Promising preclinical models for lung cancer research—lung cancer organoids: a narrative review
Introduction
Major improvements have been made in lung cancer treatment with the emergence of molecular targeted therapies and immunotherapies (1-3). The presence of an oncogenic driver mutation, such as epidermal growth factor receptor (EGFR) and anaplastic lymphoma kinase (ALK), renders a tumor sensitivity to target tyrosine kinase inhibition (4,5). In addition, with the emergence of immune checkpoint inhibitors (ICIs), significant survival benefits have been produced in the treatment of some advanced lung cancer and cancer therapy has been greatly enriched (6,7). Despite the huge improvement, identifying new therapeutic targets and overcoming drug resistance remain a big challenge. Therefore, it is crucial to undertake cancer biology and translational research via in vitro and in vivo model systems that can accurately recapitulate patients’ tumors.
Conventional 2D cell lines are easy to obtain and culture, and they represent the widely used in vitro preclinical model which is used for basic research and anti-tumor drug testing. Up to now, more than 300 lung cancer cell lines have been established in vitro via a culture system (8). Yet, a large number of studies using cell line models with dramatic success eventually failed in clinical trials. The reason might be related to the inherent genetic drift during the process of passage, and clonal selection is usually performed in fast-growing tumor populations (9). Therefore, tumor heterogeneity and actual drug response of parental tumor might not be able to be reflected. By contrast, in vivo patient-derived xenograft (PDX) models generated from patient tumor tissue implantation in immunodeficient or humanized mice can better retain tumor heterogeneity and mimic tumor microenvironment (TME). However, the establishment of PDX models is more time-consuming and costly (10,11).
To address these problems, 3D biomimetic in vitro models have been sequentially developed, with which patient-derived tumor spheroids have been the first studied. Through 3D cell culture in vitro, cancer cells can aggregate into microsized spherical structures, mimicking histological structures and growth kinetics of solid tumors to a certain extent. On this basis, patient-derived organoid models have been developed to further maintain the characteristics of the primary tumor. Through using specific animal-derived proteinaceous extracellular matrix (ECM) gels and growth factors cocktails, self-organization and self-assembly of the organoids can be guided. Currently, 3D organoid culture technologies have emerged as a relatively low-cost and representative platform to model cancer heterogeneity (12-14). Though several studies have reported the successful experience of lung cancer organoids (LCOs) generation, the culturing methods and conditions remain immature. In this review, we mainly focused on the culturing method of LCOs, including tissue collection, culture medium, and procession, all of these are the key factors to the success. Besides, we also discussed the big challenges and application of LCOs. We present this article in accordance with the Narrative Review reporting checklist (available at https://tlcr.amegroups.com/article/view/10.21037/tlcr-23-341/rc).
Methods
This review consists of relevant data of LCOs searched in the PubMed database, of which “lung cancer” and “organoid” were used as the search terms. In addition, the literatures we reviewed were published in English in the recent ten years and the process for identifying the literature search is listed in Table 1.
Table 1
Items | Specification |
---|---|
Date of search | May, 2023 |
Databases and other sources searched | PubMed |
Search terms used | Lung cancer, organoid |
Timeframe | From 2013 until 2023 |
Inclusion criteria | Literature in English were all included |
Selection process | Studies were initially screened by X.C. and L.Y., reviewed by Y.H. and obtained consensus via discussion among all authors |
Methodology of LCOs
The LCOs derived from cancer tissues are considered to be able to represent the genetic and epigenetic characteristics of cancer. Currently, one of the most commonly used approaches for organoid generation is the ECM scaffold method (15,16). Briefly, the tumor samples were collected from the clinical sites and minced into pieces (1–2 mm3), followed by incubation with digestive enzymes. Then the dissociated cells and cell clusters were resuspended with cold Matrigel before being seeded in plates, and the Matrigel drops were left solidified and overlaid with tumor-type specific culture medium. After verification by histology and sequencing, further experiments can be performed smoothly (Figure 1).
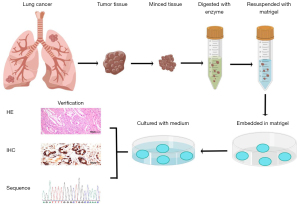
Patient specimen collection
The sufficient number of active cancer cells is the key factor for the success of organoids culture. Most studies used surgically resected samples with 1 to 4 cm3 for harvesting more cancer cells to generate organoids, and the success rate reported previously varied widely, from 40.7% to 100% (17-19). Biopsy specimens with the size of 0.2 to 0.4 cm3 have also been used in some studies, of which the success rate was reported to be only 23% to 28% (20,21). Besides, lymph node metastases and malignant pleural effusions have also been used to generate organoids (22-24).
Tissue digestion
The final number of active cells mainly depends on the samples after disaggregation. Enzymatic digestion is the most common way in several studies, and few studies chose mechanical processing. To obtain more active cells, the digestive enzyme cocktail and digestion time are particularly important. Collagenase, neutral proteases, and elastase hyaluronidase were the most commonly used enzymes for tissues (25). By using digestive enzymes, the attachments between cells and ECM can be cleaved, and there is no need to pursue a state of excessive digestion. Cattaneo et al. reported that the ideal digestion condition was a mix of 70% single cells and 30% clusters of 7–10 cells (26). The choice of digestive enzyme used for detaching cells mainly includes trypsin and trypLE (an analogue of trypsin). Although trypsin with a stronger enzymolysis effect has limited adverse effects on cell characteristics, the use of trypLE was more common in organoid digestion, because its milder effect can avoid overtrypsinization (27,28). The total digestion time mainly depends on the sample size and tumor type, and generally, it may vary from 15 to 30 minutes for biopsies and 45 to 60 minutes for surgical resections. We should avoid overtrypsinization as much as possible, and check the status of the digestion by using a microscope to evaluate whether the tissue has dissociated into single cells.
