Potential predictors of the pathologic response after neoadjuvant chemoimmunotherapy in resectable non-small cell lung cancer: a narrative review
Introduction
Currently, immunotherapy is considered the standard of care for most cancers, thereby contributing to long-term patient survival. Patients with non-small cell lung cancer (NSCLC) are candidates for immune checkpoint inhibitors (ICIs), including anti-programmed death-1 (PD-1)/programmed death ligand-1 (PD-L1) antibodies. Notably, ICI therapy has been approved for both early and late-stage patients with NSCLC (1-4). Recently, neoadjuvant therapy comprising platinum-based chemotherapy plus PD-1 blockade with nivolumab (chemoimmunotherapy) was identified as a standard regimen for patients with resectable NSCLC (5). The CheckMate 816 trial compared chemotherapy plus nivolumab regimen with chemotherapy alone in terms of the pathological complete response (pCR), revealing rates of 24% and 2.2%, respectively (P<0.001). Moreover, the event-free survival (EFS) in the chemotherapy plus nivolumab group was significantly superior to that in the chemotherapy alone group (31.6 vs. 20.8 months, P=0.005) (5). Patients who achieved pCR had a favorable prognosis after neoadjuvant chemoimmunotherapy (NACI) treatment. In contrast, the frequency of recurrence post-surgery was markedly increased in those who failed to achieve pCR (5). An explorative analysis of the CheckMate 816 trial revealed that the trends of circulating tumor DNA (ctDNA) immediately before NACI could help predict pCR (6). However, ctDNA was not detected in 75% of the eligible patients, highlighting major concerns regarding the clinical usefulness of ctDNA as a predictor of pCR. Although predicting pCR based on morphological changes observed in radiological imaging can be challenging, the Response Evaluation Criteria in Solid Tumors (RECIST) revealed complete response (CR) rates of 0.6% and 1.7% for chemoimmunotherapy and chemotherapy, respectively, without significant differences in the CheckMate 816 trial (5). Moreover, curative thoracic surgery was not performed in ~15% to 20% of eligible patients who experienced exacerbations after NACI (5). Considering the results of the CheckMate 816 trial, the optimal prediction of pCR could impact the potential of curative surgery and consecutive administration of chemoimmunotherapy, thereby identifying patients as non-candidates for NACI. A review of 20,000 patients with breast cancer in the National Cancer Database (NCDB) indicated that patients who achieved pCR after neoadjuvant chemotherapy had a survival benefit (7). Although the concept of pCR has been previously established in patients with breast cancer receiving neoadjuvant chemotherapy, biomarkers for predicting the therapeutic efficacy of NACI in patients with resectable NSCLC remain elusive.
Recent studies have focused on the optimal prediction of the major pathological response (MPR) and pCR after NACI in NSCLC (8-12). Notably, the presence of viable cells rather than the morphological changes needs to be elucidated for the optimal prediction of pathological response after NACI. Thus, 18F-fluorodeoxyglucose (18F-FDG) positron emission tomography (PET) is effective for evaluating metabolic tumor activity after any treatment (13,14). In terms of biological approaches, tumor microenvironment (TME), immunological blood analysis, or genetic alternations may be useful for predicting the therapeutic efficacy after NACI.
In this review, we aimed to summarize evidence from recent reports and highlight potential biomarkers for predicting the pathological response, including pCR, after NACI in patients with resectable NSCLC. We present this article in accordance with the Narrative Review reporting checklist (available at https://tlcr.amegroups.com/article/view/10.21037/tlcr-24-142/rc).
Methods
We identified research article published in English by using the PubMed database of the United States National Library of Medicine. A database search was done on December 12, 2023, and the search strategy included articles published between 2018 and 2022 using the following keywords: “chemoimmunotherapy” or “neoadjuvant” or “pathological complete response”; “pCR” or “major pathological response”; “MPR” or “non-small cell lung cancer”; “NSCLC” or “predictive marker” or “prognostic”. The search did not restrict the type of publication or periodical. We selected all published articles that clearly reported the predictive marker of pathological response in patients with resectable NSCLC receiving chemoimmunotherapy. The search strategy is summarized in Table 1.
Table 1
Items | Specification |
---|---|
Date of search | December 12, 2023 |
Databases and other sources searched | PubMed |
Search terms used | (chemoimmunotherapy[Title] or (neoadjuvant[Title]) or (pathological complete response [Title]) or (pCR [Title]) or (major pathological response [Title]) or (MPR [Title]) or (non-small cell lung cancer [Title]) or (NSCLC [Title]) or (predictive marker [Title]) and (prognostic [Title]) |
Timeframe | 2018 to 2022 |
Inclusion and exclusion criteria | Only full text articles published in English were included. Articles without relationship between pathological response and predictive marker were excluded |
Selection process | K.K. conducted selection independently, and the authors discussed the scientific and clinical value of controversial articles to reach an agreement |
NSCLC, non-small cell lung cancer.
Predictive biomarkers according to different modality
Therapeutic efficacy of NACI in clinical trials
Recent systematic reviews and meta-analyses have focused on the clinical efficacy of NACI for resectable NSCLC (15-20). Table 2 presents four studies reporting meta-analysis of neoadjuvant immunotherapy and NACI in resectable NSCLC. Wu et al. analyzed the pathological response after NACI in patients with resectable stage I–III NSCLC using 66 articles, including 8 randomized studies, 39 prospective nonrandomized studies, and 19 retrospective studies, revealing a pooled pCR of 28.1% (16). Although the authors included immunotherapy alone, immunotherapy plus radiation therapy (RT), and chemoimmunotherapy (as a regimen of neoadjuvant therapy), the MPR and pCR for chemoimmunotherapy ranged from 57% to 83% and from 18% to 63%, respectively (16). However, the efficacy of pathological response was non-uniform according to the individual studies, owing to the different sample sizes. Liu et al. focused on the NACI in patients with stage III NSCLC, and nine trials containing 382 patients were examined by performing a meta-analysis (15). The estimated pooled results for MPR and pCR were 56% and 39%, respectively, thereby suggesting that NACI is beneficial for stage III NSCLC (15). Chen et al. reviewed 53 trials (7 randomized, 29 prospective nonrandomized, and 17 retrospective trials) to validate the MPR after NACI in resectable stage I–II NSCLC. The authors revealed a pooled MPR of 53.8% and suggested that increased MPR may be associated with a survival benefit (19). Upon analyzing eight comparative studies of chemoimmunotherapy versus chemotherapy alone, the meta-analysis demonstrated that the MPR for chemoimmunotherapy was substantially higher than that of chemotherapy, without any information regarding the pCR (19). Jiang et al. performed a meta-analysis using a total of 988 patients from 16 trials to evaluate the efficacy and tolerability of neoadjuvant immunotherapy with single/combined ICI or chemoimmunotherapy (18). Considering all patients, the pooled MPR and pCR were 43.5% and 21.9%, respectively (18). However, patients receiving NACI had a markedly increased pathological response when compared with those treated with neoadjuvant single-agent immunotherapy (MPR: 53.3% vs. 28.6%; pCR: 28.6% vs. 9.9%) (18). These results emphasized that NACI could afford a substantial pathological response when compared with single-agent immunotherapy with slightly increasing toxicities. Overall, several previous meta-analyses have concluded that NACI can contribute to improvements in the pathological response of resectable NSCLC when compared with single-agent chemotherapy or immunotherapy.
