PD-L1 mRNA derived from tumor-educated platelets as a potential immunotherapy biomarker in non-small cell lung cancer
Highlight box
Key findings
• This research presents the data of tumor-educated platelet (TEP)-derived programmed death ligand-1 (PD-L1) messenger RNA (mRNA) in advanced non-small cell lung cancer (NSCLC) patients following immunotherapy, and discusses the potential advantage of using it as a surrogate biomarker for predicting the progression-free survival (PFS) and overall survival (OS) of NSCLC patients following immunotherapy.
What is known and what is new?
• It has been reported that the platelet PD-L1 protein in NSCLC could reflect collective tumor PD-L1 expression, play important roles in tumor immune evasion, and overcome the limitations associated with the histological quantification of often heterogeneous intratumoral PD-L1 expression. TEP-derived mRNA has been employed for cancer diagnosis, mutation detection, and treatment monitoring.
• In this study, we prospectively detected pre-treatment PD-L1 mRNA and analyzed its prediction value for immunotherapy in advanced NSCLC patients.
What is the implication, and what should change now?
• In the present study, we demonstrated that TEP-derived PD-L1 mRNA could predict the response to immunotherapy, PFS, and OS of advanced NSCLC patients. The relationship between the level of TEP-derived PD-L1 and immune checkpoint inhibitor (ICI) resistance needs to be investigated further in the future. Additionally, the differences between TEP-derived PD-L1 and other biomarkers, such as tumor mutational burden and exosome PD-L1, also need to be studied.
Introduction
Lung cancer is the primary cause of cancer-related death worldwide (1). Every year, many people are diagnosed with or die from lung cancer (1). Lung cancer includes non-small cell lung cancer (NSCLC) (which accounts for 85% of all lung cancer cases) and small cell lung cancer (SCLC) (which accounts for 15% of all lung cancer cases) (2,3). Recently, unprecedented survival benefits have been achieved in selected patients using small molecule tyrosine kinase inhibitors or immunotherapy; however, the survival and overall cure rates of NSCLC patients remain low. Thus, continued research needs to be conducted into new therapy strategies to improve the clinical benefits and outcomes of NSCLC patients (4).
Immune checkpoint inhibitors (ICIs) have demonstrated encouraging success in the treatment of NSCLC patients, including locally advanced and metastatic patients without driver mutations. ICIs are now a primary part of the first- and second-line treatment armamentarium as monotherapy, combined with chemotherapy, or after definite chemoradiotherapy in advanced NSCLC patients (3). ICI monotherapy produced better clinical results than traditional chemotherapy in an unselected population of patients with advanced NSCLC (5). Further, extensive research has been conducted to identify predictive biomarkers for the initial ICI response. Tumor programmed death ligand-1 (PD-L1) expression, the tumor mutational burden (TMB), deficient mismatch repair, tumor infiltrating lymphocytes, and related gene expression signatures have been identified as potential predictors, and various other markers are under investigation (6-12). However, due to tumor heterogeneity, sampling bias, antibody discrepancy, and the poor feasibility of dynamic monitoring, obvious limitations exist among these biomarkers. Conversely, liquid biopsy has many advantages, as it enables the non-invasive overview of real-time changes in cancer and other diseases, including circulating tumor cells, circulating tumor DNA, circulating messenger RNA (mRNA), microRNA, exosomes, and tumor-educated platelets (TEPs) (13,14).
