Immunotherapy for patients with advanced non-small cell lung cancer harboring oncogenic driver alterations other than EGFR: a multicenter real-world analysis
Highlight box
Key findings
• Immune checkpoint inhibitors (ICIs) showed clinical effectiveness in KRAS-mutant advanced non-small cell lung cancer (NSCLC) in the first-line treatment setting or after progression on chemotherapy.
• For advanced NSCLC with ALK, RET, ROS1 rearrangement, MET exon 14 skipping mutation, and BRAF V600E mutation, effectiveness of single or combined ICI therapy remains limited, therefore, targeted therapies should be considered prior to immunotherapy regimens.
What is known and what is new?
• The clinical exploration of various ICI regimens and their sequencing for NSCLC with oncogenic driver alterations, especially rare alterations, is an area of ongoing research.
• We ascertained treatment outcomes of ICI treatment for patients with advanced NSCLC having KRAS, HER2, MET, BRAF, ALK, ROS1, and RET alterations in a real-world setting.
What is the implication, and what should change now?
• ICI treatment may be an option for advanced NSCLC patients with oncogenic drivers, but each alteration portends a different response to ICI and the treatment strategies should be tailored based on different types of gene alteration.
Introduction
Management of advanced non-small cell lung cancer (NSCLC) has changed drastically over the last few decades with the development of targeted therapies and immunotherapy. The unique pathogenesis of oncogene-driven NSCLC affects the clinical progression of disease and with major therapeutic implications. Many oncogenic drivers including EGFR, KRAS, MET, BRAF, HER2 mutation and ALK, ROS1, RET rearrangement have been identified (1). Although molecularly targeted agents have been approved for first-line or later-line settings in NSCLC patients with some of these oncogenic alterations and led to improved clinical outcomes (2-4), there is a large spectrum of heterogeneity in duration and rate of response to targeted therapies across different oncogene-driven subtypes. In addition, acquired resistance to targeted therapies is inevitable and poses a significant clinical challenge due to the paucity of effective subsequent treatment options (5). Thus, developing new treatment strategies to address this treatment shortcoming is urgently needed.
Immune checkpoint inhibitors (ICIs) targeting programmed cell death 1 (PD-1) and PD-ligand 1 (PD-L1) have prolonged the overall survival (OS) of advanced NSCLC patients without oncogenic alterations (6), in addition to creating a durable response in the selected group of patients (7). Even though the durable responses obtained with immunotherapy are desired for a large population of NSCLC patients, their efficacy in oncogene-driven NSCLC has not been fully clarified. The majority of driver oncogenes identified so far in NSCLC lead to tumor cell proliferation by constitutively activating various signaling pathways. Multiple studies have suggested that activation of oncogenic signaling pathways alters tumor immune microenvironment, excluding activated T cells while promoting proliferation of the immunosuppressive cell population (8-11). Subsequently, this may contribute to tumor evasion of immunosurveillance and also affect the efficacy of immunotherapy in oncogene-driven subtypes of NSCLC (12).
KRAS-mutant NSCLC is mostly seen in smoker population and is associated with the high expression of PD-L1 and tumor mutation burden (TMB) (13-17), suggesting a high tumor immunogenicity and inflammatory microenvironment, which is thought to confer sensitivity to ICIs. Data of clinical trials showed that KRAS-mutant NSCLC benefited from ICI monotherapy or combination chemoimmunotherapy similarly to or better than the KRAS wild type NSCLC (14,18-22). BRAF- and MET-altered NSCLC may occur in both smokers and nonsmokers (23-26). For BRAF-mutant NSCLC with a history of smoking and a high level of PD-L1 expression, the rates of response to anti-PD-1/PD-L1 therapies are similar to those in the non-selected population (13,27). And some studies demonstrate that MET exon 14 skipping induces PD-L1 expression (28,29). A substantial proportion of MET exon 14 alterations patients even with high levels of PD-L1 yet experience only modest efficacy with ICI monotherapy (30). ALK-, ROS1-, RET-rearrangement, and HER2-altered NSCLC tend to occur in nonsmokers (26,31). Studies investigating the efficacy of ICI in HER2 mutated NSCLC report variable findings with overall response rate (ORR) of 7–27% and with progression-free survival (PFS) of 2.2–2.5 months (32,33). Patients with driver rearrangement in ALK, ROS1, and RET rarely receive benefit from ICI monotherapy, and in most cases, the PFS barely exceeds 3 months (33). This could be due to the low TMB and PD-L1 expression (13,34,35) in these patients. It is worth mentioning that although there is poor efficacy of ICI monotherapy in oncogenic-driven NSCLC mentioned above other than KRAS, a few studies have still reported some encouraging results with ICI-based combination therapy (36,37). The consensus among Chinese experts on immunotherapy for advanced driver gene-positive NSCLC is that targeted therapy should be given priority if the targeted drugs are available. A re-biopsy should be performed again if conditions permit when after resistance to targeted therapy. In cases where targeted therapy is resistant or not available, ICI treatment decisions need to be comprehensively considered (38).
Given the heterogeneous and modest benefits of immunotherapy in patients with actionable genetic alterations, discerning the extent to which anti-PD-1/PD-L1 inhibitors confer advantages for patients with different oncogenes is a matter of substantial clinical relevance. However, the general occurrence rate of the abovementioned gene alterations in NSCLC is not high, thus making the implementation of prospective studies challenging. Considering these issues, we conducted a retrospective, multicenter study to explore the real-world immunotherapy patterns, survival outcome data, and safety in advanced NSCLC patients with KRAS, BRAF, MET, HER2, ALK, ROS1, or RET alteration. We present this article in accordance with the STROBE reporting checklist (available at https://tlcr.amegroups.com/article/view/10.21037/tlcr-24-116/rc).
Methods
Study design and patients
This multicenter, retrospective cohort study included four hospitals (West China Hospital, Sichuan Cancer Hospital, 363 Hospital, Leshan People’s Hospital) in Sichuan Province. The study was conducted in accordance with the Declaration of Helsinki (as revised in 2013), and the study protocol was reviewed and approved by the Ethics Committee of West China Hospital (No. 2023-1004). All participating hospitals were informed and agreed with this study. Informed consent was waived due to the retrospective nature of the study.
