Does subtyping of high-grade pulmonary neuroendocrine carcinomas have an impact on therapy selection?
Highlight box
Key findings
• ASCL1, NeuroD1, and POU2F3 subtypes were identified in small cell lung cancer (SCLC). Similar subtypes were identified in large cell neuroendocrine carcinomas (LCNEC). YAP1 did not fulfil the criteria of a separate subtype. ATOH although expressed in many cases does not justify a separate subtype.
What is known and what is new?
• Subtypes of SCLC were already defined. Within LCNEC two types are known with different responses to either NSCLC-based chemotherapy of a schema used for SCLC. Few reports have focused on C-MYC, or not specified the MYC type. Fibroblast growth factor receptors have been identified in SCLC, however their frequency and the involvement were not investigated. AURKA was seen in SCLC and a clinical study is ongoing. No biomarker protocol is done.
• ASCL1, NeuroD1, and POU2F3 subtypes were identified in LCNEC. All three forms of MYC were investigated, surprisingly N-MYC was the most common form in SCLC and LCNEC. We also demonstrated their heterogeneity in expression intensity. Whereas FGFR1 was not identified, FGFR2 might be a target for treatment. The frequency of AURKA expression in SCLC and LCNEC has not been investigated. Here we show, that this enzyme is frequently expressed in SCLC and LCNEC.
What is the implication, and what should change now?
• AURKA testing should be implemented, if anti-AURKA treatment is planned. The expression level should be correlated with response. Combined chemo- and immunotherapy should be decided based on PD-L1 staining of stroma cells in SCLC and LCNEC.
Introduction
High-grade neuroendocrine lung carcinomas are divided into small cell lung cancer (SCLC) and large cell neuroendocrine carcinoma (LCNEC). They are very aggressive tumor types with limited therapeutic options and poor prognosis. For decades the therapy protocol for SCLC did not change. For LCNEC treatment some reports proposed SCLC chemotherapy protocols as the best option (1-4). However, based on the recent molecular profiling data LCNEC can be classified as SCLC-like and NSCLC (non-small cell lung cancer)-like. It is still unclear if therapy protocols should be adjusted accordingly (1,3).
The histologic diagnosis of SCLC can be made purely by morphology. It can be confirmed by a positive reaction for neuroendocrine markers [chromogranin A, synaptophysin, neural cell adhesion molecule (NCAM)/CD56, insulinoma-associated protein 1 (INSM1)]. Furthermore, low-molecular-weight cytokeratin antibodies show a typical, so-called “caping”, staining reaction, which is due to a polar accumulation of intermediate filaments. A high proportion of SCLC aberrantly express the second isoform of TTF-1 (5). The mitotic rate is high, which is visible in frozen sections, but not easily seen in formalin-fixed tissues. Staining with Ki67 will show a high proliferation rate in fixed specimens. Few SCLC cases do not express neuroendocrine markers and some of them are even negative for cytokeratin, and instead express vimentin (6,7).
Recently, four subtypes of SCLC were identified in cell cultures (8). Two of these subtypes are characterized by the high expression of neuroendocrine master genes achaete scute family BHLH transcription factor 1 (ASCL1) and NeuroD1. The third subtype expresses POU class 2 homeobox 3 (POU2F3), which is a gene expressed in tuft cells in the lung periphery (9). The expression of neuroendocrine markers such as chromogranin A, synaptophysin, and NCAM is predominantly found in the ASCL1 and NeuroD1 subtypes, whereas the POU2F3 group is usually negative for these markers. The fourth subtype was defined by the expression of YAP1. YAP1 is often amplified or overexpressed in NSCLC and might suppress a neuroendocrine profile (10). It is an important regulator of tumor cell migration and regulator of the epithelial-to-mesenchymal transition (EMT) (11). The role of YAP1 as a gene characterizing the fourth subtype of SCLC was questioned by recent publications using immunohistochemistry (12,13). Furthermore, Gay et al. (14) in their analysis of 4 subtypes, confirmed the existence of ASCL1, NeuroD1, and POU2F3 subtypes, and identified an additional subtype, which they called SCLC-inflamed (SCLC-I). This subtype had lower expression of ASCL1, NeuroD1, and POU2F3, but had high expression of genes for human leukocyte antigens and immune checkpoints, such as programmed cell death ligand 1 (PD-L1) (“inflamed gene signature”). This is also one of the rare papers analyzing the therapeutic relevance of those 4 different subtypes. In cell lines and tumor xenografts, the POU2F3 (15) subtype turned out to be very sensitive to cisplatin, whereas SCLC-I was resistant. Furthermore, the SCLC-I population emerges during cisplatin chemotherapy. Using publicly available data from the Impower133 trial (16) they also showed that SCLC-I tumors have greater benefits from immune checkpoint inhibitor therapy. Some authors proposed a treatment response for DLL3 and BCL2 inhibitors in the ASCL1 group (17,18), and the response for Aurora kinase inhibitors in the NeuroD1 group was suggested by Mollaoglu et al. (19).
LCNEC expresses neuroendocrine markers, but often in a lower percentage of tumor cells, and the cytokeratin reaction is present on the entire circumference of the tumor cells. In contrast to SCLC, in addition to neuroendocrine morphology, at least one neuroendocrine marker must be positive for the diagnosis of LCNEC (WHO, Classification of Thoracic Tumors) (20). On the molecular level, LCNEC is also more heterogeneous. Some cases of LCNEC present with mutations similar to SCLC such as alterations in MYCL1 and MYC genes (21,22), and others with mutations found in NSCLC like alterations in FGFR1 and NKX2-1 genes (15).
In LCNEC two variants were identified with losses of either RB1 or PTEN, respectively, or alternatively alterations of STK11/KEAP1 and/or PI3KCA (21,22). These subtypes might explain different treatment responses for LCNEC: those with RB1-loss might respond better to a SCLC-like regime, whereas the other subtype might respond better to a NSCLC-like regime (21,23).
Our study aimed to retrospectively evaluate the expression of ASCL1, NeuroD1, POU2F3, and YAP1 in SCLC and LCNEC. Furthermore, we investigated if possible therapeutic targets can be evaluated by immunohistochemistry and might be used to identify patients, who might benefit from such treatment. As the expression of Myc-genes was also reported to be associated with NeuroD1 and YAP1, and in addition might induce vulnerability to aurora kinase A (AURKA) treatment, we evaluated this by using different antibodies for all three Myc-variants (19,24,25). We present this article in accordance with the MDAR reporting checklist (available at https://tlcr.amegroups.com/article/view/10.21037/tlcr-23-505/rc).
