Association between methyltransferase-like 3 and non-small cell lung cancer: pathogenesis, therapeutic resistance, and clinical applications
Introduction
Non-small cell lung cancer (NSCLC) is a common malignancy worldwide (1). Currently, effective early diagnosis of NSCLC remains limited primarily by the lack of accurate biomarkers, and NSCLC is usually diagnosed late in its progression (2,3). Clinical strategies for NSCLC treatment usually have a high risk of recurrence and metastasis, with 5-year survival of less than 30% after treatment (4,5). Given these characteristics, there is an urgent need to understand the mechanisms underlying NSCLC pathogenesis and treatment failure.
Both pathogenesis and therapeutic failure are often accompanied by intricate mechanisms, encompassing genetic factors and environmental influences (5,6). Currently, the rapid advancement of sequencing technologies, including RNA-seq and MeRIP-seq, has improved our understanding of epigenetic modifications at the post-transcriptional level (7-9). In particular, the most quintessential post-transcriptional regulation of gene function, RNA N6-methyladenosine (m6A), in NSCLC has been extensively documented (10). This effect on RNA, specifically RRACH (R for A or G, H for A, U or C) is dynamically governed by three key enzymes that are often called “writers, erasers, and readers” (11,12). Writers primarily include the methyltransferase complex of methyltransferase-like 3/14 (METTL3/14)-WT1-associated protein (WTAP), which adds methyl groups (-CH3) to the sixth nitrogen atom of adenines of newly transcribed RNAs (naïve RNAs). Erasers include demethylases, including fat mass and obesity-associated protein (FTO), and AlkB homolog 5 (ALKBH5) that remove methyl groups (11,13). These modifications by writers and erasers transmute naïve RNAs into pre-RNAs, which subsequently undergo further splicing modifications to produce mature RNAs, which are then transported out of the nucleus. Readers, including YTH N6-methyladenosine RNA binding protein (YTHDF), YTHDF domain-containing protein (YTHDC), heterogeneous nuclear ribonucleoparticles (hnRNPs), and insulin-like growth factor 2 mRNA-binding protein (IGF2BP), discern these mature RNAs, influencing both their stability and translation (11-13) (Figure 1).
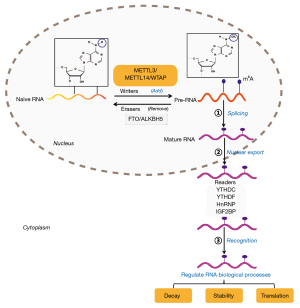
The METTL protein family is composed of METTL3, 4, 5, 14, 16, and 25B, which share a conserved S-adenosyl methionine binding domain and contribute to m6A modification (14). Specifically, METTL3, together with METTL14 and WTAP, forms the primary heterocomplex of m6A writers (14). Other METTL family members form the auxiliary structures involved in catalysis. METTL3 is the primary m6A-producing enzyme and the only subunit with catalytic activity, whereas METTL14 lacks catalytic activity and is commonly considered a structural subunit that binds to target RNA, promoting the action of METTL3 (15). METTL3 is predominantly localized to human chromosome 14q11.2, with pronounced enrichment in nuclear speckles. METTL3 is also present in the cytoplasm, possibly engaging in methyltransferase-independent functions related to translation initiation (16,17). As studies on METTL3-mediated RNA m6A modification have progressed, studies have highlighted the active involvement of METTL3 in both NSCLC pathogenesis and therapeutic resistance, as well as using METTL3 as a potential therapeutic target (17-19). However, a comprehensive analysis of these studies to discuss the relationship between METTL3 and NSCLC, as well as suggestions for the prospective applications of METTL3 in clinical settings, is currently lacking.
To address this gap in literature, we reviewed the current understanding of the roles and mechanisms of METTL3 in NSCLC. Furthermore, we suggest prospective applications and future perspectives of METTL3 (Figure 2).
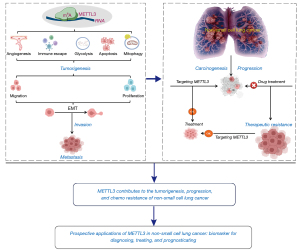
METTL3 contributes to NSCLC tumorigenesis and progression
Recent epigenetic study has revealed a strong correlation between RNA m6A modification by METTL3 and both tumorigenesis and malignant progression of NSCLC (18). This modification plays a significant role in the general regulation of cancer-cell proliferation, migration, and invasion (20). Moreover, METTL3-mediated m6A modification regulates the epithelial-mesenchymal transition (EMT), abnormal angiogenesis, and the tumour microenvironment (TME) by targeting multiple RNAs (18). METTL3 is a potential independent prognostic factor to predict NSCLC survival (21).
METTL3 functions in NSCLC by regulating mRNAs
Mutations in multiple critical genes, including those encoding epidermal growth factor receptor (EGFR), TP53, and KRAS, contribute to the onset and progression of NSCLC (22). Interestingly, METTL3 expression is increased in patients with NSCLC with EGFR exon 19 mutations compared with patients with wild-type EGFR; this promotes EMT, migration, and proliferation by regulating the translation of EGFR mRNA (23). Patients with NSCLC with high METTL3 expression combined with EGFR mutations exhibit shorter progression-free survival compared to those with EGFR mutations and METTL3 low expression, suggesting that METTL3 and EGFR work synergistically (24). In contrast, the expression of METTL3 is downregulated in KRAS mutant NSCLC tissues (24). Compared with the expression levels in TP53 non-mutated NSCLC tissues, METTL3 expression is not significantly altered in TP53-mutant NSCLC tissues (25).
Cigarette smoking is a major risk factor contributing to both onset and progression of NSCLC by upregulating METTL3 expression, which subsequently silences tumour-suppressor gene expression or activates oncogenes expression that are regulated by METTL3-mediated RNA m6A modification (26,27). For example, hypoxia-inducible factor-1 alpha (HIF-1α) is a key transcription factor in cancer (28). High expression of HIF-1α and downstream genes promotes cancer progression through multiple mechanisms (28). Cigarette smoking increases HIF-1α expression that, in turn, increases METTL3 transcription in NSCLC (29). Increased METTL3 expression mediates m6A modification of cyclin-dependent kinase 2-associated protein 2 (CDK2AP2) mRNA, suppressing CDK2AP2 expression that promotes cell-cycle and NSCLC progression (29). Death-associated protein kinase 2 (DAPK2), a tumour suppressor gene, contributes significantly to smoking-related NSCLC progression. Jin et al. (30) demonstrated that METTL3 and YTHDF2 induce aberrant m6A modification of DAPK2 mRNA in NSCLC tissues, resulting in the reduced expression of DAPK2, which significantly facilitates the proliferation and migration abilities of NSCLC by activating the nuclear factor (NF)-κB signalling pathway. Secreted frizzled-related protein 2 (SFRP2) also inhibits NSCLC. Zhao et al. (31) found that METTL3 negatively regulates SFRP2 expression, which, in turn, activates the Wnt/β-catenin signalling pathway to promote NSCLC tumorigenesis. Consistent with this study, the Fraser syndrome protein 1 (FRAS1) transcript undergoes METTL3-regulated m6A modification that correlates with poor prognosis in NSCLC (32). The METTL3-FRAS1 axis contributes to NSCLC cell proliferation, colony formation, and tumour growth by regulating CDON which cooperates with YTHDF1 (32). Furthermore, METTL3 acts as an oncogene directly by promoting BCL2 mRNA translation and expression via m6A modification in NSCLC, enhancing the viability and migration of tumour cells (33).
