Entrectinib as first-line vs. second-line therapy in ROS1 fusion-positive non-small cell lung cancer: a cost-effectiveness analysis
Highlight box
Key findings
• Compared to chemotherapy, the utilization of entrectinib as a first-line therapy yielded an additional 0.07 quality-adjusted life years (QALYs) at a cost of $113,738, resulting in an incremental cost-effectiveness ratio (ICER) of $1,090,594.30 per QALY.
• In the setting of second-line treatment, entrectinib demonstrated an incremental gain of 0.11 QALYs at a cost of $93,766, leading to an ICER of $494,290.39 per QALY.
What is known and what is new?
• The monthly price of entrectinib stands at approximately $20,109.6, which is considerably high.
• This study represents the inaugural endeavor to assess the cost-effectiveness of entrectinib for managing patients with ROS1 fusion-positive advanced non-small cell lung cancer (NSCLC).
What is the implication, and what should change now?
• Compared to chemotherapy, entrectinib did not demonstrate cost-effectiveness for patients with ROS1 fusion-positive advanced NSCLC, whether as a first-line or second-line therapy. Adopting a strategy of reserving entrectinib until second-line therapy could be a compromise. This approach aims to maintain control over healthcare expenses while still achieving favorable clinical outcomes.
Introduction
In the United States, lung cancer has the highest rate of occurrence among malignant tumors, with 238,340 new cases and 127,070 deaths from the disease reported annually (1). Non-small cell lung cancer (NSCLC), which accounts for 85% of cases, typically has an unfavorable prognosis (2). The majority of patients are diagnosed at advanced stages or have already encountered metastasis at the time of initial diagnosis (3). ROS1 gene fusions are found in NSCLC, occurring in approximately 1–2% of cases (4-6). This modification is more commonly observed in adenocarcinoma, particularly among younger patients who have either never smoked or have a minimal smoking history (7). This fusion results in the continuous activation of kinase activity, leading to the overexpression of MAPK/ERK, PI3K/AKT, and JAK/STAT signaling pathways, and ultimately enhancing cellular proliferation, survival, and migration (8,9).
Entrectinib is an oral potent multikinase blocking agent that has demonstrated efficacy against ROS1, as well as tropomyosin receptor kinase (TRK) A, B and C and ALK (10). It has exhibited impressive and long-lasting efficacy in individuals diagnosed with ROS1 fusion (+) NSCLC (11,12). In the phase I/II registrational trial STARTRK-1, STARTRK-2, and ALKA-372-001, entrectinib showed durable disease control and favorable tolerability in individuals with ROS1 fusion (+) advanced NSCLC (12). Entrectinib was granted U.S. Food and Drug Administration approval on August 15, 2019 for the treatment of metastatic NSCLC with ROS1 fusion (+) status, and it has since been granted regulatory approval in several other regions (13).
Taking into account the possibility of prolonged treatment duration and a monthly cost of approximately $20,109.60 (14), cost-effectiveness should be an important consideration when making decisions about health plan coverage (15). Data regarding the costs and efficacy of entrectinib can provide valuable insights for making decisions about clinical practices and coverage. However, the cost-effectiveness of this treatment has not been evaluated within the high-cost U.S. healthcare system and the presence of other competing demands for innovative services. We present this article in accordance with the CHEERS reporting checklist (available at https://tlcr.amegroups.com/article/view/10.21037/tlcr-24-8/rc).