Circulating exosomal PD-L1 has been shown to be immunosuppressive and predict an anti-programmed death-1 (PD-1) response in patients with metastatic melanoma, which highlighted the potential of circulating biomarkers for cancer immunotherapy (15,16). Similarly, as the second most abundant cells in the blood, TEPs were studied in cancers by various ways, which could transfer information between tumors and immune cells (17). One study suggested that the platelet PD-L1 protein in NSCLC could reflect collective tumor PD-L1 expression, play important roles in tumor immune evasion, and overcome the limitations associated with the histological quantification of often heterogeneous intratumoral PD-L1 expression (18). The mRNA in TEPs has been employed for cancer diagnosis, mutation detection, and treatment monitoring (19-22). The role of PD-L1 mRNA derived from TEPs has not yet been well investigated. However, few reports have indicated that the mRNA in TEPs can predict the clinical response of advanced NSCLC patients after immunotherapy. In this study, we prospectively detected the pre-treatment PD-L1 mRNA and analyzed its prediction value for immunotherapy in advanced NSCLC patients. We present this article in accordance with the REMARK reporting checklist (available at https://tlcr.amegroups.com/article/view/10.21037/tlcr-24-29/rc).
Methods
Patient population and study design
Advanced NSCLC patients receiving a combination of immunotherapy and chemotherapy, or immunotherapy alone as the first- or second-line treatment at the Fudan University Shanghai Cancer Center (FUSCC) from January 2016 to July 2018 were enrolled in this study. All the patients had wild-type epidermal growth factor/anaplastic lymphoma kinase receptor. The patients were enrolled in clinical trials for ICIs, including nivolumab, pembrolizumab, atezolizumab, durvalumab, tremelimumab, and camrelizumab. The ICIs were administrated as per the study protocols, and the therapeutic effects were evaluated as a complete/partial response (CR/PR), stable disease (SD), or progressive disease (PD) according to the response evaluation criteria in solid tumors (RECIST) 1.1. This study was performed in accordance with the principles of the Declaration of Helsinki (as revised in 2013). The Institutional Review Board of Fudan University Shanghai Cancer Center approved the study (Date 2012-12-24; No. 050432-4-1212B), and all patients provided informed consent.
Tumoral PD-L1 expression was tested by immunohistochemistry (PD-L1 22C3 pharmDx kit, Agilent, Santa Clara, CA, USA) in archived tissue samples, when available, to calculate the tumor proportion scores (TPSs). 5 mL of peripheral blood samples were collected before immunotherapy from all the patients.
Platelet collection and purification
Platelets and plasma were isolated from the same sample of whole blood in tubes containing ethylenediamine tetra acetic acid anti-coagulant. The red cells, white cells, and aggregates were removed by centrifugation at room temperature for 20 min at 1,200 r/min, resulting in platelet-rich plasma. 10 µL of each obtained sample was used for the subjecting Swiss Giemsa staining. Samples with high purity, devoid of impurities such as leukocytes in the field of view, proceeded to the next steps of the operation. Thereafter, the platelets were isolated from the platelet-rich plasma by centrifugation for 20 min at 2,100 r/min. Platelet RNA and exosomal RNA were isolated using the Yunying RNA extraction kit (Yunying Medicine, Shanghai, China). The concentration and quality of the RNA was determined using a Qubit fluorometer (Life Technologies, Carlsbad, CA, USA). As a standard control, three healthy subjects provided equivalent blood samples for mRNA detection.
PD-L1 mRNA detection
Total RNA was stored at −80 ℃ awaiting use, and the complementary DNA was synthesized using a RT MasterMix kit (Yunying Medicine). The quantitative polymerase chain reaction (PCR) was run on the ABI7500 (Applied Biosystems, Foster City, CA, USA) for 10 min at 95 ℃ followed by 50 cycles of 10 s at 95 ℃ and 50 s at 60 ℃. The expression of human glyceraldehyde 3-phosphate dehydrogenase (GAPDH) was used as the normalization control in the real-time PCR reactions. The following primers were used: PD-L1 forward, 5'-AGCACTGACATTCATCTTCCG-3'; PD-L1 reverse, 5'-GCTGGATTACGTCTCCTCCA-3'; PD-L1 probe, 5'-FAM-AATGTGGCATCCAAGATAC-MGB-3'; GAPDH forward, 5'-CTCTGCTCCTCCTGTTCGAC-3', GAPDH forward, 5'-ATGGTGTCTGAGCGATGTGG-3'; GAPDH probe, 5'-FAM-CGTCGCCAGCCGA-MGB-3'. The cycle threshold (Ct) value was recorded as the threshold cycle of the PCR reaction, and the final mRNA expression value was generated from the PD-L1 Ct and GAPDH Ct value.