Lung adenocarcinoma (LUAD), the dominant type of NSCLC, is common in non-smokers and typically arises from the smaller bronchial epithelium (34). LUAD tissue often has poorly defined boundaries; moreover, it is frequently accompanied by fibrosis and subleukocyte scar formation (35). Current LUAD analyses have revealed significant increases of METTL3 expression in cancer tissues, demonstrating its pivotal role in the pathogenesis of LUAD, as well as strong links to decreased overall survival (36,37). A large amount of evidence has demonstrated the significant roles of METTL3 in LUAD, including stemness maintenance, proliferation promotion, migration facilitation, progression acceleration, and apoptosis inhibition (36,37). The function of METTL3 in LUAD onset is contingent on its methyltransferase activity. For example, enolase 1 (ENO1) is a glycolysis enzyme, which participates in cancer progression. Ma et al. (38) reported that the increased METTL3 expression facilitates the binding of ENO1 mRNA with YTHDF1, resulting in enhanced ENO1 translation. This consequently promoted spheroid generation in LUAD cells and intrapulmonary tumour formation in mice by stimulating glycolysis and tumorigenesis in patients with LUAD. Similarly, Choe et al. (39) reported that increased METTL3 expression enhances the translation of oncogenic bromodomain-containing protein 4 (BRD4) mRNA, thereby promoting LUAD tumorigenicity. F-box and WD repeat domain containing 7 (FBXW7) functions as a tumour suppressor in human cancer. Wu et al. (40) reported that METTL3 functions in increasing m6A modification of FBXW7 mRNA, promoting its translation, inhibiting apoptosis while promoting proliferation in LUAD cells (40).
EMT is a significant factor in tumour progression (41). Current study has suggested significant contributions of METTL3 to its regulation by targeting multiple mRNAs. For example, JUNB is a dominant transcriptional regulator of EMT (42). A study by Wanna-Udom et al. (42) demonstrated that the increased expression of METTL3 in LUAD enriches m6A modification of JUNB mRNA and promotes its stability, which subsequently leads to transforming growth factor β-induced EMT, facilitating LUAD progression. In line with this, it has been shown that in an inflammatory microenvironment, interleukin (IL)-6 transcriptionally activates METTL3 expression (43). This activation promotes the proliferation, migration, invasion, and EMT of LUAD cells by increasing Yes-associated protein 1 (YAP1) mRNA expression, activating the YAP1/TEAD signalling pathway (43). Trophinin-associated protein (TROAP) mRNA expression is regulated by METTL3 and is highly expressed in NSCLC, which accelerates its progression through the PI3K/AKT and EMT pathways, suggesting TROAP as a novel target for NSCLC therapy (44).
Communication between tumour cells and the TME facilitates tumour growth by regulating immune escape, inflammation, and metastasis, contributing to the tumorigenesis and tumour progression (45,46). Cancer-associated fibroblasts (CAFs) are a major component of the TME that are linked strongly to NSCLC metastasis by mediating m6A modifications in tumour cells (47-49). CAFs accelerate the malignant progression of tumours by producing cytokines and growth factors (49,50). For example, CAFs secrete collagen type X alpha 1 (COL10A1) and RAC3 that indicate poor prognosis in NSCLC. Li et al. (51) and Chen et al. (52) reported that the function of CAFs in NSCLC depends on METTL3 modification of RAC3 and COL10A1 mRNAs that upregulates their m6A levels, stability, and translation. METTL3-modified RAC3 and COL10A1 promote NSCLC growth, suggesting that they are downstream targets of METTL3. The axes of METTL3-RAC3 and METTL3-COL10A1 may provide therapeutic targets for NSCLC treatment. Moreover, CAFs may secrete METTL3 (53). The CAF-derived METTL3 alleviates programmed cell death ligand-1 (PD-L1)-mediated immunosuppression of NSCLC by targeting IL-18. Subsequently, IL-18 enhances NSCLC immunosuppression by stimulating NF-κB signalling (53).
Together, these findings demonstrate that the abnormal expression of METTL3 functions both directly as an oncogene and regulates oncogene expression indirectly. Specifically, the aberrant m6A modification mediated by METTL3 on tumour-related genes such as CDK2AP2, DAPK2, SFRP2, FRAS1, BRD4, JUNB, YAP1, TROAP, COL10A1, and RAC3, as well as serving directly as an oncogene by regulating Bcl-2, ENO1, FBXW7, and IL-18, triggering the onset and exacerbating the progression of NSCLC, provides novel insights into the mechanisms driving NSCLC advancement, showing great potential for diagnosis and prognosis in NSCLC, and identifying a potential therapeutic target for patients with NSCLC (Figure 3).
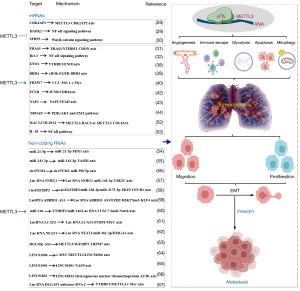
METTL3 functions in NSCLC by regulating non-coding RNAs
Non-coding RNAs, including microRNAs (miRNAs), circular RNAs (circRNAs), and long non-coding RNAs (lncRNAs) play important roles in NSCLC proliferation, apoptosis, migration, and invasion, in which METTL3 is essential for their regulation. For example, increased expression of METTL3 enhances miR-21-5p maturation, which, in turn, targets FDX1 and increases tumorigenicity (54). Besides, Wang et al. (55) demonstrated that the increased expression of METTL3 promotes the splicing of a miR-143-3p precursor to enhance its biogenesis, augmenting lung cancer angiogenesis by targeting the 3’-untranslated region (3’-UTR) of vasotocin-1 (VASH1) mRNA and suppressing its expression. Therefore, METTL3/miR-143-3p/VASH1 axis is an unfavourable prognostic factor for the progression and overall survival rate of LUAD (55). Another study demonstrated that METTL3 is significantly upregulated in LUAD cell lines and regulates LUAD progression by interacting with miR-590-5p to increase the expression of its direct target lncRNA NUTM2A-AS1 (68).
RNA pumilio RNA binding family member 1 (circPUM1) plays important roles in the tumorigenesis of several cancers and is expressed in both NSCLC tissues and cell lines (69). Li et al. (56) discovered that the increased circPUM1 expression leads to NSCLC tumour proliferation and glycolysis by downregulating miR-590-5p and upregulating METTL3. Ubiquitin-conjugating enzyme 2C (UBE2C), often overexpressed in cancers, plays an indispensable role in cancer progression (57). Given the correlation between UBE2C expression and pan-cancer as well as poor prognosis in humans, Jiang et al. (57) reported that METTL3 upregulation reduces the degradation rate of lncRNA SNHG1 in NSCLC. This particular RNA functions as a competing endogenous RNA that sponges miR-140-3p and increases the expression of UBE2C in lung squamous cell carcinoma (LUSC) cell lines (57). These findings suggest that METTL3 contributes to the function of the SNHG1/miRNA-140-3p axis, regulating UBE2C expression and NSCLC progression. Another study demonstrated that the coordination between METTL3 and YTHDC1 promotes the upregulation of circIGF2BP3 (58). This RNA then acts as a sponge for miR-328-3p and miR-3173-5p, which competitively increases the expression of PKP3 (58). Subsequently, PKP3 interacts with the RNA-binding protein FXR1 to stabilize OTUB1 mRNA (58). The resulting increase in OTUB1 facilitates PD-L1 deubiquitination, leading to CD8+ T cell immune escape in patients with NSCLC, ultimately exacerbating the disease (58).