Table 2
Ref. | Number of trials | No. of analyzed patients | Clinical stage | Regimens | MPR (%) | pCR (%) | Correlation of pCR or MPR with DFS | TRAEs (%) |
---|---|---|---|---|---|---|---|---|
(15) | 9 | 382 | III | Chemotherapy plus ICI | 56 | 39 | NA | 65 |
(16) | 66 | 2,205 | I–III | ICI | NA | 28.1 | Significant | NA |
(18) | 16 | 988 | I–III | Chemotherapy plus ICI or ICI | 43.5 | 21.9 | NA | 54.8 |
(19) | 53 | 2,205 | I–II | ICI | 53.8 | NA | Significant | NA |
ICI, immune checkpoint inhibitor; Ref. reference; No., number; MPR, major pathological response; pCR, pathological complete response; DFS, disease free-survival; TRAEs, treatment related adverse events; NA, not available.
Radiological assessment in 18F-FDG PET
Typically, radiological modalities are suitable for evaluating the therapeutic response in most patients with cancer. In clinical practice, computed tomography (CT) RECIST assessment is employed for therapeutic monitoring after any systemic treatment. In the CheckMate 816 trial, CR based on the RECIST and pCR after NACI were 0.6% and 24%, respectively, indicating considerable discrepancies between the obtained values (5). Accordingly, morphological changes after NACI may not be associated with pCR prediction. Therefore, “metabolic tumor activity” rather than “morphological change” could be an optimal assessment of residual viable cells. Considering all radiographic modalities, 18F-FDG PET has been deemed the most suitable to quantitatively analyze viable tumor cells. Compared with CT scan, 18F-FDG PET was found to be useful for therapeutic prediction of early response after immunotherapy in patients with advanced NSCLC (14,21).
Recent reports have highlighted the usefulness of 18F-FDG PET after NACI and before surgery for predicting the pathological response to neoadjuvant immunotherapy or NACI in patients with resectable NSCLC (8-12). For example, 18F-FDG PET before surgery was shown to predict the MPR after neoadjuvant sintilimab (PD-1 blockade) in 36 patients with resectable NSCLC (Table 3) (8). The authors found that pathological regression strongly correlated with the percentage changes of metabolic parameters after neoadjuvant therapy, and all patients (100%, 13/13) with partial metabolic response (PMR; 36.1%, 13/36), as determined by the PET response criteria in solid tumors (PERCIST), displayed MPR (36.1%, 13/36), including pCR of 38.5% (8). However, no patient achieved CMR; thus, the relationship between the prevalence of pCR and CMR was not established (7). Another retrospective study compared the pathological response between 18F-FDG PET and CT imaging before NACI and surgery in 67 patients with stage II/III NSCLC (Table 3) (9). 18F-FDG PET assessment exhibited high consistency with pathological response, but CT assessment showed low consistency (9). The percentage changes in 18F-FDG uptake before and after NACI differed significantly between pCR (n=28) and non-pCR (n=39) groups (9). Twenty-eight patients achieved pCR, 15 showed MPR, and 24 patients were non-MPR; the postoperative MPR and pCR rates were 64.2% (43/67) and 41.8% (28/67), respectively. Among the 28 patients who achieved pCR, 20 patients (71.4%) had CMR and 8 (28.6%) achieved PMR determined by 18F-FDG PET, whereas 4 patients (14.3%) had CR, 18 (64.3%) had partial response (PR), and 6 patients (21.4%) achieved stable disease (SD) by CT scan; non-MPR was not observed in the patients with CMR or CR. Accordingly, to evaluate the pathological response after NACI and before surgery, PERCIST was found to be more sensitive and accurate than RECIST for identifying more responders (9). Based on these findings, CMR determined by 18F-FDG PET could predict the pCR after NACI in ~70% of patients. Nevertheless, given that predicting pCR using18F-FDG PET can be challenging in approximately 30% of the patients who received chemoimmunotherapy, further investigations are warranted to determine the potential of different PET tracers in addition to 18F-FDG as predictors of pCR. Zhuang et al. described the utility of 18F-FDG PET uptake before surgery values for predicting pathological response to NACI in resectable NSCLC (Table 4) (10). The authors found that the percentage changes in maximum standardized uptake value (SUVmax) on 18F-FDG uptake before and after NACI and post-therapy SUVmax could accurately predict the MPR, whereas baseline SUVmax was not associated with MPR (10). Although there was no information regarding pCR, SUVmax on 18F-FDG uptake was highlighted as an accurate predictor for the MPR after NACI. These results were substantiated in a subsequent study (10), describing that post-therapy SUVmax and the percentage changes of SUVmax [ΔSUVmax% = (baseline SUVmax − post SUVmax)/baseline SUVmax × 100%] were significantly lower in patients with MPR than in those with non-MPR (Table 4) (12). These studies highlighted the clinical usefulness of post-therapy SUVmax and the percentage changes in SUVmax for predicting MPR after NACI (10,12). Chen et al. reported the usefulness of 18F-FDG PET before surgery for predicting the MPR after NACI in NSCLC (Table 4) (11). The SUVmax value on 18F-FDG uptake after NACI was significantly higher in tumors with MPR than in those without MPR, and an SUVmax reduction exceeding 60% was more likely to result in MPR by the probability of sensitivity of 89.3% and specificity of 62.5% (11).
