Cathepsin detection to identify malignant cells during robotic pulmonary resection
Highlight box
Key findings
• Robotic-assisted thoracic surgery with intraoperative molecular imaging (RIMI) is a valuable tool for visualization of occult disease using VGT-309, a quenched-activity based probe, through identifying areas of increased cathepsin activity.
• RIMI and VIMI showed clinical equivalence in sensitivity and positive predictive value (PPV) of detecting cathepsin activity.
What is known and what is new?
• IMI allows the surgical oncologist to detect occult disease using a fluorescent probe. This has been applied in RIMI and VIMI.
• RIMI can detect cathepsin activity as a surrogate for malignancy and guide oncologic resection. It has shown equivalent sensitivity and PPV of to VIMI.
What is the implication, and what should change now?
• IMI may be used for its initial intended purpose of localizing disease, assessing margins, and identifying synchronous lesion together with quantifying tumor aggression.
• Future focus can work on further modifying this technology to quantify tumor characteristics based on fluorescence intensity.
Introduction
Robotic-assisted thoracic surgery (RATS) has had a wide adoption in the thoracic surgery community as it offers improved vision and wider degrees of freedom in instrument manipulation (1,2). It has a similar safety profile and oncologic outcomes to video-assisted thoracic surgeries (VATS) and the more traditional open surgeries (3,4). However, a limitation it shares with VATS is the lack of tactile sensation. Parenchymal tumors that are not clearly visible become a more serious concern when tactile sensation is lost and necessitate a non-tactile optical scanner. Our group has been investigating the use of intraoperative molecular imaging (IMI) to meet this need.
IMI is a concept whereby patients are infused with a fluorescent probe the day of or the day before surgery. They then undergo standard of care surgery in addition to using a near-infrared (NIR) laser. The laser excites the fluorescent probe and a camera then detects the fluorescence emitted and localizes the target tissue. There are currently a few fluorescent probes and several imaging devices in the market (5). Most of the efforts have focused on developing contrast agents that have minimal non-specific fluorescence and are only activated in specific tumor microenvironments.
A promising novel probe is VGT-309 which is a quenched activity-based probe (qABPs) (6). This fluorescent probe is activated by cathepsins, enzymes that are overexpressed in cancer cells (7). Essentially, this unique contrast agent is an indirect measure of cathepsin activity. In other words, areas that fluoresce are areas that have increased cathepsin activity. These enzymes are overly expressed in almost all solid tumors and have been the target of cancer therapies (8,9). This helps to minimize the resection of normal parenchyma surrounding the tumor. However, it is important to note that other non-cancerous inflammatory processes might also cause an increased cathepsin expression and could potentially be a cause of a false positive (10).
The parent molecule of VGT-309 is BMV109 (11). The main difference is that the fluorophore used with VGT-309 is indocyanine green (ICG). ICG has a maximum excitation at 789 nm and emission at 814 nm. Thus, this modification is quite advantageous as it can be detected using widely available and FDA-approved surgical imaging cameras from companies such as Intuitive, Stryker, Olympus, Karl Storz, Medtronic, and Johnson and Johnson. Furthermore, the NIR technology allows for better depth of penetration and detection of tumors that tend to be further below the surface. Initially, a phase 2 safety randomized, double-blind, placebo-controlled, single ascending dose study was performed in healthy subjects in Australia (anzctr.org.au; registration number ACTRN12621000301864) (7). This was performed to evaluate the safety, tolerability, and pharmacokinetics of VGT-309. Recently, our group completed a single center phase 2 study for patients with suspected cancer in the lungs (ClinicalTrials.gov Identifier: NCT05400226) which showed promising results in using VGT-309 with NIR light to localize lesions not seen under white light, identification of synchronous lesions, and identifying positive or close margins. It also appears to be safe and well tolerated in this patient population.
While the earlier applications of VGT-309 focused on video-assisted thoracic surgery (VATS) with intraoperative molecular imaging (VIMI), we wanted to evaluate its application during robotic-assisted thoracic surgery with intraoperative molecular imaging (RIMI). This is the first study that compares both VATS and robotic detection of increased enzymatic activity using NIR light as a guide for tumor localization and resection. Our objective is to measure the sensitivity and positive predictive value (PPV) of RIMI to detect cathepsin activity by localizing areas that have been targeted by VGT-309. A second objective was to compare RIMI to VIMI. We present this article in accordance with the TREND reporting checklist (available at https://tlcr.amegroups.com/article/view/10.21037/tlcr-23-370/rc).