LncRNAs contain over 200 bases that do not code for proteins; however, they are linked to tumorigenesis and metastasis by serving as oncogenes or by interacting with target mRNAs, miRNAs and proteins (70,71). The functions of lncRNAs in NSCLC have been extensively reported; they are considered as risk factors for NSCLC that regulate the proliferation, metastasis, and immune resistance of NSCLC via interactions with METTL3 (72). For example, lncRNA ABHD11-AS1 is overexpressed in NSCLC tissues and cells; moreover, this overexpression is strongly correlated with an unfavourable NSCLC prognosis (59). Xue et al. (59) demonstrated that the upregulation of METTL3 caused the m6A modification on ABHD11-AS1 to enhance its expression, which subsequently targeted and suppressed the expression of KLF4 (a cancer inhibitor), thereby promoting proliferation and the Warburg effect in NSCLC cells. These findings collectively suggest that ABHD11-AS1 might act as an oncogene in NSCLC tumorigenesis, which partially depends on the assistance of METTL3-mediated RNA m6A modification. Similarly, Li et al. (60) revealed that increased expression of METTL3, together with YTHDF2, controlled the stemness and EMT features of LUAD cell lines by modulating the intrinsic levels of miR-146 and lncRNA TUSC7 by activating the Notch signalling pathway and targeting Snail mRNA. The lung cancer-associated transcript 3 (LCAT3), a novel oncogenic lncRNA, is overexpressed in LUAD; this overexpression correlates with poor prognosis (61). The upregulation of LCAT3 is attributed to m6A modification facilitated by METTL3, which subsequently results in the stabilization of LCAT3 (61). Mechanistically, LCAT3 recruits far upstream element binding protein 1 (FUBP1) to the MYC far upstream element sequence, activating MYC transcription and promoting lung cancer cell proliferation, survival, invasion, and metastasis (61). Collectively, these oncogenic effects are achieved through the METTL3/LCAT3- FUBP1/FUSE axis.
The increased expression of lncRNA nuclear paraspeckle assembly transcript 1 (NEAT1) is associated with decreased survival in patients with NSCLC. Qi et al. (62) found that the abnormally increased expression of NEAT1 was strongly linked to METTL3-mediated m6A modification that stabilized NEAT1. This subsequently increased the expression of high-mobility group AT-hook 1 (HMGA1) by sponging miR-361-3p. Therefore, METTL3 triggered the signalling via the NEAT1/miR-361-3p/HMGA1 axis that functioned to promote NSCLC tumorigenesis and metastasis. Similarly, lncRNA deoxyguanosine kinase antisense RNA 1 (DGUOK-AS1) has been reported to be a driver in NSCLC. Feng et al. (63) found that the upregulation of DGUOK-AS1 promoted NSCLC metastasis indirectly by targeting TRPM7 mRNA, which was regulated through METTL3/IGF2BP2-mediated m6A modification.
The oncogenes in NSCLC are c-MYC, METTL3, and LINC01006. Liu et al. (64) found that c-MYC increased METTL3 expression, and METTL3 in turn functioned as an upstream regulator of LINC01006 via m6A modification to stabilize LINC01006. Moreover, both c-MYC and LINC01006 functioned by targeting miR-34a/b/c and miR-2682 (64). This study indicated a positive feedback loop, with METTL3 as its central regulator. This provides another reason to explore the therapeutic targeting of METTL3 for NSCLC. Exosomes also have been found to increase the expression of METTL3. Xu et al. (65) demonstrated that M2 macrophage exosomal LINC01001 induced METTL3 expression, which, in turn, targeted NASP mRNA to alter glycolysis in NSCLC cells to promote NSCLC progression. Other lncRNAs that have been regulated by METTL3 in NSCLC include LINC01833, which promotes NSCLC progression by regulating heterogeneous nuclear ribonucleoprotein A2/B1 expression (66); lncRNA DLGAP1 antisense RNA 2 promotes NSCLC aerobic glycolysis and tumorigenesis by regulating the YTHDF1/METTL3/c-Myc axis (67) (Figure 3).
In summary, recent investigations into the relationship between METTL3 and NSCLC collectively affirm that METTL3-mediated m6A epigenetic modifications to RNAs play significant roles in the pathogenesis, development, and overall health status of NSCLC. Consequently, using METTL3 as a biomarker can possibly help in the clinical diagnosis and prognostic assessment of patients with NSCLC. Finally, targeting the regulation of METTL3 is a potential strategy for treating patients at multiple stages of NSCLC.
METTL3 is strongly associated with therapeutic resistance in NSCLC
The resistance of tumour cells to therapeutic drugs is a significant contributor to treatment failure. Besides its role in regulating cellular functions, METTL3 has been implicated in the modulation of therapeutic sensitivity in NSCLC.
METTL3 contributes to NSCLC chemoresistance
Chidamide, cisplatin, and gefitinib are usually used as the first-line drugs in NSCLC treatment. However, resistance is common during treatment. Ding et al. (73) found that chidamide upregulates the expression of METTL3 and WTAP, which subsequently promotes c-MET mRNA m6A modification to increase c-MET expression and reduce sensitivity to crizotinib (a kinase inhibitor used for treating NSCLC with ALK mutations), in a c-MET/hepatocyte growth factor (HGF)-dependent manner. Furthermore, a separate study demonstrated that miR-4443 expression is elevated in exosomes derived from cisplatin-resistant NSCLC tumour tissues compared to those derived from cisplatin-sensitive tissues (74). This elevation inhibited cisplatin-induced FSP1-mediated ferroptosis in vitro and promoted tumour growth in vivo (74). Further mechanistic analyses revealed that miR-4443 regulates the expression of FSP1 through an m6A-dependent mechanism involving METTL3, which has been identified as a direct target of miR-4443 (74). Additionally, increased METTL3 expression significantly correlated with susceptibility to cisplatin, more advanced tumour stage, nodal involvement, and lymph-node metastasis in NSCLC (75). This suggests that METTL3 plays a role in disease progression and chemoresistance by regulating AKT1 mRNA m6A levels to promote its expression (75). Similarly, study on the mechanism of cisplatin resistance in NSCLC cells has revealed that exosome-mediated circVMP1 promotes NSCLC tumour progression and resistance to cisplatin by targeting miR-524-5p-METTL3-SOX2 signalling (76). Therefore, targeting METTL3 also may be a viable therapeutic strategy for overcoming chemoresistance in NSCLC.
Gefitinib often leads to acquired drug resistance in patients with LUAD, resulting in treatment failure. Increased expression of small nucleolar host gene 17 (SNHG17) contributes to LUAD progression and gefitinib resistance by aggravating the malignant phenotypes (77). METTL3-mediated SNHG17 m6A modification stabilizes SNHG17 transcripts and induces SNHG17 overexpression, which subsequently represses LATS2 expression by recruiting EZH2 to the promoter region of LATS2 (77). Collectively, these findings suggest that METTL3 triggers the action of SNHG17/EZH2/LATS2 signalling in promoting gefitinib resistance. Additionally, gefitinib resistance induced by EGFR mutations is also regulated by METTL3-mediated m6A modification. The EGFR-tyrosine kinase inhibitor (EGFR-TKI) gefitinib is the standard first-line therapy for patients with EGFR mutant NSCLC (78,79). However, acquired resistance often occurs during this treatment (80). Gao et al. (81) demonstrated that relative to gefitinib-sensitive tissues, METTL3 expression is increased in gefitinib-resistant tissues. Further mechanistic study has revealed that the combination of METTL3 and EMT gives rise to the activation of the PI3K/Akt signalling pathway, modulating gefitinib sensitivity (81). In line with this study, Dai et al. (82) indicated that LINC00969 regulated resistance to gefitinib by interacting with EZH2 and METTL3, transcriptionally regulating the level of H3K27me3 in the NLRP3 promoter, and post-transcriptionally modifying the m6A level of NLRP3 in an m6A-YTHDF2-dependent manner, thus epigenetically repressing NLRP3 expression to suppress the activation of classical NLRP3/caspase-1/GSDMD-related pyroptosis signalling, endowing an antipyroptotic phenotype, and promoting TKI resistance. Therefore, METTL3 can provide a molecular marker for predicting the efficacy of EGFR-TKI therapy and represents a potential therapeutic target. Liu et al. (83) demonstrated that METTL3-mediated autophagy plays a crucial regulatory role in reversing β-elemene resistance to gefitinib in NSCLC cells. Increased expression of METTL3 in LUAD tissues has been shown to reduce the methylation of RNA m6A in drug-resistant cells, increase the expression of key autophagy pathway genes, including those that encode chelate 1, microtubule-associated protein 1b-light chain 3-II, autophagy-related genes (ATG5 and ATG7), increasing β-elemene production, and ultimately reversing gefitinib resistance (83). These findings provide insights into additional potential targets for molecular therapy and NSCLC therapeutics for patients whose tumours are resistant to gefitinib (Figure 4).