Table 3
Ref. | Number | Regimens | Clinical stage | MPR (%) | pCR (%) | PET response based on PERCIST (%) |
PPV by PMR (%) | PPV by CMR (%) | |||||
---|---|---|---|---|---|---|---|---|---|---|---|---|---|
CMR | PMR | MPR | pCR | MPR | pCR | ||||||||
(8) | 36 | ICI | IA–IIIB | 36.1 | 13.9 | 0 | 36.1 | 100 | 38.5 | NA | NA | ||
(9) | 67 | ICI + chemotherapy | II–III | 64.2 | 41.8 | 29.9 | 11.9 | 39.5 | 28.6 | 21.1 | 71.4 |
18F-FDG, 18F-fluorodeoxyglucose; PET, positron emission tomography; Ref. reference; MPR, major pathological response; pCR, pathological complete response; ICI, immune checkpoint inhibitor; PERCIST, PET response evaluation criteria in solid tumors; PPV, positive predictive value; PMR, partial metabolic response; CMR, complete metabolic response; NA, not available.
Table 4
Ref. | Number | Regimens | Clinical stage | MPR (%) | pCR (%) | Significant cut-off value for ΔSUVmax% | Correlation with MPR | |||
---|---|---|---|---|---|---|---|---|---|---|
MPR | pCR | ∆SUVmax% | Post-therapy SUVmax | |||||||
(10) | 49 Cohort A | ICI + chemotherapy | IIA–IIIB | 59.2 | 30.6 | 54.4 | 36.8 | <0.001 | 0.004 | |
49 Cohort C | ICI + chemotherapy | 55.1 | 40.8 | NA | NA | NA | ||||
(11) | 44 | ICI + chemotherapy | II–III | 63.6 | NA | 60 | NA | 0.01 | NA | |
(12) | 36 | ICI | I–IIIB | 36.1 | NA | 35.1 | NA | <0.001 | <0.001 | |
68 | ICI + chemotherapy | 60.3 | NA | NA | NA | <0.001 | <0.001 |
ΔSUVmax% = (baseline SUVmax − post SUVmax)/baseline SUVmax × 100%. 18F-FDG, 18F-fluorodeoxyglucose; PET, positron emission tomography; SUVmax, maximum standardized uptake value; Ref. reference; MPR, major pathological response; pCR, pathological complete response; ICI, immune checkpoint inhibitor; NA, not available.
The potential of percentage changes in SUVmax before and after NACI or immunotherapy have been underscored for the prediction of MPR post-surgery. The criteria of ΔSUVmax and PERCIST were used to measure the percentage change following 18F-FDG uptake. However, the 18F-FDG uptake value could not comprehensively predict the pCR after NACI. It should be noted that previous studies did not focus on the relationship between pCR and 18F-FDG uptake. Although SUVmax was evaluated as a measurement of 18F-FDG on PET, the assessment of metabolic tumor activity, including metabolic tumor volume (MTV) or total lesion glycolysis (TLG), has not been explored, with the exception of one study (10). Previous studies successfully demonstrated that MTV or TLG, but not SUVmax, could predict the outcome in the early phase after ICI monotherapy in patients with advanced NSCLC (14,21). Moreover, metabolic tumor activity, as determined by MTV or TLG at baseline, was found to be a better predictor of prognosis after ICI monotherapy than SUVmax (22-24). Future investigations should focus on assessing MTV or TLG to predict pCR and MPR after ICI or ICI plus chemotherapy.
Tumor immune microenvironment and blood biomarkers
Tumor-infiltrating lymphocytes (TILs) in the tumor and stroma are expected to accurately predict the outcome of ICI therapy. Several researchers have reported that CD8+ TILs in the tumor or stroma were the strongest predictors of progression-free survival (PFS) and overall survival (OS) following PD-1 blockade therapy in the patients with advanced NSCLC (25-27). Duhen et al. reported that CD103+ CD39+ CD8+ T cells killed cancer cells in a major histocompatibility complex (MHC) class I-restricted manner, and the greater the presence of this population in TILs, the better the prognosis (28). Banchereau et al. revealed that intratumoral CD103+ CD8+ T cells could predict therapeutic efficacy in patients with lung and bladder cancer undergoing anti-PD-L1 antibody therapy in advanced cases (29). Considering anti-PD-1 antibody-treated patients with advanced lung cancer, gastric cancer, and melanoma, PD-1+ CD8+ T cells and PD-1+ Tregs in TILs were associated with a favorable and poor prognosis, respectively. Reportedly, the balance in the PD-1 expression ratio of PD-1+ CD8+ T cells and PD-1+ Tregs can be a useful biomarker for anti-PD-1 antibody therapy (30). We recently described that stromal CD4+ TILs and intratumoral CD8+ TILs were crucial markers for predicting the outcome after pembrolizumab in patients with advanced NSCLC (31). According to Casarrubios et al., the T cell receptor (TCR) repertoire evenness and higher frequency of the top 1% most common TCRs in the pretreatment tumor tissue were more accurate predictors of CPR in patients with NSCLC receiving NACI than TMB and PD-L1 (32). Accordingly, CPR could be achieved after NACI if a high-frequency population of the dominant TCR repertoire is present in the pretreatment tumor tissue. In addition, immunotherapy is thought to induce tertiary lymphoid structure (TLS) formation, and TLS maturation may serve as a biomarker for favorable disease-free survival and MPR in patients with NSCLC receiving NACI (33). However, there is limited evidence regarding the predictive significance of TILs in tumor specimens before and after NACI in patients with resectable NSCLC (Table 5). Overall, the presence of many TILs is thought to be a factor that enhances the effect of NACI, but there are also populations of TILs that attenuate immune responses, such as PD-1+ Tregs, and further analysis results are awaited.