Methods
Model structure
We utilized the software of TreeAge Pro 2022 to execute this Markov model assessing pharmacoeconomic characteristics. R software was also utilized for statistical analyses. Figure 1 illustrates the transition state diagram, which outlines our Markov model’s five distinct and mutually independent health states: progression-free survival (PFS), disease progression, subsequent disease progression, end stage disease, and death. To simulate the participants enrolled in the STARTRK-1, STARTRK-2, and ALKA-372-001 trials (12), a notional group of individuals with advanced NSCLC positive for ROS1 fusion was constructed. Based on the recommended dosage, all patients were administered a daily quantity of 600 mg of entrectinib. The diagram in Figure 1 examines a total of three treatment strategies: (I) implementing entrectinib as the first-line treatment option; (II) implementing entrectinib as the second-line treatment option; and (III) choosing chemotherapy as the sole treatment approach. Our study included advanced/metastatic ROS1 fusion (+) NSCLC patients who had not received tyrosine kinase inhibitor (TKI) therapy before, with a median age of 60. The treatment regimen was adopted based on the guidelines from National Comprehensive Cancer Network (NCCN) (16). In the entrectinib treatment arm, individuals who had disease progression during the first-line treatment with entrectinib were subsequently administered second-line pemetrexed based chemotherapy. Conversely, patients who experienced progression while receiving first-line chemotherapy were subsequently treated with second-line entrectinib (17). The third-line treatment was consistent across both arms, where it was simulated as docetaxel chemotherapy (18). In chemotherapy arm, individuals who experienced disease progression following pemetrexed-based chemotherapy were subsequently treated with docetaxel chemotherapy (18). Patients who encounter cancer progression while undergoing docetaxel chemotherapy would undergo a transition to an end stage disease status and opt for best supportive care until the end of life (Figure 1).
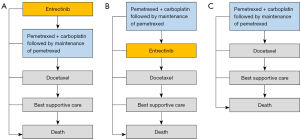
Our study solely examined the direct medical costs, with each model having a defined cycle length of 3 weeks, similar to the interval between chemotherapy cycles. We employed a lifetime horizon to assess the cost and effectiveness based on each therapeutic regimen. Given the assumption that expenses were accrued at the start of each cycle, there was no cost adjustment for half of the cycle (19). The main outcomes encompassed costs, utilities, quality-adjusted life years (QALYs), and incremental cost-effectiveness ratios (ICERs), which are subject to an annual discount rate of 3% (20). The cost-effectiveness of the treatment was assessed based on a willingness-to-pay (WTP) threshold of $150,000/QALY, aligning with the recommendation made by Neumann et al. (21).
The cost-effectiveness analysis approach was employed to assess and compare the utility and cost of three different therapeutic regimens. The ICERs formula quantifies the ratio between the cost and effectiveness disparities among the various alternatives, represented as:
C represents the cost of treatment and E represents the effectiveness of treatment in the formula.
Model survival and progression risk assessment
The potential risks associated with each treatment regimen were evaluated based on the corresponding clinical trials. The GetData Graph Digitizer and R software were used to extrapolate the transition probability over a lifetime horizon from the PFS Kaplan-Meier curves obtained from the ALKA-372-001, STARTRK-1, STARTRK-2, PARAMOUNT, IFCT-1103 ULTIMATE studies (12,17,18), as well as the survival curve of best supportive care (22). We generated simulated patient-level data utilizing the algorithm devised by Hoyle et al. to enhance the precision of average survival assessments (23,24). The pseudo-individual patient data were used to fit corresponding distributions, employing various functions of survival such as Gamma, Gengamma, Weibull, Exponential, Loglogistic, Gompertz, and Lognormal. The best model fits were selected using the Akaike and Bayesian information criteria. Model fit status can be found in Table S1 and Figure S1. Subsequently, this model parameters were employed to assess the transition probabilities between the Markov states. The usage of U.S. life tables in this study is adopted to estimate background mortality rates for each age (Table S2) (25).
Cost estimates
We took into account the healthcare resources utilization and the associated direct medical costs, which encompassed costs related to drug procurement and administration, disease management, as well as adverse events (AEs) associated with the treatment regimen (Table S3). The calculation of drug dosage was performed using a body weight of 70 kg and a body surface area of 1.82 m2 (20,26). In our model, we considered only Grade ≥3 AEs with an incidence rate higher than 4%.