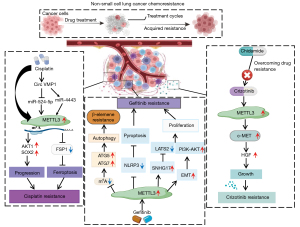
METTL3 is strongly linked to NSCLC immunotherapeutic resistance
Immunotherapy has become the first-line treatment for advanced NSCLC, but most patients experience treatment failure (84,85). Interaction between PD-1 and PD-L1 drives resistance (86). METTL3 showed significant associations with the immune microenvironment, as well as tumour mutation burden and PD-L1 levels, suggesting that METTL3 mediated m6A RNA methylation is indicative of therapeutic effects of anti-PD-L1 treatment (21,87). Sun et al. (88) reported that in NSCLC, METTL3/YTHDF2-mediated m6A modification increased the expression of LINC02418, which subsequently interacted with TRIM21 mRNA to suppress PD-L1 expression and promote CD8+ T cell infiltration. Therefore, the METTL3/YTHDF2-LINC02418-Trim21-PD-L1 axis contributes to NSCLC immunotherapeutic resistance. M2 phenotype tumour-associated macrophages (TAMs) are enriched in the tumour tissues of patients with immunoresistant LUAD (89,90). Wu et al. (91) found that M2-TAMs function to promote immunoresistance by increasing METTL3 expression and the total m6A RNA level.
METTL3 functions in NSCLC radiotherapy resistance
Ionizing radiation is the standard radiation therapy for NSCLC; particularly carbon-ion radiotherapy, a radical nonsurgical treatment with high local control rates and rare serious adverse events (92,93). A recent study found that NSCLC cells developed resistance to carbon ion radiotherapy that might be due to METTL3-mediated m6A modification. Xu et al. (94) showed that METTL3 expression was increased in NSCLC cells with carbon-ion radiotherapy, which modified H2A histone family member X (H2AX) mRNA to decrease its expression, reducing DNA damage repair and cell survival.
In summary, these studies demonstrate that METTL3-mediated RNA m6A methylation plays a significant role in modulating therapeutic sensitivity in NSCLC, highlighting METTL3 as a potential predictor or target of resistance to chemotherapeutic drugs, immunotherapy, and radiotherapy.
Prospective clinical applications
Increased METTL3 expression in NSCLC tissues and advanced-stage lung cancers compared to adjacent tissues has been demonstrated (5). Furthermore, increased METTL3 expression triggers the onset and progression of NSCLC by modulating the expression for both coding and non-coding RNAs (5). Therefore, m6A modification by METTL3 could be useful as prospective diagnostic or prognostic biomarkers for NSCLC (5). For example, Zhang et al. (95) tested FTO and METTL3 as prognostic biomarkers for evaluating benign and malignant tumours and predicting outcomes in patients with NSCLC. These studies also underscore the potential of downregulating METTL3 expression or regulating METTL3 targets in NSCLC treatment. Li et al. (96) recently reported that ammonium tetrathiomolybdate enhanced cell growth at low concentrations by increasing METTL3 expression in LUAD. Inhibition of METTL3 significantly attenuated proliferation by enhancing the expression of the eukaryotic translation initiation factor (eIF), underscoring the pivotal role of METTL3 in governing protein synthesis and cellular growth (96).
Currently, multiple methods have been reported for suppressing METTL3 expression in NSCLC treatment, for example, by using small-molecule inhibitors. As reported by Xiao et al. (97), STM2457, a small molecule, decreased METTL3 expression in NSCLC and increased PD-L1 expression, improving immunotherapy outcomes based on PD-L1 upregulation. Consistent with these data, Yu et al. (98) showed that the targeted regulation of METTL3 reprogramed the TME and improved immunotherapy. Interestingly, Du et al. (99) reported that miR-33a decreased the expression of METTL3 at both the mRNA and protein levels. They transfected miR-33a, targeting the 3'UTR of METTL3 mRNA, and attenuated survival and proliferation of NSCLC cell lines (99). In addition, Wei et al. (100) demonstrated that transfection with miR-600 decreased expression of METTL3 and induced apoptosis in NSCLC cell lines (as evidenced by increased Bax/Bcl-2 ratios) via the PI3K/AKT pathway. Moreover, Huang et al. (101) found that METTL3 upregulation led to increased m6A modification of miR-1246, promoting NSCLC progression by inhibiting the expression of paternally expressed gene 3 (PEG3). Conversely, knockdown of METTL3 or overexpression of PEG3 suppressed the malignant behaviour of NSCLC cells. Chen et al. (102) demonstrated that simvastatin treatment induced decreased expression of METTL3, which inhibited EMT progression through an IGF2BP2-dependent m6A modification on the target EZH2 mRNA (102). This suppressed the malignant characteristics of lung cancer, curbing further progression.
Besides inhibiting METTL3 directly for NSCLC treatment, targeting METTL3-regulated RNAs may be promising therapeutically. For example, metformin has been reported to improve the prognosis of patients with malignant tumours by inhibiting METTL3-mediated m6A modification of THRAP3, RBM25, and USP4 mRNAs to repress their expression, hamper cell proliferation, and promote apoptosis (103). Besides, Feng et al. (104) reported that decreasing expression of METTL3 by β-elemene attenuated the malignant behaviour of NSCLC by inhibiting phosphatase and tensin homolog (PTEN) mRNA degradation. RNA binding motif 10 (RBM10) is a potential tumour-suppressor protein that inhibits proliferation and promotes NSCLC apoptosis. Cao et al. (105) found that RBM10 functions as an RNA-binding protein that inhibits the m6A methylation of MALAT1 by recruiting METTL3 and altering phosphorylation of the downstream PI3K/AKT/mTOR pathway, ultimately inhibiting the invasion and migration of NSCLC by binding and regulating MALAT. Similarly, miR-1915-3p suppression significantly impedes migration, invasion, and EMT in NSCLC tissues and cell lines (106). Pan et al. (106) reported that METTL3/YTHDF2 signalling decreased miR-1915-3p levels through transcription factor KLF4, which directly binds to the 3'UTR of SET mRNA and modulates its expression via JNK/Jun and NF-kB signalling (Table 1).