Table 5
Ref. | Number | Regimens | Clinical stage | MPR (%) | pCR (%) | Sampling | Significant marker for MPR | Significant marker for pCR | |||
---|---|---|---|---|---|---|---|---|---|---|---|
Increase | Decrease | Increase | Decrease | ||||||||
(32) | 40 | ICI + chemotherapy | IIIA | 75 | 56.8 | Tissue, blood | None | None | Tissue TCR repertoire evenness | None | |
(33) | 40 | ICI + chemotherapy | IA–IIIB | NA | NA | Tissue | TLS maturation | None | None | None | |
(34) | 32 | ICI + chemotherapy | IIA–IIIC | 43.7 | NA | Tissue | CD8+ T cells | CD3+ T cells, Foxp3 T cells | NA | NA | |
(35) | 29 | ICI + chemotherapy | III–IV | 55 | 41 | Tissue | NA | NA | CD3+PD-L1+ TILs | CD4+TILs CD4+Foxp3+ TILs | |
(36) | 27 | ICI + chemotherapy | IIIA | NA | 55.6 | Blood | NA | NA | CD4+PD-1+ cells | CD3+CD56+CTLA-4+ cells | |
(37) | 59 | ICI + chemotherapy | IIA–IIIB | NA | 28.8 | Blood | NA | NA | NK cells | Total T cells, Th cells | |
(38) | 23 | ICI + chemotherapy | IA–IIIB | 52 | 39 | Blood | None | None | None | MDSCs, Tregs |
Ref. reference; MPR, major pathological response; pCR, pathological complete response; sampling, sampling lesion to assess biomarkers; ICI, immune checkpoint inhibitor; none, no statistically significant biomarkers; TCR, T cell receptor; NA, not available; TLS, tertial lymphoid structure; PD-L1, programmed death ligand-1; TILs, tumor infiltrative lymphocytes; PD-1, programmed death-1; NK, natural killer; MDSCs, myeloid-derived suppressors cells.
Chen et al. described that the infiltration of immune cells such as CD3, Foxp3+, and CD8+/PD-1+ cells before NACI correlated with the pathological response (pCR or MPR) in 32 patients with stage IIA–IIIC NSCLC and TILs increased after NACI (34). The authors emphasized that responders (MPR or pCR) had substantially higher levels of CD8+/PD-1+ T cells in the tumor tissue prior to NACI than non-responders (non-MPR), thereby suggesting potential clinical benefits (34). Han et al. reported the clinical significance of TME as a predictor of pathological response after NACI in 29 patients with stage III and IV locally advanced or oligometastatic NSCLC (35). Based on the observed findings, the authors suggested that NACI led to increased immune infiltration and baseline TILs correlated with the pathological response. Patients who achieved pCR were likely to demonstrate higher infiltration of CD3+PD-L1+ TILs and lower infiltration of CD4+ or CD4+Foxp3+ TILs in the stroma of the baseline specimen. Conversely, higher tumor CD8+ TIL infiltration was predominant in patients with non-MPR (35).
As a non-invasive modality to explore the TME, peripheral blood mononuclear cells (PBMCs) can be valuable and have been widely investigated as predictive markers of immunotherapy. Although the trend of CD4+ T cells in peripheral blood could predict the outcome of ICI monotherapy in patients with advanced NSCLC (39), there is no established biomarker for predicting immunotherapy based on PBMCs. A recent study investigated the relationship between blood biomarkers and pCR in 27 patients with stage IIIA NSCLC who received NACI (36). Of the 27 patients, 15 who achieved pCR revealed a trend toward higher baseline levels of PD-1+ cells, which was statistically significant only for CD4+ but not for CD8+ T cells. Moreover, the peripheral blood immune status at diagnosis seemed to be associated with the prediction of pCR (36). Ma et al. described that the peripheral blood immune cell subsets were closely associated with pathological response in patients with resectable stage IIA–IIIB NSCLC (n=59) after NACI (37). Total T cells and activated T cells were markedly increased in patients who achieved pCR, and no correlation was detected between pathological response and PD-L1 expression (37). On the other hand, Wen et al. examined the predictive significance of circulating myeloid-derived suppressor cells (MDSCs) in 23 patients with NSCLC receiving NACI (38). Although the level of MDSCs positively correlated with Treg levels and negatively correlated with CD4+ T cells in peripheral blood, baseline levels of MDSCs and Tregs in patients who achieved pCR were substantially lower than in those who did not achieve pCR (38). Overall, NACI could induce notable changes in peripheral blood immune levels in patients with NSCLC (37).
Foxp3+ T cells were found to be reduced in NACI responders, while CD8+/PD-1+ TILs were enhanced in non-responders, thereby suggesting that NACI suppressed the infiltration of regulatory T cells in responders and promoted CD8+ T cells in non-responder (34). The distinct function of TILs could be attributed to differences in TIL infiltration between responders and non-responders after NACI. One explanation could be the presence of dysfunctional CD8+ TILs in the tumor and stroma of non-responders after NACI (40). Dysfunctional or exhausted T cells are characterized by defective effector function (41), and a subset of effector burned-out CD8+ T cells in NSCLC tumors was found to correlate with a worse response to PD-1 blockade (40). If dysfunctional TILs containing CD8+/PD-1+ T cells infiltrated into tumors without a response to PD-1 blockade, measuring TILs to predict the pCR after NACI could present a considerable challenge. Accordingly, differences between functional and dysfunctional T-cells need to be detected by employing more useful methodologies.
Genetic alternations
According to the NADIM phase II study, preoperative ctDNA levels were substantially associated with OS after NACI (6). TMB and PD-L1 expression was not predictive of OS, whereas low pretreatment levels of ctDNA and undetectable ctDNA levels after NACI were substantially associated with PFS and OS (6). Although baseline ctDNA was closely associated with tumor size, baseline ctDNA could not be detected in approximately 30% of the pretreatment plasma samples. Moreover, the relationship between the levels of ctDNA and pCR remains unclear.
Zeng et al. presented the relationship between copy number variations and MPR after NACI in 94 patients with unresectable stage IIIA–IIIB lung cancer (42). The authors reported MPR and pCR values of 65.6% and 42.2%, respectively (42), and two clusters of hot and cold tumors were defined according to the enrichment score of 28 different immune cell infiltrations (42). There was no difference in the proportion of hot tumors at baseline between MPR and non-MPR; however, the infiltration of immature dendritic cells was substantially higher in MPR than in non-MPR. The proportion of hot tumors was considerably increased in surgically resected samples than in baseline specimens, indicating that all cold tumors converted to hot tumors after NACI. These findings suggested a significant association between copy number variations status and MPR. Likewise, Casarrubios et al. analyzed a panel targeting 395 genes related to immunological processes using baseline and post-treatment tissue specimens from 41 patients with resectable stage IIIA NSCLC treated with NACI from the NADIM study (43). The authors demonstrated that tumors with pCR had a stronger pre-established immune infiltrate (high level of IFNG, GZMB, NKG7, and M1 macrophages) at baseline than non-pCR tumors. In addition, the higher expression of several genes (AKT1, BST2, OAS3, CD8B) in post-treatment non-pCR samples was related to relapse after surgery (43). Moreover, the authors identified the differences in achieving possible response and relapse mechanisms in relapse between pCR and non-pCR after neoadjuvant therapy of NSCLC (43). Gene expression associated with tumor immune infiltration was analyzed using small sample sizes in previous studies. Therefore, only exploratory evidence can be established to distinguish pCR and non-pCR. Further investigations are warranted to investigate the relationship between the expression of immune-related genes and the pathological response after NACI using a large sample size.