Methods
Patient selection
Our study is part of a completed phase 2 clinical trial (NCT05400226) that began in June 2022 (patients included up to June 2023) at The Hospital of the University of Pennsylvania. The study was conducted in accordance with the Declaration of Helsinki (as revised in 2013). It was approved by the institutional ethics board of The University of Pennsylvania (IRB Approval Number: 822153) and informed consent was obtained from all individual participants.
Patients included in our study had a T1 (<3 cm) pulmonary lesion suspicious for malignancy who underwent surgery using RATS. Patients provided informed consent and underwent computed tomographic (CT) scanning with 1-mm slice thickness and standard evaluations at the discretion of the surgeon [e.g., positron emission tomography (PET), pulmonary function tests (PFT), biopsy]. Baseline characteristics such as age, sex (male or female), body mass index (kg/m2), lesion size, distance of tumor from the pleural surface, and PET avidity in standardized uptake value (SUV) were collected. Exclusion criteria included people under the age of 18, people unable to give informed consent, non-English-speaking people, and patients with prior chest surgery.
Study design
Study design and study size were based on consensus guidelines provided by the World Molecular Imaging Society (12). All subjects were scheduled for minimally invasive RATS. On the day prior to resection, study participants received 0.32 mg/kg (12 mg/m2) VGT-309 intravenously over 15–20 minutes at the infusion center of the Hospital of the University of Pennsylvania by trained nurses. The 24-hour timeframe and optimal dose has been validated using a phase II Australian trial registered with the Australian New Zealand Clinical Trials Registry (anzctr.org.au; registration number ACTRN12620000948998), approved by the Alfred Human Research Ethics Committee, and performed at the Centre for Clinical Studies, Nucleus Network, Melbourne, Victoria, Australia.
Intraoperatively, patients underwent the previously described RIMI technique (13). First, a white-light robotic scope was utilized to examine the thoracic cavity and identify the tumor in question. Secondly, the surgeon activated the da Vinci Xi FireFly sensitive mode that can detect the wavelength of ICG, the fluorophore associated with VGT-309. After the lesion was identified using IMI, the FireFly mode is turned off. Thirdly, the VATS VisonSense thoracoscope (Iridium Medtronic, Minneapolis, MN, USA) was advanced through the assistant port to visualize that same lesion (14). The Iridium can also see NIR tracers such as ICG. Finally, the resected specimen was evaluated on the back table using an exoscope that can detect VGT-309 (Medtronic, Minneapolis, MN, USA) and then submitted for histopathologic evaluation. The primary objective of our study was to assess the sensitivity and PPV of robotic detection of cathepsin activity by detecting the targeted fluorescence of VGT-309. The secondary objective was to compare RIMI to VIMI in terms of identifying occult disease using VGT-309.
Study drug
VGT-309 (chemical formula C127H142ClF4N10Na3O23S5; molecular weight, 2,517.29 Da) is a qABP that consists of a phenoxymethyl ketone electrophile that covalently and irreversibly binds active cysteine cathepsins, coupled to an ICG fluorophore (excitation peak =789 nm, emission peak =814 nm) and a IRDye QC-1 quencher (LI-COR Biosciences, Lincoln, NE, USA). Cathepsin binds to the molecule, causing cleavage of the quencher and allowing the fluorescence of the ICG fluorophore to be detected by NIR imaging systems. Vials containing 11 mg of lyophilized VGT-309 drug product were reconstituted with water for injection to a final concentration of 5 mg/mL and diluted with Ringer’s lactate solution.
Imaging devices
The VS3 Iridium High-definition system (Medtronic, Minneapolis, MN, USA) was used as our gold standard for IMI. It is designed to detect ICG and is comprised of a camera having an excitation wavelength of 805 nm and emission band between 825 and 860 nm. It is also compatible with the standard VATS 5-mm, 0-degree thoracoscope. VS3 Iridium is a dual band system that allows to capture both white light in parallel with NIR light and displays both to the surgeon simultaneously. It served as our gold standard for IMI against which we compared RIMI.