Table 1
Inhibitor | METTL3 | ||
---|---|---|---|
Target | Function | Reference | |
STM2457 | PD-L1 | Improve immunotherapy | (97) |
miR-33a | METTL3 | Attenuate proliferation | (99) |
miR-600 | Bax/Bcl-2 ratio | Induces apoptosis | (100) |
PEG3 | miR-1246 | Inhibit malignant behaviour | (101) |
Simvastatin | EZH2 | Inhibit EMT progression | (102) |
Metformin | THRAP3, RBM25, USP4 | Hamper proliferation, promote apoptosis | (103) |
β-elemene | PTEN | Malignant behaviour | (104) |
RBM10 | MALAT1 | Inhibit proliferation, promote apoptosis | (105) |
miR-1915-3p | KLF4 | Impede migration, invasion, EMT | (106) |
Downregulation of METTL3 directly or indirectly by different inhibitors rescues NSCLC via different targets and mechanisms. NSCLC, non-small cell lung cancer; PD-L1, programmed cell death ligand-1; EMT, epithelial-mesenchymal transition.
In summary, elevated METTL3 expression plays a crucial role in NSCLC pathogenesis via diverse mechanisms and pathways. Therefore, METTL3 is a promising biomarker for the clinical diagnosis and prognosis of NSCLC. Moreover, downregulating METTL3 expression or regulating its targets may be effective by inhibiting cell proliferation and progression or inducing apoptosis.
Conclusions
Ongoing studies on m6A modification show an increasing number of reports highlighting the role of METTL3 in regulating NSCLC. However, a comprehensive summary and analysis of the relevance and potential of METTL3 in the pathogenesis, clinical diagnosis, treatment, and prognosis of NSCLC are lacking. This review provides an updated summary of studies on METTL3 in NSCLC initiation, progression, chemoresistance, immunoresistance, and radioresistance and analysis their interrelationships, particularly their potential significance in clinical settings.
Our review analyzes how METTL3 contributes to the tumorigenesis and progression of NSCLC, especially LUAD, by regulating both mRNA and non-coding RNAs through distinct mechanisms. These include the regulation of cellular functions, including stemness, proliferation, migration, pyroptosis, autophagy, and apoptosis. Moreover, the mechanism of METTL3 action in NSCLC also involves the regulation of biological processes including the EMT, cell cycle, angiogenesis, TME, energy metabolism of the Warburg effect and glycolysis, immune escape, and immune microenvironment.
These data provide strong evidence to support the use of METTL3 expression as a promising biomarker for the diagnosis and prognosis of NSCLC. The downregulation of METTL3 may provide a potential therapeutic strategy for NSCLC treatment by inhibiting cell proliferation and progression or inducing cell death.
However, despite extensive research, we infer that current studies on METTL3 modifications in NSCLC are limited in their ability to explain the dynamic regulatory mechanisms involved in the onset and progression of these conditions. Although some studies have suggested that targeting METTL3-related regulatory factors and signalling pathways may have therapeutic potential for NSCLC, further large-scale clinical data are needed to support this hypothesis. Therefore, we propose some new avenues for future investigation. First, adenosine-to-inosine (A-to-I) editing of RNA is a common RNA modification in mammals that is catalysed by adenosine deaminase acting on RNA (ADAR) enzymes (107). Several groups have indicated the interaction between METTL3 and ADAR-mediated RNA editing in multiple cancers, indicating that METTL3 RNA is subject to RNA editing; moreover, METTL3 itself participates in RNA editing, e.g., in glioma (107). However, to the best of our knowledge, studies of the interaction between METTL3 and ADAR-mediated RNA editing in NSCLC are lacking. Therefore, exploring the interaction between METTL3 and ADAR-mediated RNA editing might be a novel perspective for understanding mechanisms of NSCLC pathogenesis. Second, although several methods have been reported to be effective in inhibiting METTL3 expression, exploring more specific methods with large-scale clinical data are needed for guiding more precise targeted therapies in clinical setting. Finally, the downstream targets of METTL3 need to be further identified and characterized, which will help us further understand the mechanism of METTL3 in NSCLC.
To sum up, this review has analysed the complex relationships between METTL3 and NSCLC, enhancing our understanding of its pathogenesis and treatment from a novel perspective of METTL3-mediated RNA m6A modification, serving as a valuable reference for both research and clinical settings.
Acknowledgments
Funding: This work was supported by
Footnote
Peer Review File: Available at https://tlcr.amegroups.com/article/view/10.21037/tlcr-24-85/prf
Conflicts of Interest: All authors have completed the ICMJE uniform disclosure form (available at https://tlcr.amegroups.com/article/view/10.21037/tlcr-24-85/coif). The authors have no conflicts of interest to declare.
Ethical Statement: The authors are accountable for all aspects of the work in ensuring that questions related to the accuracy or integrity of any part of the work are appropriately investigated and resolved.
Open Access Statement: This is an Open Access article distributed in accordance with the Creative Commons Attribution-NonCommercial-NoDerivs 4.0 International License (CC BY-NC-ND 4.0), which permits the non-commercial replication and distribution of the article with the strict proviso that no changes or edits are made and the original work is properly cited (including links to both the formal publication through the relevant DOI and the license). See: https://creativecommons.org/licenses/by-nc-nd/4.0/.
References
- Sack C, Simpson C, Pacheco K. The Emerging Spectrum of Respiratory Diseases in the U.S. Cannabis Industry. Semin Respir Crit Care Med 2023;44:405-14. [Crossref] [PubMed]
- Batra U, Munshi A, Kabra V, et al. Relevance of multi-disciplinary team approach in diagnosis and management of Stage III NSCLC. Indian J Cancer 2022;59:S46-55. [Crossref] [PubMed]
- Alexander M, Kim SY, Cheng H. Update 2020: Management of Non-Small Cell Lung Cancer. Lung 2020;198:897-907. [Crossref] [PubMed]
- Pasello G, Scattolin D, Bonanno L, et al. Secondary prevention and treatment innovation of early stage non-small cell lung cancer: Impact on diagnostic-therapeutic pathway from a multidisciplinary perspective. Cancer Treat Rev 2023;116:102544. [Crossref] [PubMed]
- Alduais Y, Zhang H, Fan F, et al. Non-small cell lung cancer (NSCLC): A review of risk factors, diagnosis, and treatment. Medicine (Baltimore) 2023;102:e32899. [Crossref] [PubMed]