Culture system
To mimic the tissue conditions the original tumor growing in, the culture system of LCOs commonly consists of ECM and culture medium containing various growth factor cocktails. The obtained single tumor cells or cell clusters are plated in the 3D ECM hydrogel such as basement membrane extract (BME), which was used as an artificial lamina propria in organoid models inspired by an approach pioneered by Lee et al. (29). Matrigel or other BME both are commonly used ECM protein mix, a complex mixture of different ECM proteins including laminin, fibronectin, collagen and heparin sulfate proteoglycans, which can maintain necessary cell-ECM interactions (30). As for culture medium, it usually consists of various supplements, such as B27, N-acetylcysteine, nicotinamide, noggin, WNT/R-spondins, epidermal growth factor (EGF), and fibroblast growth factor (FGF), all of which are associated with cell survival and stemness/differentiation balance (21,31). Therefore, understanding the function of these supplements is essential for LCO generation (Table 2) (21).
Table 2
Supplements | Signaling pathway | Function |
---|---|---|
B27 supplement | A serum-free culture supplement | Increase survival rate of tumorspheres and prevention of adherences |
Improve the sphere-forming efficiency and sustain the propagation of tumor spheres | ||
N2 supplement | A serum-free culture supplement | Maintain an undifferentiated state of organoids |
N-acetylcysteine | Antioxidant | Protect cells from severe levels of stress |
Nicotinamide | Co-enzyme precursor | Increase the efficiency of organoids differentiation |
SB202190 | p38 MAPK signaling | Protect cells from environmental stress-induced apoptosis |
Y-27632 | Inhibition of ROCK signaling | Prevents anoikis-induced cell death |
A83-01 | Inhibition of TGF-β/Smad signaling | Promote the proliferation of stem cells |
Maintain the undifferentiated state of stem cells | ||
Noggin | Inhibition of TGF-β/Smad signaling | Promote the proliferation of stem cells |
Maintain the undifferentiated state of stem cells | ||
R-spondin-1 | Wnt/β-catenin signaling | Maintain the stemness and promote the self-renewal of stem cells |
Wnt 3A | Wnt/β-catenin signaling | Maintain the stemness and promote the self-renewal of stem cells |
FGF-2 | FGFR1c, 3c signaling | Keep the survival of organoids |
FGF-4 | FGFR1c, 2c signaling | Keep the survival of organoids |
Maintain the stemness of stem cells | ||
FGF-7 | FGFR2b signaling | Induce organoid branching |
FGF-10 | FGFR2b signaling | Promote differentiation of lung stem cells |
Inducer of lung organoid formation and branching | ||
EGF | PI3K, ERK1/2, JAK/STAT, β-catenin, and calcium signaling | Drive proliferation of organoids |
IGF-1 | IGF-1R signaling | Keep the survival of organoids |
Nutlin-3a | Inhibition of P53/MDM2 signaling | Select TP53 mutated cancer organoids |
Smoothened agonist | Hedgehog signaling | Promote differentiation of stem cells |
CHIR 99021 | Inhibition of GSK-3β signaling | Promote self-renewal of stem cells |
Prostaglandin E2 | E series prostaglandin receptors signaling | Keep the survival of organoids |
Dexamethasone | Corticosteroid receptor signaling | Induces alveolar maturation and increases the versatility of lung organoids. |
Forskolin | cAMP signaling | Promote cell proliferation |
FGF, fibroblast growth factor; EGF, epidermal growth factor.
Verification and characterization of LCOs
Once organoids are established, the next important thing is to identify the organoids for further experiments. Evaluating the similarity between LCOs and the primary tumor is critical for the value of LCOs. Several approaches for organoid validations have been proposed to verify the characteristics of LCOs. These include histomorphology, immunohistochemistry, and genetic profiles. Firstly, hematoxylin-and-eosin (H&E) stains can be used to assess the morphological features which consist of the major structure of LCOs and the morphology of the cells. The study by Dijkstra et al. demonstrated that normal lung airway organoids (LAOs) grew as relatively well-organized cystic structures, while the LCOs grew mostly as irregular solid structures. However, they found that a few LCOs can be both cystic or solid (18). Therefore, it is not enough to evaluate the LCOs via histomorphological features alone. Immunohistochemistry (IHC) is another useful method to further aid in the development of classification. Thyroid transcription factor 1 (TTF-1), cytokeratin 7 (CK7), tumor protein 63 (p63), and CK5/6 were the most commonly used markers for IHC (32). In the study of Dijkstra et al., the authors further suggested that the loss of polarized p63 staining pattern can be used to differentiate LAOs from LCOs (18). In addition, in clinical practice, these markers can be used to distinguish the subtypes of lung cancer, especially for lung adenocarcinoma (LUAD) and lung squamous cell carcinoma (LUSC), where LUAD usually shows positive TTF-1 and CK7, and LUSC shows positive p63 and CK5/6.
Genetic profiles help to further describe the characteristics of established LCOs compared with primary tumors. Dijkstra and colleagues first generated copy number (CN) profiling to distinguish normal LAOs and LCOs (18). The result showed that CN was normal in LAOs, while most of the primary non-small cell lung cancer (NSCLC) samples showed multiple gains and losses. Besides, whole exome sequencing (WES), whole genome sequencing (WGS), and targeted gene panel sequencing might provide additional molecular information, which can differentiate between tumor and normal organoid as well as confirming the parental accordance and clonal heterogeneity of the LCOs (33). Among them, WGS is considered the most accurate method for identification of the patient-derived organoids due to the access to the whole genome information, but considering the high cost, many researchers have chosen WES as the more cost-effective and less labor-intensive alternative (34). Similarly, detection of commonly mutated genes in lung cancer using a small panel such as EGFR, TP53, KRAS, and so on can save the cost, but some potential discordances between organoid and the original tumor may not be found, given that the tumors may not necessarily carry these mutations. RNA sequencing was another way to identify the gene expression profiles in LCOs (35). We, therefore, recommend a complete verification and characterization of LCOs should include the morphological and IHC assessment, combined with a genetic profile analysis by CN, WES, or RNA sequencing.