The methodology for cost calculations in this study was based on the framework proposed by Tumeh et al. (27). The costs related to procuring entrectinib, pemetrexed, cisplatin, docetaxel, and follow-up therapies were obtained from publicly available databases, which were all current as of May 2023 (14,28). The estimated administration costs were according to the 2023 Centers for Medicare and Medicaid Service Physician Fee Schedule (29). The expenses resulting from AEs, end-of-life palliative care, and best supportive care were obtained from previously published research (30,31). The assumption was made that individuals only received treatment during the first cycle after the onset of AEs, and the cost associated with these AE was incurred as a one-time occurrence. Administration costs in this manuscript refer to ward management and administrative expenses, which are only recorded once per cycle for treatments requiring intravenous medication administered in a hospital setting. As oral medications do not necessitate hospitalization, their associated expenses are not included in the administration costs (29). Laboratory testing costs and physician visit costs were measured at a frequency of once per treatment cycle (20,26,31), whereas tumor imaging costs were documented every two treatment cycles (20). Adjustments for inflation were made using the U.S. Consumer Price Index, allowing for the presentation of costs in 2023 U.S. dollars when applicable.
Utility estimates
The measurement of treatment effectiveness was based on QALYs, which is a composite score obtained by assigning American-specific utility values to different health states. Health utility values ranging from 0, representing dead, to 1, representing perfect health, were derived for different health states in NSCLC based on existing literature on American NSCLC patients (32). The health states considered include PFS, disease progression after 1st-line treatment, 2nd-line therapy, 3rd-line therapy, and death. The corresponding health utility values were found to be 0.71, 0.67, 0.59, 0.46, and 0, respectively (33). To quantify the impact of AEs on QALYs, we computed the product of AE incidences and their corresponding disutilities (34-36). The resulting overall decrease in QALYs attributed to all AEs was integrated into the 1st cycle of the model (37). Table S3 provides a comprehensive compilation of parameters pertaining to these utilities.
Sensitivity analyses
To assess the influence of parameter uncertainty on outcomes, sensitivity analyses were conducted. Within the univariate sensitivity analyses, we varied clinical parameters within reasonable ranges, with a ±20% from the baseline. The lower and upper bounds specified in Table S3 were employed to adjust each parameter. In order to conduct probability sensitivity analyses (PSA) and examine the impact of price fluctuations on ICERs, a series of 1,000 Monte Carlo simulations were conducted based on specific distribution patterns (Table S3). To evaluate the cost-effectiveness of each strategy, scatter plots and cost-effectiveness acceptability curves were employed, using a WTP threshold of $150,000 per QALY (26,38).
Results
Model validation
In terms of PFS, the findings from our model are in line with the respective clinical studies, as indicated by the simulated clinical results (Figure S1). The median PFS derived from this model does not significantly deviate from the PFS data reported in clinical trials (Table S4).
Base case results
Based on the analysis, the mean costs of entrectinib as a first-line treatment, second-line treatment, and chemotherapy were $113,738, $93,766, and $40,286 per patient, respectively. The corresponding QALYs for patients in these three arms were 0.44, 0.49, and 0.38, respectively. When utilized as a first-line treatment, entrectinib resulted in an incremental gain of 0.07 QALYs at an incremental cost of $73,453 compared to chemotherapy, yielding an ICER of $1,090,594.30 per QALY. Similarly, as a second−line treatment compared to chemotherapy, entrectinib offered an incremental gain of 0.11 QALYs at an incremental cost of $53,480, resulting in an ICER of $494,290.39 per QALY (Table 1). Both ICER values exceed the predetermined WTP threshold of $150,000 per QALY, indicating that entrectinib is not cost-effective compared to chemotherapy (Table 1).
Table 1
Arm | Mean costs, US $ | ∆Costs, US $ | QALYs | ∆QALYs | ICER US $/QALY |
---|---|---|---|---|---|
Chemotherapy | 40,286 | – | 0.38 | – | – |
Entrectinib 1st line | 113,738 | 73,453 | 0.44 | 0.07 | 1,090,594.30 |
Entrectinib 2nd line | 93,766 | 53,480 | 0.49 | 0.11 | 494,290.39 |
QALY, quality-adjusted life years; ICER, incremental cost-effectiveness ratio.