Patients with histologic confirmation of NSCLC who were treated with anti-PD-1/PD-L1 treatment (monotherapy or anti-PD-1/PD-L1 inhibitor combined with any other drugs) between May 2015 and October 2022 were reviewed. The cutoff for the follow-up data was May 10, 2023. Eligible patients were aged 18 years or older, treated with anti-PD-1 or PD-L1 monotherapy or combination with other drugs, and diagnosed as stage IV NSCLC with at least one of the following somatic de novo oncogenic driver alteration: HER2 (amplification or exon 20 activating mutation), KRAS mutation, BRAF mutation (V600E or non-V600E), MET amplification or exon 14 skipping mutation, ALK rearrangement, ROS1 rearrangement, and RET rearrangement. Patients with incomplete medical records or included in a clinical immunotherapy trial were excluded.
Data collection
This study did not interfere with any clinical practice, and all patients’ treatments were prescribed by their own physicians. Electronic medical records were reviewed, and data regarding clinicopathologic characteristics and follow-up information were extracted, including age, gender, Eastern Cooperative Oncology Group (ECOG) score, histological types, smoking status, oncogene alteration subtype, PD-L1 status, details of ICI treatment and outcomes, and follow-up status.
Response assessment and outcomes
PFS was calculated from the day of ICI treatment initiation to disease progression or death from any cause, and data was censored if the patient was alive without known progression of disease at the time of the last follow-up (May 10, 2023). OS was calculated from the day of ICI treatment initiation to death, and the outcome data was censored if a patient was alive at the time of the last follow-up (May 10, 2023).
ORR was calculated as a percentage of patients with an evaluated complete response (CR) or partial response (PR). Real-world treatment efficacy was determined by physician assessment according to Response Evaluation Criteria in Solid Tumor 1.1 (RECIST 1.1) guidelines (39).
Statistical analysis
Descriptive statistics were presented as mean ± standard deviation and the absolute number with proportions (percentage) for continuous and categorical variables, respectively. If the data conformed to a normal distribution, the two-tailed independent samples t-test was used to compare the difference; otherwise, a nonparametric test was used. Cox proportional hazard regression models were used to obtain estimates of hazard ratios (HRs) and 95% confidence intervals (95% CIs) in univariate and multivariable analyses. PFS and OS were estimated using the Kaplan-Meier method and compared using the log-rank test. All P values were two-sided, and P<0.05 was determined as indicative of statistical significance. All statistical analyses were performed using SPSS version 25.0 (IBM Corp., Armonk, NY, USA) and GraphPad Prism version 8.0 (GraphPad Software).
Results
Patient characteristics
Between May 2015 and October 2022, 1,453 patients with advanced NSCLC who received ICI monotherapy or combined immunotherapy (anti-PD-1/PD-L1 inhibitor with other drugs) in four medical centers were identified. According to the inclusion and exclusion criteria, 216 patients with at least one oncogenic alteration (KRAS, HER2, BRAF, MET, RET, ALK, or ROS1) were finally included in our study (Figure 1). The genes of 177 patients were detected by next-generation sequencing (NGS) and the rest 39 patients were via amplification refractory mutation system polymerase chain reaction (ARMS-PCR).
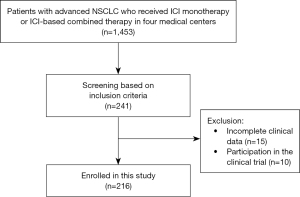
The median age of patients was 60 (range, 37 to 83) years, and 72.7% (157/216) were male. Among the total population, 101 (46.8%) patients had a smoking history, and 177 patients (81.9%) were diagnosed with lung adenocarcinoma. The molecular alterations included were KRAS (n=95), HER2 (n=42), BRAF (n=22), MET (n=21), RET (n=14), ALK (n=14), and ROS1 (n=8) (Figure 2A). At the time of immunotherapy initiation, most patients had an ECOG performance status (PS) of 0 or 1 (n=206, 95.4%). Details of the patient characteristics by genetic alteration are shown in Table 1.
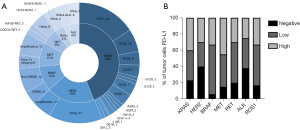
Table 1
Characteristic | KRAS (N=95) | HER2 (N=42) | BRAF (N=22) | MET (N=21) | RET (N=14) | ROS1 (N=8) | ALK (N=14) |
---|---|---|---|---|---|---|---|
Age at diagnosis, years, median [range] |
59 [41–83] | 58 [37–77] | 63 [34–74] | 66 [46–83] | 55 [37–75] | 51 [41–71] | 53 [28–80] |
Gender, n (%) | |||||||
Male | 82 (86.3) | 22 (52.4) | 17 (77.3) | 17 (81.0) | 8 (57.1) | 3 (37.5) | 8 (57.1) |
Female | 13 (13.7) | 20 (47.6) | 5 (22.7) | 4 (19.0) | 6 (42.9) | 5 (62.5) | 6 (42.9) |
Smoking, n (%) | |||||||
Never | 40 (42.1) | 29 (69.0) | 12 (54.5) | 9 (42.9) | 9 (64.3) | 7 (87.5) | 9 (64.3) |
Current/former | 55 (57.9) | 13 (31.0) | 10 (45.5) | 12 (57.1) | 5 (35.7) | 1 (12.5) | 5 (35.7) |
ECOG, n (%) | |||||||
0–1 | 90 (94.7) | 42 (100.0) | 22 (100.0) | 18 (85.7) | 14 (100.0) | 8 (100.0) | 12 (85.7) |
≥2 | 5 (5.3) | 0 | 0 | 3 (14.3) | 0 | 0 | 2 (14.3) |
Histological type, n (%) | |||||||
Adenocarcinoma | 83 (87.4) | 35 (83.3) | 18 (81.8) | 11 (52.4) | 11 (78.6) | 7 (87.5) | 12 (85.7) |
Squamous | 9 (9.5) | 6 (14.3) | 4 (18.2) | 9 (42.9) | 2 (14.3) | 1 (12.5) | 1 (7.1) |
Other | 3 (3.2) | 1 (2.4) | 0 | 1 (4.8) | 1 (7.1) | 0 | 1 (7.1) |
Stage at diagnosis, n (%) | |||||||
IVa | 45 (47.4) | 14 (33.3) | 10 (45.5) | 12 (57.1) | 5 (35.7) | 3 (37.5) | 8 (57.1) |
IVb | 50 (52.6) | 28 (66.7) | 12 (54.5) | 9 (42.9) | 9 (64.3) | 5 (62.5) | 6 (42.9) |
Treatment line, n (%) | |||||||
1 | 61 (64.2) | 22 (52.4) | 15 (68.2) | 12 (57.1) | 10 (71.4) | 0 | 2 (14.3) |
2 | 25 (26.3) | 14 (33.3) | 4 (18.2) | 5 (23.8) | 3 (21.4) | 5 (62.5) | 6 (42.9) |
≥3 | 9 (9.5) | 6 (14.3) | 3 (13.6) | 4 (19.0) | 1 (7.1) | 3 (37.5) | 6 (42.9) |
Immunotherapy, n (%) | |||||||
Monotherapy | 29 (30.5) | 10 (23.8) | 6 (27.3) | 7 (33.3) | 6 (42.9) | 1 (12.5) | 7 (50.0) |
Combined therapy | 66 (69.5) | 32 (76.2) | 16 (72.7) | 14 (66.7) | 8 (57.1) | 7 (87.5) | 7 (50.0) |
Distant metastasis site, n (%) | |||||||
Brain | 23 (24.2) | 11 (26.2) | 6 (27.3) | 7 (33.3) | 4 (28.6) | 2 (25.0) | 5 (35.7) |
Liver | 16 (16.8) | 8 (19.0) | 3 (13.6) | 0 | 4 (28.6) | 2 (25.0) | 2 (14.3) |
Bone | 39 (41.1) | 23 (54.8) | 11 (50.0) | 7 (33.3) | 6 (42.9) | 6 (75.0) | 4 (28.6) |
ECOG, Eastern Cooperative Oncology Group.