Methods
From the pulmonary pathology archive of the D&R Institute of Pathology, Medical University of Graz, high-grade neuroendocrine carcinomas surgically removed in a period from 1992 to 2020 were selected. A part of them were available as a tissue microarray (TMA), and other cases as regular tissue blocks. For the TMA at least 4 cores, 0.6 mm in diameter, of tumor tissue and one core of normal adjacent lung tissue were inserted into the recipient block. The exploration set consisted of 41 SCLC and 49 LCNEC, including also combined SCLC [combined with LCNEC (n=1), adenocarcinoma (AC, n=2), squamous cell carcinoma (SCC, n=1) and pleomorphic carcinoma, n=1], and combined LCNEC (one of each with AC or SCC, respectively).
Since our cases on the TMA showed a predominance of NeuroD1 positive cases, we suspected a selection bias as these were from surgical resections (lung and/or lymph nodes). therefore, we added another set of cases (validation) using bronchial and transthoracic needle biopsies. A total of 36 consecutive cases were selected based on available tissues on the paraffin blocks.
Four µm thick sections were cut from all tissues and were incubated with antibodies for ASCL1, NeuroD1, YAP, POU2F3, TAZ, C-MYC, L-MYC, N-MYC, vimentin, HES1, FGFR1, FGFR2, AURKA, PD-L1, RB1, PTEN, and p53 (details for the antibodies used are presented in Table 1). As some SCLC cases were negative for all major genes, atonal homolog 1 (ATOH1), a gene responsible for nerve development, was added to our immunohistochemistry panel. The antibodies used in this study were tested for specificity on TMAs with cancers of different organ systems. In addition, antibodies were selected, if the company provided a Western blot showing the specificity of the antibody. Patient characteristics are given in Table 2. This study was approved by the Ethical Committee of the Medical University of Graz (EK 24-135 ex 11/12). As no patient identification data were used in this study, informed consent of patients was not required. The study was conducted in accordance with the Declaration of Helsinki (as revised in 2013).
Table 1
Antibody | Company, clone | Dilution | Pretreatment | Detection |
---|---|---|---|---|
ASCL1 | Sigma, polyclonal | 1:50 | Ventana CC1 32 min | Ventana ultraView |
NeuroD1 | Abcam, polyclonal | 1:500 | MW9.0 40 min | DAKO K5007 Envision DAB |
POU2F3 | Sigma, polyclonal | 1:500 | MW9.0 40 min | DAKO K5007 Envision DAB |
YAP1 | Abcam, EP1675Y | 1:300 | Ventana CC1 32 min | Ventana ultraView |
TAZ | Santa Cruz, polyclonal | 1:50 | MW6.0 40 min | DAKO K5007 Envision DAB |
Vimentin | Dako, V9 | Rtu | OMNIS, Low ph | OMNIS Flex DAB |
C-MYC | Abcam, Y69 | 1:100 | OMNIS high ph | OMNIS Flex DAB |
L-MYC | Invitrogen, Polyclonal PA5-41114 | 1:500 | Ventana CC1 64 min | Ventana Optiview + Amplification |
N-MYC | Abcam, Ab198912 | 1:1,000 | Ventana CC1 64 min | Ventana Optiview + Amplification |
HES1 | Cell signaling, D6P2U | 1:1,000 | Ventana CC1 64 min | Ventana Optiview + Amplification |
FGFR1 | Abcam, polyclonal, EPR806Y | 1:100 | MW9.0 40 min | DAKO K5007 Envision DAB |
FGFR2 | Abcam, polyclonal, ab10648 | 1:1,000 | MW9.0 40 min | DAKO K5007 Envision DAB |
Aurora A | Biosource, polyclonal | 1:100 | Ventana CC1 32 min | Ventana ultraView |
PD-L1 | Ventana 741-4905, SP263 | Rtu | Ventane CC1 64 min | Ventana optiView |
RB1 | Santa Cruz, sc-102 | 1:100 | OMNIS high ph | OMNIS Flex DAB |
PTEN | Dako M3627 | 1:100 | MW6.0 40 min | DAKO K5007 Envision DAB |
P53 | Dako, GA616 | Rtu | OMNIS high ph | OMNIS Flex DAB |
ATOH | LSBio, LS-B6529 | 1:50 | Citrate solution, pH 6.0 | DAKO K5007 Envision DAB |
ASCL1, achaete scute family BHLH transcription factor 1; POU2F3, POU class 2 homeobox 3; YAP1, Yes1 associated transcription regulator 1; TAZ, Tafazzin; Rtu, ready to use; HES1, Hes family bHLH transcription factor1; AURKA, aurora kinase A; FGFR, fibroblast growth factor receptor; PD-L1, programmed cell death ligand 1; RB1, retinoblastoma gene 1; PTEN, phosphatase and tensin homolog; ATOH1, atonal homolog 1.
Table 2
Cancer type | Gender | Age range at diagnosis, years | Range of OS months | Therapy |
---|---|---|---|---|
SCLC, all cases in stage IV | M=35, F=6 | 54–79 | 14–26 | Chemotherapy in all cases: cisplatin + etoposide; in cases with cerebral metastasis additional radiation |
LCNEC, stages IIB–IIIB | M=37, F=12 | 27–75 | 22–42 | Resection; preoperative chemotherapy in one case |
OS, overall survival; SCLC, small cell lung cancer; LCNEC, large cell neuroendocrine carcinoma.
Statistical analysis
Statistical analysis was done for patient data, using mean and standard deviation of age and overall survival (see Table 2). For an immunoscore the intensity of staining (0–3+) and the percentage of stained tumor cells were multiplied, giving a range of 0–300. Percentages were calculated with 10% increments. No further statistical analysis was required.
Results
In the exploration set, 17/41 SCLC cases expressed ASCL1 and 32/41 NeuroD1, respectively (Figure 1A,1B). In 8/41 cases POU2F3 was expressed (Figure 1C). A combined expression of ASCL1 and NeuroD1 was seen in 9/41. In LCNEC ASCL1 was expressed in 10/49, NeuroD1 in 30/49 cases (Figure 1D). A combination of expression patterns for ASCL1 and NeuroD1 was seen in 6/49 cases. Five cases (5/49) expressed POU2F3, two of them also expressing NeuroD1 (Table 3). In cases showing coexpression of these proteins, a higher percentage of tumor cells usually expressed one of the proteins and a smaller percentage expressed the other marker. This can be interpreted as the presence of different tumor cell populations. However, there were also cases coexpressing both ASCL1 and NeuroD1 at a high percentage, which has to be interpreted that the same tumor cells expressed both. YAP1 was expressed in 2/41 and 17/49 of SCLC and LCNEC cases, respectively. TAZ was expressed in 6/41 SCLC and 5/49 LCNEC. YAP1 expression was combined in all cases with the expression of either POU2F3 or NeuroD1, but never with ASCL1. A combination of YAP1 and TAZ was seen in five cases (Figure 1E,1F). 17/41 SCLC and 24/49 LCNEC cases expressed ATOH1. ATOH1 was always combined with the expression of one of the two neuroendocrine master genes, as well as with POU2F3 (Figure 2A).