Sensitivity analyses
The tornado diagrams (Figure 2) presented the results of univariable sensitivity analyses, illustrating the impact of various parameters on the ICERs. Among these parameters, both the cost of entrectinib and the utility values associated with progressed disease emerged as the most significant factors. Despite considerable variations in the values of each parameter, the ICER consistently exceeded $150,000/QALY. The influence of anemia costs and discount rate in the first-line treatment model, as well as the effect of discount rate and end-of-life care expenses in the second-line model, had a relatively minor impact on the ICER. The impact of other variables was found to be negligible.
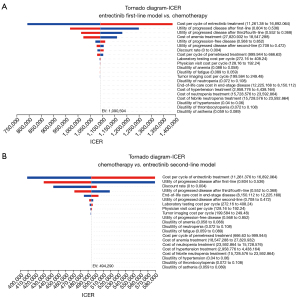
In the PSA, a scatter plot (Figure 3) shows the ICER for 1,000 samples. The four quadrants of the plot represent various combinations of costs and effectiveness, while a line denotes the threshold of $150,000/QALY. Across all models, it is evident that the respective data points are consistently positioned above the $150,000/QALY threshold. The cost-effectiveness acceptability curves (Figure 4) illustrate the different values of WTP per QALY. These curves suggest that, when comparing three therapy regimens simultaneously, neither the 1st-line nor the 2nd-line entrectinib is considered cost-effective at the WTP threshold of $150,000/QALY.
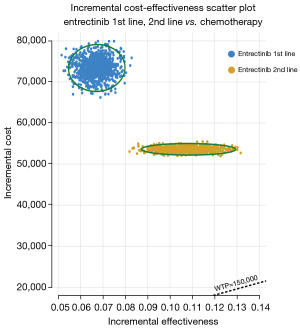
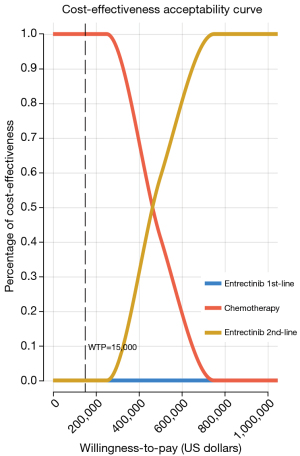
Discussion
As far as we know, this analysis was the first study to estimate the cost-effectiveness of entrectinib for individuals with ROS1 fusion (+) advanced NSCLC. Our analytical model provides a framework for managing this disease and serves as a versatile tool for assessing the cost-effectiveness of entrectinib across different treatment scenarios.
Targeted therapy has demonstrated significant potential as a treatment approach for individuals diagnosed with ROS1 fusion-positive advanced NSCLC, whether as first-line or second-line therapy. However, determining optimal treatment regimens that balance economic efficiency with therapeutic efficacy poses a challenge for oncologists. This underscores the importance of conducting a cost-effectiveness evaluation to facilitate informed decision-making. Based on our model, we found that entrectinib offered some advantages as both first-line and second-line therapy. Nonetheless, these benefits came at a significantly higher cost per QALY. Our PSA analysis revealed that entrectinib was not considered cost-effective as either first- or second-line therapy, given a WTP threshold of $150,000/QALY.
In our model, the primary factors determining cost-effectiveness were the price of entrectinib and the utility values assigned to PFS and progressive disease (PD). Therefore, in order for the entrectinib strategy to be more cost-effective than chemotherapy, the price of entrectinib needs to be reduced in both 1st-line and 2nd-line settings. Utility values for PFS and PD were determined using health utility data as reported in a previous study (33). To prevent undue influence of specific utility values on our findings, we expanded the range of these values in our sensitivity analyses. Our results suggest that variations in utility values had no impact on the conclusion about the overall cost-effectiveness. Furthermore, whether considered for first- or second-line therapy, entrectinib appeared not to be cost-effective across the spectrum of utility values tested.