PD-L1 expression
PD-L1 expression was measured by the immunohistochemistry (IHC) assay via 22C3. In patients with available PD-L1 expression data (n=175), 41 (23.4%) patients were PD-L1 negative [tumor proportion score (TPS) <1%], 71 (40.6%) patients had low PD-L1 expression (TPS 1–49%), and 63 (36.0%) patients were strongly positive for PD-L1 (TPS ≥50%). According to different gene variants, 39.8% of patients in the KRAS group, 30.0% in the HER2 group, 33.3% in the BRAF group, 45.0% in the MET group, 30.0% in the RET group, 33.3% in the ROS1 group, and 25% in the in ALK group had a high expression of PD-L1 (TPS ≥50%) (Figure 2B).
Treatment characteristics
A total of 122 (56.5%) patients received immunotherapy in the first-line, with the KRAS group (n=61, 50.0%) accounting for the vast majority, followed by the HER2 (n=22, 18.0%), BRAF (n=15, 12.3%), MET (n=12, 9.8%), RET (n=10, 8.2%), and ALK (n=2, 1.6%) groups. Each gene cohort were mainly treated with ICI-based combination therapy. The remaining 94 patients received immunotherapy as a second-line therapy or beyond, with the majority also being treated with ICI-based combination therapy (Figure 3). Immunotherapy based combination regimens were distributed as follows: 125 patients received ICI with chemotherapy, 17 received ICI with chemotherapy and antiangiogenic agents, 6 received ICI with antiangiogenetic agents, and 2 received ICI with tyrosine kinase inhibitors (TKIs).
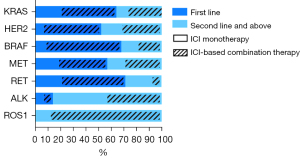
The overwhelming majority (n=206, 95.4%) of patients received anti-PD-1 inhibitors (nivolumab n=21, pembrolizumab n=83, sintilimab n=56, camrelizumab n=27, tislelizumab n=19), and only 10 patients received anti-PD-L1-antibodies (durvalumab n=7, atezolizumab n=3).
Clinical outcomes
Total population
As of the data cut off on May 10, 2023, the median follow-up was 29.8 months (95% CI: 27.3–32.3), 185 patients (85.6%) had disease progression, and 130 patients (60.2%) died. Among the total population, the ORR was 34.7% and the disease control rate (DCR) was 75.5%. The median PFS and median OS in the overall population was 6.0 months (95% CI: 5.2–6.9) and 21.3 months (95% CI: 20.0–22.6), respectively.
The median PFS and OS of the total population treated with first-line immunotherapy were 7.5 months (95% CI: 6.5–8.6) and 24.8 months (95% CI: 22.5–27.0), respectively. In the second-line and beyond, the median PFS and OS of immunotherapy were 4.7 months (95% CI: 3.9–5.6) and 17.1 months (95% CI: 14.9–19.3), respectively (Figure 4).
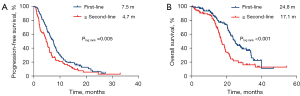
When further analyzing whether ICI alone or is combined with chemotherapy, the results showed that in the first-line treatment setting, the median PFS and OS of ICI monotherapy were 4.9 months (95% CI: 2.9–6.9) and 20.9 months (95% CI: 16.2–25.6), and the median PFS and OS of ICI plus chemotherapy were 7.8 months (95% CI: 6.8–8.8) and 25.8 months (95% CI: 21.3–30.3) (Figure 5).
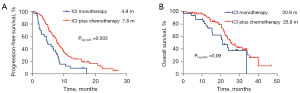
In the second-line and above treatment setting, the median PFS and OS of ICI monotherapy were 2.1 months (95% CI: 1.7–2.3) and 14.5 months (95% CI: 11.9–17.1), and the median PFS and OS of ICI plus chemotherapy were 6.0 months (95% CI: 5.1–7.0) and 19.3 months (95% CI: 16.6–21.9) (Figure 6).
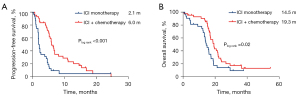
Gene alteration subgroup analysis
KRAS mutation
KRAS mutations were detected in 95 patients, with G12C (35.8%) being the most frequent type, followed by G12D (13.7%) and G12A (8.4%); details on other mutation types are displayed in Figure 2A. A total of 61 (64.2%) patients received immunotherapy in the first-line, with 20 (32.8%) patients receiving ICI monotherapy and 41 (67.2%) patients receiving ICI plus chemotherapy with or without antiangiogenic drugs. The median PFS and OS of patients with the KRAS mutation treated with first-line immunotherapy were 7.8 months (95% CI: 6.9–8.7) and 26.1 months (95% CI: 22.0–30.3), respectively. The remaining 34 (35.8%) patients received ICI monotherapy or combined therapy in the second-line and beyond, achieving a median PFS and OS of 5.9 months (95% CI: 4.0–7.7) and 17.1 months (95% CI: 14.9–19.3), respectively.
We examined the impact of clinical and treatment characteristics of age, ECOG score, gender, PD-L1 expression, number of treatment lines, treatment regimen, mutation type, and smoking status on PFS or OS. The results of the multivariate Cox analysis showed that a good ECOG (0–1), PD-L1 positive expression (TPS ≥1%), ICI-based combined therapy, and KRAS G12C mutation subtype were independent positive predictors of PFS (Table S1). Moreover, a better OS was correlated with good ECOG PS (0–1), PD-L1-positive expression (TPS ≥1%), and the KRAS G12C mutation (Table S2).