Statistical methods
Progression-free survival (PFS) and overall survival (OS) were estimated using the Kaplan-Meier method. PFS was defined as the time from ICI initiation until the date of disease progression or death. OS was defined as the time from ICI initiation until the date of death or last follow-up date. Patients were deemed to have a clinical benefit if they achieved an objective response (CR/PR) or had a SD duration of over 6 months. The median value was used as the PD-L1 mRNA cut-off value to define high PD-L1 expression or low PD-L1 expression. Using Cox regression analysis, we also analyzed the relationship between prognosis and clinical-pathological characteristics, such as age, gender, pathology, TPS (PD-L1 expression), with or without chemotherapy, lines of therapy, and expression level of TEP-derived PD-L1 mRNA. A two-sided P value <0.05 level was considered statistically significant.
Results
Patient characteristics
Of the 72 patients enrolled in this study, 64 (88.9%) received immunotherapy only, while the others were treated with immunotherapy in combination with platinum-contained chemotherapy. In this study, most of the patients were male (n=54, 75.0%), had adenocarcinoma (n=49, 68.1%), and received single-agent immunotherapy as a second-line therapy (n=58, 80.6%). The median follow-up was 6.3 months. Nivolumab was the most commonly used inhibitor (n=50, 69.4%) followed by atezolizumab (n=11, 15.3%) (Table 1).
Table 1
Characteristics | Values |
---|---|
Gender | |
Male | 54 (75.0) |
Female | 18 (25.0) |
Age (years) | 61 [42–75] |
Pathology | |
Adeno | 49 (68.1) |
Squamous | 23 (31.9) |
PD-L1 TPS (%) | |
<1 | 25 (34.7) |
1–49 | 9 (12.5) |
≥50 | 7 (9.7) |
Unknown | 31 (43.1) |
Treatment line | |
1st | 14 (19.4) |
2nd | 58 (80.6) |
Chemo combined | |
No | 64 (88.9) |
Pemetrexed/carboplatin | 5 (6.9) |
Paclitaxel/carboplatin | 3 (4.2) |
Checkpoint inhibitors | |
Nivolumab | 50 (69.4) |
Atezolizumab | 11 (15.3) |
Pembrolizumab | 1 (1.4) |
Camrelizumab | 4 (5.6) |
Tremelimumab | 6 (8.3) |
Best response | |
Partial response | 21 (29.2) |
Stable disease | 24 (33.3) |
Progressive disease | 23 (31.9) |
Unevaluable | 4 (5.6) |
Data are presented as n (%) or median [range]. PD-L1, programmed death ligand-1; TPS, tumor proportion score.
According to the RECIST 1.1 criteria, 21 patients achieved PR, with an objective response rate (ORR) of 29.2% as shown in Table 1. Seven patients (9.7%) had a SD duration of over 6 months. After a median follow-up of 15.8 months, there were 52 events of PD or death. The median PFS was 3.81 months (95% confidence interval: 2.0 to 5.6 months). There were 20 deaths (34.5%) in the second-line cohort; however, there was only one death (7.1%) in the first-line cohort (P=0.015).
TEP-derived PD-L1 mRNA was uncorrelated with PD-L1 TPS
Blood samples were collected from all patients. The range of PD-L1 mRNA expression of TEPs detected in 72 patients was from 1.7 to 9.9, in which the median value was 3.17. PD-L1 expression was examined successfully in the archived tissue of 41 patients (56.9%). Based on the review by two independent pathologists on PD-L1 TPS, the rate of <1%, 1–49%, ≥50% was 56.8%, 20.5% and 15.9%, respectively. The range of the PD-L1 TPS was 0–75%, in which the median TPS was 0.
