Diagnosis and treatment of non-small cell lung cancer (NSCLC) harboring MET Ex14 skipping: have we met the desired drug?
In 2006, the MET proto-oncogene, receptor tyrosine kinase (MET) exon 14 skipping mutation (METex14 skipping) was reported as a new driver gene abnormality in approximately 3% of patients with non-small cell lung cancer (NSCLC) (1). This mutation affects both sexes equally and is present in young non-smokers, elderly individuals, and smokers. Furthermore, it also has been identified in 8–17% of sarcomatoid carcinoma (2-4). In March 2020, the MET inhibitor tepotinib was approved in Japan, and capmatinib was approved in June of the same year. The U.S. Food and Drug Administration (FDA) approved capmatinib in May 2020 and tepotinib in February 2021 (5). Therefore, detection of this mutation is crucial for all patients with advanced NSCLC. This review provides an overview of MET and MET receptor tyrosine kinases, the results of pivotal clinical trials (VISION and GEOMETRY mono-1 trials) on MET-tyrosine kinase inhibitors (Table 1), and the testing systems used for the genetic diagnosis of METex14 skipping.
Table 1
Study (phase) | Drug | MET detection | Treatment line | N | ORR, % [95% CI] |
mDOR, months [95% CI] |
mPFS, months [95% CI] |
---|---|---|---|---|---|---|---|
VISION (phase II) | Tepotinib 500 mg/day |
Ex14, tissue | ≥1st line | 60 | 50 [37–63] | 15.7 [9.7–NR] | 11.0 [5.7–17.1] |
Ex14, liquid | 66 | 48 [36–61] | 9.9 [7.2–NR] | 8.5 [5.1–11.0] | |||
Ex14, combined | 99 | 46 [36–57] | 11.1 [7.2–NR] | 8.5 [6.7–11.0] | |||
VISION (phase II), update data | Tepotinib 500 mg/day |
Ex14, tissue | ≥1st line (overall) | 208 | 54 [47–61] | 18.0 [10.8–46.4] | 13.7 [11.0–17.1] |
Ex14, liquid | 178 | 52 [44–59] | 15.2 [9.7–33.6] | 8.9 [7.8–11.0] | |||
Ex14, combined | 313 | 51 [46–57] | 18.0 [12.4–46.4] | 11.2 [9.5–13.8] | |||
Ex14, tissue | 1st line | 111 | 59 [49–68] | 46.4 [15.2–NR] | 15.9 [11.0–49.7] | ||
Ex14, liquid | 95 | 59 [48–69] | 19.4 [8.3–NR] | 10.3 [8.0–16.5] | |||
Ex14, combined | 164 | 57 [49–65] | 46.4 [13.8–NR] | 12.6 [9.7–17.7] | |||
Ex14, tissue | ≥2nd line | 97 | 50 [39–60] | 12.4 [8.3–18.0] | 11.5 [8.2–14.7] | ||
Ex14, liquid | 83 | 43 [33–55] | 12.4 [8.4–33.6] | 8.2 [5.7–11.0] | |||
Ex14, combined | 149 | 45 [37–53] | 12.6 [9.5–18.5] | 11.0 [8.2–13.7] | |||
GEOMETRY mono-1 (phase II) |
Capmatinib 800 mg/day | Ex14 (cohort 5b) | 1st line | 28 | 68 [48–84] | 12.6 [5.6–NR] | 12.4 [8.2–NR] |
Ex14 (cohort 4) | ≥2nd line | 69 | 41 [29–53] | 9.7 [5.6–13.0] | 5.4 [4.2–7.0] |
NSCLC, non-small cell lung cancer; ORR, objective response rate; mDOR, median duration of response; mPFS, median progression-free survival; CI, confidence interval; NR, not reached.
MET is a proto-oncogene located on chromosome 7q21-31, consisting of 21 exons. Transcription of this gene produces MET receptor tyrosine kinase, which is composed of 1,390 amino acids (NM_000245.2, variant 2). The extracellular portion of the MET receptor comprises of three domains: semaphorin (SEMA), plexin-semaphorin-integrin (PSI), and four immunoglobulin-plexin-transcription (IPT) repeats. The intracellular portion consists of a transmembrane domain (TM), juxtamembrane (JM) domain, tyrosine kinase domain, and a multifunctional docking site (MFDS) at the carboxyl-terminal (Figure 1). This receptor binds to hepatocyte growth factor (HGF) as a ligand, activating downstream signaling pathways that promote cell proliferation, anti-apoptosis, and migration. These pathways contribute to cancer progression, metastasis, and drug resistance (6,7). The METex14 skipping mutation results in loss of the JM domain of the MET receptor. This domain is essential for binding to E3 ubiquitin ligase casitas B-lineage lymphoma (CBL), leading to the ubiquitination and degradation of the MET receptor protein (3,6,7). Therefore, this domain regulates MET receptor turnover by interacting with CBL at ligand-dependent sites (Figure 1).