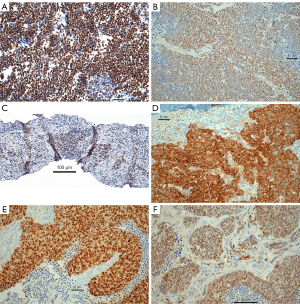
Table 3
Gene/marker | Test set SCLC (N=41) | Test set LCNEC (N=49) | Validation-set SCLC (N=36) |
---|---|---|---|
ASCL1 | 17/41 | 10/49 | 20/36 |
NeuroD | 32/41 | 30/49 | 25/36 |
POU2F3 | 8/41 | 5/49 | 1/36 |
ASCL1/NeuroD | 9/41 | 6/49 | 14/36 |
ATOH1 | 17/41 | 24/49 | n.d. |
YAP1 | 2/41 | 17/49 | n.d. |
TAZ | 6/41 | 5/49 | n.d. |
HES1 | 7/41 | 14/49 | n.d. |
AURKA | 24/41 | 37/49 | n.d. |
FGFR1 | 0 | 0 | n.d. |
FGFR2 | 5/41 | 5/49 | n.d. |
PD-L1 | 5/41 | 17/49 | n.d. |
C-MYC | 5/41 | 4/49 | n.d. |
L-MYC | 0/41 | 3/49 | n.d. |
N-MYC | 41/41 | 49/49 | n.d. |
SCLC, small cell lung cancer; LCNEC, large cell neuroendocrine carcinoma; ASCL1, achaete scute family BHLH transcription factor 1; POU2F3, POU class 2 homeobox 3; ATOH1, atonal homolog 1; n.d., not done; YAP1, Yes1 associated transcription regulator 1; TAZ, Tafazzin; HES1, Hes family bHLH transcription factor 1; AURKA, Aurora kinase A; FGFR, fibroblast growth factor receptor.
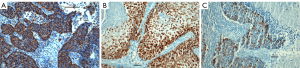
As HES1 is an antagonist for the neuroendocrine markers, especially ASCL1, we were interested if HES expression can be found in untreated SCLC and LCNEC. HES1 expression was seen in the study set in 7/41 SCLC, however, only in cases of combined SCLC (SCLC combined with NSCLC), whereas HES1 expression was seen in 14/49 LCNEC cases (Figure 2B,2C, Table 3). Out of these seven SCLC cases, five expressed NeuroD1, and two expressed POU2F3. HES1 expression was restricted to a few SCLC cells, but was highly expressed for example in the squamous cell component in combined SCLC. In LCNEC more cells expressed HES1. So probably HES1 expression is already found in single tumor cells and might expand during chemotherapy. As we did not have enough cases with pre- and post-chemotherapy biopsies, we cannot finally answer the question if HES1 upregulation is a regular mechanism for chemotherapy resistance. This will have to be addressed in a future investigation.
In 36 biopsy cases of SCLC (Table 3), ASCL1 was expressed in 20, and NeuroD1 in 25 cases, respectively, whereas POU2F3 was seen in one case only (Figure 3A-3C; for NeuroD1 in LCNEC see also Figure 1D). Coexpression of ASCL1 and NeuroD1 was seen in 14 cases. There were variations such as a majority of cells expressing either one and a minority of the other gene, but again here in a few cases both genes were coexpressed in high percentages in the same tumor cells.
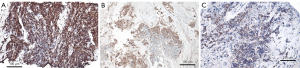
Identification of possible therapeutical targets?
AURKA was expressed in 24/41 and 37/49 cases of SCLC and LCNEC, respectively (Figure 4A,4B). There was a trend of higher positivity in those cases expressing NeuroD1, but no significance was reached (Table 3). There was some variation with respect to staining intensity and percentage of positive tumor cells, as can be seen in Figure 4A,4B. Only few cases showed a high staining intensity combined with high percentage of stained tumor cells (Table S1; H-score for each case). No case expressed FGFR1, whereas FGFR2 was seen in 5 cases each of SCLC and LCNEC (Figure 4C,4D, Table 4).
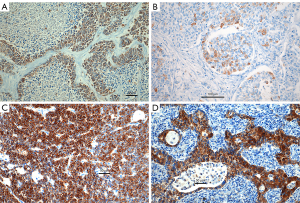
Table 4
Possible targets | Tumor type | ASCL1 | NeuroD | Combined ASCL1/NeuroD | POU2F3 | ATOH1 | Combined POU2F3/NeuroD | Null |
---|---|---|---|---|---|---|---|---|
AURKA expression and subtypes of SCLC and LCNEC | SCLC | 2 | 8 | 7 | 2 | 4 | 1 | |
H-Score, mean ± STD |
245±63 | 158±86 | 125±44 | 132±17 | 187+140 | 80 | ||
LCNEC | 1 | 20 | 8 | 0 | 3 | 5 | ||
H-Score, mean ± STD |
290 | 99±50 | 115±42 | 250±78 | 117±38 | |||
Expression of FGFR2 in relation to the expression of neuroendocrine master genes | SCLC | 0 | 2 | 2 | 0 | 0 | 1 | |
H-Score, mean ± STD |
25±21 | 73±38 | 60 | |||||
LCNEC | 0 | 2 | 1 | 0 | 0 | 2 | ||
H-Score, mean ± STD |
97±60 | 130 | 112±38 | |||||
Expression of PD-L1 in correlation to master genes; study set | SCLC | 1 | 0 | 3 | 0 | 1* | 1 | |
LCNEC | 1 | 7 | 5 | 1 | 3 | |||
Expression of RB1 and PTEN in LCNEC, and correlation to master genes | LCNEC, RB1 loss | 2 | 4 | 6 | 1 | 3 | ||
LCNEC, RB1 retained, PTEN loss | 8 | 1 | 2 | 1 | 5 |
*, ATOH1 positive cases included in ASCL1 case. Due to the small amount of tissues in the validation set (biopsies) these markers were not evaluated. Null = in these cases no staining for any of the three neuroendocrine master genes was seen. ASCL1, achaete scute family BHLH transcription factor 1; POU2F3, POU class 2 homeobox 3; AURKA, aurora kinase A; FGFR2, fibroblasts growth factor 2; PD-L1, programmed cell death ligand 1; RB1, retinoblastoma gene 1; PTEN, phosphatase and tensin homolog; STD, standard deviation; SCLC, Small cell lung cancer; LCNEC, large cell neuroendocrine carcinomas.