The mRNA derived from TEP showed a mild inverse relationship with PD-L1 TPS (Figure 1). There was no significant correlation between the TEP-derived mRNA of PD-L1 and tumoral PD-L1 expression based on the results of the Pearson Correlation test (r=−0.19, P=0.233). For those with positive tumoral PD-L1 (TPS ≥1%), the correlation was also insignificant (r=−0.066, P=0.8).
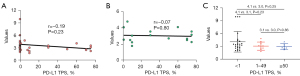
PD-L1 mRNA predicts patient survival after immunotherapy
Based on the median of TEP-derived PD-L1 mRNA, all patients were allocated to the high PD-L1 group (n=36) or the low PD-L1 group (n=36). Table 2 shows patient background and the effect of ICI treatment for each group. In the high PD-L1 group, 16 patients achieved PR, which was more than five patients in the low PD-L1 group (44.4% vs. 13.9%, P<0.01). We also found that 19 patients (44.4%) responded to immunotherapy (PR or PFS >6 months) in the high PD-L1 group, but only five patients responded to immunotherapy in the low PD-L1 group (52.8% vs. 13.9%, P<0.01).
Table 2
Characteristics | Total (n=72) | High PD-L1 group (n=36) | Low PD-L1 group (n=36) | P value (χ2 test) |
---|---|---|---|---|
Gender | 0.414 | |||
Male | 54 | 29 | 25 | |
Female | 18 | 7 | 11 | |
Age (years) | 61 [42–75] | 61 [48–75] | 60 [42–72] | 0.131 |
Pathology | 0.613 | |||
Adeno | 49 | 23 | 26 | |
Squamous | 23 | 13 | 10 | |
PD-L1 TPS (%) | 0.037 | |||
<1 | 25 | 14 | 11 | |
1–49 | 9 | 3 | 6 | |
≥50 | 7 | 2 | 5 | |
Unknown | 31 | 17 | 14 | |
Treatment line | 0.372 | |||
1st | 14 | 9 | 5 | |
2nd | 58 | 27 | 31 | |
Chemo combined | 0.196 | |||
No | 64 | 31 | 33 | |
Pemetrexed/carboplatin | 5 | 2 | 3 | |
Paclitaxel/carboplatin | 3 | 3 | 0 | |
Response | 0.019 | |||
Partial response | 21 | 16 | 5 | |
Stable disease | 24 | 7 | 17 | |
<6 months | 7 | 4 | 3 | |
≥6 months | 17 | 3 | 14 | |
Progressive disease | 23 | 11 | 12 | |
Unevaluable | 4 | 2 | 2 |
Data are presented as n or median [range]. ICI, immune checkpoint inhibitor; PD-L1, programmed death ligand-1; mRNA, messenger RNA; TPS, tumor proportion score.
As Figure 2 shows, the median PFS of the low PD-L1 group was significantly shorter than that of the high PD-L1 group (median PFS: 2.8 vs. 8.3 months, P<0.001, Figure 2A). The median OS was not reached in the high PD-L1 group, while it was 13.5 months in the low PD-L1 group (P=0.0047, Figure 2B). For the patients who were treated with immunotherapy alone, a similar PFS advantage was observed in the high PD-L1 group (2.8 vs. 8.0 months, P=0.002, Figure 2C). In the Figure 2D, the median OS of patients treated only with immunotherapy in the high PD-L1 group was greater than that in the low PD-L1 group (P=0.0126, Figure 2D). What is more, the median PFS of the high PD-L1 group with negative TPS, was also significantly longer than that of the low PD-L1 group (P=0.0074, Figure 2E). In the population, the median OS was not reached in the high PD-L1 group, while it was 13.47 months in the low PD-L1 group (P=0.0344, Figure 2F).