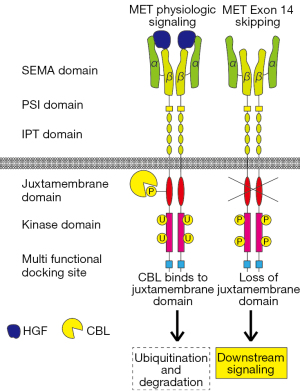
Exon 14 of MET encodes amino acids 963-1,010 of the MET protein, which comprises the JM domain (NM_000245.2, variant 2). Therefore, complete loss of METex14 and base substitutions or indels (insertions/deletions) in the splicing acceptor/donor sites of introns flanking METex14 can occur. In such cases, ubiquitination and degradation of the MET receptor are inhibited, leading to enhanced and sustained activation of MET signaling and tumorigenicity (Figure 1). In addition, mutations in MET Y1003, which is critical for CBL binding (Y1003F/N/S/C), exhibit molecular and physiological effects equivalent to METex14 skipping. A point mutation in the last nucleotide of METex14 (c.3028, D1010X, variant 2) also causes this. Therefore, skipping of exon 14 may result from various genetic abnormalities (2,3,6).
METex14 skipping often co-occurs with MET amplification, suggesting a correlation with high MET protein expression. However, immunohistochemistry (IHC) may not adequately detect METex14 skipping, making it an unsuitable screening method (8). Some reports indicate a high frequency of programmed death-ligand 1 (PD-L1) high expression (≥50%) in lung cancer with METex14 skipping (9,10). Nevertheless, there is no consensus regarding the responsiveness of lung cancer patients with METex14 skipping mutations to immune checkpoint inhibitors.
The VISION trial was an international, collaborative, non-blinded, single-arm phase II study (11). This study aimed to evaluate the antitumor effects and tolerability of tepotinib as 1st to 3rd-line treatment in patients with advanced or recurrent NSCLC who tested positive for METex14 skipping and had an Eastern Cooperative Oncology Group Performance Status (ECOG-PS) of 0–1.
In total, 152 patients identified as METex14 skipping-positive using tumor tissue or plasma samples were enrolled. METex14 skipping was detected in tissue samples using the Oncomine Focus Assay, whereas plasma samples were analyzed using Guardant360. Tepotinib was administered orally at a dose of 500 mg daily. The primary endpoint, objective response rate (ORR), was 46% [95% confidence interval (CI): 36–57], and the median duration of response (mDOR) was 11.1 months (95% CI: 7.2–not reached). The median progression-free survival (mPFS) was 8.5 months (95% CI: 6.7–11.0), and the median overall survival (mOS) was 17.1 months (95% CI: 12.0–26.8).
Furthermore, the response rate and response mechanisms of the plasma-positive subgroup were comparable to those of the tumor tissue-positive subgroup, demonstrating the utility of METex14 skipping diagnosis using plasma samples. The main adverse events included peripheral edema, nausea, and diarrhea, with severe adverse events observed in 15% of cases. Most adverse events are manageable with supportive measures, dose reduction, or treatment interruption (12).