- Waterer G. The global burden of respiratory infectious diseases before and beyond COVID. Respirology 2023;28:95-6. [Crossref] [PubMed]
- Chang YS, Tu SJ, Chen YC, et al. Mutation profile of non-small cell lung cancer revealed by next generation sequencing. Respir Res 2021;22:3. [Crossref] [PubMed]
- Li Q, Wang R, Yang Z, et al. Molecular profiling of human non-small cell lung cancer by single-cell RNA-seq. Genome Med 2022;14:87. [Crossref] [PubMed]
- Leader AM, Grout JA, Maier BB, et al. Single-cell analysis of human non-small cell lung cancer lesions refines tumor classification and patient stratification. Cancer Cell 2021;39:1594-1609.e12. [Crossref] [PubMed]
- Cesaro B, Tarullo M, Fatica A. Regulation of Gene Expression by m6Am RNA Modification. Int J Mol Sci 2023;24:2277. [Crossref] [PubMed]
- Liu Y, Yang D, Liu T, et al. N6-methyladenosine-mediated gene regulation and therapeutic implications. Trends Mol Med 2023;29:454-67. [Crossref] [PubMed]
- You S, Su X, Ying J, et al. Research Progress on the Role of RNA m6A Modification in Glial Cells in the Regulation of Neurological Diseases. Biomolecules 2022;12:1158. [Crossref] [PubMed]
- Fang Z, Mei W, Qu C, et al. Role of m6A writers, erasers and readers in cancer. Exp Hematol Oncol 2022;11:45. [Crossref] [PubMed]
- Wong JM, Eirin-Lopez JM. Evolution of Methyltransferase-Like (METTL) Proteins in Metazoa: A Complex Gene Family Involved in Epitranscriptomic Regulation and Other Epigenetic Processes. Mol Biol Evol 2021;38:5309-27. [Crossref] [PubMed]
- Qi YN, Liu Z, Hong LL, et al. Methyltransferase-like proteins in cancer biology and potential therapeutic targeting. J Hematol Oncol 2023;16:89. [Crossref] [PubMed]
- Liu H, Huang Y, Lu S, et al. Bibliometric analysis of METTL3: Current perspectives, highlights, and trending topics. Open Life Sci 2023;18:20220586. [Crossref] [PubMed]
- Li G, Sun Z, Deng W, et al. METTL3 plays a crucial function in multiple biological processes. Acta Histochem 2022;124:151916. [Crossref] [PubMed]
- Ledford H. How air pollution causes lung cancer - without harming DNA. Nature 2023;616:419-20. [Crossref] [PubMed]
- Jin Q, Qu H, Quan C. New insights into the regulation of METTL3 and its role in tumors. Cell Commun Signal 2023;21:334. [Crossref] [PubMed]
- Weissferdt A, Sepesi B, Ning J, et al. Optimal Combination of Neuroendocrine Markers for the Detection of High-Grade Neuroendocrine Tumors of the Sinonasal Tract and Lung. Curr Oncol Rep 2023;25:1-10. [Crossref] [PubMed]
- Liu X, Ma C, Liu H, et al. M6A regulator expression patterns predict the immune microenvironment and prognosis of non-small cell lung cancer. J Cancer Res Clin Oncol 2022;148:2803-14. [Crossref] [PubMed]
- Diao MN, Zhang XJ, Zhang YF. The critical roles of m6A RNA methylation in lung cancer: from mechanism to prognosis and therapy. Br J Cancer 2023;129:8-23. [Crossref] [PubMed]
- Lin S, Choe J, Du P, et al. The m(6)A Methyltransferase METTL3 Promotes Translation in Human Cancer Cells. Mol Cell 2016;62:335-45. [Crossref] [PubMed]
- Li Y, Zhang X, Cai J, et al. The pathological tissue expression pattern and clinical significance of m6A-regulatory genes in non-small cell lung cancer. J Gene Med 2022;24:e3397. [Crossref] [PubMed]
- Zhao Z, Wan J, Guo M, et al. Expression and prognostic significance of m6A-related genes in TP53-mutant non-small-cell lung cancer. J Clin Lab Anal 2022;36:e24118. [Crossref] [PubMed]
- Jhanwar SC, Xu XL, Elahi AH, et al. Cancer genomics of lung cancer including malignant mesothelioma: A brief overview of current status and future prospects. Adv Biol Regul 2020;78:100723. [Crossref] [PubMed]
- Molina JR, Yang P, Cassivi SD, et al. Non-small cell lung cancer: epidemiology, risk factors, treatment, and survivorship. Mayo Clin Proc 2008;83:584-94. [Crossref] [PubMed]
- Satija S, Kaur H, Tambuwala MM, et al. Hypoxia-Inducible Factor (HIF): Fuel for Cancer Progression. Curr Mol Pharmacol 2021;14:321-32. [Crossref] [PubMed]
- Yang Y, Cheng C, He B, et al. Cigarette smoking, by accelerating the cell cycle, promotes the progression of non-small cell lung cancer through an HIF-1α-METTL3-m(6)A/CDK2AP2 axis. J Hazard Mater 2023;455:131556. [Crossref] [PubMed]
- Jin M, Li G, Liu W, et al. Cigarette smoking induces aberrant N6-methyladenosine of DAPK2 to promote non-small cell lung cancer progression by activating NF-κB pathway. Cancer Lett 2021;518:214-29. [Crossref] [PubMed]
- Zhao S, Song P, Zhou G, et al. METTL3 promotes the malignancy of non-small cell lung cancer by N6-methyladenosine modifying SFRP2. Cancer Gene Ther 2023;30:1094-104. [Crossref] [PubMed]
- Dou X, Wang Z, Lu W, et al. METTL3 promotes non-small cell lung cancer (NSCLC) cell proliferation and colony formation in a m6A-YTHDF1 dependent way. BMC Pulm Med 2022;22:324. [Crossref] [PubMed]
- Zhang Y, Liu S, Zhao T, et al. METTL3-mediated m6A modification of Bcl-2 mRNA promotes non-small cell lung cancer progression. Oncol Rep 2021;46:163. [Crossref] [PubMed]
- Fassi E, Mandruzzato M, Zamparini M, et al. Clinical presentation and outcome of patients with enteric-type adenocarcinoma of the lung: A pooled analysis of published cases. Lung Cancer 2023;179:107176. [Crossref] [PubMed]
- Lucà S, Zannini G, Morgillo F, et al. The prognostic value of histopathology in invasive lung adenocarcinoma: a comparative review of the main proposed grading systems. Expert Rev Anticancer Ther 2023;23:265-77. [Crossref] [PubMed]
- Bolan PO, Zviran A, Brenan L, et al. Genotype-Fitness Maps of EGFR-Mutant Lung Adenocarcinoma Chart the Evolutionary Landscape of Resistance for Combination Therapy Optimization. Cell Syst 2020;10:52-65.e7. [Crossref] [PubMed]
- Wang Y, Zhao X, Li J, et al. Four m6A RNA Methylation Gene Signatures and Their Prognostic Values in Lung Adenocarcinoma. Technol Cancer Res Treat 2022;21:15330338221085373. [Crossref] [PubMed]
- Ma L, Xue X, Zhang X, et al. The essential roles of m(6)A RNA modification to stimulate ENO1-dependent glycolysis and tumorigenesis in lung adenocarcinoma. J Exp Clin Cancer Res 2022;41:36. [Crossref] [PubMed]
- Choe J, Lin S, Zhang W, et al. mRNA circularization by METTL3-eIF3h enhances translation and promotes oncogenesis. Nature 2018;561:556-60. [Crossref] [PubMed]
- Wu Y, Chang N, Zhang Y, et al. METTL3-mediated m(6)A mRNA modification of FBXW7 suppresses lung adenocarcinoma. J Exp Clin Cancer Res 2021;40:90. [Crossref] [PubMed]
- Tan T, Shi P, Abbas MN, et al. Epigenetic modification regulates tumor progression and metastasis through EMT Int J Oncol 2022;60:70. (Review). [Crossref] [PubMed]