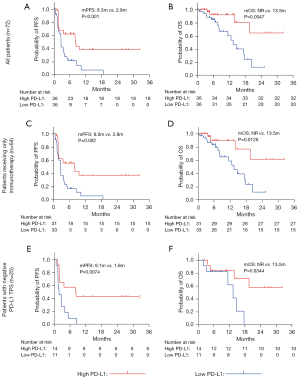
We also analyzed the difference in the expression of TEP-derived mRNA across different clinical responses (Figure 3). We found that patients with clinical benefit (PR or PFS >6 months) had higher TEP-derived mRNA expression than the other patients (median: 2.97 vs. 3.44 months, P=0.012).
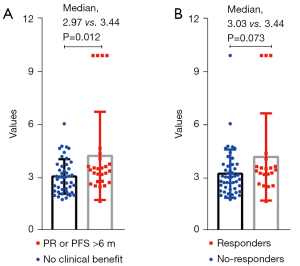
Univariate analysis showed that female, lines of therapy are associated with worse prognosis, while high expression level of TEP-derived mRNA relates to longer survival (Tables S1,S2). By using Cox’s hazard regression model, we found that the pathologic type of NSCLC, expression of PD-L1 protein and high expression level of TEP-derived mRNA constitute the independent risk factors.
Discussion
In the era of immunotherapy, biomarkers are becoming increasingly important in patient selection to avoid unnecessary costs and toxicity (23). To date, most immunotherapy biomarkers took only tumor biological information (e.g., the TPS, TMB, or mismatch repair) or host factors (e.g., patient smoking status or immune cell PD-L1) into account (23,24). However, the therapeutic effects of ICIs are obviously affected by factors related both to the cancer and patient. In the present study, we showed that TEP-derived PD-L1 mRNA could predict the immunotherapy response, PFS, and OS of advanced NSCLC patients.
A substantial information exchange occurs in the circulation between tumor cells, immune cells and circulating platelets (TEPs) (25-27). One study reported that PD-L1 mRNA expression in plasma-derived exosomes could help predict the clinical responses of melanoma or NSCLC patients who received anti-PD-1 therapy (28). Colarusso et al. reported that PD-L1 on platelets represent an easy-to-use clinical approach to predict ICI responsiveness (29). Taken together, the predictive value of TEP PD-L1 in immunotherapy is supported by its biological process and related research findings (including those of the present study). TPSs are commonly used in clinic to guide ICI treatment (e.g., the companion diagnostic antibody DAKO 22C3 is used for pembrolizumab) (30). However, the predictive value of the TPS has not been validated in combination therapies of some ICIs and chemotherapy. In the present study, we found that TEP-derived PD-L1 was not correlated with the TPS, which suggests that TEP PD-L1 mRNA has independent value in predicting immunotherapy outcomes for advanced NSCLC patients. These findings had strongly promoting influence on current treatment protocols or future research directions of NSCLC. For example, it is worth to study the potential value of TEP PD-L1 mRNA in other treatment, such as targeted therapy or the combination of chemotherapy and immunotherapy. Additionally, it is also valuable to explore the predictive capacity of TEP PD-L1 mRNA in early stage of lung cancer and other cancers.
For the biological mechanism of this observation in the study, we think it may be related to the function of blood platelets. Platelets come from megakaryocytes and circulate in peripheral blood, which plays their strong role in hemostasis (31,32). Meanwhile, platelets have the capacity of to transfer tumor-associated biomolecules, which includes mRNA from cancer (33). Thus, platelets have potential applicability to predict the cancer diagnostics and therapy, which is consistent with our conclusions. However, one study reported that platelet RNA may have small capacity for predicting anti-PD-1 nivolumab therapy (34). The unexpected difference might be due to the distinct races, machines and limited therapeutic.
It is interesting that significant correlation between TEP-derived mRNA and tumoral PD-L1 expression was not detected. There are several reasons possibly as followed. Firstly, it is different for the samples tested. The tumoral PD-L1 expression was tested in archived tissue samples, while TEP-derived mRNA was isolated from blood. Secondly, the study should include more samples to define the conclusion in the study. Thirdly, TEP-derived mRNA and tumoral PD-L1 are distinct matters affected by different conditions. Finally, the time interval between tissue collection and platelet collection was within one year, which might influence the negative correlation. Therefore, it is rational that there was a mild inverse relationship between TEP-derived mRNA and PD-L1 TPS.