Clinical biomarkers according to patient’s demographics
Modalities such as gene analysis, immunohistochemistry, and radiological devices can aid in predicting the therapeutic efficacy after any treatment. However, it is crucial to obtain sufficient tumor tissue during biopsy to analyze biological alternations, and economic issues associated with the measurement of certain biomarkers need to be considered. If any affordable biomarkers, such as patient characteristics or routine blood samples, can effectively predict therapeutic efficacy, physicians can easily confirm appropriate predictors according to different treatments. Recently, the potential of clinical markers capable of predicting the pathological response after NACI in resectable NSCLC has been reported (44-48). The prognostic significance of inflammatory biomarkers in the peripheral blood has been examined in patients with advanced NSCLC who received immunotherapy (49-51). Inflammatory indices, such as the neutrophil-to-lymphocyte ratio (NLR), platelet-to-lymphocyte ratio (PLR), and systemic immune-inflammation index (SII), have been explored as potential prognostic and predictive biomarkers in patients with advanced NSCLC who were treated with ICIs (49). Li et al. reported that NLR, PLR, and SII values approximately 3 weeks after initiating NACI were markedly lower in NSCLC patients with MPR than in those with non-MPR (discovery cohort, 122 patients; validation cohort 92 patients), with SII identified as an independent predictor of MPR by multivariate analysis (44). Although the relationship between pCR and these inflammatory markers was not investigated, resected tumor samples from 37 patients collected for RNA-sequencing revealed that the patients with low SII exhibited substantially higher intratumoral infiltration of activated CD8+ T cells, T helper T cells, macrophages, and activated dendritic cells than those with high SII (44). Liu et al. also examined the predictive role of pCR based on baseline and preoperative NLR values after NACI in 116 patients with resectable NSCLC (45). Multivariate analyses revealed that patients with high baseline NLR had a lower incidence of pCR, with a shorter disease-free survival identified in those with high preoperative NLR (45).
Two studies reported the pathological response according to the number of NACI cycles in patients with NSCLC (46,47). In both studies, the patients were divided into the 2-cycle group and the more than 2-cycle (>2-cycle group); however, MPR did not differ significantly between the two groups (45,47). Accordingly, the number of neoadjuvant cycles administered does not substantially impact the pathological response of chemoimmunotherapy.
According to Yang et al., smoking signature had better performance than PD-L1 expression in terms of predicting the pathological response after NACI in 39 patients with NSCLC (48). The authors found that heavy smokers with >40 pack-years were favorable for predicting pathological response, and the pathological response included pCR and MPR (48). Thus, the association between pCR and smoking signature remains elusive.
Overall, it remains unclear whether the smoking signature or the cycle number could predict the pCR after NACI. NLR may be useful in predicting the pathological response; however, further investigations are warranted to identify whether baseline or post-treatment inflammatory markers would serve as optimal predictors for the pCR.
Discussion
The current review focuses on predictive markers for pathological response to NACI in patients with resectable NSCLC. In particular, it is crucial to identify and establish novel predictors for pCR, not MPR, given the close relationship between achieving pCR and favorable survival outcomes, as demonstrated in the CheckMate 816 study (5).
Reportedly, the number of NACI cycles is not associated with its pathological response. Although a smoking history of >40 pack-years may be related to the increased pathological response, the precise level of smoking that could predict the pCR after NACI remains unknown. However, clinical markers such as NLR or SII can be easily assessed using routinely collected blood samples for systemic chemotherapy and may be helpful in predicting ICI therapy. A recent meta-analysis has highlighted the considerable evidence supporting the application of NLR as a prognostic marker for advanced NSCLC treated with immunotherapy (49). In addition to the prognostic significance of NLR, a recent study demonstrated that a lower baseline NLR value was closely associated with an increased response rate after ICI therapy in advanced NSCLC (50). To utilize NLR as an optimal predictor, the ideal measurement time better before and after neoadjuvant therapy and a cut-off value are crucial issues that need to be addressed. Recruiting larger cohorts could resolve these critical challenges.
Radiological assessment or the detection of promising biomarkers related to the TME could be critical for the early prediction after NACI in patients with resectable NSCLC. The pathological response by 18F-FDG PET was assessed by PET response based on PERCIST and ΔSUVmax. The CMR by PET response achieved a positive predictive value (PPV) of 71.4% for the detection of pCR after NACI (9) (Table 3), whereas the change in SUVmax, as determined by ΔSUVmax before and after NACI elicited a PPV of 36.8% for pCR (10) (Table 4). Overall, the change in ΔSUVmax closely is correlated with MPR (Table 4). Accordingly, CMR determined by PERCIST can be a promising predictor of pCR.
Pathological response determined by 18F-FDG PET has been examined in patients with non-thoracic neoplasms who received neoadjuvant ICI therapy (51-53). The high tumor SUVmax and low total MTV on 18F-FDG PET were identified as independent factors for pCR in 100 patients with early-stage triple-negative breast cancer after NACI (51). In 58 patients with esophageal squamous cell carcinoma receiving NACI, 18F-FDG PET was acquired at baseline and after NACI, and SUVmax, MTV, and TLG after NACI were substantially lower in pCR patients than in non-pCR patients (52). Furthermore, primary tumor response assessment using 18F-FDG PET-based MTV and TLG could accurately identify MPR after neoadjuvant ICI monotherapy in 32 patients with head and neck squamous cell carcinoma (53). Based on these reports, 18F-FDG PET parameters exhibit good performance for predicting pCR after NACI in patients with resectable cancer.
Notably, studies that explored the relationship between pathological response and TME after NACI involved markedly small sample sizes (Table 5), complicating the establishment of accurate conclusions. Significant biomarkers for pCR differed according to individual studies. Moreover, materials that could improve the detection of TME, as well as the assessment of blood or tissue samples, are yet to be established. Further investigations are warranted to elucidate optimal predictors using a large sample size.