HER2 alteration
A total of 42 patients with HER2 alteration were included, 31 of whom had the exon 20 insertion (20Ins) and 11 of whom had gene amplification. Nine of the patients had PD-L1 ≥50% and 21 patients had PD-L1 <50%. PFS correlated with a high expression of PD-L1 [21.8 months (five of nine patients had disease progression) for PD-L1 ≥50% vs. 5.1 months for PD-L1 <50%; P=0.006] and the treatment line of immunotherapy (7.6 months for the first-line vs. 4.9 months for the second-line and beyond; P=0.03). OS correlated with treatment line of immunotherapy (24.7 vs. 17.4 months; P=0.02) (Table S3).
As for those with 20Ins, 16 received immunotherapy in the first-line, 2 of whom received ICI monotherapy, with the other 14 patients being treated with ICI plus chemotherapy. Patients with 20Ins treated with ICI plus chemotherapy in the first-line had a median PFS of 7.6 months (95% CI: 2.3–12.9) and a median OS of 22.1 months (95% CI: 19.5–24.7). The other 15 patients with 20Ins received immunotherapy in the second-line and experienced a median PFS of 5.0 (95% CI: 0.3–9.6) months and a median OS of 17.4 months (95% CI: 12.5–22.3). Before immunotherapy, 8 patients received TKI therapy with afatinib. Patients who did not receive afatinib before immunotherapy had a median PFS of 5.9 months (95% CI: 3.5–8.4) and a median OS of 17.4 months (95% CI: 14.8–20.0), while the patients treated with immunotherapy after progression on afatinib obtained a median PFS of 2.3 months (95% CI: 0.5–4.0) and a median OS of 19.1 months (95% CI: 11.4–26.8).
In the gene amplification cohort, there was no TKI treatment before immunotherapy, 6 patients received immunotherapy in the first-line, and 5 patients received immunotherapy in the second-line and beyond. The median PFS and OS was 5.1 months (95% CI: 2.5–7.7) and 24.7 months (95% CI: 7.0–42.5) in the first-line, respectively, and the median PFS and OS was 4.9 months (95% CI: 0–20.3) and 11.7 months (95% CI: 10.8–12.7) in the second-line and beyond, respectively.
BRAF alteration
BRAF molecular alterations were found in 22 patients, 8 of whom had the BRAF V600E mutation and 14 of whom harbored the non-V600E mutation, including 1 with BRAF-SND1 fusion, 2 with BRAF K601E mutation, 2 with BRAF D594N mutation, 1 with BRAF G464V mutation, and 8 with non-V600 mutation (the details for these patients are not available). The PFS and OS did not correlate with gene subtype, smoking, age, PD-L1 expression (cutoff of 1% or 50%), age, gender, or the treatment line of immunotherapy (Table S4).
Five patients harboring the BRAF V600E mutation received immunotherapy in the first line setting with a median PFS of 3.9 months (95% CI: 3.4–4.3) and an ORR of 40% (2/5). Two patients received immunotherapy after the progression on dabrafenib and trametinib treatment: the patient who received ICI monotherapy had a PFS of 1.1 months, and the patient who received chemoimmunotherapy had a PFS of 5.2 months.
Patients with BRAF non-V600E mutation received immunotherapy in the first line (n=10) and had a median PFS of 7.7 months (95% CI: 1.7–13.7) and a median OS of 23.7 months (95% CI: 18.8–28.6).
MET alteration
Of patients with MET alteration, 12 patients with copy number gains and 9 patients had exon 14 skipping mutation. PD-L1 positivity was significantly correlated with a longer OS (21.6 months for PD-L1 ≥1% vs. 12.1 months for PD-L1 <1%; P=0.004) (Table S5).
Four patients with MET exon 14 skipping mutation received immunotherapy in the first-line and achieved a median PFS of 6.0 months (95% CI: 2.0–9.9) and an ORR of 25%. A total of four patients received immunotherapy in the second-line and beyond, 2 of whom received crizotinib before immunotherapy; patients who received immunotherapy in the second-line or later-lines had a median PFS of 2.4 months (95% CI: 0.1–4.7) and a median OS of 17.5 months (95% CI: 13.8–21.2).
Patients with MET gene amplification in our study were primary MET amplification rather than acquired MET amplification secondary to other driver gene alterations. Four of these patients received TKI therapy before immunotherapy: three with crizotinib and one with savolitinib. Eight patients with MET amplification received immunotherapy in the first-line and experienced a median PFS of 7.5 months (95% CI: 0.2–14.8) and an ORR of 50%. The remaining five patients who received immunotherapy in the second-line and beyond achieved a median PFS of 5.3 months (95% CI: 1.7–9.0) and an ORR of 20%.
ALK, ROS1, and RET rearrangement
ALK, ROS1, and RET were analyzed together due to the limited sample size for each group. A longer PFS and OS was more associated with ICI-based combination therapy vs. monotherapy (median PFS: 4.1 vs. 2.0 months, P=0.01; median OS: 23.7 vs. 12.4 months, P=0.01) (Table S6).
A total of 4 patients had fusion with EML4 using NGS, and the remaining 10 patients were identified as ALK positive via IHC. In the first-line immunotherapy setting, one patient received ICI monotherapy and another patient received ICI plus chemotherapy and antiangiogenic drugs, experiencing a poor PFS of 1.8 and 2.8 months, respectively. The other 12 patients with ALK fusion received ALK-TKIs before immunotherapy, 5 of whom received a single ICI agent and had a median PFS of 2.0 months (95% CI: 1.8–2.2) and a median OS of 13.3 months (95% CI: 11.4–15.2). The other seven patients received ICI-based combination therapy and had a median PFS of 3.9 months (95% CI: 1.2–6.7) and a median OS of 24.0 months (95% CI: 5.5–42.5).
In the ROS1 group, one patient had fusion with SCAF8 and another had fusion with CD74, but the fusion partner of remaining six patients was unknown. One patient received ICI monotherapy after progression on crizotinib plus chemotherapy and had a PFS of only of 0.7 months and an OS of 9.2 months. The other seven patients received ICI-based combination therapy (ICI plus chemotherapy with or without antiangiogenic drugs) after progressing on crizotinib, achieving a median PFS of 5.6 months (95% CI: 3.1–8.2), but the median OS could not be evaluated.