The mechanisms of acquired resistance to ICIs have been extensively studied recently (35). For example, overactive PI 3-kinase in cancer and immune-dysregulation was reported to account for immunotherapy resistance (36). We intend to study the relationship between the level of TEP-derived PD-L1 and ICI resistance in the future. This study had several limitations. First, it was a study with a moderate sample size, which limits the confidence in determining the prediction potential of TEP-derived PD-L1. Thus, further research needs to be conducted to confirm the predictive value of TEP-derived PD-L1. Second, we did not compare it with other biomarkers, such as the TMB or exosome PD-L1. Third, we did not investigate the presence of PD-L1 in blood platelets using fluorescence in situ hybridization (FISH)/confocal microscopy. However, these limitations do not affect the conclusions of this study. Nevertheless, this study identified a surrogate biomarker that can be used in future studies to help select or stratify patients undergoing different immunotherapies. Further, our present study showed that TEP could be used in liquid biopsy-based analyses and thus guide clinical decisions about immunotherapy in advanced NSCLC patients.
Conclusions
In summary, this article presented the first data of TEP-derived PD-L1 mRNA of advanced NSCLC patients who underwent immunotherapy and showed the potential advantage of using it as a surrogate biomarker to predict PFS and OS following immunotherapy.
Acknowledgments
Funding: This work was supported financially by
Footnote
Reporting Checklist: The authors have completed the REMARK reporting checklist. Available at https://tlcr.amegroups.com/article/view/10.21037/tlcr-24-29/rc
Data Sharing Statement: Available at https://tlcr.amegroups.com/article/view/10.21037/tlcr-24-29/dss
Peer Review File: Available at https://tlcr.amegroups.com/article/view/10.21037/tlcr-24-29/prf
Conflicts of Interest: All authors have completed the ICMJE uniform disclosure form (available at https://tlcr.amegroups.com/article/view/10.21037/tlcr-24-29/coif). S.W. receives payment or honoraria from Lilly, Novartis Pharma, Chugai Pharma, Bristol-Myers, Ono Pharmaceutical, Daiichi Sankyo, Taiho Pharmaceutical, Nippon Kayaku, Kyowa Kirin, Merck, Takeda Pharmaceutical, Celltrion and AstraZeneca. D.Z. and Z.G. are from Yunying Medicine Inc., Shanghai, China. The other authors have no conflicts of interest to declare.
Ethical Statement: The authors are accountable for all aspects of the work in ensuring that questions related to the accuracy or integrity of any part of the work are appropriately investigated and resolved. This study was performed in accordance with the principles of the Declaration of Helsinki (as revised in 2013). Approval was granted by the Ethics Committee of Fudan University Shanghai Cancer Center (Date 2012-12-24; No. 050432-4-1212B). Informed consent was obtained from all the individual participants included in the study.
Open Access Statement: This is an Open Access article distributed in accordance with the Creative Commons Attribution-NonCommercial-NoDerivs 4.0 International License (CC BY-NC-ND 4.0), which permits the non-commercial replication and distribution of the article with the strict proviso that no changes or edits are made and the original work is properly cited (including links to both the formal publication through the relevant DOI and the license). See: https://creativecommons.org/licenses/by-nc-nd/4.0/.