To see if the SCLC-I can be identified by PD-L1 immunohistochemical staining, the cases were studied for this immune-checkpoint molecule. An expression on tumor cells was seen in only two cases (one LCNEC, and one combined SCLC-LCNEC). In SCLC cases, the expression of PD-L1 was almost exclusively seen in lymphocytes and dendritic cells in ten cases (Figure 5A,5B, Table 4). PD-L1 positivity was found in two cases positive for ASCL1, three cases with a combined expression of ASCL1 and NeuroD1, two cases expressing NeuroD1, and one case of each expressing POU2F3, POU2F3/NeuroD1, and ATOH1. In LCNEC, PD-L1 was expressed in the stroma of 17 cases (5 combined ASCL1/NeuroD1, 7 NeuroD1, 1 ASCL1, 1 POU2F3/NeuroD1, and 3 without neuroendocrine gene expression). Neither in SCLC nor LCNEC did the expression for PD-L1 define a subtype. The percentage of positively stained stroma cells was less than 50% in all cases.
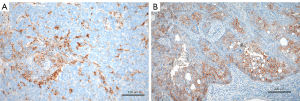
In our study we used antibodies for three MYC variants, C-MYC, L-MYC, and N-MYC. Interestingly N-MYC was expressed in all cases of SCLC and LCNEC. C-MYC was expressed in 5/41 cases of SCLC and 4/49 cases of LCNEC, the reaction for L-MYC was negative in all SCLC cases, but positive in three cases of LCNEC (Figure 6A-6F). A semiquantitative H-score was established for the expression of the MYC proteins: N-MYC 190±59 (median ± standard deviation; range, 120–300), C-MYC 30±23 (range, 10–70), L-MYC 140±65 (range, 80–210). An association of MYC and aurora kinase expression could not be established in our study.
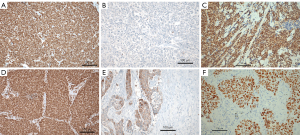
LCNEC and RB1-loss
A loss of RB1 was seen in 16/49 but retained in 17/49 cases (Figure 7A-7C, Table 4). PTEN was lost in 17/49 of LCNEC, but retained in 6/49 cases. Interestingly in 2/41 cases of SCLC PTEN was retained. In the majority of SCLC and LCNEC cases, TP53 was mutated resulting in positive staining for the p53 protein. Only 4/41 cases of SCLC and 9/49 cases of LCNEC were unstained. This could be due to a gene truncation with the inability to synthesize a protein detectable for the p53 antibody.
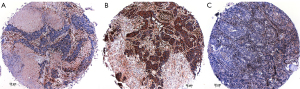
Discussion
Our study confirmed the existence of three subtypes in both SCLC and LCNEC. Interestingly, the NeuroD1 gene expression was more often seen in the surgical samples and in biopsy samples (validation set) compared to ASCL1. This is in contrast to published series from North America, China, and Europe (12,13,26). If this is due to population differences or due to a selection bias in this retrospective series of a rather small number of cases cannot be answered. Larger prospective series might clarify this issue. Interestingly, and previously reported NeuroD1 was more often expressed in LCNEC (27).
The function of the neuroendocrine master genes has been elucidated only in part. Wang et al did a secretome analysis in cell lines and showed that ASCL1 enhances the transcription of insulin growth factor binding protein 5 (IGFBP5). If suppressed, reduced IGFBP5 leads to hyperactivation of IGFR1 signaling. Downregulation of both might, however, downregulate the proliferation of SCLC (28). ASCL1 suppresses HES1, which in concert with NOTCH1/2/3 inhibits neuroendocrine and neuronal differentiation in the fetal lung (29). In EGFR-mutated AC treated with EGFR inhibitor transdifferentiation has been seen, where besides loss of RB1 and mutations in TP53, ASCL1 upregulation inhibited HES1 and NOTCH expression, resulting in a neuroendocrine phenotype (30-32). Much less is known about NeuroD1. NeuroD1 is a basic helix-loop-helix type transcription factor involved in neuroendocrine differentiation (29). It was reported to be more frequently expressed in LCNEC compared to ASCL1 (27), which we can confirm from our cases. Similarly, to ASCL1, NeuroD1 also binds to another IGF-binding protein, namely IGFBP2 (33). ATOH1 has been identified to regulate gut development but also is involved in nerve development (34,35). This protein was expressed in several cases of our cohort, both SCLC and LCNEC. However, the expression of ATOH1 did not define a new subtype in contrast to a previous report (36). But, as ATOH1 is involved in the regulation of several cancer genes within the Wnt pathway, its expression might be more generally associated with the carcinogenesis of these tumors (37). The POU2F3 gene is normally expressed in peripheral chemosensory lung cells (38), and therefore unexpected in tumors, usually arising in central lung. However, these chemosensory cells can also be found in nasal epithelia and down along the airways, and even in the thymus sensing foreign invaders. Therefore, the expression of POU2F3 in SCLC is not so unexpected anymore.
The coexpression of ASCL1 and NeuroD1 in tumor cells, as seen in our investigation, does not seem logically. Why both markers are expressed, when each alone is sufficient to keep a neuroendocrine phenotype? This phenomenon has been studied experimentally. Transcriptomic analysis showed a shift from ASCL1 to NeuroD11 expression (24). Another study showed plasticity and phenotypic switching between the subtypes (39). Therefore, the coexpression seen in our study probably reflects this kind of transition stages between the two neuroendocrine master genes. In a recent investigation by Wollenzien et al. (40) subclonal analysis showed the presence of ASCL1 and NeuroD1 clones, where during evolution of the carcinoma a shift towards NeuroD1 occurred. Although confirming our finding, it still does not explain the reasons for such switching, as a response to chemotherapy cannot be the case in our samples.