Problems and solutions in the LCOs generation
Currently, although researchers are struggling for optimizing the LCO generation procedures, there are a number of problems for these models. Due to the complexity of lung cancer and the immaturity of organoid technology, it is worth thinking during establishment of LCO models about how to determine the composition of conditioned medium, maintain the tumor heterogeneity and shorten the time for organoid preparation.
The choice of conditioned medium for LCO generation
The biggest problem for organoids generation is that normal lung epithelial cells in the lung organoids often grow faster than the lung tumoroids, leading to the failures of LCOs establishment (13,18). To overcome this obstacle, some studies on tumor purifying have been reported. Sachs et al. reported the use of nutlin-3a, an MDM2 inhibitor, to lead airway organoids with TP53 wild-type into apoptosis, and at the same time, to allow the outgrowth of tumoroids with mutant TP53 (20). Moreover, it has been confirmed that deprivation of specific growth factor based on the culture medium for normal airway can avoid the normal cell contamination, and subsequently increase the survival rate of organoids generation (36). Yokota et al. have compared the growth of lung tumoroids in the LAO medium developed by the Clevers laboratory with those in other LCO culture media reported by the other three laboratories (37). Ultimately, the author found that LAO medium with nutlin-3a was superior to other different media. However, only approximately 50–60% of lung cancer patients harbor the TP53 mutation (38), of which might not be suitable for the generation of LCOs with wild TP53. To avoid eradication of the LCOs without TP53 mutation, Ebisudani et al. prepared a second cancer-selective condition based on nutlin-3a, where they halted ERBB signaling by removing EGF/insulin growth factor-1 (IGF-1)/FGF-2 and adding a pan-ERBB inhibitor to restrict normal airway and alveolar organoids growing and obtain pure LCOs (39). Through the investigation of genotype-phenotype correlations, they found that LCOs of different histologic subtypes exhibited different EGFR pathway dependency and drug sensitivity. All in all, it remains unknown whether all growth factors would be required for each LCO and the culture medium formulations from different laboratories are still immature, due to the complexity of histology and genetic background of lung cancer. With the deepening understanding of organoid culture technology, the improvements and adjustments for culture systems are increasing, and the different LCO systems published are compared in Table 3.
Table 3
Reference | Sample | Histology | Medium/ECM | Supplements | Success rate | Applications |
---|---|---|---|---|---|---|
Pauli et al. 2017 (33) | Resection/biopsy | LUAD | DMEM/Matrigel | B27, N-acetylcysteine, R-spondin-1, noggin, FGF-10, FGF-2, EGF, A83-01, Y-27632, SB202190, nicotinamide, prostaglandin E2 | 50% (1/2) | High throughput drug screening |
Dijkstra et al. 2018 (19) | Resection/biopsy | LUAD/LUSC/other | DMEM/F12/Geltrex | B27, N-acetylcysteine, R-spondin-1, noggin, FGF-10, FGF-7, A83-01, Y-27632, SB202190, nicotinamide, nutlin-3a | 100% (6/6) | Induce and analyze tumor-specific T cell responses via organoids co-cultured with PBMC |
Sachs et al. 2019 (20) | Resection/biopsy | NSCLC | DMEM/F12/BME | B27, N-acetylcysteine, R-spondin-1, noggin, FGF-10, FGF-7, A83-01, Y-27632, SB202190, nicotinamide, nutlin-3a | • Resections of primary NSCLC: 88% (14/16) | Long-term expansion of LCOs, validation, and drug testing |
• Biopsies of metastatic NSCLC: 28% (5/18) | ||||||
Kim et al. 2019 (40) | Resection | LUAD/LUSC/SCLC/LCNEC | DMEM/F12/Matrigel | B2, N2, FGF-2, EGF, ROCK inhibitor | 87% (20/23) | Established a living biobank of 80 lung cancer organoids |
Dijkstra et al. 2020 (18) | Resection/biopsy | LUAD/LUSC/SCLC/LCNEC/others | DMEM/F12/Geltrex | B27, N-acetylcysteine, R-spondin-1, noggin, FGF-10, FGF-7, A83-01, Y-27632, SB202190, nicotinamide | 24/59 (40.7%) | Evaluation of methods to determine tumor purity of organoids of lung cancer organoids |
Li et al. 2020 (17) | Resection | LUAD | DMEM/F12/Matrigel | B27, N-acetylcysteine, R-spondin-1, noggin, FGF-10, FGF-7, A83-01, Y-27632, SB202190, nicotinamide | 12/15 (80%) | Established a living biobank of lung cancer organoids and undertaken high throughput drug screening |
Shi et al. 2020 (35) | Resection/PDX | LUAD/LUSC | DMEM/F12/Matrigel | B27 supplement, N-acetylcysteine, noggin, FGF-10, FGF-4, EGF, A83-01, Y-27632, CHIR-99021, SAG | • Organoids maintained in short-term culture: 72% (47/65) | Established a protocol for the development of NSCLC organoids and may be useful for future drug screening |
• Organoids maintained in long-term culture: 15% (10/65) | ||||||
Kim et al. 2021 (41) | Resection/pleural effusion | LUAD | DMEM/F12/Matrigel | B27, R-spondi-1, FGF-7, FGF-10, noggin, A83-01, SB202190, Y- 27632 | 83% (83/100) | Examined the ability of lung cancer organoids to predict clinical responses |
Hu et al. 2021 (36) | Resection/biopsy | LUAD/LUSC/SCLC/others | DMEM/F12/Matrigel | B27 N2, nicotinamide, N-acetylcysteine, Y-27632, EGF, SB202190, A83-01, Forskolin, Dexamethasone | 78.6% (81/103) | Establishing Lung cancer organoids analyzed on microwell arrays predict drug responses |