Data derived from morphological imaging or radiomics by deep learning remain underdeveloped for predicting pCR, with only limited investigations reported to date. Additional investigations are crucial to elucidate the relationship between radiomics and the prediction of pCR based on established programming systems.
Predictive perspective for NACI
Based on previous studies, changes in MTV determined by PET or lymphocyte surface markers related to TME could be developed as optimal predictors of pCR after NACI. Although accurately predicting pCR using 18F-FDG uptake at baseline NACI remains a challenge, the reduced rate of 18F-FDG uptake immediately after NACI indicates the possibility of pCR with a probability of approximately 70%. However, critical issues such as false-positive 18F-FDG accumulation need to be resolved (54). To overcome these challenges, new PET tracers, such as proliferation with 18F-fluoro-thymidine (FLT), hypoxia with 18F-fluoromisonidazole (FMISO), and amino acid metabolism with L-[3-18F]-α-methyltyrosine (18F-FAMT), are expected to replace the presence of 18F-FDG uptake, given their more specific detection in tumor cells (55). Future studies should focus on the possibility of optimal predictors of pCR after NACI using these new PET tracers.
Biomaterials at baseline NACI may be better candidates for predicting pCR than those after NACI. Instead of PET imaging, blood samples or tumor specimens could facilitate the discovery of predictive biomarkers related to TME. Considering blood samples at baseline NACI, CD4-related markers need to be further explored, whereas CD8 or Foxp3-related markers should be examined as promising predictors of pCR if tumor tissue samples are available. From the perspective of both blood and tissue samples, further investigations are necessary to establish biomarkers of NACI.
Conclusions
The current review focuses on the pathological response in terms of pCR after NACI. Overall, several studies using limited sample sizes have reported potential predictors of pathological response after NACI in patients with resectable NSCLC. Based on accumulated evidence, a reduced rate of 18F-FDG uptake after NACI and surface markers on TME-related lymphocytes may be promising as optimal predictors of pCR (Figure 1). However, the evidence regarding potential pCR predictors after NACI needs to be further substantiated. Given the paucity of reports on radiomics or clinical markers such as NLR or smoking signature, the potential of these pCR predictors remains uncertain, owing to differences in study design. Nevertheless, achieving long-term survival after ICI therapy, not increasing the response rate to ICIs, remains the primary endpoint. Given that previous reports on NACI in patients with resectable NSCLC are limited by a follow-up period, it is difficult to discuss the relationship between potential predictors and outcomes after NACI. Future reviews should focus on the outcome after NACI in resectable NSCLC.
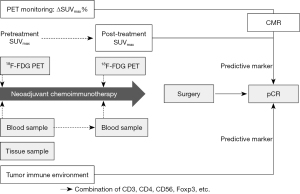
Acknowledgments
The authors thank Ms. Kozue Watanabe, Saki Toita, and Koko Kodaira for their assistance in preparing this manuscript. The authors also thank Editage (www.editage.jp) for English language editing.
Funding: None.
Footnote
Reporting Checklist: The authors have completed the Narrative Review reporting checklist. Available at https://tlcr.amegroups.com/article/view/10.21037/tlcr-24-142/rc
Peer Review File: Available at https://tlcr.amegroups.com/article/view/10.21037/tlcr-24-142/prf
Conflicts of Interest: All authors have completed the ICMJE uniform disclosure form (available at https://tlcr.amegroups.com/article/view/10.21037/tlcr-24-142/coif). The authors have no conflicts of interest to declare.
Ethical Statement: The authors are accountable for all aspects of the work in ensuring that questions related to the accuracy or integrity of any part of the work are appropriately investigated and resolved.
Open Access Statement: This is an Open Access article distributed in accordance with the Creative Commons Attribution-NonCommercial-NoDerivs 4.0 International License (CC BY-NC-ND 4.0), which permits the non-commercial replication and distribution of the article with the strict proviso that no changes or edits are made and the original work is properly cited (including links to both the formal publication through the relevant DOI and the license). See: https://creativecommons.org/licenses/by-nc-nd/4.0/.
References
- Garon EB, Hellmann MD, Rizvi NA, et al. Five-Year Overall Survival for Patients With Advanced Non-Small-Cell Lung Cancer Treated With Pembrolizumab: Results From the Phase I KEYNOTE-001 Study. J Clin Oncol 2019;37:2518-27. [Crossref] [PubMed]
- Gandhi L, Rodríguez-Abreu D, Gadgeel S, et al. Pembrolizumab plus Chemotherapy in Metastatic Non-Small-Cell Lung Cancer. N Engl J Med 2018;378:2078-92. [Crossref] [PubMed]
- Antonia SJ, Villegas A, Daniel D, et al. Durvalumab after Chemoradiotherapy in Stage III Non-Small-Cell Lung Cancer. N Engl J Med 2017;377:1919-29. [Crossref] [PubMed]
- Felip E, Altorki N, Zhou C, et al. Adjuvant atezolizumab after adjuvant chemotherapy in resected stage IB-IIIA non-small-cell lung cancer (IMpower010): a randomised, multicentre, open-label, phase 3 trial. Lancet 2021;398:1344-57. [Crossref] [PubMed]
- Forde PM, Spicer J, Lu S, et al. Neoadjuvant Nivolumab plus Chemotherapy in Resectable Lung Cancer. N Engl J Med 2022;386:1973-85. [Crossref] [PubMed]
- Provencio M, Serna-Blasco R, Nadal E, et al. Overall Survival and Biomarker Analysis of Neoadjuvant Nivolumab Plus Chemotherapy in Operable Stage IIIA Non-Small-Cell Lung Cancer (NADIM phase II trial). J Clin Oncol 2022;40:2924-33. [Crossref] [PubMed]