The da Vinci Xi Surgical System, which is equipped with a fully integrated NIR imaging system termed Firefly Fluorescence Imaging, was used for RATS and RIMI. Firefly provides visualization in two modes: standard, visible-light imaging mode and a NIR fluorescence imaging mode, consisting of either a black-and-white surgical image (i.e., Standard Firefly mode) or black background image with the NIR fluorescence displayed in green (i.e., Sensitive Firefly mode). Sensitive Firefly mode has higher sensitivity for NIR signal compared to Standard Firefly mode and was used to detect fluorescence from VGT-309. The Firefly mode provides real-time NIR fluorescence imaging with λexcitation at ~805 nm and λimaging at ~830 nm.
For ex vivo evaluation, an exoscope on the VS3 Iridium system was used.
Post-resection specimen analysis
After examination on the back table, the tumors were sent for formal histopathological evaluation. They were formalin fixed and paraffin embedded. Sequential 5-µm sections were obtained and underwent histopathologic and fluorescent analysis by a board-certified thoracic pathologist. Sections were stained using standard hematoxylin and eosin (H&E) staining and cathepsins B/L/S staining. An additional unstained 5-µm section was evaluated using the Odyssey Imaging System (LI-COR Biosciences) and a fluorescence microscope (Leica Microsystems, Wetzlar, Germany). Regions of fluorescence were correlated to the H&E and cathepsin-stained specimens.
Post hoc image analysis
ImageJ (http://rsb.info.nih.gov/ij) was used for post hoc image analysis. Fluorescence was quantified via mean fluorescence intensity (MFI) by analyzing monochromatic NIR images and measuring regions of interest. Background fluorescence (0.5–1.0 cm from tumor) was also obtained (MFIbackground). Calculations were repeated in triplicate, and tumor-to-background ratios (TBR) were calculated using the following equation (MFIlesion/MFIbackground).
Statistical analysis
The data was analyzed using IBM SPSS Statistics software version 28 (IBM Corp., Armonk, NY, USA). Means were compared using the independent student t-tests. A P value of 0.05 or less was considered statistically significant. Imaging modalities were compared by computing the sensitivity and PPV.
Results
Patient and lesion characteristics
Between June 2022 and June 2023, 10 subjects with suspicious pulmonary nodules underwent RATS resection with VGT-309 based RIMI followed by VGT-309 based VIMI. All patients had received the full dose of the fluorescent probe, tolerated it well, and reported no drug-associated adverse events. Two subjects were males and eight females. The mean age was 68.8 years [standard deviation (SD) =10.5]. All patients underwent standard preoperative imaging. Tumors ranged in size between 1 to 2.6 cm with a mean of 1.71 cm (SD =0.49). The tumors were mostly superficial with most located on the pleural surface (3/10) and the deepest being 1.6 cm away. The mean distance from the pleural distance was 0.5 cm (SD =0.63). Tumor histologies included invasive adenocarcinoma, mucinous adenocarcinoma, adenocarcinoma in situ neuroendocrine tumors, and benign fibrosis. Individual patient characteristics are summarized in Table 1.