- Wanna-Udom S, Terashima M, Lyu H, et al. The m6A methyltransferase METTL3 contributes to Transforming Growth Factor-beta-induced epithelial-mesenchymal transition of lung cancer cells through the regulation of JUNB. Biochem Biophys Res Commun 2020;524:150-5. [Crossref] [PubMed]
- Ni XF, Xie QQ, Zhao JM, et al. The hepatic microenvironment promotes lung adenocarcinoma cell proliferation, metastasis, and epithelial-mesenchymal transition via METTL3-mediated N6-methyladenosine modification of YAP1. Aging (Albany NY) 2021;13:4357-69. [Crossref] [PubMed]
- Xu M, Yu J, Liu X, et al. METTL3 regulatory TROAP can regulate the progression of non-small cell lung cancer through PI3K/AKT and EMT signaling pathway. Med Oncol 2023;40:274. [Crossref] [PubMed]
- Hinshaw DC, Shevde LA. The Tumor Microenvironment Innately Modulates Cancer Progression. Cancer Res 2019;79:4557-66. [Crossref] [PubMed]
- Xiao Y, Yu D. Tumor microenvironment as a therapeutic target in cancer. Pharmacol Ther 2021;221:107753. [Crossref] [PubMed]
- Sahai E, Astsaturov I, Cukierman E, et al. A framework for advancing our understanding of cancer-associated fibroblasts. Nat Rev Cancer 2020;20:174-86. [Crossref] [PubMed]
- Lavie D, Ben-Shmuel A, Erez N, et al. Cancer-associated fibroblasts in the single-cell era. Nat Cancer 2022;3:793-807. [Crossref] [PubMed]
- Liao Z, Tan ZW, Zhu P, et al. Cancer-associated fibroblasts in tumor microenvironment - Accomplices in tumor malignancy. Cell Immunol 2019;343:103729. [Crossref] [PubMed]
- Chen Y, McAndrews KM, Kalluri R. Clinical and therapeutic relevance of cancer-associated fibroblasts. Nat Rev Clin Oncol 2021;18:792-804. [Crossref] [PubMed]
- Li Y, Li X, Deng M, et al. Cancer-Associated Fibroblasts Hinder Lung Squamous Cell Carcinoma Oxidative Stress-Induced Apoptosis via METTL3 Mediated m(6)A Methylation of COL10A1. Oxid Med Cell Longev 2022;2022:4320809. [Crossref] [PubMed]
- Chen M, Zhang Q, Zheng S, et al. Cancer-associated fibroblasts promote migration and invasion of non-small cell lung cancer cells via METTL3-mediated RAC3 m(6)A modification. Int J Biol Sci 2023;19:1616-32. [Crossref] [PubMed]
- Xu L, Li K, Li J, et al. IL-18 serves as a main effector of CAF-derived METTL3 against immunosuppression of NSCLC via driving NF-κB pathway. Epigenetics 2023;18:2265625. [Crossref] [PubMed]
- Qian S, Liu J, Liao W, et al. METTL3 promotes non-small-cell lung cancer growth and metastasis by inhibiting FDX1 through copper death-associated pri-miR-21-5p maturation. Epigenomics 2023;15:1237-55. [Crossref] [PubMed]
- Wang H, Deng Q, Lv Z, et al. N6-methyladenosine induced miR-143-3p promotes the brain metastasis of lung cancer via regulation of VASH1. Mol Cancer 2019;18:181. [Crossref] [PubMed]
- Li M, Wang Q, Zhang X, et al. CircPUM1 promotes cell growth and glycolysis in NSCLC via up-regulating METTL3 expression through miR-590-5p. Cell Cycle 2021;20:1279-94. [Crossref] [PubMed]
- Jiang X, Yuan Y, Tang L, et al. Comprehensive Pan-Cancer Analysis of the Prognostic and Immunological Roles of the METTL3/lncRNA-SNHG1/miRNA-140-3p/UBE2C Axis. Front Cell Dev Biol 2021;9:765772. [Crossref] [PubMed]
- Liu Z, Wang T, She Y, et al. N(6)-methyladenosine-modified circIGF2BP3 inhibits CD8(+) T-cell responses to facilitate tumor immune evasion by promoting the deubiquitination of PD-L1 in non-small cell lung cancer. Mol Cancer 2021;20:105. [Crossref] [PubMed]
- Xue L, Li J, Lin Y, et al. m(6) A transferase METTL3-induced lncRNA ABHD11-AS1 promotes the Warburg effect of non-small-cell lung cancer. J Cell Physiol 2021;236:2649-58. [Crossref] [PubMed]
- Li K, Peng ZY, Gao S, et al. M6A associated TSUC7 inhibition contributed to Erlotinib resistance in lung adenocarcinoma through a notch signaling activation dependent way. J Exp Clin Cancer Res 2021;40:325. [Crossref] [PubMed]
- Qian X, Yang J, Qiu Q, et al. LCAT3, a novel m6A-regulated long non-coding RNA, plays an oncogenic role in lung cancer via binding with FUBP1 to activate c-MYC. J Hematol Oncol 2021;14:112. [Crossref] [PubMed]
- Qi L, Yin Y, Sun M. m6A-mediated lncRNA NEAT1 plays an oncogenic role in non-small cell lung cancer by upregulating the HMGA1 expression through binding miR-361-3p. Genes Genomics 2023;45:1537-47. [Crossref] [PubMed]
- Feng Y, Wu F, Wu Y, et al. LncRNA DGUOK-AS1 facilitates non-small cell lung cancer growth and metastasis through increasing TRPM7 stability via m6A modification. Transl Oncol 2023;32:101661. [Crossref] [PubMed]
- Liu C, Ren Q, Deng J, et al. c-MYC/METTL3/LINC01006 positive feedback loop promotes migration, invasion and proliferation of non-small cell lung cancer. Biomed J 2023; Epub ahead of print. [Crossref] [PubMed]
- Xu L, Li K, Li J, et al. M2 macrophage exosomal LINC01001 promotes non-small cell lung cancer development by affecting METTL3 and glycolysis pathway. Cancer Gene Ther 2023;30:1569-80. [Crossref] [PubMed]
- Li D, Fu Z, Dong C, et al. Methyltransferase 3, N6-adenosine-methyltransferase complex catalytic subunit-induced long intergenic non-protein coding RNA 1833 N6-methyladenosine methylation promotes the non-small cell lung cancer progression via regulating heterogeneous nuclear ribonucleoprotein A2/B1 expression. Bioengineered 2022;13:10493-503. [Crossref] [PubMed]
- Zhang Q, Zhang Y, Chen H, et al. METTL3-induced DLGAP1-AS2 promotes non-small cell lung cancer tumorigenesis through m(6)A/c-Myc-dependent aerobic glycolysis. Cell Cycle 2022;21:2602-14. [Crossref] [PubMed]
- Wang J, Zha J, Wang X. Knockdown of lncRNA NUTM2A-AS1 inhibits lung adenocarcinoma cell viability by regulating the miR-590-5p/METTL3 axis. Oncol Lett 2021;22:798. [Crossref] [PubMed]
- Silva ILZ, Kohata AA, Shigunov P. Modulation and function of Pumilio proteins in cancer. Semin Cancer Biol 2022;86:298-309. [Crossref] [PubMed]
- Peng PH, Hsu KW, Chieh-Yu Lai J, et al. The role of hypoxia-induced long noncoding RNAs (lncRNAs) in tumorigenesis and metastasis. Biomed J 2021;44:521-33. [Crossref] [PubMed]
- Ahadi A. Functional roles of lncRNAs in the pathogenesis and progression of cancer. Genes Dis 2021;8:424-37. [Crossref] [PubMed]
- Yi WW, Guo XQ, Xu Y, et al. A prognostic model based on ferroptosis-related long non-coding RNA signatures and immunotherapy responses for non-small cell lung cancer. Eur Rev Med Pharmacol Sci 2023;27:2591-604. [PubMed]
- Ding N, You A, Tian W, et al. Chidamide increases the sensitivity of Non-small Cell Lung Cancer to Crizotinib by decreasing c-MET mRNA methylation. Int J Biol Sci 2020;16:2595-611. [Crossref] [PubMed]