- Fayanju OM, Ren Y, Thomas SM, et al. The Clinical Significance of Breast-only and Node-only Pathologic Complete Response (pCR) After Neoadjuvant Chemotherapy (NACT): A Review of 20,000 Breast Cancer Patients in the National Cancer Data Base (NCDB). Ann Surg 2018;268:591-601. [Crossref] [PubMed]
- Tao X, Li N, Wu N, et al. The efficiency of (18)F-FDG PET-CT for predicting the major pathologic response to the neoadjuvant PD-1 blockade in resectable non-small cell lung cancer. Eur J Nucl Med Mol Imaging 2020;47:1209-19. [Crossref] [PubMed]
- Cheng Y, Chen ZY, Huang JJ, et al. Efficacy evaluation of neoadjuvant immunotherapy plus chemotherapy for non-small-cell lung cancer: comparison of PET/CT with postoperative pathology. Eur Radiol 2023;33:6625-35. [Crossref] [PubMed]
- Zhuang F, Haoran E, Huang J, et al. Utility of (18)F-FDG PET/CT uptake values in predicting response to neoadjuvant chemoimmunotherapy in resectable non-small cell lung cancer. Lung Cancer 2023;178:20-7. [Crossref] [PubMed]
- Chen ZY, Fu R, Tan XY, et al. Dynamic (18) F-FDG PET/CT can predict the major pathological response to neoadjuvant immunotherapy in non-small cell lung cancer. Thorac Cancer 2022;13:2524-31. [Crossref] [PubMed]
- Chen X, Bai G, Zang R, et al. Utility of (18)F-FDG uptake in predicting major pathological response to neoadjuvant immunotherapy in patients with resectable non small cell lung cancer. Transl Oncol 2023;35:101725. [Crossref] [PubMed]
- Yamaguchi O, Kasahara N, Soda H, et al. Predictive significance of circulating tumor DNA against patients with T790M-positive EGFR-mutant NSCLC receiving osimertinib. Sci Rep 2023;13:20848. [Crossref] [PubMed]
- Yamaguchi O, Kaira K, Naruse I, et al. Prospective assessment using (18)F-FDG PET/CT as a novel predictor for early response to PD-1 blockade in non-small-cell lung cancer. Sci Rep 2022;12:11832. [Crossref] [PubMed]
- Liu W, Zhang T, Zhang Q, et al. A systematic review and meta-analysis of neoadjuvant chemoimmunotherapy in stage III non-small cell lung cancer. BMC Pulm Med 2022;22:490. [Crossref] [PubMed]
- Wu Y, Verma V, Gay CM, et al. Neoadjuvant immunotherapy for advanced, resectable non-small cell lung cancer: A systematic review and meta-analysis. Cancer 2023;129:1969-85. [Crossref] [PubMed]
- Franzi S, Mattioni G, Rijavec E, et al. Neoadjuvant Chemo-Immunotherapy for Locally Advanced Non-Small-Cell Lung Cancer: A Review of the Literature. J Clin Med 2022;11:2629. [Crossref] [PubMed]
- Jiang J, Wang Y, Gao Y, et al. Neoadjuvant immunotherapy or chemoimmunotherapy in non-small cell lung cancer: a systematic review and meta-analysis. Transl Lung Cancer Res 2022;11:277-94. [Crossref] [PubMed]
- Chen Y, Qin J, Wu Y, et al. Does major pathological response after neoadjuvant Immunotherapy in resectable nonsmall-cell lung cancers predict prognosis? A systematic review and meta-analysis. Int J Surg 2023;109:2794-807. [Crossref] [PubMed]
- Chen LN, Wei AZ, Shu CA. Neoadjuvant immunotherapy in resectable non-small-cell lung cancer. Ther Adv Med Oncol 2023;15:17588359231163798. [Crossref] [PubMed]
- Kaira K, Higuchi T, Naruse I, et al. Metabolic activity by 18F-FDG-PET/CT is predictive of early response after nivolumab in previously treated NSCLC. Eur J Nucl Med Mol Imaging 2018;45:56-66. [Crossref] [PubMed]
- Hashimoto K, Kaira K, Yamaguchi O, et al. Potential of FDG-PET as Prognostic Significance after anti-PD-1 Antibody against Patients with Previously Treated Non-Small Cell Lung Cancer. J Clin Med 2020;9:725. [Crossref] [PubMed]
- Yamaguchi O, Kaira K, Hashimoto K, et al. Tumor metabolic volume by (18)F-FDG-PET as a prognostic predictor of first-line pembrolizumab for NSCLC patients with PD-L1 ≥ 50. Sci Rep 2020;10:14990. [Crossref] [PubMed]
- Hashimoto K, Kaira K, Imai H, et al. Prognostic Potential of Metabolic Activity on 18 F-FDG Accumulation in Advanced NSCLC Receiving Combining Chemotherapy Plus PD-1 Blockade. J Immunother 2022;45:349-57. [Crossref] [PubMed]
- Hashemi S, Fransen MF, Niemeijer A, et al. Surprising impact of stromal TIL's on immunotherapy efficacy in a real-world lung cancer study. Lung Cancer 2021;153:81-9. [Crossref] [PubMed]
- Hu-Lieskovan S, Lisberg A, Zaretsky JM, et al. Tumor Characteristics Associated with Benefit from Pembrolizumab in Advanced Non-Small Cell Lung Cancer. Clin Cancer Res 2019;25:5061-8. [Crossref] [PubMed]
- Uryvaev A, Passhak M, Hershkovits D, et al. The role of tumor-infiltrating lymphocytes (TILs) as a predictive biomarker of response to anti-PD1 therapy in patients with metastatic non-small cell lung cancer or metastatic melanoma. Med Oncol 2018;35:25. [Crossref] [PubMed]
- Duhen T, Duhen R, Montler R, et al. Co-expression of CD39 and CD103 identifies tumor-reactive CD8 T cells in human solid tumors. Nat Commun 2018;9:2724. [Crossref] [PubMed]
- Banchereau R, Chitre AS, Scherl A, et al. Intratumoral CD103+ CD8+ T cells predict response to PD-L1 blockade. J Immunother Cancer 2021;9:e002231. [Crossref] [PubMed]
- Kumagai S, Togashi Y, Kamada T, et al. The PD-1 expression balance between effector and regulatory T cells predicts the clinical efficacy of PD-1 blockade therapies. Nat Immunol 2020;21:1346-58. [Crossref] [PubMed]
- Kaira K, Yamaguchi O, Kawasaki T, et al. Prognostic significance of tumor infiltrating lymphocytes on first-line pembrolizumab efficacy in advanced non-small cell lung cancer. Discov Oncol 2023;14:6. [Crossref] [PubMed]
