KLC1-ALK: a novel fusion in lung cancer identified using a formalin-fixed paraffin-embedded tissue only. PLoS One 2012;7:e31323. [Crossref] [PubMed]
- Solomon BJ, Mok T, Kim DW, et al. First-line crizotinib versus chemotherapy in ALK-positive lung cancer. N Engl J Med 2014;371:2167-77. [Crossref] [PubMed]
- Daum G, Eisenmann-Tappe I, Fries HW, et al. The ins and outs of Raf kinases. Trends Biochem Sci 1994;19:474-80. [Crossref] [PubMed]
- Cardarella S, Ogino A, Nishino M, et al. Clinical, pathologic, and biologic features associated with BRAF mutations in non-small cell lung cancer. Clin Cancer Res 2013;19:4532-40. [Crossref] [PubMed]
- Davies H, Bignell GR, Cox C, et al. Mutations of the BRAF gene in human cancer. Nature 2002;417:949-54. [Crossref] [PubMed]
- Naoki K, Chen TH, Richards WG, et al. Missense mutations of the BRAF gene in human lung adenocarcinoma. Cancer Res 2002;62:7001-3. [PubMed]
- Paik PK, Arcila ME, Fara M, et al. Clinical characteristics of patients with lung adenocarcinomas harboring BRAF mutations. J Clin Oncol 2011;29:2046-51. [Crossref] [PubMed]
- Pratilas CA, Hanrahan AJ, Halilovic E, et al. Genetic predictors of MEK dependence in non-small cell lung cancer. Cancer Res 2008;68:9375-83. [Crossref] [PubMed]
- Schreiber RD, Old LJ, Smyth MJ. Cancer immunoediting: integrating immunity's roles in cancer suppression and promotion. Science 2011;331:1565-70. [Crossref] [PubMed]
- Banchereau J, Steinman RM. Dendritic cells and the control of immunity. Nature 1998;392:245-52. [Crossref] [PubMed]
- Dieu-Nosjean MC, Antoine M, Danel C, et al. Long-term survival for patients with non-small-cell lung cancer with intratumoral lymphoid structures. J Clin Oncol 2008;26:4410-7. [Crossref] [PubMed]
- Zhuang X, Xia X, Wang C, et al. A high number of CD8+ T cells infiltrated in NSCLC tissues is associated with a favorable prognosis. Appl Immunohistochem Mol Morphol 2010;18:24-8. [Crossref] [PubMed]
- Villegas FR, Coca S, Villarrubia VG, et al. Prognostic significance of tumor infiltrating natural killer cells subset CD57 in patients with squamous cell lung cancer. Lung Cancer 2002;35:23-8. [Crossref] [PubMed]
- Hodi FS, O'Day SJ, McDermott DF, et al. Improved survival with ipilimumab in patients with metastatic melanoma. N Engl J Med 2010;363:711-23. [Crossref] [PubMed]
- Lynch TJ, Bondarenko I, Luft A, et al. Ipilimumab in combination with paclitaxel and carboplatin as first-line treatment in stage IIIB/IV non-small-cell lung cancer: results from a randomized, double-blind, multicenter phase II study. J Clin Oncol 2012;30:2046-54. [Crossref] [PubMed]
- Kazandjian D, Suzman DL, Blumenthal G, et al. FDA Approval Summary: Nivolumab for the treatment of metastatic non-small cell lung cancer with progression on or after platinum-based chemotherapy. Oncologist 2016;21:634-42. [Crossref] [PubMed]
- Borghaei H, Paz-Ares L, Horn L, et al. Nivolumab versus docetaxel in advanced nonsquamous non-small-cell lung cancer. N Engl J Med 2015;373:1627-39. [Crossref] [PubMed]
- Brystol-Myers Squibb. CheckMate-017, A phase 3 study of opdivo (nivolumab) compared to docetaxel in patients with second-line squamous cell non-small cell lung cancer, stopped early. Available online: http://news.bms.com/press-release/checkmate-017-phase-3-study-opdivo-nivolumab-compared-docetaxel-patients-second-line-s
- Rizvi NA, Garon EB, Patnaik A, et al. Safety and clinical activity of MK-3475 as initial therapy in patients with advanced non-small cell lung cancer (NSCLC). ASCO Annual Meeting Pro 2014;32:8007.