Mazieres et al. reported additional long-term follow-up data from the VISION trial (13), examining tepotinib efficacy in two cohorts (A and C) with different enrollment periods in the VISION trial. In this additional VISION trial report, tepotinib efficacy in METex14-positive cases in Cohort C (enrolled from August 2019 to May 2021) is published, along with the previously reported long-term follow-up data of cohort A (enrolled from September 2016 to December 2019). In the integrated analysis of these two cohorts, 164 treatment-naive and 149 previously treated patients were enrolled. In treatment-naive patients, ORR was 57.3% (95% CI: 49.4–65.0%), mDOR was 46.4 months (95% CI: 13.8–undetermined), mPFS was 12.6 months (95% CI: 9.7–17.7), and mOS was 21.3 months (95% CI: 14.2–25.9). In previously treated patients, ORR was 45.0% (95% CI: 36.8–53.3), mDOR was 12.6 months (95% CI: 9.5–18.5), mPFS was 11.0 months (95% CI: 8.2–13.7), and mOS was 19.3 months (95% CI: 15.6–22.3), demonstrating consistent efficacy, even though it was inferior to treatment-naive patients. Of 164 treatment-naive patients, 111 tested positive on tissue biopsy. In these patients, ORR was 58.6% (95% CI: 48.8–67.8%), mDOR was 46.4 months (95% CI: 15.2–undetermined), mPFS was 15.9 months (95% CI: 11.0–49.7), and mOS was 29.7 months (95% CI: 18.8–undetermined). On the other hand, among 95 liquid biopsy-positive patients out of 164, ORR was 58.9% (95% CI: 48.4–68.9%), mDOR was 19.4 months (95% CI: 8.3–undetermined), mPFS was 10.3 months (95% CI: 8.0–16.5), and mOS was 17.6 months (95% CI: 10.4–23.7), suggesting a potentially lower treatment effect in liquid biopsy-positive compared to tissue biopsy-positive patients. Although liquid biopsy is less invasive than tissue biopsy, it has limited sensitivity for low ctDNA-shedding tumors and low tumor burden (14). Therefore, the authors noted that patients with worse prognoses might have been selected because of a higher tumor burden and/or ctDNA shedding in the liquid biopsy cohort.
The GEOMETRY mono-1 trial was an international, collaborative, non-blinded, single-arm, Phase II trial conducted to evaluate the antitumor efficacy, tolerability, and safety of capmatinib (15). In this trial, patients with advanced or recurrent NSCLC diagnosed as METex14 skipping-positive by reverse transcription-polymerase chain reaction (RT-PCR) of the tumor tissue samples were enrolled. The patients received 400 mg of capmatinib orally twice daily. Results from the analysis of cohorts targeting initial treatment cases (28 cases) and cases of second- and third-line treatments (69 cases) were reported. The primary endpoint, ORR, was 67.9% (95% CI: 47.6–84.1%) in the initial treatment group and 40.6% (95% CI: 28.9–53.1%) in the second- and third-line treatment groups. The mDOR and mPFS were 12.6 months (95% CI: 5.6–not reached) and 12.4 months (95% CI: 8.2–not reached) in the initial treatment group, and 9.7 months (95% CI: 5.6–13.0) and 5.4 months (95% CI: 4.2–7.0) in the second and third-line treatment group, respectively, demonstrating superior results in the initial treatment group.
In both cohorts, 13 patients exhibited intracranial lesions, with seven (54%) showing a response. Disease control was achieved in 12 (91%) patients, suggesting that capmatinib was effective against central nervous system metastases. The main adverse events included peripheral edema, nausea, increased blood creatinine levels, vomiting, and diarrhea. Serious adverse events were observed in approximately 15% of the cases.
As of January 2024, the detection of METex14 skipping mutations involves three companion diagnostic tools in Japan: (I) AmoyDx Pan Lung Cancer PCR Panel (tissue) for tepotinib, (II) FoundationOne CDx (tissue/plasma) for capmatinib, and (III) Lung Cancer Compact Panel (tissue/cytology) for tepotinib. ArcherMET (tissue/plasma) was also approved as a companion diagnostic tool for METex14 skipping before 2023. However, this was discontinued in May of 2023. In the United States, FoundationOne CDx and FoundationOne Liquid CDx have been approved as companion diagnostic tools for capmatinib. However, no companion diagnostic tools are currently available for tepotinib treatment. These companion diagnostics are essential tools in the clinical setting and offer reliable methods for accurately diagnosing METex14 skipping mutations. Clinicians must select an appropriate diagnostic approach based on the type of patient sample available and the specific diagnostic requirements of an individual case.
AmoyDx Pan Lung Cancer PCR Panel is a multiplex gene panel which covers seven driver genes (EGFR, ALK, ROS1, RET, BRAF, MET, and KRAS) in NSCLC. In August 2021, it was approved as a companion diagnostic tool for METex14 skipping in combination with tepotinib. The panel was designed to test formalin-fixed paraffin-embedded (FFPE) or fresh-frozen tissues using real-time PCR, including RT-PCR.
METex14 skipping was detected by RT-PCR, using tumor-extracted RNA as a template. The recommended RNA concentrations for testing are 10–100 ng/µL for FFPE tissues and 2–30 ng/µL for fresh-frozen tissues, with a minimum mutation detection sensitivity of 25 copies/µL.
Performance testing using 127 samples confirmed for METex14 skipping in the VISION trial showed a high overall concordance rate of 98.4% compared with next-generation sequencing (NGS). The panel demonstrated 100% positive and 97.7% negative concordance, indicating excellent performance.