Previous studies have shown that crizotinib is not cost-effective as a first-line treatment for ROS1+ NSCLC in Canada (39). Compared to our research on entrectinib in the United States, although entrectinib is not cost-effective in either the first- or second-line settings, the ICER for second-line treatment is relatively more favorable, being lower than that for first-line treatment (first-line $1,090,594.30 vs. second-line $494,290.39/QALY). This suggests that it could be considered as a compromise solution.
In this current era of personalized medicine, it is crucial to address the unique needs of individuals diagnosed with ROS1 fusion (+) NSCLC or other malignant tumors and accordingly develop innovative therapies. However, there has been a substantial surge in drug expenses over the past few years, making it crucial to tailor the use of novel treatments in a way that maximizes cost-effectiveness for these specific individuals. In light of these circumstances, coupled with the constant rise in new cases of NSCLC, additional research efforts are warranted to generate valuable data that can inform the appropriate allocation of healthcare resources. It becomes essential to prioritize resource allocation and ensure that the benefits of expensive drugs are maximized.
It is worth mentioning that over half of patients with genetic alterations do not receive the targeted therapy recommended by clinical guidelines (40). This suboptimal utilization may result from economic constraints, causing some patients to opt for chemotherapy as an alternative. Additionally, the implementation of targeted therapy in certain patient populations may be hindered, which in turn leads to higher rates of dose reduction and treatment discontinuation (6).
The rising cost of newly registered oncology drugs poses a serious threat to the sustainability of national health service systems, particularly in countries with limited public control and cost oversight, such as the United States (41). This trend underscores the need for transparency and value-based pricing in the pharmaceutical industry.
To address this issue, pharmaceutical companies should disclose their research and development costs. Ensuring that the pricing of new treatments reflects not only their added benefits but also considers the societal and personal costs is crucial. This approach emphasizes the importance of a ‘just price’ that aligns with the true value of the treatments (41,42).
Furthermore, efforts should be made to explore various options for reducing the financial burden on patients. These could include insurance coverage programs or patient assistance initiatives designed to make these critical treatments more accessible.
Additionally, it is worth noting that immune checkpoint inhibitors (ICIs) targeting programmed cell death protein 1 (PD-1) or its ligand (PD-L1), can be employed either alone or alongside platinum-based chemotherapy for patients lacking driver gene mutations (43). However, in patients with ROS1 fusion-positive NSCLC, the efficacy of ICIs remains unsatisfactory, regardless of PD-L1 expression (44). Therefore, we did not pursue the inclusion of ICIs in our cost-effectiveness analysis.
Our study, like all models, also has certain limitations that partly depend on data availability and our underlying assumptions (45). First, the reliability of our model heavily depends on findings from the STARTRK-1, STARTRK-2, and ALKA-372-001 trials. Conducting large-scale phase III clinical trials is challenging due to the rarity of ROS1 fusion-positive NSCLC and its small patient population. Therefore, our model is based on single-arm phase I/II clinical studies of entrectinib. Any inherent biases in these trials will also be reflected in our model. Second, treatment regimen choices may vary depending on individual patient characteristics, emphasizing the need to incorporate real-world research data for long-term model validation. Third, our model employed certain simplifications that limited its scope. To estimate progression rates, we incorporated survival data from various clinical study cohorts. To address this concern, we conducted sensitivity analyses that considered a wide range of potential costs related to entrectinib.
Conclusions
Entrectinib has shown significant efficacy in treating ROS1 fusion (+) NSCLC. However, it is important to consider that this treatment option also incurs substantial additional costs per QALY for both 1st-line and 2nd-line therapies from the perspective of the U.S. payer. Delaying the use of entrectinib to second-line therapy could be a potential strategy to balance healthcare costs without compromising positive clinical outcomes.