Yokota et al. 2021 (37) | Resection/biopsy | LUAD/LUSC/SCLC/others | DMEM/F12/Matrigel | B27, N-acetylcysteine, R-spondin-1, noggin, FGF-10, FGF-7, A83-01, Y-27632, SB202190, nicotinamide, nutlin-3a | • Organoid/tumoroid formations: 82.9% (34/41) | Comparing different culture mediums to determine which tumoroid culture system is most efficient |
• Tumoroid lines for long-term culture: 7% (3/41) | ||||||
Wang et al. 2023 (24) | Malignant pleural effusion/resection/biopsy | LUAD/LUSC/SCLC/ASC/PSC | DMEM/F12/Matrigel | B27, N2, EGF, FGF, Y-27632 | 74.8% (160/214) | Prediction of locally advanced or metastatic lung cancer tumor in response to chemotherapy and targeted therapy |
Ebisudani et al. 2023 (39) | Malignant pleural effusion/resection/biopsy/circulating tumor cells/sputum | LUAD/LUSC/SCLC/LCNEC | DMEM/F12/Matrigel | B27, N-acetylcysteine, EGF, IGF-1, FGF-2, R-spondin-1, noggin, A83-01, Wnt 3A, A83-01, Y-27632 | Malignant pleural effusion/resection/biopsy: 17.8% (35/197) | Established a biobank of lung cancer organoids for investigation of genotype-phenotype mapping |
LCOs enrichment: nutlin-3a, pan-ErbB inhibitor, remove of EIF (EGF, IGF-1 and FGF-2) | Circulating tumor cells: 8.3% (3/36) | |||||
Sputum: 20% (5/25) |
LCO, lung cancer organoid; ECM, extracellular matrix; LUAD, lung adenocarcinoma; DMEM, Dulbecco’s modified eagle medium; FGF, fibroblast growth factor; EGF, epidermal growth factor; LUSC, lung squamous cell carcinoma; PBMC, peripheral blood mononuclear cell; NSCLC, non-small cell lung cancer; BME, basement membrane extract; SCLC, small cell lung cancer; LCNEC, large cell neuroendocrine carcinoma; PDX, patient-derived xenograft; SAG, Smoothened agonist; ASC, adenosquamous carcinoma; PSC, pulmonary sarcomatoid carcinoma; IGF, insulin growth factor.
Maintenance of the tumor heterogeneity
It has been shown that organoids can capture tumor heterogeneity and serve as an ex vivo platform for personalized treatment, though this model cannot fully reflect the disease in vivo (42). According to the results of genomic profiling in LCO research, the discordance between the organoid and the original tumor is usually obvious but acceptable for recapitulating primary tumors. Roerink et al. have reported that the colorectal cancer (CRC) organoids generated from different tumor clones of the same tumor exhibited obvious genetic diversification and different responsiveness to therapy (43), indicating that intra-tumor diversification might be a reason for the discordance. Moreover, the ongoing normal tumor evolution and the heterogeneous organoid cultures for tumor selection might also lead to the discordance, so the use of low passage cultures can minimize the effect of potential in vitro selection by media or accumulation of in vitro mutations. To confirm the obtained organoid with maintenance of the original tumor heterogeneity, genetic characterization is necessary.
Long time for LCO generation
Currently, the lengthy time in generating LCOs hampers the application of organoids in clinical practice, especially for drug sensitivity tests to predict the drug response, which requires prolonged in vitro expansion to generate enough quantities of LCOs and obtain quick responses for drugs. However, a few weeks or even months are required for the current drug test. Recently, Hu et al. by employing an integrated superhydrophobic microwell array chip (InSMARchip), demonstrated that LCOs, even at passage 0, can be used to perform one-week drug tests, which holds great promise for clinical drug sensitivity screening in vitro (36). For future studies, more efforts should be made to improve the culture technique to shorten the time.
Application and limitation of LCOs
Drug sensitive screening
Currently, common preclinical models used for drug screening in lung cancer therapy include 2D cell culture, spheroids, organoids, and xenografts. Patient-derived tumor cell lines grown in 2D cultures are ideal for studying single cancer cells in response to drugs via rapid and long-term expansion with low costs and high reproducibility, but they cannot fully recapitulate the biological in vivo interaction within the entire human body and the genetic background of parental tumors. As for the 3D tumor spheroids model, it can further maintain parental characteristics and predict treatment response compared to cell lines model, attributed to its high number of cell-cell interactions, cell–ECM interactions, and the gradients of gases, nutrients, and pH from inner to outer. Organoids model as a special 3D model with higher thresholds and costs for generation is capable of recapitulating primary tumors more accurately, which is similar to the PDX model. As for the PDX model with the transplanted patient-derived tumor tissue growing in the biological system of model animals, it contains more complete TME, which may give the treatment response closer to human body compared to the organoids model. However, the PDX model is unsuitable for large-scale drug screening due to their time-consuming nature and high costs (44,45). In conclusion, the high similarity to their parental tumors in respect of phenotypes and genotypes as well as the possibility of rapid and long-term expansion, make LCOs more suitable for the high-throughput drug screening.