As for the RET group, three patients had CCDC6-RET fusion, and three patients had KIF5B-RET fusion, but the fusion partner was unknown for the remaining eight patients. Ten patients received immunotherapy in the first-line and had a median PFS of 3.9 months (95% CI: 3.0–4.9) and a median OS of 22.2 months (95% CI: 8.2–36.3). Four patients received immunotherapy in the second-line and beyond, and the median PFS and OS of the posterior line were 2.2 months (95% CI: 1.7–2.6) and 14.5 months (95% CI: 0–29.2), respectively.
Safety
All grades of treatment-related adverse events (TRAEs) occurred in 39.4% (85/216) of patients. Leukopenia, rash, granulocytopenia, increased aspartate transaminase and alanine aminotransferase, and pneumonitis were the most common adverse events (AEs). Grade 3 AEs were observed in 6.9% (15/216) of patients, with the leukopenia (33.3%, 4/12) being the most common event. No unexpected AEs or AE-related deaths occurred. Three patients (1.4%, 3/216) discontinued treatment due to a grade 3 cutaneous hemangioma after four cycles of ICI plus chemotherapy (paclitaxel and carboplatin) (Table 2).
Table 2
AEs | Grade 1, n (%) | Grade 2, n (%) | Grade 3, n (%) | Grade 4 | Grade 5 |
---|---|---|---|---|---|
Fatigue | 1 (0.5) | – | – | – | – |
Rash | 4 (1.9) | 4 (1.9) | 1 (0.5) | – | – |
Vomit | 3 (1.4) | – | – | – | – |
Diarrhea | 1 (0.5) | – | – | – | – |
Anemia | – | 2 (0.9) | – | – | – |
Granulocytopenia | 2 (0.9) | 4 (1.9) | 3 (1.4) | – | – |
Thrombocytopenia | – | 1 (0.5) | 2 (0.9) | – | – |
Hypothyroidism | 5 (2.3) | 2 (0.9) | – | – | – |
Hyperthyroidism | 1 (0.5) | – | – | – | – |
Increased AST/ALT | 7 (3.2) | 2 (0.9) | 1 (0.5) | – | – |
Increased creatinine | 3 (1.4) | 1 (0.5) | – | – | – |
Pneumonitis | 1 (0.5) | 9 (4.2) | 3 (1.4) | – | – |
Encephalitis | – | 1 (0.5) | – | – | – |
Ostealgia | 1 (0.5) | – | – | – | – |
Pyrexia | – | 1 (0.5) | – | – | – |
Nausea | 3 (1.4) | – | – | – | – |
Leukopenia | 4 (1.9) | 4 (1.9) | 4 (1.9) | – | – |
Hematochezia | 1 (0.5) | – | – | – | – |
Cutaneous hemangioma | 2 (0.9) | 2 (0.9) | 1 (0.5) | – | – |
Myocarditis | 1 (0.5) | – | – | – | – |
AE, adverse event; AST, aspartate transaminase; ALT, alanine aminotransferase.
Two patients (HER2 20Ins and BRAF V600E) received anti-PD-1 inhibitor combined with TKI after progression on the previous chemotherapy in our study, and these patients did not experience any TKI or ICI-related AEs. Moreover, a total of 14 patients received TKIs after progression on ICI treatment, and no grade 3 or higher AEs were observed.
Discussion
This retrospective, multicenter study demonstrates the effectiveness of ICI treatment for patients with advanced NSCLC harboring KRAS, HER2, MET, BRAF, ALK, ROS1, and RET alterations. We demonstrated that in the front-line and subsequent-line setting, patients’ response to ICI treatment varies based on the specific gene alteration.
In our study, patients received ICI therapy mainly as first-line to third line and beyond treatment. The objective response rate of the whole cohort was 35.0%, the median PFS and OS were 6.0 and 21.3 months, respectively. These survival outcomes were better than certain retrospective studies of immunotherapy in oncogene-driven NSCLC cohorts (32,33) and the results of immunotherapy in the unselected NSCLC population in the second-line (ORR 15–20%, median PFS 2.3–4 months) (40,41). The potential reasons for the superior survival outcomes in our study may be attributed to substantial proportion of patients (95.3%) with a good ECOG PS (0 or 1) and the prevalent use of combination ICI therapy in the majority of patients (69.2%). The co-occurring mutations may affect the efficacy (41), but further analysis was not conducted due to limited data. Our findings suggest that certain subsets of NSCLC with driver gene alterations can still benefit from ICI treatment, and thus identifying these specific subsets and the ideal treatment regimens is clinically important. The KRAS mutation cohort in our study obtained a median PFS of 7.3 months and an OS of 22.3 months. KRAS mutation is known to be a poor prognostic factor (42), development of targeted therapies has been challenging for this subset of NSCLC for years, but there are now approved targeted inhibitors in the second-line and beyond therapy only for KRAS G12C mutation (43). The majority of patients with KRAS mutation in our study received immunotherapy in the first and second-line, and more than half received a combination therapy of ICI, with chemotherapy being the most common combination. ICI-based combination therapy demonstrated superior PFS compared to ICI monotherapy among KRAS-mutant patients regardless of treatment lines. The PFS benefits have not been significantly transformed into OS benefits may be caused by the small sample size. Furthermore, the KRAS G12C mutation type was found to be significantly associated with a better PFS and OS of immunotherapy. The KRAS G12C group obtained an ORR of 52.9% and a median PFS of 9.4 months, and the median OS was not reached.
PD-L1 expression is a widely used predictive indicator for non-oncogene addicted NSCLC treated with anti-PD-1/PD-L1 inhibitors (44). KRAS-mutant NSCLC has been demonstrated to have an inflammatory phenotype with high PD-L1 expression in prior studies (10-14), but no significant expression advantages in PD-L1 among KRAS mutation over other gene variants in our study were observed. We analyzed patients who have PD-L1 expression data. In our study, PD-L1 positivity was predictive of immunotherapy response either PFS or OS benefit for patients with KRAS mutation, and the patients with PD-L1 ≥1% had a median PFS of 8.2 months and a median OS of 28.2 months. However, in subset analysis of other genetic alterations, PD-L1 positivity did not correlate with survival benefit except for MET alteration group where PD-L1 ≥1% was associated with a favorable OS. For the HER2 group, high PD-L1 expression (≥50%) correlated with better PFS only. No statistical significance was found between PD-L1 expression (≥1% or ≥50%) and survival benefit in BRAF group and ALK, ROS1, RET group. These findings indicate that screening beneficiaries solely based on the PD-L1 expression status in these population may be less than ideal in clinic. In addition, there was no correlation between smoking exposure, gender, or age with ICI treatment benefit in any gene group in our study. This suggests that other factors such as co-occurring mutations or TMB may impact clinical outcomes from immune checkpoint blockade therapy. However, due to the limited information, this could not be investigated in this study.