Acknowledgments
Funding: This work was funded by
Footnote
Reporting Checklist: The authors have completed the CHEERS reporting checklist. Available at https://tlcr.amegroups.com/article/view/10.21037/tlcr-24-8/rc
Peer Review File: Available at https://tlcr.amegroups.com/article/view/10.21037/tlcr-24-8/prf
Conflicts of Interest: All authors have completed the ICMJE uniform disclosure form (available at https://tlcr.amegroups.com/article/view/10.21037/tlcr-24-8/coif). The authors have no conflicts of interest to declare.
Ethical Statement: The authors are accountable for all aspects of the work in ensuring that questions related to the accuracy or integrity of any part of the work are appropriately investigated and resolved.
Open Access Statement: This is an Open Access article distributed in accordance with the Creative Commons Attribution-NonCommercial-NoDerivs 4.0 International License (CC BY-NC-ND 4.0), which permits the non-commercial replication and distribution of the article with the strict proviso that no changes or edits are made and the original work is properly cited (including links to both the formal publication through the relevant DOI and the license). See: https://creativecommons.org/licenses/by-nc-nd/4.0/.
References
- Siegel RL, Miller KD, Wagle NS, et al. Cancer statistics, 2023. CA Cancer J Clin 2023;73:17-48. [Crossref] [PubMed]
- de Groot PM, Wu CC, Carter BW, et al. The epidemiology of lung cancer. Transl Lung Cancer Res 2018;7:220-33. [Crossref] [PubMed]
- Reade CA, Ganti AK. EGFR targeted therapy in non-small cell lung cancer: potential role of cetuximab. Biologics 2009;3:215-24.
- Lipson D, Capelletti M, Yelensky R, et al. Identification of new ALK and RET gene fusions from colorectal and lung cancer biopsies. Nat Med 2012;18:382-4. [Crossref] [PubMed]
- Tsuta K, Kohno T, Yoshida A, et al. RET-rearranged non-small-cell lung carcinoma: a clinicopathological and molecular analysis. Br J Cancer 2014;110:1571-8. [Crossref] [PubMed]
- Drilon A, Hu ZI, Lai GGY, et al. Targeting RET-driven cancers: lessons from evolving preclinical and clinical landscapes. Nat Rev Clin Oncol 2018;15:151-67. [Crossref] [PubMed]
- Lin JJ, Shaw AT. Recent Advances in Targeting ROS1 in Lung Cancer. J Thorac Oncol 2017;12:1611-25. [Crossref] [PubMed]
- Roskoski R Jr. ROS1 protein-tyrosine kinase inhibitors in the treatment of ROS1 fusion protein-driven non-small cell lung cancers. Pharmacol Res 2017;121:202-12. [Crossref] [PubMed]
- Rosell R, Jain A, Codony-Servat J, et al. Biological insights in non-small cell lung cancer. Cancer Biol Med 2023;20:500-18. [Crossref] [PubMed]
- Drilon A, Siena S, Ou SI, et al. Safety and Antitumor Activity of the Multitargeted Pan-TRK, ROS1, and ALK Inhibitor Entrectinib: Combined Results from Two Phase I Trials (ALKA-372-001 and STARTRK-1). Cancer Discov 2017;7:400-9. [Crossref] [PubMed]
- Drilon A, Chiu CH, Fan Y, et al. Long-Term Efficacy and Safety of Entrectinib in ROS1 Fusion-Positive NSCLC. JTO Clin Res Rep 2022;3:100332. [Crossref] [PubMed]
- Drilon A, Siena S, Dziadziuszko R, et al. Entrectinib in ROS1 fusion-positive non-small-cell lung cancer: integrated analysis of three phase 1-2 trials. Lancet Oncol 2020;21:261-70. [Crossref] [PubMed]
- Jiang Q, Li M, Li H, et al. Entrectinib, a new multi-target inhibitor for cancer therapy. Biomed Pharmacother 2022;150:112974. [Crossref] [PubMed]
- Drugs.com. Drug Price Information. Accessed May 10, 2023. Available online: https://www.drugs.com/price-guide/
- Wickwire EM, Shaya FT, Scharf SM. Health economics of insomnia treatments: The return on investment for a good night's sleep. Sleep Med Rev 2016;30:72-82. [Crossref] [PubMed]
- National Comprehensive Cancer Network. Clinical Practice Guidelines in Oncology: Colon Cancer. 2023.