AmoyDx Pan Lung Cancer PCR Panel, which has high sensitivity and a short turnaround time of approximately seven days owing to the characteristics of real-time PCR, is valuable, especially in cases of NSCLC, where prompt determination of treatment strategies is crucial. We previously reported a case of lung adenocarcinoma harboring METex14 skipping with lung abscess formation that was successfully and promptly diagnosed using this panel and treated with tepotinib (16). However, based on the results of this panel, tepotinib was the only MET inhibitor applicable.
FoundationOne CDx is an NGS assay system that uses a hybrid capture method. It comprehensively analyzes 324 cancer-related genes using tumor tissue DNA for substitutions, insertions, deletions, copy number abnormalities, fusion genes, and gene rearrangements. Additionally, it calculates other biomarkers, such as microsatellite instability (MSI) and tumor mutation burden (TMB). In the GEOMETRY mono-1 trial, patient selection was performed using RT-PCR at a central measuring institution (15). FoundationOne was used for the exploratory analysis, and the concordance rate with RT-PCR for METex14 skipping was 99%, demonstrating analytical equivalence. It was approved in Japan in May 2020 as a companion diagnostic test for capmatinib in patients with advanced or recurrent NSCLC harboring METex14 skipping mutations.
In Japan, in the typical flow of NSCLC diagnosis, the FoundationOne CDx is performed as a CGP when the standard treatment (second-line treatment) is expected to end. However, the efficacy of capmatinib, which can be used based on the results of Foundation One CDx, was shown to be better in untreated cases than in previously treated cases in the GEOMETRY mono-1 trial (15,17). Therefore, the organization of companion diagnostics and their integration into insurance treatments are eagerly awaited for standard NSCLC treatment in the future.
The Lung Cancer Compact Panel was introduced in February 2023 and has exhibited a high success rate in genetic analyses, even for cellular specimens. This panel presents a novel, accurate, and versatile NGS method. Notably, it can be used to analyze cell suspensions, needle wash fluids, and liquid samples, such as pleural and pericardial effusion, confirming the presence of malignant cells in paired samples. Furthermore, a high concordance rate (94.9%) was reported in bronchoscopic biopsy forceps-washed samples compared with tissue specimens derived from the primary tumor (18).
A distinctive feature is the modular processing of target genes. The detection sensitivity was exceptionally high, and sequencing was conducted with sufficient depth. Amplicon optimization was achieved by narrowing down the targets, enabling the detection of mutations, even in samples with advanced decomposition due to necrosis or prolonged storage. Ten ng or more of DNA and RNA was required for analysis. Accurate measurements were ensured when the percentage of cancer cells in the tissue was 2% or higher (allelic frequency of 1% or higher), which is a significant improvement over conventional gene multiplex assays (typically, 5–10% or higher).
The development of GeneMetrix Nucleic Acid Preservation Solution Containers (GM tubes) with excellent nucleic acid preservation properties allows the direct submission or placement of liquid samples in GM tubes after distant centrifugation for subsequent analysis. The GM tube is a nucleic acid-stabilizing solution containing ammonium sulfate as the main component. Two milliliters of the solution are filled into a 5 mL container tube, contributing to exceptional practicality. Implementing modular multiplex PCR at the module level provides a straightforward evaluation of the clinical validity of companion diagnostics because the conditions are fixed for each module to demonstrate analytical performance (19). In addition, the ability to add new modules and evaluate their performance separately from existing modules facilitates the measurement of novel genetic abnormalities.
Table 2 compares the three METex14 skipping detection tests: AmoyDx Pan Lung Cancer PCR Panel, FoundationOne CDx, and Lung Cancer Compact Panel. AmoyDx includes multiple genes, such as EGFR, ALK, and MET. It uses RT-PCR on RNA from FFPE and fresh frozen samples, offering a quick turnaround time of seven days; however, it is not compatible with capmatinib. FoundationOne CDx covers 324 cancer-related genes, employs NGS technology on DNA from FFPE and plasma samples, and calculates MSI and TMB. However, this method is costly and requires 2–3 weeks of processing time. It was approved for use in conjunction with capmatinib therapy in May 2020. Finally, the Lung Cancer Compact Panel covers a similar range of genes as AmoyDx. It can use cytology samples with RNA and DNA as bases and tepotinib as its companion drug, with approval in Japan as of February 2023.