- Song Z, Jia G, Ma P, et al. Exosomal miR-4443 promotes cisplatin resistance in non-small cell lung carcinoma by regulating FSP1 m6A modification-mediated ferroptosis. Life Sci 2021;276:119399. [Crossref] [PubMed]
- Shi L, Gong Y, Zhuo L, et al. Methyltransferase-like 3 upregulation is involved in the chemoresistance of non-small cell lung cancer. Ann Transl Med 2022;10:139. [Crossref] [PubMed]
- Xie H, Yao J, Wang Y, et al. Exosome-transmitted circVMP1 facilitates the progression and cisplatin resistance of non-small cell lung cancer by targeting miR-524-5p-METTL3/SOX2 axis. Drug Deliv 2022;29:1257-71. [Crossref] [PubMed]
- Zhang H, Wang SQ, Wang L, et al. m6A methyltransferase METTL3-induced lncRNA SNHG17 promotes lung adenocarcinoma gefitinib resistance by epigenetically repressing LATS2 expression. Cell Death Dis 2022;13:657. [Crossref] [PubMed]
- Remon J, Steuer CE, Ramalingam SS, et al. Osimertinib and other third-generation EGFR TKI in EGFR-mutant NSCLC patients. Ann Oncol 2018;29:i20-7. [Crossref] [PubMed]
- Passaro A, Jänne PA, Mok T, et al. Overcoming therapy resistance in EGFR-mutant lung cancer. Nat Cancer 2021;2:377-91. [Crossref] [PubMed]
- Girard N. New Strategies and Novel Combinations in EGFR TKI-Resistant Non-small Cell Lung Cancer. Curr Treat Options Oncol 2022;23:1626-44. [Crossref] [PubMed]
- Gao F, Wang Q, Zhang C, et al. RNA methyltransferase METTL3 induces intrinsic resistance to gefitinib by combining with MET to regulate PI3K/AKT pathway in lung adenocarcinoma. J Cell Mol Med 2021;25:2418-25. [Crossref] [PubMed]
- Dai J, Qu T, Yin D, et al. LncRNA LINC00969 promotes acquired gefitinib resistance by epigenetically suppressing of NLRP3 at transcriptional and posttranscriptional levels to inhibit pyroptosis in lung cancer. Cell Death Dis 2023;14:312. [Crossref] [PubMed]
- Liu S, Li Q, Li G, et al. The mechanism of m6A methyltransferase METTL3-mediated autophagy in reversing gefitinib resistance in NSCLC cells by β-elemene. Cell Death Dis 2020;11:969. [Crossref] [PubMed]
- Otano I, Ucero AC, Zugazagoitia J, et al. At the crossroads of immunotherapy for oncogene-addicted subsets of NSCLC. Nat Rev Clin Oncol 2023;20:143-59. [Crossref] [PubMed]
- Desai A, Peters S. Immunotherapy-based combinations in metastatic NSCLC. Cancer Treat Rev 2023;116:102545. [Crossref] [PubMed]
- Chen Y, Gao M, Huang Z, et al. SBRT combined with PD-1/PD-L1 inhibitors in NSCLC treatment: a focus on the mechanisms, advances, and future challenges. J Hematol Oncol 2020;13:105. [Crossref] [PubMed]
- Li X, Ma J, Sun Z, et al. Development and validation of a N6 methylation regulator-related gene signature for prognostic and immune response prediction in non-small cell lung cancer. Am J Cancer Res 2023;13:2984-97. [PubMed]
- Sun Z, Mai H, Xue C, et al. Hsa-LINC02418/mmu-4930573I07Rik regulated by METTL3 dictates anti-PD-L1 immunotherapeutic efficacy via enhancement of Trim21-mediated PD-L1 ubiquitination. J Immunother Cancer 2023;11:e007415. [Crossref] [PubMed]
- Sumitomo R, Hirai T, Fujita M, et al. M2 tumor-associated macrophages promote tumor progression in non-small-cell lung cancer. Exp Ther Med 2019;18:4490-8. [Crossref] [PubMed]
- Li Z, Wang YJ, Zhou J, et al. The prognostic role of M2 tumor-associated macrophages in non-small-cell lung cancer. Histol Histopathol 2022;37:1167-75. [PubMed]
- Wu L, Cheng D, Yang X, et al. M2-TAMs promote immunoresistance in lung adenocarcinoma by enhancing METTL3-mediated m6A methylation. Ann Transl Med 2022;10:1380. [Crossref] [PubMed]
- Yang C, Liang Y, Liu N, et al. Role of the cGAS-STING pathway in radiotherapy for non-small cell lung cancer. Radiat Oncol 2023;18:145. [Crossref] [PubMed]
- D'Andrea MA, Reddy GK. Systemic Immunostimulatory Effects of Radiation Therapy Improves the Outcomes of Patients With Advanced NSCLC Receiving Immunotherapy. Am J Clin Oncol 2020;43:218-28. [Crossref] [PubMed]
- Xu X, Zhang P, Huang Y, et al. METTL3-mediated m6A mRNA contributes to the resistance of carbon-ion radiotherapy in non-small-cell lung cancer. Cancer Sci 2023;114:105-14. [Crossref] [PubMed]
- Zhang K, Han Z, Zhao H, et al. An integrated model of FTO and METTL3 expression that predicts prognosis in lung squamous cell carcinoma patients. Ann Transl Med 2021;9:1523. [Crossref] [PubMed]
- Li X, Li N, Huang L, et al. Is Hydrogen Sulfide a Concern During Treatment of Lung Adenocarcinoma With Ammonium Tetrathiomolybdate? Front Oncol 2020;10:234. [Crossref] [PubMed]
- Xiao H, Zhao R, Meng W, et al. Effects and translatomics characteristics of a small-molecule inhibitor of METTL3 against non-small cell lung cancer. J Pharm Anal 2023;13:625-39. [Crossref] [PubMed]
- Yu H, Liu J, Bu X, et al. Targeting METTL3 reprograms the tumor microenvironment to improve cancer immunotherapy. Cell Chem Biol 2024;31:776-791.e7. [Crossref] [PubMed]
- Du M, Zhang Y, Mao Y, et al. MiR-33a suppresses proliferation of NSCLC cells via targeting METTL3 mRNA. Biochem Biophys Res Commun 2017;482:582-9. [Crossref] [PubMed]
- Wei W, Huo B, Shi X. miR-600 inhibits lung cancer via downregulating the expression of METTL3. Cancer Manag Res 2019;11:1177-87. [Crossref] [PubMed]
- Huang S, Luo S, Gong C, et al. MTTL3 upregulates microRNA-1246 to promote occurrence and progression of NSCLC via targeting paternally expressed gene 3. Mol Ther Nucleic Acids 2021;24:542-53. [Crossref] [PubMed]
- Chen WW, Qi JW, Hang Y, et al. Simvastatin is beneficial to lung cancer progression by inducing METTL3-induced m6A modification on EZH2 mRNA. Eur Rev Med Pharmacol Sci 2020;24:4263-70. [PubMed]
- Chen CJ, Huang JY, Huang JQ, et al. Metformin attenuates multiple myeloma cell proliferation and encourages apoptosis by suppressing METTL3-mediated m6A methylation of THRAP3, RBM25, and USP4. Cell Cycle 2023;22:986-1004. [Crossref] [PubMed]
- Feng Y, Li C, Liu S, et al. β-Elemene Restrains PTEN mRNA Degradation to Restrain the Growth of Lung Cancer Cells via METTL3-Mediated N6 Methyladenosine Modification. J Oncol 2022;2022:3472745. [Crossref] [PubMed]
- Cao Y, Di X, Cong S, et al. RBM10 recruits METTL3 to induce N6-methyladenosine-MALAT1-dependent modification, inhibiting the invasion and migration of NSCLC. Life Sci 2023;315:121359. [Crossref] [PubMed]
- Pan H, Pan Z, Guo F, et al. MicroRNA-1915-3p inhibits cell migration and invasion by targeting SET in non-small-cell lung cancer. BMC Cancer 2021;21:1218. [Crossref] [PubMed]
- Jiao Y, Xu Y, Liu C, et al. The role of ADAR1 through and beyond its editing activity in cancer. Cell Commun Signal 2024;22:42. [Crossref] [PubMed]