- Paz-Ares L, de Marinis F, Dediu M, et al. Maintenance therapy with pemetrexed plus best supportive care versus placebo plus best supportive care after induction therapy with pemetrexed plus cisplatin for advanced non-squamous non-small-cell lung cancer (PARAMOUNT): a double-blind, phase 3, randomised controlled trial. Lancet Oncol 2012;13:247-55. [Crossref] [PubMed]
- Cortot AB, Audigier-Valette C, Molinier O, et al. Weekly paclitaxel plus bevacizumab versus docetaxel as second- or third-line treatment in advanced non-squamous non-small-cell lung cancer: Results of the IFCT-1103 ULTIMATE study. Eur J Cancer 2020;131:27-36. [Crossref] [PubMed]
- Wu B, Chen H, Shen J, et al. Cost-effectiveness of adding rh-endostatin to first-line chemotherapy in patients with advanced non-small-cell lung cancer in China. Clin Ther 2011;33:1446-55. [Crossref] [PubMed]
- Ding D, Hu H, Li S, et al. Cost-Effectiveness Analysis of Durvalumab Plus Chemotherapy in the First-Line Treatment of Extensive-Stage Small Cell Lung Cancer. J Natl Compr Canc Netw 2021;19:1141-7. [Crossref] [PubMed]
- Neumann PJ, Cohen JT, Weinstein MC. Updating cost-effectiveness--the curious resilience of the $50,000-per-QALY threshold. N Engl J Med 2014;371:796-7. [Crossref] [PubMed]
- Shepherd FA, Dancey J, Ramlau R, et al. Prospective randomized trial of docetaxel versus best supportive care in patients with non-small-cell lung cancer previously treated with platinum-based chemotherapy. J Clin Oncol 2000;18:2095-103. [Crossref] [PubMed]
- Hoyle MW, Henley W. Improved curve fits to summary survival data: application to economic evaluation of health technologies. BMC Med Res Methodol 2011;11:139. [Crossref] [PubMed]
- Wan X, Peng L, Li Y. A review and comparison of methods for recreating individual patient data from published Kaplan-Meier survival curves for economic evaluations: a simulation study. PLoS One 2015;10:e0121353. [Crossref] [PubMed]
- Arias E, Heron M, Xu J. United States Life Tables, 2014. Natl Vital Stat Rep 2017;66:1-64.