To date, only a few studies have reported on the use of organoids from primary NSCLC or metastatic sites for drug screening. Sachs et al. demonstrated that LCOs are amenable to drug screening, and differential responses of NSCLC organoids to conventional chemotherapeutics such as cisplatin or paclitaxel, but also showed sensitivity towards the tyrosine kinase inhibitors (TKIs) such as erlotinib and gefitinib in ERBB2-mutant organoids (20). In the same year, another study by Kim et al. established a biobank of 80 LCOs and found a BRCA2-mutant NSCLC responded to olaparib, an EGFR-mutant LCOs responded to erlotinib and an EGFR-mutant/MET-amplified LCOs responded to crizotinib, which provided a prospect for drug testing in LCOs (40). Shi et al. also verified that KRAS mutant organoids were much more sensitive to the MEK inhibitor (35). Yokota et al. demonstrated that the combination of Bcl-2 inhibitor (navitoclax) and survivin inhibitor (YM-155) might be effective for EGFR-TKI-resistant NSCLC organoids (37). Recently, high-throughput drug screening is considered to be a more efficient way to utilize the advantages of organoids. Li and coworkers established 12 human LUAD derived organoids from 15 patients with different subtypes of LUAD and showed the ability to identify potential biomarkers and to perform high-throughput drug screening with 24 anti-cancer drugs including standard chemotherapy and targeted agents (17).
LCOs for modeling TME
Patient-derived tumor organoids have emerged as a promising model that can maintain the heterogeneity of primary cancers and recapitulate the TME. The TME comprises an immune cellular network, mesenchymal-derived cells (pericytes and fibroblasts), and endothelial cells, which greatly fosters tumor progression and the efficacy of therapeutic responses. As an important means of lung cancer treatment, immunotherapy needs the support of immune cells for its curative effect, pushing the development of corresponding co-culture models mimicking tumor immune microenvironment (TIME). Previously, 2D cell line models usually served as a common approach for exploring the mechanisms of cancer immunology by co-culture with exogenously immune cells, such as those from autologous peripheral blood mononuclear cells (PBMCs) (46-48). However, this approach was mainly based on monolayer cancer cell structures, which is far from the actual situation of TIME. The advent of 3D organoids represented a more ideal in vitro model for studying tumor immunobiology and developing novel approaches for personalized medicine in lung cancer.
Currently, there are two approaches that can be used to add immune cells to the organoid culture system to generate TIME in organoids. One is reconstituted models, in which immune cells, such as those from autologous PBMCs, are isolated and subsequently co-cultured with grown organoids. Dijkstra et al. demonstrated that co-cultures of LCOs and PBMCs could enrich the tumor-reactive T cells from patients with mismatch repair deficient (MRD) CRC and NSCLC, where LCOs were generated from six NSCLC patients and autologous tumor-reactive CD8+ T cells were obtained in two out of six cases (19). Recently, another study reported by Li et al. pointed out that CAR-T cells targeting B7-H3 (B7-H3.CAR) effectively exhibit antitumor activity in vitro against LCOs, and achieved T-cell-mediated targeting of NSCLC brain metastases (49). Another is holistic native TIME models, which preserve primary tumor cells and endogenous immune and non-immune stromal cells. Neal et al. reported an air-liquid interface (ALI) co-culture system that can be used to propagate LCOs (50). In this system, large regions of tumors could be grown in their native state and thus also faithfully preserve a diversity of native syngeneic tumor-reactive tumor-infiltrating lymphocytes, including T cells, B cells, NK cells, and macrophages, while the immune components would decline over time (<30 days), and did not persist beyond 2 months with IL-2 supplementation. Notably, although these LCO platforms have been confirmed with capacities of predicting the responsiveness to immune-checkpoint inhibitors and considered with huge potential for clinical translation, they still need more clinical data to support it.
Besides immune cells, cancer-associated fibroblasts (CAFs) as an equally important component of TME and responsible for the modification of the ECM proteins and secretion of growth factors, were also included in the study of co-culture with LCOs (51,52). Chen et al. (52) reported a 3D coculture system of LUSC organoids with CAFs and ECM that recapitulates the dynamic interactions between LUSC cells and components of the tumor TME. The result showed that CAFs and ECM could override cell intrinsic oncogenic changes in determining the disease phenotype in the context of LUSC and it is a promising model for TME research.
Organ-on-a-chip and organoid-on-a-chip
Human organ chips are microfluidic cell culture devices integrating living human cells with synthetically generated yet physiologically relevant microenvironments, which may simulate the functions of different organs (53). Compared with traditional 2D culture, organ chips emulate one specific function of an organ which closely resembles the architecture of the human body. Organ-on-a-chip technology aims to develop effective microphysiological models for studying physiological events including pulmonary thrombosis and lung inflammation (54-56). However, most organ-on-a-chip models are based on a single organ without cross-organ communication. Hence, establishing multiorgan-on-a-chip systems can incorporate different organ-on-a-chip into a dynamic circulation system, called a “body-on-a-chip” (56). However, organ-on-a-chip systems lack structural or cellular fidelity compared with organoids. Organoids rely on self-assembly for the establishment of organized tissue structure. However, insufficient nutrients and oxygen might limit the growth of organoids as the size and volume become much bigger than before, and eventually leading to cell death. Therefore, combining the advantages of the two technologies, called “organoid-on-a-chip”, can provide a more nutrient and gas exchange to organoids for avoiding cell death and achieving a long-term culture (57,58). Organoid-on-a-chip can serve as a more versatile preclinical model for the broad application of emerging drug discovery processes. Jung et al. presented a one-stop microfluidic device enabling both small cell LCOs culturing and drug sensitivity tests directly on a microphysiological system (59).