Some patients with HER2, BRAF, and MET alterations or RET and ALK rearrangement received immunotherapy in the first-line. For patients with MET exon 14 skipping, BRAF V600E, and RET rearrangement, the median PFS of first-line immunotherapy ranged from 3.5 to 6 months while the ORR ranged from 20% to 40%, which is lower compared to previously reported TKI therapy data (45-47). There was negative genetic result of lung biopsy sample in two ALK fusion-positive patients, prompting the initiation of ICI treatment before TKI therapy: one patient with a PD-L1 expression of 80% received PD-1 monotherapy and achieved a PFS of 1.8 months; the other with unknown PD-L1 expression received chemoimmunotherapy plus antiangiogenic agent and achieved a PFS of 2.8 months. This poor response suggests that targeted therapies should be considered in the first-line for these patients with the MET exon 14 skipping mutation, BRAF V600E alteration, or ALK arrangement as opposed to immunotherapy. However, for later lines, ICI-based therapy may provide benefit for these patients.
In terms of treatment safety, the overall AE rate in our study was 39.4%, with a 5.6% grade 3 AE rate. No new or unexpected or AE-associated deaths were observed. A high incidence of AEs has been reported with ICI administered sequentially or concurrently with TKI inhibitors in EGFR-mutant, ALK-rearranged, or KRAS G12C-mutant NSCLC (48-53). A total of 14 patients received TKI after the progression of ICI treatment, and no grade 3 or higher AEs were observed, which may be related to the time interval between the patients receiving two treatments being greater than 30 days thus weakening the impact of ICI on subsequent TKI treatment. Two patients (HER2 20Ins and BRAF V600E) received anti-PD-1 inhibitor combined with TKI after progression on previous chemotherapy in our study, and no TRAEs were observed. Due to the small size of our study sample, these issues may require further investigation.
The study has several limitations. First, the sample size of the different gene alteration subsets is small. The heterogeneous nature of the patient population, with varied immunotherapy-based regimens and diverse treatment lines, should be acknowledged while interpreting the results. MET and HER2 alteration subgroups also included gene amplification in addition to actionable mutations. However, this needs to be acknowledged while interpreting results since mutations and gene amplifications in these cohorts have different therapeutic implications. Additionally, data for PD-L1 expression, TMB, and co-occurring mutations were unavailable for all patients. Finally, the generalizability of our findings is limited by the fact that this retrospective study only assessed data from patients across four medical centers in Sichuan Province. Therefore, it must be acknowledged that the results of our study need to be interpreted with caution because of the limitations. More prospective studies are still needed in the future.
Conclusions
In conclusion, this multicenter retrospective study demonstrated that immunotherapy for NSCLC patients with oncogenic driver alterations is tolerable. The effectiveness of immunotherapy varies on the oncogenic driver, and need for expanding studies. An association between PD-L1 expression level and the responses to ICIs was not consistently observed across different driver alterations in our study. For NSCLC with KRAS, ICIs may be recommended both for treatment-naïve or pretreated patients. However, for NSCLC patients with ALK, ROS1 rearrangement, BRAF V600E, and MET-14 skipping, targeted therapies should be considered prior to immunotherapy.
Acknowledgments
We thank all participating centers for supporting this study.
Funding: None.
Footnote
Reporting Checklist: The authors have completed the STROBE reporting checklist. Available at https://tlcr.amegroups.com/article/view/10.21037/tlcr-24-116/rc
Data Sharing Statement: Available at https://tlcr.amegroups.com/article/view/10.21037/tlcr-24-116/dss
Peer Review File: Available at https://tlcr.amegroups.com/article/view/10.21037/tlcr-24-116/prf
Conflicts of Interest: All authors have completed the ICMJE uniform disclosure form (available at https://tlcr.amegroups.com/article/view/10.21037/tlcr-24-116/coif). Y.O. serves as an unpaid editorial board member of Translational Lung Cancer Research from January 2024 to December 2025, and reports speakers bureaus from Astra Zeneca, Boehringer Ingelheim, Chugai Pharmaceutical Co. Ltd., Daiichi-Sankyo, Eisai, Ely-Lilly, MSD, Ono Pharmaceutical Co. Ltd., Taiho Pharmaceutical Co. Ltd., Takeda Pharmaceutical Co. Ltd. A.E.C.D. reports support for attending meetings and/or travel from MJH Life Sciences. A.P. reports speaker’s fee from MSD, Daiichi Sankyo, Astra Zeneca, Sanofi, Merck, Takeda, Lilly, BMS, Roche; payment or honoraria for lectures, presentations, speakers bureaus from Daiichi Sankyo and BMS; support for attending meetings and/or travel from Merck and Sandoz. The other authors have no conflicts of interest to declare.
Ethical Statement: The authors are accountable for all aspects of the work in ensuring that questions related to the accuracy or integrity of any part of the work are appropriately investigated and resolved. The study was conducted in accordance with the Declaration of Helsinki (as revised in 2013) and was approved by the Ethics Committee of West China Hospital (No. 2023-1004). All participating hospitals were informed and agreed with this study. Individual consent for this retrospective analysis was waived.
Open Access Statement: This is an Open Access article distributed in accordance with the Creative Commons Attribution-NonCommercial-NoDerivs 4.0 International License (CC BY-NC-ND 4.0), which permits the non-commercial replication and distribution of the article with the strict proviso that no changes or edits are made and the original work is properly cited (including links to both the formal publication through the relevant DOI and the license). See: https://creativecommons.org/licenses/by-nc-nd/4.0/.