Table 1
Subject | Age, years | Sex | Lesion size* (cm) | Depth from pleural surface (cm) | SUV | Location | Visualized by white light | Visualized by VIMI | Visualized by RIMI | Diagnosis | Operation performed |
---|---|---|---|---|---|---|---|---|---|---|---|
1 | 73 | F | 1.3 | 0 | 6.3 | LUL | Yes | Yes | Yes | Invasive adenocarcinoma | Wedge resection |
2 | 55 | F | 1 | 0 | 5.4 | LLL | Yes | No | No | Neuroendocrine | Lobectomy |
3 | 53 | F | 2 | 1.5 | 0 | LUL | Yes | No | No | Fibrotic tissue | Wedge resection |
4 | 77 | F | 1.3 | 0 | 0 | LLL | Yes | Yes | Yes | Mucinous adenocarcinoma | Lobectomy |
5 | 86 | F | 2.6 | 0.4 | 2.1 | RUL | No | Yes | Yes | Invasive Adenocarcinoma | Lobectomy |
6 | 70 | M | 1.7 | 0.1 | 1.7 | RLL | Yes | Yes | Yes | Adenocarcinoma in situ | Segmentectomy |
7 | 73 | M | 2.3 | 0.3 | 9.1 | LLL | Yes | Yes | Yes | Invasive Adenocarcinoma | Lobectomy |
8 | 65 | F | 1.4 | 1 | 13.7 | LLL | No | Yes | Yes | Neuroendocrine | Lobectomy |
9 | 60 | F | 1.8 | 1.6 | 0.9 | Right middle lobe | No | Yes | Yes | Invasive Adenocarcinoma | Lobectomy |
10 | 76 | F | 1.7 | 0.1 | 0 | RLL | No | Yes | Yes | Invasive adenocarcinoma | Segmentectomy |
*, size is based on the greatest dimension. SUV, standardized uptake value; VIMI, video-assisted thoracic surgery with intraoperative molecular imaging; RIMI, robotic-assisted thoracic surgery with intraoperative molecular imaging; RUL, right upper lobe; RLL, right lower lobe; LLL, left lower lobe; LUL, left upper lobe.
Lesion localization in vivo using RATS and RIMI
Initially, the surgeon used the Intuitive X/Xi Endoscope Plus to inspect the thoracic cavity using white light. Six out of 10 (60%) tumors were visualized by a morphologic distortion of the lung parenchyma. The other 4 appeared to have normal lung parenchyma. The sensitivity of RATS in detecting pulmonary nodules was 55.6% and PPV in detecting malignancy was 83.3%. There were no statically significant differences between the baseline characteristics of those who were visualized under white light compared to those who were not (Table 2).
Table 2
Characteristic | RATS | P value | |
---|---|---|---|
No (n=6) | Yes (n=4) | ||
Age (years) | 66.83 | 71.75 | 0.5 |
Sex | * | ||
Male | 2 | 0 | |
Female | 4 | 4 | |
Size (cm) | 1.87 | 1.60 | 0.4 |
Depth (cm) | 0.78 | 0.32 | 0.3 |
SUV | 3.75 | 3.75 | 0.9 |
*, could not perform Chi-squared test as had one value of 0. RATS, robotic-assisted thoracic surgery; SUV, standardized uptake value.
Following white light inspection, the surgeon switched to the da Vinci “Sensitive Firefly” mode for IMI. RIMI detected cathepsin activity in 8/10 (80%) tumors marked with fluorescence. It detected cathepsin activity in all 4 nodules that were not visualized under white light. The sensitivity of RIMI was 88.9% and PPV was 100%. The nodules that were not visualized by RIMI included one with benign fibrosis and one with carcinoid morphology (Figure 1).
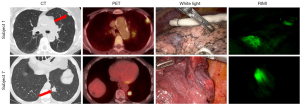
VIMI detects tumors during pulmonary resection
VIMI has been demonstrated to detect the ICG fluorophore associated with VGT-309 (7). Hence, after patients underwent RATS followed by RIMI, they were imaged with an Iridium Visionsense (Medtronic, Minneapolis, MN, USA) thoracoscope through the assistant port. The VIMI was able to detect 8/10 (80%) of the total nodules and all 4 of the nodules not detected by white light. The two histologies that were not visualized by VIMI were the same as those not visualized using RIMI: benign fibrosis and neuroendocrine. The addition of VIMI did not identify any lesions that RIMI may have missed. The sensitivity of VIMI was 88.9% and PPV was 100%.
Analysis of resected specimens
To further evaluate the fluorescent signal after resection, all specimens were imaged using a NIR exoscope designed to detect VGT-309 both as intact and once bisected. On back-table analysis, the mean signal-to-background ratio (SBR) of tumors was 2.40 (SD =0.9; range, 1.8–4.1) when intact and 3.69 (SD =2.11; range, 1.93–8.06) when bisected (Figure 2).