- Lin S, Luo S, Zhong L, et al. Cost-effectiveness of atezolizumab plus chemotherapy for advanced non-small-cell lung cancer. Int J Clin Pharm 2020;42:1175-83. [Crossref] [PubMed]
- Tumeh JW, Moore SG, Shapiro R, et al. Practical approach for using Medicare data to estimate costs for cost-effectiveness analysis. Expert Rev Pharmacoecon Outcomes Res 2005;5:153-62. [Crossref] [PubMed]
- CMS. The Centers for Medicare & Medicaid Services. Accessed May 10, 2023. Available online: https://www.cms.gov/
- CMS. Centers for Medicare & Medicaid Service. Physician Fee Schedule. Accessed May 10, 2023. Available online: https://www.cms.gov/medicare/payment/fee-schedules/physician
- Wan X, Luo X, Tan C, et al. First-line atezolizumab in addition to bevacizumab plus chemotherapy for metastatic, nonsquamous non-small cell lung cancer: A United States-based cost-effectiveness analysis. Cancer 2019;125:3526-34. [Crossref] [PubMed]
- Liu Q, Luo X, Yi L, et al. First-Line Chemo-Immunotherapy for Extensive-Stage Small-Cell Lung Cancer: A United States-Based Cost-Effectiveness Analysis. Front Oncol 2021;11:699781. [Crossref] [PubMed]
- Whitehead SJ, Ali S. Health outcomes in economic evaluation: the QALY and utilities. Br Med Bull 2010;96:5-21. [Crossref] [PubMed]
- Chouaid C, Agulnik J, Goker E, et al. Health-related quality of life and utility in patients with advanced non-small-cell lung cancer: a prospective cross-sectional patient survey in a real-world setting. J Thorac Oncol 2013;8:997-1003. [Crossref] [PubMed]
- Nafees B, Stafford M, Gavriel S, et al. Health state utilities for non small cell lung cancer. Health Qual Life Outcomes 2008;6:84. [Crossref] [PubMed]
- Westwood M, Joore M, Whiting P, et al. Epidermal growth factor receptor tyrosine kinase (EGFR-TK) mutation testing in adults with locally advanced or metastatic non-small cell lung cancer: a systematic review and cost-effectiveness analysis. Health Technol Assess 2014;18:1-166. [Crossref] [PubMed]
- Nafees B, Lloyd AJ, Dewilde S, et al. Health state utilities in non-small cell lung cancer: An international study. Asia Pac J Clin Oncol 2017;13:e195-203. [Crossref] [PubMed]
- Su D, Wu B, Shi L. Cost-effectiveness of Atezolizumab Plus Bevacizumab vs Sorafenib as First-Line Treatment of Unresectable Hepatocellular Carcinoma. JAMA Netw Open 2021;4:e210037. [Crossref] [PubMed]
- Rabin R, de Charro F. EQ-5D: a measure of health status from the EuroQol Group. Ann Med 2001;33:337-43. [Crossref] [PubMed]
- Beca JM, Walsh S, Raza K, et al. Cost-effectiveness analysis of first-line treatment with crizotinib in ROS1-rearranged advanced non-small cell lung cancer (NSCLC) in Canada. BMC Cancer 2021;21:1162. [Crossref] [PubMed]
- Singal G, Miller PG, Agarwala V, et al. Association of Patient Characteristics and Tumor Genomics With Clinical Outcomes Among Patients With Non-Small Cell Lung Cancer Using a Clinicogenomic Database. JAMA 2019;321:1391-9. [Crossref] [PubMed]
- Giuliani J, Bonetti A. Pharmacologic Costs of Tyrosine Kinase Inhibitors in First-Line Therapy for Advanced Non-Small-Cell Lung Cancer With Activating Epidermal Growth Factor Receptor Mutations: A Review of Pivotal Phase III Randomized Controlled Trials. Clin Lung Cancer 2016;17:91-4. [Crossref] [PubMed]
- Bonetti A, Giuliani J. Implications of drugs with rebate in Europe. Lancet Reg Health Eur 2021;3:100060. [Crossref] [PubMed]
- European Society for Medical Oncology. Metastatic non-small cell lung cancer: ESMO Clinical Practice Guidelines for diagnosis, treatment and follow-up. 2020. Accessed May 10, 2023. Available online: https://www.esmo.org/content/download/347819/6934778/1/ESMO-CPGmNSCLC-15SEPT2020.pdf
- Seegobin K, Majeed U, Wiest N, et al. Immunotherapy in Non-Small Cell Lung Cancer With Actionable Mutations Other Than EGFR. Front Oncol 2021;11:750657. [Crossref] [PubMed]
- Husereau D, Drummond M, Petrou S, et al. Consolidated Health Economic Evaluation Reporting Standards (CHEERS)--explanation and elaboration: a report of the ISPOR Health Economic Evaluation Publication Guidelines Good Reporting Practices Task Force. Value Health 2013;16:231-50. [Crossref] [PubMed]