Myc oncogenes plays a role in SCLC. In cooperation with RB1-loss and TP53 mutation, it caused chemotherapy resistance in SCLC in a mouse model (41), and similar findings were reported in human tumors (19). In neuroendocrine cells, MYC activates NOTCH to dedifferentiate tumor cells, promoting a temporal shift in SCLC from ASCL1high to NeuroD11high and YAP1high states. MYC alternatively promotes POU2F3+ tumors as discussed above (24). In addition, a vulnerability was proposed for Aurora A kinase treatment when MYC genes are activated (19,42). In these reports no information was provided, which of the three forms of MYC proteins were identified. Three different isoforms of MYC are known, labeled as C-, L-, and N-MYC. All of them exert similar functions in carcinogenesis and if amplified or overexpressed act as oncogenes. Whereas many reports can be found for C-MYC and N-MYC not much has been reported about L-MYC. N-MYC is expressed predominantly in tumors of the nervous system, for example as a progression factor in neuroblastoma (43). However, it was found as a downstream target of the RET oncogene, which acts via gene fusion in pulmonary ACs (44). C-MYC acts as a transcription factor in many cancer types. C-MYC deregulates the microenvironment in tumors, plays a role in tumor cell metabolism for glucose and lactate, and plays a promoting role in the chemoresistance of cancer cells (45-47). We have demonstrated, that C-MYC is expressed in a minority of SCLC cases, whereas N-MYC was expressed in all cases of SCLC and LCNEC. L-MYC was only seen in three cases of LCNEC. This is in contrast to the reports by George et al. (48). In these reports amplifications were found for C-MYC and L-MYC, however in a minority of cases. A protein expression was not evaluated. Activation of oncogenes is not only based on amplification, but also on posttranslational modification, such as pathway activation. There were some variations in staining intensity: those cases expressing C-MYC showed a reduced staining intensity for N-MYC (score 2+), whereas all other cases showed strong reactivity for N-MYC (score 3+). There was no association of N-MYC expression and subtypes of SCLC, and no correlation with the expression of AURKA. As all our cases are therapy-naïve, MYC expression is not related to a previous therapy. Both SCLC and LCNEC have different mechanisms to facilitate proliferation, such as inactivation of RB1, loss of PTEN, and others (49,50). Therefore very likely MYC functions in tumor cells by regulating glucose metabolism fueling the cancer cell needs for energy (51).
We confirmed that YAP1 is not a separate subtype neither in SCLC or LCNEC (12). In our series, YAP1 was constantly expressed together with one of the neuroendocrine master genes. The function of YAP1 was recently identified as being important in cell migration and induction of EMT (11,52). As SCLC predominantly migrates in small cell complexes or single cells similar but not identical to classical EMT, the expression of YAP1 fits well with this function. However, as only a minority of SCLC and LCNEC cases expressed YAP1, yet another gene might drive migration in these carcinomas. TAZ was reported to replace YAP1 functionally, therefore we evaluated this marker too. There was an overlap of TAZ and YAP1 expression in five cases, which make it unlikely, that YAP1 can be replaced by TAZ expression. It is more likely, that TAZ has other functions in these carcinomas. YAP1 and TAZ are both transcriptional cofactors, which do not bind to the DNA, but are both regulators of the Hippo pathway and influence the rigidity of the matrix components (53). Recently DLL3-SNAI1 were experimentally shown to enhance migration in SCLC, while another group reported on N-acetylglucosaminyltransferase V inducing N-cadherin and ZEB2, both associated with migration (54,55). A so-called inflammatory subtype was recently proposed and besides different cytokines, PD-L1 was also upregulated in these cases (14). We tried to identify this group using the expression of PD-L1 on stroma cells as a surrogate marker. The expression of PD-L1 in tumor stroma (lymphocytes, dendritic cells) in our cases did not identify an unique subtype weather in SCLC or LCNEC. Therefore, PD-L1 should be assessed by immunohistochemistry to select those patients, which might benefit from immune-oncologic treatment. Further investigation to more precisely define the inflammatory subtype of SCLC is necessary. In such an investigation, markers should be identified, which allows an easy separation.
For decades no improvement in the treatment of SCLC was achieved. The standard of care was (and still is) chemotherapy often combined with radiation. The overall survival of patients with SCLC is very short, as most of them present with stage IV disease. Due to recent progress in the molecular characterization of SCLC, new targets have been identified and clinical trials are showing new treatment options (14,16,19,42,56-58). In these trials no analysis of the expression patterns of these new targets have been reported. However, it might be important to select patients, who have a high probability to respond to such treatments. It was proposed, that SCLC showing upregulation of the MYC oncogene will respond to an AURKA inhibition (58,59). We, therefore, evaluated AURKA for its immunohistochemical expression. AURKA was expressed in two-thirds of our SCLC and LCNEC cases. A blockade of this kinase in combination with conventional therapy might increase the survival of patients. A clinical study is underway (19), but patients have not been selected for the kinase expression, but instead retrospectively been evaluated for MYC expression. The type of MYC protein was not reported. In our study, AURKA was not associated with any SCLC or LCNEC subtype, and no association between AURKA expression with any of the three variants of MYC proteins was seen. But we saw some variation in the expression of the kinase illustrated by staining intensity and percentage of positively stained tumor cells. This might correspond to the response to the tyrosine kinase inhibitor therapy (TKI).
FGFR2 might be another target for TKI since inhibitors for the fibroblast growth factor receptors already exist (60). In our cases, strong immunohistochemical staining was observed in five cases, and these cases might be candidates for FGFR2 inhibitor therapy. In a recent investigation of multiple carcinomas, inhibition of tumor growth was only seen, when the mutation of the receptor lies within exon 18, resulting in a truncated protein (61). If the response to TKI will be dependent similarly on the type of FGFR2 mutation needs to be investigated in a new study.
Immuno-oncologic treatment is now being applied to almost every solid tumor. Based on some case reports, clinical trials are being conducted also in SCLC. Different approaches are followed, but in all, a combination with chemotherapy is applied, either simultaneously or subsequentially (56,62). In our study, we could identify the expression of PD-L1 in stroma cells in a small subset of both high-grade neuroendocrine carcinomas, which might correlate with a response to a combined chemo- and immunotherapy. Therefore, staining for PD-L1 and probably other checkpoint inhibitors should be routinely performed using an immunoscore. A recent investigation pointed to another option to assess the possibility of immunotherapy: high expression of stimulator of interferon genes (STING) was associated with enrichment with immune cell infiltration. As STING expression can be therapeutically influenced, this opens another treatment option (63).
HES1 is an antagonist for ASCL1 and was seen in some of our SCLC and LCNEC cases. Strong expression was seen in some cases of combined SCLC, where the non-neuroendocrine part was intensely positive. However, even within SCLC and LCNEC cases few scattered positive carcinoma cells were encountered. If these cells could be the source of chemotherapy-resistant SCLC could not be answered, as we had only two cases available with tissue biopsies before and after chemotherapy and therefore an answer to the question could not be provided. Here further studies are necessary.