Future challenges and perspectives
Increasing evidence has shown that LCOs can recapitulate the true genetic, phenotypic, and morphological diversity of distinct neoplastic cell subclones. Despite being widely used, several challenges remain to be addressed for facilitating their future use in clinical practice.
Due to the lack of a standard process for LCO culture, such as tissue processing and medium formulations, current techniques for LCO culture are inherently uncontrolled and irreproducible (60). One goal for LCOs is to obtain a sufficient number of viable cancer cells and to timely establish the organoids. However, the problems of establishing long-term cultured tumoroids and improving the survival rates of pure LCOs are currently limiting factors for the broad clinical application of LCOs. Hence, available protocols will have to undergo additional optimization procedures to further develop and enhance the methodology. In addition, most of studies established organoids by only including cancer cells but without co-culture of mesenchymal-derived cells, endothelial cells, and immune cells. The mainstream approach for studying the association of organoids and the immune microenvironment is co-cultured organoids with immune cells. However, because TIME cells play diverse roles in cancer organoids, progression, and treatment, which are yet to be studied clearly, it remains unknown which kinds of TIME cells should be added to the organoid system.
Currently, researchers have successfully identified the required or dispensable medium components for cancer organoid culture. However, they mainly focus on some interconnected pathways, and some of the medium components are sorely stratified by the mutational status of tumors. Considering that various subtypes of lung cancer have distinct molecular characteristics and evolutionary processes, future efforts should be made to investigate the tumor-specific medium formulations to better suit organoid growth.
Conclusions
Increasing evidence has demonstrated that LCOs are a promising in vitro model that is suitable for future basic and translational cancer studies. Though there are high expectations for LCOs, we have to face several challenges so that LCOs can be better applied in clinical practice. Currently, the standard culture protocol remains immature. More experiments are required to compare the different culture protocols on the NSCLC with different histological types and genetic backgrounds, which may help to identify potential genotype-specific supplements that improve the success rate of organoid generation. Besides, LCO as an ideal model for the investigation of anti-cancer treatment response in vitro, especially for the immune-checkpoint inhibitors, holds great potential in immunotherapy in lung cancer. Finally, more advanced technology, such as organoid-on-a-chips, which imitates organ development and human diseases, will forge a path toward realizing the considerable promise of therapy response prediction and personalized medicine.
Acknowledgments
Funding: This study was supported in part by a grant of
Footnote
Reporting Checklist: The authors have completed the Narrative Review reporting checklist. Available at https://tlcr.amegroups.com/article/view/10.21037/tlcr-23-341/rc
Peer Review File: Available at https://tlcr.amegroups.com/article/view/10.21037/tlcr-23-341/prf
Conflicts of Interest: All authors have completed the ICMJE uniform disclosure form. (available at https://tlcr.amegroups.com/article/view/10.21037/tlcr-23-341/coif). The authors have no conflicts of interest to declare.
Ethical Statement: The authors are accountable for all aspects of the work in ensuring that questions related to the accuracy or integrity of any part of the work are appropriately investigated and resolved.
Open Access Statement: This is an Open Access article distributed in accordance with the Creative Commons Attribution-NonCommercial-NoDerivs 4.0 International License (CC BY-NC-ND 4.0), which permits the non-commercial replication and distribution of the article with the strict proviso that no changes or edits are made and the original work is properly cited (including links to both the formal publication through the relevant DOI and the license). See: https://creativecommons.org/licenses/by-nc-nd/4.0/.
References
- Howlader N, Forjaz G, Mooradian MJ, et al. The Effect of Advances in Lung-Cancer Treatment on Population Mortality. N Engl J Med 2020;383:640-9. [Crossref] [PubMed]
- Tang Q, Chen Y, Li X, et al. The role of PD-1/PD-L1 and application of immune-checkpoint inhibitors in human cancers. Front Immunol 2022;13:964442. [Crossref] [PubMed]
- Girard N. New Strategies and Novel Combinations in EGFR TKI-Resistant Non-small Cell Lung Cancer. Curr Treat Options Oncol 2022;23:1626-44. [Crossref] [PubMed]
- Tan AC, Tan DSW. Targeted Therapies for Lung Cancer Patients With Oncogenic Driver Molecular Alterations. J Clin Oncol 2022;40:611-25. [Crossref] [PubMed]