- Casarrubios M, Cruz-Bermúdez A, Nadal E, et al. Pretreatment Tissue TCR Repertoire Evenness Is Associated with Complete Pathologic Response in Patients with NSCLC Receiving Neoadjuvant Chemoimmunotherapy. Clin Cancer Res 2021;27:5878-90. [Crossref] [PubMed]
- Sun X, Liu W, Sun L, et al. Maturation and abundance of tertiary lymphoid structures are associated with the efficacy of neoadjuvant chemoimmunotherapy in resectable non-small cell lung cancer. J Immunother Cancer 2022;10:e005531. [Crossref] [PubMed]
- Chen T, Cao Z, Sun Y, et al. Neoadjuvant Chemoimmunotherapy Increases Tumor Immune Lymphocytes Infiltration in Resectable Non-small Cell Lung Cancer. Ann Surg Oncol 2023;30:7549-60. [Crossref] [PubMed]
- Han R, Zhang Y, Wang T, et al. Tumor immune microenvironment predicts the pathologic response of neoadjuvant chemoimmunotherapy in non-small-cell lung cancer. Cancer Sci 2023;114:2569-83. [Crossref] [PubMed]
- Laza-Briviesca R, Cruz-Bermúdez A, Nadal E, et al. Blood biomarkers associated to complete pathological response on NSCLC patients treated with neoadjuvant chemoimmunotherapy included in NADIM clinical trial. Clin Transl Med 2021;11:e491. [Crossref] [PubMed]
- Ma T, Wen T, Cheng X, et al. Pathological complete response to neoadjuvant chemoimmunotherapy correlates with peripheral blood immune cell subsets and metastatic status of mediastinal lymph nodes (N2 lymph nodes) in non-small cell lung cancer. Lung Cancer 2022;172:43-52. [Crossref] [PubMed]
- Wen T, Su C, Cheng X, et al. Circulating myeloid-derived suppressors cells correlate with clinicopathological characteristics and outcomes undergoing neoadjuvant chemoimmunotherapy in non-small cell lung cancer. Clin Transl Oncol 2022;24:1184-94. [Crossref] [PubMed]
- Kagamu H, Yamasaki S, Kitano S, et al. Single-Cell Analysis Reveals a CD4+ T-cell Cluster That Correlates with PD-1 Blockade Efficacy. Cancer Res 2022;82:4641-53. [Crossref] [PubMed]
- Sanmamed MF, Nie X, Desai SS, et al. A Burned-Out CD8(+) T-cell Subset Expands in the Tumor Microenvironment and Curbs Cancer Immunotherapy. Cancer Discov 2021;11:1700-15. [Crossref] [PubMed]
- Blank CU, Haining WN, Held W, et al. Defining 'T cell exhaustion'. Nat Rev Immunol 2019;19:665-74. [Crossref] [PubMed]
- Zeng L, Zhou Y, Zhang X, et al. Copy number variations mediate major pathological response to induction chemo-immunotherapy in unresectable stage IIIA-IIIB lung cancer. Lung Cancer 2023;178:134-42. [Crossref] [PubMed]
- Casarrubios M, Provencio M, Nadal E, et al. Tumor microenvironment gene expression profiles associated to complete pathological response and disease progression in resectable NSCLC patients treated with neoadjuvant chemoimmunotherapy. J Immunother Cancer 2022;10:e005320. [Crossref] [PubMed]
- Li C, Wu J, Jiang L, et al. The predictive value of inflammatory biomarkers for major pathological response in non-small cell lung cancer patients receiving neoadjuvant chemoimmunotherapy and its association with the immune-related tumor microenvironment: a multi-center study. Cancer Immunol Immunother 2023;72:783-94. [Crossref] [PubMed]
- Liu W, Ren S, Yang L, et al. The predictive role of hematologic markers in resectable NSCLC patients treated with neoadjuvant chemoimmunotherapy: a retrospective cohort study. Int J Surg 2023;109:3519-26. [Crossref] [PubMed]
- Zhu D, Huang Z, Xiao K, et al. Does the number of cycles of neoadjuvant therapy affect the efficacy of neoadjuvant chemoimmunotherapy for non-small cell lung cancer in locally advanced stage? Retrospective experience based on a single center. Asia Pac J Clin Oncol 2023; Epub ahead of print. [Crossref] [PubMed]
- Zhang B, Guo X, Jia R, et al. Neoadjuvant chemoimmunotherapy cycle number selection for non-small cell lung cancer and clinical outcomes: a real-world analysis. Front Oncol 2023;13:1200625. [Crossref] [PubMed]
- Yang H, Ma W, Sun B, et al. Smoking signature is superior to programmed death-ligand 1 expression in predicting pathological response to neoadjuvant immunotherapy in lung cancer patients. Transl Lung Cancer Res 2021;10:3807-22. [Crossref] [PubMed]
- Platini H, Ferdinand E, Kohar K, et al. Neutrophil-to-Lymphocyte Ratio and Platelet-to-Lymphocyte Ratio as Prognostic Markers for Advanced Non-Small-Cell Lung Cancer Treated with Immunotherapy: A Systematic Review and Meta-Analysis. Medicina (Kaunas) 2022;58:1069. [Crossref] [PubMed]
- Yamaguchi O, Kaira K, Imai H, et al. Clinical Utility of Inflammatory and Nutritious Index as Therapeutic Prediction of Nivolumab plus Ipilimumab in Advanced Non-Small Cell Lung Cancer. Oncology 2024;102:271-82. [Crossref] [PubMed]
- Seban RD, Arnaud E, Loirat D, et al. [18F]FDG PET/CT for predicting triple-negative breast cancer outcomes after neoadjuvant chemotherapy with or without pembrolizumab. Eur J Nucl Med Mol Imaging 2023;50:4024-35. [Crossref] [PubMed]
- Wang X, Yang W, Zhou Q, et al. The role of 18F-FDG PET/CT in predicting the pathological response to neoadjuvant PD-1 blockade in combination with chemotherapy for resectable esophageal squamous cell carcinoma. Eur J Nucl Med Mol Imaging 2022;49:4241-51. [Crossref] [PubMed]
- Vos JL, Zuur CL, Smit LA, et al. [18F]FDG-PET accurately identifies pathological response early upon neoadjuvant immune checkpoint blockade in head and neck squamous cell carcinoma. Eur J Nucl Med Mol Imaging 2022;49:2010-22. [Crossref] [PubMed]
- Kaira K, Kuji I, Kagamu H. Value of 18F-FDG-PET to predict PD-L1 expression and outcomes of PD-1 inhibition therapy in human cancers. Cancer Imaging 2021;21:11. [Crossref] [PubMed]
- Kaira K, Oriuchi N, Sunaga N, et al. A systemic review of PET and biology in lung cancer. Am J Transl Res 2011;3:383-91. [PubMed]