Chemotherapy for LCNEC has long been debated. Some reports claimed that a SCLC-based therapy protocol resulted in longer overall survival, whereas others published data on improved survival using cisplatin-based therapy (1). Recent investigation shed light on the likely background: LCNEC presenting with genetic modifications similar to SCLC respond better to an SCLC-type of treatment. Most important here is the loss of RB1 on one allele combined with the silencing of the second one. LCNEC with retained RB1 and loss of PTEN, or an amplified PI3KCA responded better to cisplatin-based therapy (23). Therefore, immunohistochemistry for RB1 and PTEN should be routinely performed in LCNEC. A clinical trial might be performed based on this assumption.
Our study has some limitations: first the number of cases is small. This is due to the decrease of SCLC in general. Whereas SCLC was common in the 1980-ties (25%), the percentage has dropped to 8% in the last decade. Using older samples might be an option, but requires many tests to exclude degradation of the proteins. Another limitation of the study is, that it was done retrospectively. With respect to our data on PD-L1 staining, probably a more detailed analysis of immune cells infiltrating the tumor should be done, especially subtyping macrophages and dendritic cells. A recent report already pointed this aspect (64).
Conclusions
In this investigation we confirmed three SCLC subtypes and excluded YAP1 as a separate one. ATOH although expressed did not create another subtype. We presented new data on LCNEC, which similar to SCLC can be separated by ASCL1, NeuroD1, and POU2F3. MYC expression was specified by N-MYC in all whereas C-MYC expression was restricted to five and four cases of SCLC and LCNEC, respectively. AURKA seems to be promising target for inhibition in both high-grade carcinomas, whereas FGFR2 inhibition might be an option in few cases. If the variation in staining intensity and percentage of tumor cells expressing AURKA has an impact on response to TKI treatment needs to be explored in further studies. Immuno-oncologic treatment in SCLC and LCNEC might be based on the expression of PD-L1 in the tumor stroma. Finally, chemotherapy of LCNEC might be guided by immunohistochemical staining for RB1 and PTEN.
Acknowledgments
Funding: None.
Footnote
Reporting Checklist: The authors have completed the MDAR reporting checklist. Available at https://tlcr.amegroups.com/article/view/10.21037/tlcr-23-505/rc
Data Sharing Statement: Available at https://tlcr.amegroups.com/article/view/10.21037/tlcr-23-505/dss
Peer Review File: Available at https://tlcr.amegroups.com/article/view/10.21037/tlcr-23-505/prf
Conflicts of Interest: All authors have completed the ICMJE uniform disclosure form (available at https://tlcr.amegroups.com/article/view/10.21037/tlcr-23-505/coif). H.P. has received unrestricted grant for a cell culture matrix printing device from Astra Zeneca, Hoffmann La Roche; support from attending advisary board meeting of AstraZeneca and is a member of Committee for the preparation of WCLC Vienna of IASLC. L.B. received grants from Takeda, AstraZeneca, BMS and Roche; he also received payment for lectures and participated in advisory boards form Invitae, Eli-Lilly, AstraZeneca, Roche, MSD, Merck, BMS, Pfizer, Novartis, Takeda, Janssen; support for attending meeting from Pfizer and MSD. He is Int. Secretary-Austrian Society of Pathology; PPS Membership and Awards Committee; Member of the Mesothelioma Committee of IASLC. The other author has no conflicts of interest to declare.
Ethical Statement: The authors are accountable for all aspects of the work in ensuring that questions related to the accuracy or integrity of any part of the work are appropriately investigated and resolved. This retrospective study was approved by the Ethical Committee of the Medical University of Graz (EK 24-135 ex 11/12). As no patient identification data were used in this study, informed consent of patients was not required. The study was conducted in accordance with the Declaration of Helsinki (as revised in 2013).
Open Access Statement: This is an Open Access article distributed in accordance with the Creative Commons Attribution-NonCommercial-NoDerivs 4.0 International License (CC BY-NC-ND 4.0), which permits the non-commercial replication and distribution of the article with the strict proviso that no changes or edits are made and the original work is properly cited (including links to both the formal publication through the relevant DOI and the license). See: https://creativecommons.org/licenses/by-nc-nd/4.0/.
References
- Rossi G, Cavazza A, Marchioni A, et al. Role of chemotherapy and the receptor tyrosine kinases KIT, PDGFRalpha, PDGFRbeta, and Met in large-cell neuroendocrine carcinoma of the lung. J Clin Oncol 2005;23:8774-85. [Crossref] [PubMed]
- Lo Russo G, Pusceddu S, Proto C, et al. Treatment of lung large cell neuroendocrine carcinoma. Tumour Biol 2016;37:7047-57. [Crossref] [PubMed]
- Derks JL, Hendriks LE, Buikhuisen WA, et al. Clinical features of large cell neuroendocrine carcinoma: a population-based overview. Eur Respir J 2016;47:615-24. [Crossref] [PubMed]
- Kujtan L, Muthukumar V, Kennedy KF, et al. The Role of Systemic Therapy in the Management of Stage I Large Cell Neuroendocrine Carcinoma of the Lung. J Thorac Oncol 2018;13:707-14. [Crossref] [PubMed]
- Sakaeda M, Sato H, Ishii J, et al. Neural lineage-specific homeoprotein BRN2 is directly involved in TTF1 expression in small-cell lung cancer. Lab Invest 2013;93:408-21. [Crossref] [PubMed]
- Broers JL, Carney DN, Klein Rot M, et al. Intermediate filament proteins in classic and variant types of small cell lung carcinoma cell lines: a biochemical and immunochemical analysis using a panel of monoclonal and polyclonal antibodies. J Cell Sci 1986;83:37-60. [Crossref] [PubMed]