- Shea M, Costa DB, Rangachari D. Management of advanced non-small cell lung cancers with known mutations or rearrangements: latest evidence and treatment approaches. Ther Adv Respir Dis 2016;10:113-29. [Crossref] [PubMed]
- Mizuno T, Katsuya Y, Sato J, et al. Emerging PD-1/PD-L1 targeting immunotherapy in non-small cell lung cancer: Current status and future perspective in Japan, US, EU, and China. Front Oncol 2022;12:925938. [Crossref] [PubMed]
- Mok TSK, Wu YL, Kudaba I, et al. Pembrolizumab versus chemotherapy for previously untreated, PD-L1-expressing, locally advanced or metastatic non-small-cell lung cancer (KEYNOTE-042): a randomised, open-label, controlled, phase 3 trial. Lancet 2019;393:1819-30. [Crossref] [PubMed]
- Gazdar AF, Gao B, Minna JD. Lung cancer cell lines: Useless artifacts or invaluable tools for medical science? Lung Cancer 2010;68:309-18. [Crossref] [PubMed]
- Tabassum DP, Polyak K. Tumorigenesis: it takes a village. Nat Rev Cancer 2015;15:473-83. [Crossref] [PubMed]
- Huo KG, D'Arcangelo E, Tsao MS. Patient-derived cell line, xenograft and organoid models in lung cancer therapy. Transl Lung Cancer Res 2020;9:2214-32. [Crossref] [PubMed]
- Schneeberger VE, Allaj V, Gardner EE, et al. Quantitation of Murine Stroma and Selective Purification of the Human Tumor Component of Patient-Derived Xenografts for Genomic Analysis. PLoS One 2016;11:e0160587. [Crossref] [PubMed]
- Drost J, Clevers H. Organoids in cancer research. Nat Rev Cancer 2018;18:407-18. [Crossref] [PubMed]
- Li M, Izpisua Belmonte JC. Organoids - Preclinical Models of Human Disease. N Engl J Med 2019;380:569-79. [Crossref] [PubMed]
- Lou YR, Leung AW. Next generation organoids for biomedical research and applications. Biotechnol Adv 2018;36:132-49. [Crossref] [PubMed]
- Velasco V, Shariati SA, Esfandyarpour R. Microtechnology-based methods for organoid models. Microsyst Nanoeng 2020;6:76. [Crossref] [PubMed]
- Li Z, Yu L, Chen D, et al. Protocol for generation of lung adenocarcinoma organoids from clinical samples. STAR Protoc 2021;2:100239. [Crossref] [PubMed]
- Li Z, Qian Y, Li W, et al. Human Lung Adenocarcinoma-Derived Organoid Models for Drug Screening. iScience 2020;23:101411. [Crossref] [PubMed]
- Dijkstra KK, Monkhorst K, Schipper LJ, et al. Challenges in Establishing Pure Lung Cancer Organoids Limit Their Utility for Personalized Medicine. Cell Rep 2020;31:107588. [Crossref] [PubMed]
- Dijkstra KK, Cattaneo CM, Weeber F, et al. Generation of Tumor-Reactive T Cells by Co-culture of Peripheral Blood Lymphocytes and Tumor Organoids. Cell 2018;174:1586-1598.e12. [Crossref] [PubMed]
- Sachs N, Papaspyropoulos A, Zomer-van Ommen DD, et al. Long-term expanding human airway organoids for disease modeling. EMBO J 2019;38:e100300. [Crossref] [PubMed]
- Ma HC, Zhu YJ, Zhou R, et al. Lung cancer organoids, a promising model still with long way to go. Crit Rev Oncol Hematol 2022;171:103610. [Crossref] [PubMed]
- Mazzocchi A, Devarasetty M, Herberg S, et al. Pleural Effusion Aspirate for use in 3D Lung Cancer Modeling and Chemotherapy Screening. ACS Biomater Sci Eng 2019;5:1937-43. [Crossref] [PubMed]
- Gmeiner WH, Miller LD, Chou JW, et al. Dysregulated Pyrimidine Biosynthesis Contributes to 5-FU Resistance in SCLC Patient-Derived Organoids but Response to a Novel Polymeric Fluoropyrimidine, CF10. Cancers (Basel) 2020;12:788. [Crossref] [PubMed]
- Wang HM, Zhang CY, Peng KC, et al. Using patient-derived organoids to predict locally advanced or metastatic lung cancer tumor response: A real-world study. Cell Rep Med 2023;4:100911. [Crossref] [PubMed]
- Reichard A, Asosingh K. Best Practices for Preparing a Single Cell Suspension from Solid Tissues for Flow Cytometry. Cytometry A 2019;95:219-26. [Crossref] [PubMed]
- Cattaneo CM, Dijkstra KK, Fanchi LF, et al. Tumor organoid-T-cell coculture systems. Nat Protoc 2020;15:15-39. [Crossref] [PubMed]
- Panchision DM, Chen HL, Pistollato F, et al. Optimized flow cytometric analysis of central nervous system tissue reveals novel functional relationships among cells expressing CD133, CD15, and CD24. Stem Cells 2007;25:1560-70. [Crossref] [PubMed]
- Tsuji K, Ojima M, Otabe K, et al. Effects of Different Cell-Detaching Methods on the Viability and Cell Surface Antigen Expression of Synovial Mesenchymal Stem Cells. Cell Transplant 2017;26:1089-102. [Crossref] [PubMed]
- Lee GY, Kenny PA, Lee EH, et al. Three-dimensional culture models of normal and malignant breast epithelial cells. Nat Methods 2007;4:359-65. [Crossref] [PubMed]
- Dutta D, Heo I, Clevers H. Disease Modeling in Stem Cell-Derived 3D Organoid Systems. Trends Mol Med 2017;23:393-410. [Crossref] [PubMed]
- Yang R, Yu Y. Patient-derived organoids in translational oncology and drug screening. Cancer Lett 2023;562:216180. [Crossref] [PubMed]
- Travis WD, Brambilla E, Nicholson AG, et al. The 2015 World Health Organization Classification of Lung Tumors: Impact of Genetic, Clinical and Radiologic Advances Since the 2004 Classification. J Thorac Oncol 2015;10:1243-60. [Crossref] [PubMed]