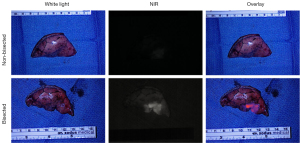
The resected specimen was then histologically analyzed. Five (50%) were invasive adenocarcinoma, two were neuroendocrine, one was mucinous adenocarcinoma, one was fibrotic tissue, and one was adenocarcinoma in situ. The slides were then sent for cathepsin staining. Tumors had the highest intensity of fluorescence under fluorescent microscopy. These areas directly correlated with areas that had a higher cathepsin expression as seen on IHC (Figure 3).
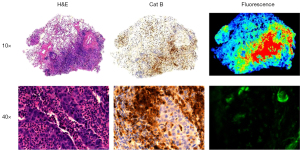
RIMI compared to VIMI
In our study, we considered VIMI as our gold standard. The MFI of tumors visualized by RIMI was 115.81 A.U. (SD =58.57) compared to 95.6 A.U. (SD =14.81) by VIMI. (P=0.41). The mean TBR of tumors visualized by RIMI was 9.20 (SD =9.12) compared to 2.29 A.U. (SD =1.11) using VIMI (P=0.1). Furthermore, both RIMI and VIMI were able to detect the same 8/10 (80%) nodules and all 4 nodules not detected by white light. There were also no statistical significance between the mean MFIs or mean TBRs when comparing VIMI and RIMI. RIMI, however, had more widespread values compared to VIMI, evident with the higher standard deviations in MFI and TBRs (Figure 4). RIMI and VIMI had the same sensitivities (88.9%) and PPV (100%) in detecting cathepsin activity. In summary, RIMI compared favorably to VIMI in detecting cathepsin activity and localizing disease.
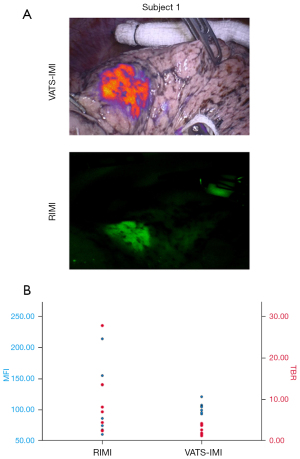
Discussion
Lung cancers have shifted from large central tumors to smaller peripheral lesions over time (15). Furthermore, more surgeons are switching to more minimally invasive approaches, where tactile sensation is limited or non-existent. As such, tumor visualization is increasingly becoming one of the most critical steps enabling an R0 resection. Currently, intraoperative frozen section is used to help surgeons decide the extent of the needed dissection. However, it adds to the overall operative time as it requires sending the specimen to pathology, processing it, and then calling the surgeon back. Also, there is a risk for a false negative if the foci of tumors are undetected on frozen and only confirmed on permanent, which might have altered the surgeons’ decision making. To address the previous concerns, IMI uses a fluorescent probe that helps localize the tumor in question in real time. It has had promising results in VIMI and RIMI in localizing occult disease (13).
Over the past decade, a few fluorescent probes have been introduced to the market such as ICG and a folate-specific fluorescent probe while probes that get activated within a specific pH or qABP such as VGT-309 are in clinical development. A cathepsin-targeted qABP such as VGT-309 can have a better selectivity to tumors, since tumors have a heightened cathepsin expression, thereby decreasing background fluorescence in non-malignant tissue (5). An additional advantage to the VGT-309 molecule is that the fluorophore is ICG, which can be detected by widely-available NIR cameras used in most hospital systems. Fluorescent probes may also allow for less operative time by identifying tumors more rapidly. It also avoids the complications associated with traditional techniques that use transthoracic localization such as pneumothoraxes and avoids the needs for an additional procedure (16). Finally, as a qABP, VGT-309 only gets activated when it encounters the increased enzymatic activity of cathepsin. In our study, we present a novel concept in IMI that focuses on identifying increased enzymatic activity in tumor cells to localize them. While enzymatic activity had been a staple of therapeutic options in cancer treatment, it has only recently been applied in the realm of imaging (17). The fluorescent probe VGT-309 is a qABPs that is activated in the presence of cathepsins (7). In our study, we have shown that areas that fluoresce on VIMI and RIMI correspond to tumor and have an increased cathepsin activity. We have also established that RIMI compares favorably to VIMI in detecting occult disease and guiding oncologic thoracic surgery.