- Pardoll DM. The blockade of immune checkpoints in cancer immunotherapy. Nat Rev Cancer 2012;12:252-64. [Crossref] [PubMed]
- Wu YL, Liang J, Zhang W, et al. Immunotherapies: the blockade of inhibitory signals. Int J Biol Sci 2012;8:1420-30. [Crossref] [PubMed]
- Phan GQ, Yang JC, Sherry RM, et al. Cancer regression and autoimmunity induced by cytotoxic T lymphocyte-associated antigen 4 blockade in patients with metastatic melanoma. Proc Natl Acad Sci U S A 2003;100:8372-7. [Crossref] [PubMed]
- Topalian SL, Drake CG, Pardoll DM. Targeting the PD-1/B7-H1(PD-L1) pathway to activate anti-tumor immunity. Curr Opin Immunol 2012;24:207-12. [Crossref] [PubMed]
- Okazaki T, Honjo T. PD-1 and PD-1 ligands: from discovery to clinical application. Int Immunol 2007;19:813-24. [Crossref] [PubMed]
- Woo EY, Chu CS, Goletz TJ, et al. Regulatory CD4(+)CD25(+) T cells in tumors from patients with early-stage non-small cell lung cancer and late-stage ovarian cancer. Cancer Res 2001;61:4766-72. [PubMed]
- Woo EY, Yeh H, Chu CS, et al. Cutting edge: Regulatory T cells from lung cancer patients directly inhibit autologous T cell proliferation. J Immunol 2002;168:4272-6. [Crossref] [PubMed]
- Nemunaitis J, Dillman RO, Schwarzenberger PO, et al. Phase II study of belagenpumatucel-L, a transforming growth factor beta-2 antisense gene-modified allogeneic tumor cell vaccine in non-small-cell lung cancer. J Clin Oncol 2006;24:4721-30. [Crossref] [PubMed]
- Giaccone G, Bazhenova LA, Nemunaitis J, et al. A phase III study of belagenpumatucel-L, an allogeneic tumour cell vaccine, as maintenance therapy for non-small cell lung cancer. Eur J Cancer 2015;51:2321-9. [Crossref] [PubMed]
- Vansteenkiste J, Zielinski M, Linder A, et al. Final results of a multi-center, double-blind, randomized, placebo-controlled phase II study to assess the efficacy of MAGE-A3 immunotherapeutic as adjuvant therapy in stage IB/II non-small cell lung cancer (NSCLC). ASCO Annual Meeting Pro 2007;25:7554.
- Tyagi P, Mirakhur B. MAGRIT: the largest-ever phase III lung cancer trial aims to establish a novel tumor-specific approach to therapy. Clin Lung Cancer 2009;10:371-4. [Crossref] [PubMed]
- Butts C, Murray N, Maksymiuk A, et al. Randomized phase IIB trial of BLP25 liposome vaccine in stage IIIB and IV non-small-cell lung cancer. J Clin Oncol 2005;23:6674-81. [Crossref] [PubMed]
- Butts C, Maksymiuk A, Goss G, et al. Updated survival analysis in patients with stage IIIB or IV non-small-cell lung cancer receiving BLP25 liposome vaccine (L-BLP25): phase IIB randomized, multicenter, open-label trial. J Cancer Res Clin Oncol 2011;137:1337-42. [Crossref] [PubMed]
- Butts C, Socinski MA, Mitchell PL, et al. Tecemotide (L-BLP25) versus placebo after chemoradiotherapy for stage III non-small-cell lung cancer (START): a randomised, double-blind, phase 3 trial. Lancet Oncol 2014;15:59-68. [Crossref] [PubMed]
- Neninger Vinageras E, de la Torre A, et al. Osorio Rodríguez M, Phase II randomized controlled trial of an epidermal growth factor vaccine in advanced non-small-cell lung cancer. J Clin Oncol 2008;26:1452-8. [Crossref] [PubMed]
- Ramlau R, Quoix E, Rolski J, et al. A phase II study of Tg4010 (Mva-Muc1-Il2) in association with chemotherapy in patients with stage III/IV Non-small cell lung cancer. J Thorac Oncol 2008;3:735-44. [Crossref] [PubMed]
- Quoix E, Ramlau R, Westeel V, et al. Therapeutic vaccination with TG4010 and first-line chemotherapy in advanced non-small-cell lung cancer: a controlled phase 2B trial. Lancet Oncol 2011;12:1125-33. [Crossref] [PubMed]
- de Gruijl TD, van den Eertwegh AJ, Pinedo HM, et al. Whole-cell cancer vaccination: from autologous to allogeneic tumor- and dendritic cell-based vaccines. Cancer Immunol Immunother 2008;57:1569-77. [Crossref] [PubMed]
- Iyengar P, Gerber DE. Locally advanced lung cancer: an optimal setting for vaccines and other immunotherapies. Cancer J 2013;19:247-62. [Crossref] [PubMed]
- Rook AH, Kehrl JH, Wakefield LM, et al. Effects of transforming growth factor beta on the functions of natural killer cells: depressed cytolytic activity and blunting of interferon responsiveness. J Immunol 1986;136:3916-20. [PubMed]
- Kong F, Jirtle RL, Huang DH, et al. Plasma transforming growth factor-beta1 level before radiotherapy correlates with long term outcome of patients with lung carcinoma. Cancer 1999;86:1712-9. [Crossref] [PubMed]
- Linsley PS, Brady W, Grosmaire L, et al. Binding of the B cell activation antigen B7 to CD28 costimulates T cell proliferation and interleukin 2 mRNA accumulation. J Exp Med 1991;173:721-30. [Crossref] [PubMed]
- Vlad AM, Kettel JC, Alajez NM, et al. MUC1 immunobiology: from discovery to clinical applications. Adv Immunol 2004;82:249-93. [Crossref] [PubMed]
- Gonzalez G, Crombet T, Torres F, et al. Epidermal growth factor-based cancer vaccine for non-small-cell lung cancer therapy. Ann Oncol 2003;14:461-6. [Crossref] [PubMed]
- Trevor KT, Hersh EM, Brailey J, et al. Transduction of human dendritic cells with a recombinant modified vaccinia Ankara virus encoding MUC1 and IL-2. Cancer Immunol Immunother 2001;50:397-407. [Crossref] [PubMed]