References
- Hirsch FR, Scagliotti GV, Mulshine JL, et al. Lung cancer: current therapies and new targeted treatments. Lancet 2017;389:299-311. [Crossref] [PubMed]
- Molina JR, Yang P, Cassivi SD, et al. Non-small cell lung cancer: epidemiology, risk factors, treatment, and survivorship. Mayo Clin Proc 2008;83:584-94. [Crossref] [PubMed]
- Duma N, Santana-Davila R, Molina JR. Non-Small Cell Lung Cancer: Epidemiology, Screening, Diagnosis, and Treatment. Mayo Clin Proc 2019;94:1623-40. [Crossref] [PubMed]
- Herbst RS, Morgensztern D, Boshoff C. The biology and management of non-small cell lung cancer. Nature 2018;553:446-54. [Crossref] [PubMed]
- Liao D, Yu L, Shangguan D, et al. Recent Advancements of Monotherapy, Combination, and Sequential Treatment of EGFR/ALK-TKIs and ICIs in Non-Small Cell Lung Cancer. Front Pharmacol 2022;13:905947. [Crossref] [PubMed]
- Sukumar J, Gast K, Quiroga D, et al. Triple-negative breast cancer: promising prognostic biomarkers currently in development. Expert Rev Anticancer Ther 2021;21:135-48. [Crossref] [PubMed]
- Cristescu R, Mogg R, Ayers M, et al. Pan-tumor genomic biomarkers for PD-1 checkpoint blockade-based immunotherapy. Science 2018;362:eaar3593. [Crossref] [PubMed]
- Chen PL, Roh W, Reuben A, et al. Analysis of Immune Signatures in Longitudinal Tumor Samples Yields Insight into Biomarkers of Response and Mechanisms of Resistance to Immune Checkpoint Blockade. Cancer Discov 2016;6:827-37. [Crossref] [PubMed]
- Hugo W, Zaretsky JM, Sun L, et al. Genomic and Transcriptomic Features of Response to Anti-PD-1 Therapy in Metastatic Melanoma. Cell 2016;165:35-44. [Crossref] [PubMed]
- McGranahan N, Furness AJ, Rosenthal R, et al. Clonal neoantigens elicit T cell immunoreactivity and sensitivity to immune checkpoint blockade. Science 2016;351:1463-9. [Crossref] [PubMed]
- Garon EB, Rizvi NA, Hui R, et al. Pembrolizumab for the treatment of non-small-cell lung cancer. N Engl J Med 2015;372:2018-28. [Crossref] [PubMed]
- Ramundo V, Palazzo ML, Aldieri E. TGF-β as Predictive Marker and Pharmacological Target in Lung Cancer Approach. Cancers (Basel) 2023;15:2295. [Crossref] [PubMed]
- Nagasaka M, Uddin MH, Al-Hallak MN, et al. Liquid biopsy for therapy monitoring in early-stage non-small cell lung cancer. Mol Cancer 2021;20:82. [Crossref] [PubMed]
- Chen K, Yang F, Shen H, et al. Individualized tumor-informed circulating tumor DNA analysis for postoperative monitoring of non-small cell lung cancer. Cancer Cell 2023;41:1749-1762.e6. [Crossref] [PubMed]
- Fujii T, Naing A, Rolfo C, et al. Biomarkers of response to immune checkpoint blockade in cancer treatment. Crit Rev Oncol Hematol 2018;130:108-20. [Crossref] [PubMed]
- Osmani L, Askin F, Gabrielson E, et al. Current WHO guidelines and the critical role of immunohistochemical markers in the subclassification of non-small cell lung carcinoma (NSCLC): Moving from targeted therapy to immunotherapy. Semin Cancer Biol 2018;52:103-9. [Crossref] [PubMed]
- Varkey J, Nicolaides T. Tumor-Educated Platelets: A Review of Current and Potential Applications in Solid Tumors. Cureus 2021;13:e19189. [Crossref] [PubMed]
- Hinterleitner C, Strähle J, Malenke E, et al. Platelet PD-L1 reflects collective intratumoral PD-L1 expression and predicts immunotherapy response in non-small cell lung cancer. Nat Commun 2021;12:7005. [Crossref] [PubMed]