Thoracic surgeons always strive their best to achieve a R0 resection as it is associated with a better prognosis, decreased recurrence and improved overall survival (18). An analysis of the National Cancer Database (NCDB) between 2004 to 2011 of patients who had non-small cell lung cancer showed that there were roughly 5% of patients who had a positive margin after surgery with 57% having an R1 (microscopic) margin (19). IMI helps to address the R1 margins by fluorescently labelling lesions, providing additional information to the surgeon during margin assessment. This was the case in 4 lesions in our study. These patients all underwent R0 resections and benefited from a more parenchymal-sparing wedge resections as opposed to more extensive surgery.
Among the 10 patients examined, RIMI was able to detect 8 out of the 10 lesions. RIMI allowed for visualization of subpleural lesions ranging from 0.1 to 1.4 cm. This is particularly important as smaller and subpleural lesions are more likely to be missed under white light if there is no associated contour distortion of the lung parenchyma. Identification of subpleural and small lesions may allow for a more conservative surgical approach in the setting of the mounting evidence to suggest that sublobar resection is equivalent to formal lobectomy in early-stage lung cancer (20). Furthermore, VGT-309 was able to localize lesions in different lobes of the lung and hence was location agnostic.
The only two lesions that did not fluoresce on RIMI were histologically benign fibrosis and carcinoid. Benign fibrosis is a non-malignant condition and hence expectedly a true negative. Carcinoid tumors vary in degree of cathepsin expression and while some fluoresce, some do not. One factor that might have affected fluorescence in this patient was her being a current smoker and having a 40 pack-year smoking history. There is evidence that smoking induces anthracosis in lungs and can prevent fluorescent signal transmission (21). However, while we expect to see these anthracitic changes in smokers, sometimes that may elicit a more pronounced signal in the lesion compared to background autofluorescence. In other words, while the VGT-309 signal might be slightly dampened, the signal from the background will be more significantly dampened, thereby increasing the TBR.
Aside from the benefit of using RIMI to identify lesions, robotic surgery may offer other advantages. There have been many studies comparing RATS and VATS to open lung surgery. Most studies have shown the superiority of minimally invasive approaches in terms of decreased operative stay and postoperative pain (22). However, it has some disadvantages. First, robotic surgery is associated with an increased cost (23). Second, RATS was associated with an increased operative time, which was institution and surgeon dependent. Meanwhile, one study showed that the median operating time using RATS was lower than VATS (24). These two limitations may be addressed by the wider adoption of robotic surgery early on in surgical training to familiarize surgeons with this technology and improve operative time. Also, it has been shown that consumables are a major attributing cost to RATS and reducing them can also reduce cost (24).
The study does have some limitations. On one hand, we have a small sample size of 10. As such, we recommend replicating this study on a larger cohort to improve the power of this study. Also, the tumor histologies that were studied were heterogenous (6 adenocarcinoma, 2 neuroendocrine, 1 benign/fibrosis, and 1 mucinous adenocarcinoma). It was hence more challenging to do a subgroup analysis with a smaller number per cohort, further bringing up the need for a future study with a bigger cohort. Lastly, deeper tissue penetrance remains a concern with fluorescent probes. While in this study, the sample size is not large enough to address this question, it is something that needs to be further studies in larger scale studies and compared to other imaging modalities.
Conclusions
There is an abundance in the literature about the use and the value of IMI in oncologic resections (25). In our study we present a proof of concept of not only using this technology to localize occult lesions but also potentially using it to quantify enzymatic expression. Hence, this opens a potential new era of using IMI for its initial intended purpose of localizing disease, assessing margins, and identifying synchronous lesion together with quantifying tumor aggression. There is potential for using this technology not only for primary pulmonary malignancies, but also to identify tumors that have metastasized to the lung especially if the tumors have an increased cathepsin expression. It may also help in localizing lesions for targeted biopsies, localizing positive lymph nodes, and localizing other primary malignancies in the chest such as esophageal or tracheal cancer.