References
- Jordan EJ, Kim HR, Arcila ME, et al. Prospective Comprehensive Molecular Characterization of Lung Adenocarcinomas for Efficient Patient Matching to Approved and Emerging Therapies. Cancer Discov 2017;7:596-609. [Crossref] [PubMed]
- Le T, Gerber DE. ALK alterations and inhibition in lung cancer. Semin Cancer Biol 2017;42:81-8. [Crossref] [PubMed]
- Melosky B, Wheatley-Price P, Juergens RA, et al. The rapidly evolving landscape of novel targeted therapies in advanced non-small cell lung cancer. Lung Cancer 2021;160:136-51. [Crossref] [PubMed]
- Majeed U, Manochakian R, Zhao Y, et al. Targeted therapy in advanced non-small cell lung cancer: current advances and future trends. J Hematol Oncol 2021;14:108. [Crossref] [PubMed]
- Schrank Z, Chhabra G, Lin L, et al. Current Molecular-Targeted Therapies in NSCLC and Their Mechanism of Resistance. Cancers (Basel) 2018;10:224. [Crossref] [PubMed]
- Mamdani H, Matosevic S, Khalid AB, et al. Immunotherapy in Lung Cancer: Current Landscape and Future Directions. Front Immunol 2022;13:823618. [Crossref] [PubMed]
- Cali Daylan AE, Halmos B. Long-term benefit of immunotherapy in metastatic non-small cell lung cancer: the tale of the tail. Transl Lung Cancer Res 2023;12:1636-42. [Crossref] [PubMed]
- Spranger S, Gajewski TF. Impact of oncogenic pathways on evasion of antitumour immune responses. Nat Rev Cancer 2018;18:139-47. [Crossref] [PubMed]
- Zhang Y, Yang Q, Zeng X, et al. MET Amplification Attenuates Lung Tumor Response to Immunotherapy by Inhibiting STING. Cancer Discov 2021;11:2726-37. [Crossref] [PubMed]
- Mugarza E, van Maldegem F, Boumelha J, et al. Therapeutic KRAS(G12C) inhibition drives effective interferon-mediated antitumor immunity in immunogenic lung cancers. Sci Adv 2022;8:eabm8780. [Crossref] [PubMed]
- Kortlever RM, Sodir NM, Wilson CH, et al. Myc Cooperates with Ras by Programming Inflammation and Immune Suppression. Cell 2017;171:1301-1315.e14. [Crossref] [PubMed]
- Otano I, Ucero AC, Zugazagoitia J, et al. At the crossroads of immunotherapy for oncogene-addicted subsets of NSCLC. Nat Rev Clin Oncol 2023;20:143-59. [Crossref] [PubMed]
- Negrao MV, Skoulidis F, Montesion M, et al. Oncogene-specific differences in tumor mutational burden, PD-L1 expression, and outcomes from immunotherapy in non-small cell lung cancer. J Immunother Cancer 2021;9:e002891. [Crossref] [PubMed]
- Liu C, Zheng S, Jin R, et al. The superior efficacy of anti-PD-1/PD-L1 immunotherapy in KRAS-mutant non-small cell lung cancer that correlates with an inflammatory phenotype and increased immunogenicity. Cancer Lett 2020;470:95-105. [Crossref] [PubMed]
- Scheel AH, Ansén S, Schultheis AM, et al. PD-L1 expression in non-small cell lung cancer: Correlations with genetic alterations. Oncoimmunology 2016;5:e1131379. [Crossref] [PubMed]
- Chen N, Fang W, Lin Z, et al. KRAS mutation-induced upregulation of PD-L1 mediates immune escape in human lung adenocarcinoma. Cancer Immunol Immunother 2017;66:1175-87. [Crossref] [PubMed]
- Mansour MSI, Malmros K, Mager U, et al. PD-L1 Expression in Non-Small Cell Lung Cancer Specimens: Association with Clinicopathological Factors and Molecular Alterations. Int J Mol Sci 2022;23:4517. [Crossref] [PubMed]
- Landre T, Justeau G, Assié JB, et al. Anti-PD-(L)1 for KRAS-mutant advanced non-small-cell lung cancers: a meta-analysis of randomized-controlled trials. Cancer Immunol Immunother 2022;71:719-26. [Crossref] [PubMed]
- Borghaei H, Paz-Ares L, Horn L, et al. Nivolumab versus Docetaxel in Advanced Nonsquamous Non-Small-Cell Lung Cancer. N Engl J Med 2015;373:1627-39. [Crossref] [PubMed]
- Mok TSK, Wu YL, Kudaba I, et al. Pembrolizumab versus chemotherapy for previously untreated, PD-L1-expressing, locally advanced or metastatic non-small-cell lung cancer (KEYNOTE-042): a randomised, open-label, controlled, phase 3 trial. Lancet. 2019;393:1819-1830. [Crossref] [PubMed]
- Uehara Y, Watanabe K, Hakozaki T, et al. Efficacy of first-line immune checkpoint inhibitors in patients with advanced NSCLC with KRAS, MET, FGFR, RET, BRAF, and HER2 alterations. Thorac Cancer 2022;13:1703-11. [Crossref] [PubMed]
- Liu L, Li F, Zhao J, et al. The Real-world Therapeutic Analysis of First-line Immunotherapy in Chinese Patients with Drive Gene Positive for Advanced Non-Small Cell Lung Cancer. J Cancer 2023;14:952-65. [Crossref] [PubMed]
- Ding X, Zhang Z, Jiang T, et al. Clinicopathologic characteristics and outcomes of Chinese patients with non-small-cell lung cancer and BRAF mutation. Cancer Med 2017;6:555-62. [Crossref] [PubMed]
- Marchetti A, Felicioni L, Malatesta S, et al. Clinical features and outcome of patients with non-small-cell lung cancer harboring BRAF mutations. J Clin Oncol 2011;29:3574-9. [Crossref] [PubMed]
- Sakamoto M, Patil T. MET alterations in advanced non-small cell lung cancer. Lung Cancer 2023;178:254-68. [Crossref] [PubMed]
- Daylan AEC, Miao E, Tang K, et al. Lung Cancer in Never Smokers: Delving into Epidemiology, Genomic and Immune Landscape, Prognosis, Treatment, and Screening. Lung 2023;201:521-9. [Crossref] [PubMed]
- Dudnik E, Peled N, Nechushtan H, et al. BRAF Mutant Lung Cancer: Programmed Death Ligand 1 Expression, Tumor Mutational Burden, Microsatellite Instability Status, and Response to Immune Check-Point Inhibitors. J Thorac Oncol 2018;13:1128-37. [Crossref] [PubMed]
- Wang F, Cali Daylan AE, Deng L, et al. Heterogeneous Expression of PD-L1, B7x, B7-H3, and HHLA2 in Pulmonary Sarcomatoid Carcinoma and the Related Regulatory Signaling Pathways. Cancers (Basel) 2023;15:3372. [Crossref] [PubMed]