- Banks-Schlegel SP, Gazdar AF, Harris CC. Intermediate filament and cross-linked envelope expression in human lung tumor cell lines. Cancer Res 1985;45:1187-97. [PubMed]
- Rudin CM, Poirier JT, Byers LA, et al. Molecular subtypes of small cell lung cancer: a synthesis of human and mouse model data. Nat Rev Cancer 2019;19:289-97. [Crossref] [PubMed]
- Huang YH, Klingbeil O, He XY, et al. POU2F3 is a master regulator of a tuft cell-like variant of small cell lung cancer. Genes Dev 2018;32:915-28. [Crossref] [PubMed]
- Garmendia I, Pajares MJ, Hermida-Prado F, et al. YES1 Drives Lung Cancer Growth and Progression and Predicts Sensitivity to Dasatinib. Am J Respir Crit Care Med 2019;200:888-99. [Crossref] [PubMed]
- Yu M, Chen Y, Li X, et al. YAP1 contributes to NSCLC invasion and migration by promoting Slug transcription via the transcription co-factor TEAD. Cell Death Dis 2018;9:464. [Crossref] [PubMed]
- Baine MK, Hsieh MS, Lai WV, et al. SCLC Subtypes Defined by ASCL1, NEUROD1, POU2F3, and YAP1: A Comprehensive Immunohistochemical and Histopathologic Characterization. J Thorac Oncol 2020;15:1823-35. [Crossref] [PubMed]
- Megyesfalvi Z, Barany N, Lantos A, et al. Expression patterns and prognostic relevance of subtype-specific transcription factors in surgically resected small-cell lung cancer: an international multicenter study. J Pathol 2022;257:674-86. [Crossref] [PubMed]
- Gay CM, Stewart CA, Park EM, et al. Patterns of transcription factor programs and immune pathway activation define four major subtypes of SCLC with distinct therapeutic vulnerabilities. Cancer Cell 2021;39:346-360.e7. [Crossref] [PubMed]
- Miyoshi T, Umemura S, Matsumura Y, et al. Genomic Profiling of Large-Cell Neuroendocrine Carcinoma of the Lung. Clin Cancer Res 2017;23:757-65. [Crossref] [PubMed]
- Liu SV, Reck M, Mansfield AS, et al. Updated Overall Survival and PD-L1 Subgroup Analysis of Patients With Extensive-Stage Small-Cell Lung Cancer Treated With Atezolizumab, Carboplatin, and Etoposide (IMpower133). J Clin Oncol 2021;39:619-30. [Crossref] [PubMed]
- Saunders LR, Bankovich AJ, Anderson WC, et al. A DLL3-targeted antibody-drug conjugate eradicates high-grade pulmonary neuroendocrine tumor-initiating cells in vivo. Sci Transl Med 2015;7:302ra136. [Crossref] [PubMed]
- Allison Stewart C, Tong P, Cardnell RJ, et al. Dynamic variations in epithelial-to-mesenchymal transition (EMT), ATM, and SLFN11 govern response to PARP inhibitors and cisplatin in small cell lung cancer. Oncotarget 2017;8:28575-87. [Crossref] [PubMed]
- Mollaoglu G, Guthrie MR, Böhm S, et al. MYC Drives Progression of Small Cell Lung Cancer to a Variant Neuroendocrine Subtype with Vulnerability to Aurora Kinase Inhibition. Cancer Cell 2017;31:270-85. [Crossref] [PubMed]
- Lokuhetty D, White VA, Cree IA, editors. WHO Classification of Tumours Editorial Board. Thoracic Tumours. IARC publications, Lyon, France; 2021:565.
- Derks JL, Leblay N, Lantuejoul S, et al. New Insights into the Molecular Characteristics of Pulmonary Carcinoids and Large Cell Neuroendocrine Carcinomas, and the Impact on Their Clinical Management. J Thorac Oncol 2018;13:752-66. [Crossref] [PubMed]
- Rekhtman N, Pietanza MC, Hellmann MD, et al. Next-Generation Sequencing of Pulmonary Large Cell Neuroendocrine Carcinoma Reveals Small Cell Carcinoma-like and Non-Small Cell Carcinoma-like Subsets. Clin Cancer Res 2016;22:3618-29. [Crossref] [PubMed]
- Derks JL, Leblay N, Thunnissen E, et al. Molecular Subtypes of Pulmonary Large-cell Neuroendocrine Carcinoma Predict Chemotherapy Treatment Outcome. Clin Cancer Res 2018;24:33-42. [Crossref] [PubMed]
- Ireland AS, Micinski AM, Kastner DW, et al. MYC Drives Temporal Evolution of Small Cell Lung Cancer Subtypes by Reprogramming Neuroendocrine Fate. Cancer Cell 2020;38:60-78.e12. [Crossref] [PubMed]
- Borromeo MD, Savage TK, Kollipara RK, et al. ASCL1 and NEUROD1 Reveal Heterogeneity in Pulmonary Neuroendocrine Tumors and Regulate Distinct Genetic Programs. Cell Rep 2016;16:1259-72. [Crossref] [PubMed]
- Qu S, Fetsch P, Thomas A, et al. Molecular Subtypes of Primary SCLC Tumors and Their Associations With Neuroendocrine and Therapeutic Markers. J Thorac Oncol 2022;17:141-53. [Crossref] [PubMed]
- Hiroshima K, Iyoda A, Shida T, et al. Distinction of pulmonary large cell neuroendocrine carcinoma from small cell lung carcinoma: a morphological, immunohistochemical, and molecular analysis. Mod Pathol 2006;19:1358-68. [Crossref] [PubMed]
- Wang XD, Hu R, Ding Q, et al. Subtype-specific secretomic characterization of pulmonary neuroendocrine tumor cells. Nat Commun 2019;10:3201. [Crossref] [PubMed]
- Ito T, Udaka N, Yazawa T, et al. Basic helix-loop-helix transcription factors regulate the neuroendocrine differentiation of fetal mouse pulmonary epithelium. Development 2000;127:3913-21. [Crossref] [PubMed]
- Xie M, Zhang L, He CS, et al. Activation of Notch-1 enhances epithelial-mesenchymal transition in gefitinib-acquired resistant lung cancer cells. J Cell Biochem 2012;113:1501-13. [Crossref] [PubMed]
- Niederst MJ, Sequist LV, Poirier JT, et al. RB loss in resistant EGFR mutant lung adenocarcinomas that transform to small-cell lung cancer. Nat Commun 2015;6:6377. [Crossref] [PubMed]
- Tenjin Y, Nakamura K, Ishizuka S, et al. Small Cell Lung Cancer Derived from Adenocarcinoma with Mutant Epidermal Growth Factor Receptor Provides a Signature of Transcriptional Alteration in Tumor Cells. Intern Med 2019;58:3261-5. [Crossref] [PubMed]
- Yazawa T, Sato H, Shimoyamada H, et al. Neuroendocrine cancer-specific up-regulating mechanism of insulin-like growth factor binding protein-2 in small cell lung cancer. Am J Pathol 2009;175:976-87. [Crossref] [PubMed]