Acknowledgments
Funding: The clinical trial reported in this publication (ClinicalTrials.gov Identifier: NCT05400226) was supported by
Footnote
Reporting Checklist: The authors have completed the TREND reporting checklist. Available at https://tlcr.amegroups.com/article/view/10.21037/tlcr-23-370/rc
Data Sharing Statement: Available at https://tlcr.amegroups.com/article/view/10.21037/tlcr-23-370/dss
Peer Review File: Available at https://tlcr.amegroups.com/article/view/10.21037/tlcr-23-370/prf
Conflicts of Interest: All authors have completed the ICMJE uniform disclosure form (available at https://tlcr.amegroups.com/article/view/10.21037/tlcr-23-370/coif). J.T.S. Jr and E.S.B. report that they receive salary and stock options as full-time employees of the company. The company also provided study drug (VGT-309) and funding for the clinical trial at the University of Pennsylvania where the data in the manuscript were generated. Vergent Bioscience is the awardee of SBIR grant R44CA277890, which supports the clinical trial at the University of Pennsylvania where the data in the manuscript were generated. As employees of the company, Vergent pays expenses for J.T.S. Jr and E.S.B. to travel to and attend scientific meetings. They are inventor on a pending patent application owned by Vergent. As employees of the company, they have stock options in the company. They are executives of the company and receives a salary as a full-time employee. The other authors have no conflicts of interest to declare.
Ethical Statement: The authors are accountable for all aspects of the work in ensuring that questions related to the accuracy or integrity of any part of the work are appropriately investigated and resolved. The study was conducted in accordance with the Declaration of Helsinki (as revised in 2013). The study was approved by the institutional ethics board of The University of Pennsylvania (IRB Approval Number: 822153) and informed consent was obtained from all individual participants.
Open Access Statement: This is an Open Access article distributed in accordance with the Creative Commons Attribution-NonCommercial-NoDerivs 4.0 International License (CC BY-NC-ND 4.0), which permits the non-commercial replication and distribution of the article with the strict proviso that no changes or edits are made and the original work is properly cited (including links to both the formal publication through the relevant DOI and the license). See: https://creativecommons.org/licenses/by-nc-nd/4.0/.
References
- Wilensky GR. Robotic Surgery: An Example of When Newer Is Not Always Better but Clearly More Expensive. Milbank Q 2016;94:43-6. [Crossref] [PubMed]
- Nasir BS, Bryant AS, Minnich DJ, et al. Performing robotic lobectomy and segmentectomy: cost, profitability, and outcomes. Ann Thorac Surg 2014;98:203-8; discussion 208-9. [Crossref] [PubMed]
- Lee BE, Korst RJ, Kletsman E, et al. Transitioning from video-assisted thoracic surgical lobectomy to robotics for lung cancer: are there outcomes advantages? J Thorac Cardiovasc Surg 2014;147:724-9. [Crossref] [PubMed]
- Gharagozloo F, Margolis M, Tempesta B, et al. Robotassisted lobectomy for early-stage lung cancer: report of 100 consecutive cases. Ann Thorac Surg 2009;88:380-4. [Crossref] [PubMed]
- Bou-Samra P, Muhammad N, Chang A, et al. Intraoperative molecular imaging: 3rd biennial clinical trials update. J Biomed Opt 2023;28:050901. [Crossref] [PubMed]
- Suurs FV, Qiu SQ, Yim JJ, et al. Fluorescent image-guided surgery in breast cancer by intravenous application of a quenched fluorescence activity-based probe for cysteine cathepsins in a syngeneic mouse model. EJNMMI Res 2020;10:111. [Crossref] [PubMed]