- Pauli C, Hopkins BD, Prandi D, et al. Personalized In Vitro and In Vivo Cancer Models to Guide Precision Medicine. Cancer Discov 2017;7:462-77. [Crossref] [PubMed]
- Wallaschek N, Niklas C, Pompaiah M, et al. Establishing Pure Cancer Organoid Cultures: Identification, Selection and Verification of Cancer Phenotypes and Genotypes. J Mol Biol 2019;431:2884-93. [Crossref] [PubMed]
- Shi R, Radulovich N, Ng C, et al. Organoid Cultures as Preclinical Models of Non-Small Cell Lung Cancer. Clin Cancer Res 2020;26:1162-74. [Crossref] [PubMed]
- Hu Y, Sui X, Song F, et al. Lung cancer organoids analyzed on microwell arrays predict drug responses of patients within a week. Nat Commun 2021;12:2581. [Crossref] [PubMed]
- Yokota E, Iwai M, Yukawa T, et al. Clinical application of a lung cancer organoid (tumoroid) culture system. NPJ Precis Oncol 2021;5:29. [Crossref] [PubMed]
- Comprehensive molecular profiling of lung adenocarcinoma. Nature 2014;511:543-50. [Crossref] [PubMed]
- Ebisudani T, Hamamoto J, Togasaki K, et al. Genotype-phenotype mapping of a patient-derived lung cancer organoid biobank identifies NKX2-1-defined Wnt dependency in lung adenocarcinoma. Cell Rep 2023;42:112212. [Crossref] [PubMed]
- Kim M, Mun H, Sung CO, et al. Patient-derived lung cancer organoids as in vitro cancer models for therapeutic screening. Nat Commun 2019;10:3991. [Crossref] [PubMed]
- Kim SY, Kim SM, Lim S, et al. Modeling Clinical Responses to Targeted Therapies by Patient-Derived Organoids of Advanced Lung Adenocarcinoma. Clin Cancer Res 2021;27:4397-409. [Crossref] [PubMed]
- Weeber F, van de Wetering M, Hoogstraat M, et al. Preserved genetic diversity in organoids cultured from biopsies of human colorectal cancer metastases. Proc Natl Acad Sci U S A 2015;112:13308-11. [Crossref] [PubMed]
- Roerink SF, Sasaki N, Lee-Six H, et al. Intra-tumour diversification in colorectal cancer at the single-cell level. Nature 2018;556:457-62. [Crossref] [PubMed]
- Zanella ER, Grassi E, Trusolino L. Towards precision oncology with patient-derived xenografts. Nat Rev Clin Oncol 2022;19:719-32. [Crossref] [PubMed]
- Genta S, Coburn B, Cescon DW, et al. Patient-derived cancer models: Valuable platforms for anticancer drug testing. Front Oncol 2022;12:976065. [Crossref] [PubMed]
- Riedl A, Schlederer M, Pudelko K, et al. Comparison of cancer cells in 2D vs 3D culture reveals differences in AKT-mTOR-S6K signaling and drug responses. J Cell Sci 2017;130:203-18. [Crossref] [PubMed]
- Faget J, Biota C, Bachelot T, et al. Early detection of tumor cells by innate immune cells leads to T(reg) recruitment through CCL22 production by tumor cells. Cancer Res 2011;71:6143-52. [Crossref] [PubMed]
- Mo X, Tang C, Niu Q, et al. HTiP: High-Throughput Immunomodulator Phenotypic Screening Platform to Reveal IAP Antagonists as Anti-cancer Immune Enhancers. Cell Chem Biol 2019;26:331-339.e3. [Crossref] [PubMed]
- Li H, Harrison EB, Li H, et al. Targeting brain lesions of non-small cell lung cancer by enhancing CCL2-mediated CAR-T cell migration. Nat Commun 2022;13:2154. [Crossref] [PubMed]
- Neal JT, Li X, Zhu J, et al. Organoid Modeling of the Tumor Immune Microenvironment. Cell 2018;175:1972-1988.e16. [Crossref] [PubMed]
- Gaggioli C, Hooper S, Hidalgo-Carcedo C, et al. Fibroblast-led collective invasion of carcinoma cells with differing roles for RhoGTPases in leading and following cells. Nat Cell Biol 2007;9:1392-400. [Crossref] [PubMed]
- Chen S, Giannakou A, Wyman S, et al. Cancer-associated fibroblasts suppress SOX2-induced dysplasia in a lung squamous cancer coculture. Proc Natl Acad Sci U S A 2018;115:E11671-80. [Crossref] [PubMed]
- Zheng F, Xiao Y, Liu H, et al. Patient-Specific Organoid and Organ-on-a-Chip: 3D Cell-Culture Meets 3D Printing and Numerical Simulation. Adv Biol (Weinh) 2021;5:e2000024. [Crossref] [PubMed]
- Zamprogno P, Wüthrich S, Achenbach S, et al. Second-generation lung-on-a-chip with an array of stretchable alveoli made with a biological membrane. Commun Biol 2021;4:168. [Crossref] [PubMed]
- Hassell BA, Goyal G, Lee E, et al. Human Organ Chip Models Recapitulate Orthotopic Lung Cancer Growth, Therapeutic Responses, and Tumor Dormancy In Vitro. Cell Rep 2017;21:508-16. [Crossref] [PubMed]
- Kratz SRA, Höll G, Schuller P, et al. Latest Trends in Biosensing for Microphysiological Organs-on-a-Chip and Body-on-a-Chip Systems. Biosensors (Basel) 2019;9:110. [Crossref] [PubMed]
- Jantaree P, Bakhchova L, Steinmann U, et al. From 3D Back to 2D Monolayer Stomach Organoids-on-a-Chip. Trends Biotechnol 2021;39:745-8. [Crossref] [PubMed]
- Zhao X, Xu Z, Xiao L, et al. Review on the Vascularization of Organoids and Organoids-on-a-Chip. Front Bioeng Biotechnol 2021;9:637048. [Crossref] [PubMed]
- Jung DJ, Shin TH, Kim M, et al. A one-stop microfluidic-based lung cancer organoid culture platform for testing drug sensitivity. Lab Chip 2019;19:2854-65. [Crossref] [PubMed]
- LeSavage BL, Suhar RA, Broguiere N, et al. Next-generation cancer organoids. Nat Mater 2022;21:143-59. [Crossref] [PubMed]