- Westerman BA, Breuer RH, Poutsma A, et al. Basic helix-loop-helix transcription factor profiling of lung tumors shows aberrant expression of the proneural gene atonal homolog 1 (ATOH1, HATH1, MATH1) in neuroendocrine tumors. Int J Biol Markers 2007;22:114-23. [Crossref] [PubMed]
- Okubo T, Hogan BL. Hyperactive Wnt signaling changes the developmental potential of embryonic lung endoderm. J Biol 2004;3:11. [Crossref] [PubMed]
- Simpson KL, Stoney R, Frese KK, et al. A biobank of small cell lung cancer CDX models elucidates inter- and intratumoral phenotypic heterogeneity. Nat Cancer 2020;1:437-51. [Crossref] [PubMed]
- Xu HT, Xie XM, Li QC, et al. Atonal homolog 1 expression in lung cancer correlates with inhibitors of the Wnt pathway as well as the differentiation and primary tumor stage. APMIS 2013;121:111-9. [Crossref] [PubMed]
- O'Leary CE, Schneider C, Locksley RM. Tuft Cells-Systemically Dispersed Sensory Epithelia Integrating Immune and Neural Circuitry. Annu Rev Immunol 2019;37:47-72. [Crossref] [PubMed]
- Sutherland KD, Ireland AS, Oliver TG. Killing SCLC: insights into how to target a shapeshifting tumor. Genes Dev 2022;36:241-58. [Crossref] [PubMed]
- Wollenzien H, Afeworki Tecleab Y, Szczepaniak-Sloane R, et al. Single-Cell Evolutionary Analysis Reveals Drivers of Plasticity and Mediators of Chemoresistance in Small Cell Lung Cancer. Mol Cancer Res 2023;21:892-907. [Crossref] [PubMed]
- Dammert MA, Brägelmann J, Olsen RR, et al. MYC paralog-dependent apoptotic priming orchestrates a spectrum of vulnerabilities in small cell lung cancer. Nat Commun 2019;10:3485. [Crossref] [PubMed]
- Helfrich BA, Kim J, Gao D, et al. Barasertib (AZD1152), a Small Molecule Aurora B Inhibitor, Inhibits the Growth of SCLC Cell Lines In Vitro and In Vivo. Mol Cancer Ther 2016;15:2314-22. [Crossref] [PubMed]
- Wang Y, Gao S, Wang W, et al. Downregulation of N Myc inhibits neuroblastoma cell growth via the Wnt/β catenin signaling pathway. Mol Med Rep 2018;18:377-84. [Crossref] [PubMed]
- Kulkarni MV, Franklin DS. N-Myc is a downstream target of RET signaling and is required for transcriptional regulation of p18(Ink4c) by the transforming mutant RET(C634R). Mol Oncol 2011;5:24-35. [Crossref] [PubMed]
- Dhanasekaran R, Deutzmann A, Mahauad-Fernandez WD, et al. The MYC oncogene - the grand orchestrator of cancer growth and immune evasion. Nat Rev Clin Oncol 2022;19:23-36. [Crossref] [PubMed]
- Dang CV, Le A, Gao P. MYC-induced cancer cell energy metabolism and therapeutic opportunities. Clin Cancer Res 2009;15:6479-83. [Crossref] [PubMed]
- Fatma H, Maurya SK, Siddique HR. Epigenetic modifications of c-MYC: Role in cancer cell reprogramming, progression and chemoresistance. Semin Cancer Biol 2022;83:166-76. [Crossref] [PubMed]
- George J, Lim JS, Jang SJ, et al. Comprehensive genomic profiles of small cell lung cancer. Nature 2015;524:47-53. [Crossref] [PubMed]
- Thirusangu P, Ray U, Sarkar Bhattacharya S, et al. PFKFB3 regulates cancer stemness through the hippo pathway in small cell lung carcinoma. Oncogene 2022;41:4003-17. [Crossref] [PubMed]
- Liang SK, Hsu CC, Song HL, et al. FOXM1 is required for small cell lung cancer tumorigenesis and associated with poor clinical prognosis. Oncogene 2021;40:4847-58. [Crossref] [PubMed]
- Teicher BA. Targets in small cell lung cancer. Biochem Pharmacol 2014;87:211-9. [Crossref] [PubMed]
- Pastushenko I, Mauri F, Song Y, et al. Fat1 deletion promotes hybrid EMT state, tumour stemness and metastasis. Nature 2021;589:448-55. [Crossref] [PubMed]
- Piccolo S, Panciera T, Contessotto P, et al. YAP/TAZ as master regulators in cancer: modulation, function and therapeutic approaches. Nat Cancer 2023;4:9-26. [PubMed]
- Furuta M, Kikuchi H, Shoji T, et al. DLL3 regulates the migration and invasion of small cell lung cancer by modulating Snail. Cancer Sci 2019;110:1599-608. [Crossref] [PubMed]
- Huang C, Huang M, Chen W, et al. N-acetylglucosaminyltransferase V modulates radiosensitivity and migration of small cell lung cancer through epithelial-mesenchymal transition. FEBS J 2015;282:4295-306. [Crossref] [PubMed]
- Iams WT, Porter J, Horn L. Immunotherapeutic approaches for small-cell lung cancer. Nat Rev Clin Oncol 2020;17:300-12. [Crossref] [PubMed]
- Mansfield AS, Każarnowicz A, Karaseva N, et al. Safety and patient-reported outcomes of atezolizumab, carboplatin, and etoposide in extensive-stage small-cell lung cancer (IMpower133): a randomized phase I/III trial. Ann Oncol 2020;31:310-7. [Crossref] [PubMed]
- Serzan MT, Farid S, Liu SV. Drugs in development for small cell lung cancer. J Thorac Dis 2020;12:6298-307. [Crossref] [PubMed]
- Kolla BC, Racila E, Patel MR. Deep and Prolonged Response to Aurora A Kinase Inhibitor and Subsequently to Nivolumab in MYCL1-Driven Small-Cell Lung Cancer: Case Report and Literature Review. Case Rep Oncol Med 2020;2020:8026849. [Crossref] [PubMed]
- Turner N, Grose R. Fibroblast growth factor signalling: from development to cancer. Nat Rev Cancer 2010;10:116-29. [Crossref] [PubMed]
- Zingg D, Bhin J, Yemelyanenko J, et al. Truncated FGFR2 is a clinically actionable oncogene in multiple cancers. Nature 2022;608:609-17. [Crossref] [PubMed]
- Chung HC, Piha-Paul SA, Lopez-Martin J, et al. Pembrolizumab After Two or More Lines of Previous Therapy in Patients With Recurrent or Metastatic SCLC: Results From the KEYNOTE-028 and KEYNOTE-158 Studies. J Thorac Oncol 2020;15:618-27. [Crossref] [PubMed]
- Li X, Li Y, Zhao Z, et al. Immunogenicity of small-cell lung cancer associates with STING pathway activation and is enhanced by ATR and TOP1 inhibition. Cancer Med 2023;12:4864-81. [Crossref] [PubMed]
- Chan JM, Quintanal-Villalonga Á, Gao VR, et al. Signatures of plasticity, metastasis, and immunosuppression in an atlas of human small cell lung cancer. Cancer Cell 2021;39:1479-1496.e18. [Crossref] [PubMed]