- Kennedy GT, Holt DE, Azari FS, et al. A CathepsinTargeted Quenched Activity-Based Probe Facilitates Enhanced Detection of Human Tumors during Resection. Clin Cancer Res 2022;28:3729-41. [Crossref] [PubMed]
- Olson OC, Joyce JA. Cysteine cathepsin proteases: regulators of cancer progression and therapeutic response. Nat Rev Cancer 2015;15:712-29. [Crossref] [PubMed]
- Palermo C, Joyce JA. Cysteine cathepsin proteases as pharmacological targets in cancer. Trends Pharmacol Sci 2008;29:22-8. [Crossref] [PubMed]
- Hannaford J, Guo H, Chen X. Involvement of cathepsins B and L in inflammation and cholesterol trafficking protein NPC2 secretion in macrophages. Obesity (Silver Spring) 2013;21:1586-95. [Crossref] [PubMed]
- Verdoes M, Oresic Bender K, Segal E, et al. Improved quenched fluorescent probe for imaging of cysteine cathepsin activity. J Am Chem Soc 2013;135:14726-30. [Crossref] [PubMed]
- Tummers WS, Warram JM, Tipirneni KE, et al. Regulatory Aspects of Optical Methods and Exogenous Targets for Cancer Detection. Cancer Res 2017;77:2197-206. [Crossref] [PubMed]
- Kennedy GT, Azari FS, Bernstein E, et al. First-inhuman results of targeted intraoperative molecular imaging for visualization of ground glass opacities during robotic pulmonary resection. Transl Lung Cancer Res 2022;11:1567-77. [Crossref] [PubMed]
- Predina JD, Newton AD, Keating J, et al. A Phase I Clinical Trial of Targeted Intraoperative Molecular Imaging for Pulmonary Adenocarcinomas. Ann Thorac Surg 2018;105:901-8. [Crossref] [PubMed]
- Wong MKH, Sit AKY, Au TWK. Minimally invasive thoracic surgery: beyond surgical access. J Thorac Dis 2018;10:S1884-91. [Crossref] [PubMed]
- Awais O, Reidy MR, Mehta K, et al. Electromagnetic Navigation Bronchoscopy-Guided Dye Marking for Thoracoscopic Resection of Pulmonary Nodules. Ann Thorac Surg 2016;102:223-9. [Crossref] [PubMed]
- Smith BL, Gadd MA, Lanahan CR, et al. Real-time, intraoperative detection of residual breast cancer in lumpectomy cavity walls using a novel cathepsin-activated fluorescent imaging system. Breast Cancer Res Treat 2018;171:413-20. [Crossref] [PubMed]
- Ettinger DS, Wood DE, Aisner DL, et al. NCCN Guidelines Insights: Non-Small Cell Lung Cancer, Version 2.2021. J Natl Compr Canc Netw 2021;19:254-66. [Crossref] [PubMed]
- Osarogiagbon RU, Lin CC, Smeltzer MP, et al. Prevalence, Prognostic Implications, and Survival Modulators of Incompletely Resected Non-Small Cell Lung Cancer in the U.S. National Cancer Data Base. J Thorac Oncol 2016;11:e5-16. [Crossref] [PubMed]
- Altorki NK, Yip R, Hanaoka T, et al. Sublobar resection is equivalent to lobectomy for clinical stage 1A lung cancer in solid nodules. J Thorac Cardiovasc Surg 2014;147:754-62; Discussion 762-4. [Crossref] [PubMed]
- Azari F, Kennedy G, Zhang K, et al. Effects of Lightabsorbing Carbons in Intraoperative Molecular ImagingGuided Lung Cancer Resections. Mol Imaging Biol 2023;25:156-67. [Crossref] [PubMed]
- Louie BE, Wilson JL, Kim S, et al. Comparison of VideoAssisted Thoracoscopic Surgery and Robotic Approaches for Clinical Stage I and Stage II Non-Small Cell Lung Cancer Using The Society of Thoracic Surgeons Database. Ann Thorac Surg 2016;102:917-24. [Crossref] [PubMed]
- Deen SA, Wilson JL, Wilshire CL, et al. Defining the cost of care for lobectomy and segmentectomy: a comparison of open, video-assisted thoracoscopic, and robotic approaches. Ann Thorac Surg 2014;97:1000-7. [Crossref] [PubMed]
- Novellis P, Bottoni E, Voulaz E, et al. Robotic surgery, video-assisted thoracic surgery, and open surgery for early stage lung cancer: comparison of costs and outcomes at a single institute. J Thorac Dis 2018;10:790-8. [Crossref] [PubMed]
- Azari F, Kennedy G, Bernstein E, et al. Intraoperative molecular imaging clinical trials: a review of 2020 conference proceedings. J Biomed Opt 2021;26:050901. [Crossref] [PubMed]