- Fischer A, Bankel L, Hiltbrunner S, et al. Mutational Landscape and Expression of PD-L1 in Patients with Non-Small Cell Lung Cancer Harboring Genomic Alterations of the MET gene. Target Oncol 2022;17:683-94. [Crossref] [PubMed]
- Sabari JK, Leonardi GC, Shu CA, et al. PD-L1 expression, tumor mutational burden, and response to immunotherapy in patients with MET exon 14 altered lung cancers. Ann Oncol 2018;29:2085-91. [Crossref] [PubMed]
- Zhou F, Zhou C. Lung cancer in never smokers-the East Asian experience. Transl Lung Cancer Res 2018;7:450-63. [Crossref] [PubMed]
- Guisier F, Dubos-Arvis C, Viñas F, et al. Efficacy and Safety of Anti-PD-1 Immunotherapy in Patients With Advanced NSCLC With BRAF, HER2, or MET Mutations or RET Translocation: GFPC 01-2018. J Thorac Oncol 2020;15:628-36. [Crossref] [PubMed]
- Mazieres J, Drilon A, Lusque A, et al. Immune checkpoint inhibitors for patients with advanced lung cancer and oncogenic driver alterations: results from the IMMUNOTARGET registry. Ann Oncol 2019;30:1321-8. [Crossref] [PubMed]
- Gainor JF, Shaw AT, Sequist LV, et al. EGFR Mutations and ALK Rearrangements Are Associated with Low Response Rates to PD-1 Pathway Blockade in Non-Small Cell Lung Cancer: A Retrospective Analysis. Clin Cancer Res 2016;22:4585-93. [Crossref] [PubMed]
- Lu C, Dong XR, Zhao J, et al. Association of genetic and immuno-characteristics with clinical outcomes in patients with RET-rearranged non-small cell lung cancer: a retrospective multicenter study. J Hematol Oncol 2020;13:37. [Crossref] [PubMed]
- Choudhury NJ, Schneider JL, Patil T, et al. Response to Immune Checkpoint Inhibition as Monotherapy or in Combination With Chemotherapy in Metastatic ROS1-Rearranged Lung Cancers. JTO Clin Res Rep 2021;2:100187. [Crossref] [PubMed]
- Guo X, Du H, Li J, et al. Efficacy of ICIs on patients with oncogene-driven non-small cell lung cancer: a retrospective study. Cancer Drug Resist 2022;5:15-24. [Crossref] [PubMed]
- Society of Cancer Precision Medicine of Chinese Anti-Cancer Association. Chinese expert consensus on immunotherapy for advanced non-small lung cancer with oncogenic driver mutations (2023 edition). Zhonghua Zhong Liu Za Zhi 2023;45:717-40. [PubMed]
- Eisenhauer EA, Therasse P, Bogaerts J, et al. New response evaluation criteria in solid tumours: revised RECIST guideline (version 1.1). Eur J Cancer 2009;45:228-47. [Crossref] [PubMed]
- Rittmeyer A, Barlesi F, Waterkamp D, et al. Atezolizumab versus docetaxel in patients with previously treated non-small-cell lung cancer (OAK): a phase 3, open-label, multicentre randomised controlled trial. Lancet 2017;389:255-65. [Crossref] [PubMed]
- Xia L, Liu Y, Wang Y. PD-1/PD-L1 Blockade Therapy in Advanced Non-Small-Cell Lung Cancer: Current Status and Future Directions. Oncologist 2019;24:S31-41. [Crossref] [PubMed]
- Wood K, Hensing T, Malik R, et al. Prognostic and Predictive Value in KRAS in Non-Small-Cell Lung Cancer: A Review. JAMA Oncol 2016;2:805-12. [Crossref] [PubMed]
- Nakajima EC, Drezner N, Li X, et al. FDA Approval Summary: Sotorasib for KRAS G12C-Mutated Metastatic NSCLC. Clin Cancer Res 2022;28:1482-6. [Crossref] [PubMed]
- Doroshow DB, Bhalla S, Beasley MB, et al. PD-L1 as a biomarker of response to immune-checkpoint inhibitors. Nat Rev Clin Oncol 2021;18:345-62. [Crossref] [PubMed]
- Mazieres J, Vioix H, Pfeiffer BM, et al. MET Exon 14 Skipping in NSCLC: A Systematic Literature Review of Epidemiology, Clinical Characteristics, and Outcomes. Clin Lung Cancer 2023;24:483-97. [Crossref] [PubMed]
- Planchard D, Smit EF, Groen HJM, et al. Dabrafenib plus trametinib in patients with previously untreated BRAF(V600E)-mutant metastatic non-small-cell lung cancer: an open-label, phase 2 trial. Lancet Oncol 2017;18:1307-16. [Crossref] [PubMed]
- Griesinger F, Curigliano G, Thomas M, et al. Safety and efficacy of pralsetinib in RET fusion-positive non-small-cell lung cancer including as first-line therapy: update from the ARROW trial. Ann Oncol 2022;33:1168-78. [Crossref] [PubMed]
- Spigel DR, Reynolds C, Waterhouse D, et al. Phase 1/2 Study of the Safety and Tolerability of Nivolumab Plus Crizotinib for the First-Line Treatment of Anaplastic Lymphoma Kinase Translocation - Positive Advanced Non-Small Cell Lung Cancer (CheckMate 370). J Thorac Oncol 2018;13:682-8. [Crossref] [PubMed]
- Gibbons DL, Chow LQ, Kim DW, et al. Efficacy, safety and tolerability of MEDI4736 (durvalumab [D]), a human IgG1 anti-programmed cell death-ligand-1 (PD-L1) antibody, combined with gefitinib (G): A phase I expansion in TKI-naïve patients (pts) with EGFR mutant NSCLC. J Thorac Oncol 2016;11:S79. [Crossref]
- Felip E, De Braud FG, Maur M, et al. Ceritinib plus nivolumab (NIVO) in patients (pts) with anaplastic lymphoma kinase positive (ALK+) advanced non-small cell lung cancer (NSCLC). J Clin Oncol 2017;35:2502. [Crossref]
- Oshima Y, Tanimoto T, Yuji K, et al. EGFR-TKI-Associated Interstitial Pneumonitis in Nivolumab-Treated Patients With Non-Small Cell Lung Cancer. JAMA Oncol 2018;4:1112-5. [Crossref] [PubMed]
- Lisberg A, Cummings A, Goldman JW, et al. A Phase II Study of Pembrolizumab in EGFR-Mutant, PD-L1+, Tyrosine Kinase Inhibitor Naïve Patients With Advanced NSCLC. J Thorac Oncol 2018;13:1138-45. [Crossref] [PubMed]
- Chour A, Denis J, Mascaux C, et al. Brief Report: Severe Sotorasib-Related Hepatotoxicity and Non-Liver Adverse Events Associated With Sequential Anti-Programmed Cell Death (Ligand)1 and Sotorasib Therapy in KRAS(G12C)-Mutant Lung Cancer. J Thorac Oncol 2023;18:1408-15. [Crossref] [PubMed]