- Waqar W, Asghar S, Manzoor S. Platelets' RNA as biomarker trove for differentiation of early-stage hepatocellular carcinoma from underlying cirrhotic nodules. PLoS One 2021;16:e0256739. [Crossref] [PubMed]
- Egan K, Crowley D, Smyth P, et al. Platelet adhesion and degranulation induce pro-survival and pro-angiogenic signalling in ovarian cancer cells. PLoS One 2011;6:e26125. [Crossref] [PubMed]
- Best MG, Sol N, Kooi I, et al. RNA-Seq of Tumor-Educated Platelets Enables Blood-Based Pan-Cancer, Multiclass, and Molecular Pathway Cancer Diagnostics. Cancer Cell 2015;28:666-76. [Crossref] [PubMed]
- Joosse SA, Pantel K. Tumor-Educated Platelets as Liquid Biopsy in Cancer Patients. Cancer Cell 2015;28:552-4. [Crossref] [PubMed]
- Walk EE, Yohe SL, Beckman A, et al. The Cancer Immunotherapy Biomarker Testing Landscape. Arch Pathol Lab Med 2020;144:706-24. [Crossref] [PubMed]
- Teng F, Meng X, Kong L, et al. Progress and challenges of predictive biomarkers of anti PD-1/PD-L1 immunotherapy: A systematic review. Cancer Lett 2018;414:166-73. [Crossref] [PubMed]
- Xu XR, Yousef GM, Ni H. Cancer and platelet crosstalk: opportunities and challenges for aspirin and other antiplatelet agents. Blood 2018;131:1777-89. [Crossref] [PubMed]
- Contursi A, Fullone R, Szklanna-Koszalinska P, et al. Tumor-Educated Platelet Extracellular Vesicles: Proteomic Profiling and Crosstalk with Colorectal Cancer Cells. Cancers (Basel) 2023;15:350. [Crossref] [PubMed]
- Pankowska KA, Będkowska GE, Chociej-Stypułkowska J, et al. Crosstalk of Immune Cells and Platelets in an Ovarian Cancer Microenvironment and Their Prognostic Significance. Int J Mol Sci 2023;24:9279. [Crossref] [PubMed]
- Del Re M, Marconcini R, Pasquini G, et al. PD-L1 mRNA expression in plasma-derived exosomes is associated with response to anti-PD-1 antibodies in melanoma and NSCLC. Br J Cancer 2018;118:820-4. [Crossref] [PubMed]
- Colarusso C, Falanga A, Terlizzi M, et al. High levels of PD-L1 on platelets of NSCLC patients contributes to the pharmacological activity of Atezolizumab. Biomed Pharmacother 2023;168:115709. [Crossref] [PubMed]
- Mok TSK, Wu YL, Kudaba I, et al. Pembrolizumab versus chemotherapy for previously untreated, PD-L1-expressing, locally advanced or metastatic non-small-cell lung cancer (KEYNOTE-042): a randomised, open-label, controlled, phase 3 trial. Lancet 2019;393:1819-30. [Crossref] [PubMed]
- George JN. Platelets. Lancet 2000;355:1531-9. [Crossref] [PubMed]
- Leslie M. Cell biology. Beyond clotting: the powers of platelets. Science 2010;328:562-4. [Crossref] [PubMed]
- Calverley DC, Phang TL, Choudhury QG, et al. Significant downregulation of platelet gene expression in metastatic lung cancer. Clin Transl Sci 2010;3:227-32. [Crossref] [PubMed]
- Muller M, Best MG, van der Noort V, et al. Blood platelet RNA profiles do not enable for nivolumab response prediction at baseline in patients with non-small cell lung cancer. Tumour Biol 2023; Epub ahead of print. [Crossref]
- Schoenfeld AJ, Hellmann MD. Acquired Resistance to Immune Checkpoint Inhibitors. Cancer Cell 2020;37:443-55. [Crossref] [PubMed]
- Hennessy BT, Smith DL, Ram PT, et al. Exploiting the PI3K/AKT pathway for cancer drug discovery. Nat Rev Drug Discov 2005;4:988-1004. [Crossref] [PubMed]