Table 2
MET detection tests | AmoyDx Pan Lung Cancer PCR Panel | FoundationOne CDx (tissue/plasma) |
Lung Cancer Compact Panel (tissue/cytology) |
---|---|---|---|
Cover genes | EGFR, ALK, ROS1, RET, BRAF, MET, KRAS | 324 cancer-related genes | EGFR, ALK, ROS1, RET, BRAF, MET, KRAS |
Companion drugs | Tepotinib | Capmatinib | Tepotinib |
Detection methods | RT-PCR | NGS | NGS |
DNA or RNA | RNA | DNA | RNA |
Sample types | FFPE, FF | FFPE, plasma | FFPE, cytology |
Advantages | Short turnaround time (7 days) | MSI, TMB can be calculated | Cytology sample can be used |
Disadvantages | Not applicable for campatinib | Long turnaround time (2–3 weeks), expensive | Not applicable for campatinib |
Approval date (in Japan) | August 2021 | May 2020 | February 2023 |
EGFR, epidermal growth factor receptor; ALK, anaplastic lymphoma kinase; ROS1, c-ros oncogene 1; RET, RET proto-oncogene; BRAF, B-Raf proto-oncogene; MET, MET proto-oncogene, receptor tyrosine kinase; KRAS, KRAS proto-oncogene; RT-PCR, reverse transcription-polymerase chain reaction; NGS, next generation sequencing; FFPE, formalin-fixed paraffin-embedded; FF, fresh-frozen; MSI, microsatellite instability; TMB, tumor mutation burden.
Multiplex testing is becoming standard practice, and the current choices for companion diagnostics as first-line treatment are multiplex AmoyDx or the Lung Cancer Compact Panel. Oncomine Dx TT may be the next candidate as a standard test for comprehensive lung cancer diagnosis. However, once the environment surrounding companion diagnostics is developed, clinicians must be well-versed in the nuances of each testing system.
Acknowledgments
Funding: None.
Footnote
Provenance and Peer Review: This article was commissioned by the editorial office, Translational Lung Cancer Research. The article has undergone external peer review.
Peer Review File: Available at https://tlcr.amegroups.com/article/view/10.21037/tlcr-24-93/prf
Conflicts of Interest: The author has completed the ICMJE uniform disclosure form (available at https://tlcr.amegroups.com/article/view/10.21037/tlcr-24-93/coif). The author has no conflicts of interest to declare.
Ethical Statement: The author is accountable for all aspects of the work in ensuring that questions related to the accuracy or integrity of any part of the work are appropriately investigated and resolved.
Open Access Statement: This is an Open Access article distributed in accordance with the Creative Commons Attribution-NonCommercial-NoDerivs 4.0 International License (CC BY-NC-ND 4.0), which permits the non-commercial replication and distribution of the article with the strict proviso that no changes or edits are made and the original work is properly cited (including links to both the formal publication through the relevant DOI and the license). See: https://creativecommons.org/licenses/by-nc-nd/4.0/.
References
- Kong-Beltran M, Seshagiri S, Zha J, et al. Somatic mutations lead to an oncogenic deletion of met in lung cancer. Cancer Res 2006;66:283-9. [Crossref] [PubMed]
- Awad MM, Oxnard GR, Jackman DM, et al. MET Exon 14 Mutations in Non-Small-Cell Lung Cancer Are Associated With Advanced Age and Stage-Dependent MET Genomic Amplification and c-Met Overexpression. J Clin Oncol 2016;34:721-30. [Crossref] [PubMed]
- Frampton GM, Ali SM, Rosenzweig M, et al. Activation of MET via diverse exon 14 splicing alterations occurs in multiple tumor types and confers clinical sensitivity to MET inhibitors. Cancer Discov 2015;5:850-9. [Crossref] [PubMed]
- Mazieres J, Vioix H, Pfeiffer BM, et al. MET Exon 14 Skipping in NSCLC: A Systematic Literature Review of Epidemiology, Clinical Characteristics, and Outcomes. Clin Lung Cancer 2023;24:483-97. [Crossref] [PubMed]
- Mathieu LN, Larkins E, Akinboro O, et al. FDA Approval Summary: Capmatinib and Tepotinib for the Treatment of Metastatic NSCLC Harboring MET Exon 14 Skipping Mutations or Alterations. Clin Cancer Res 2022;28:249-54. [Crossref] [PubMed]
- Recondo G, Che J, Jänne PA, et al. Targeting MET Dysregulation in Cancer. Cancer Discov 2020;10:922-34. [Crossref] [PubMed]
- Petrini I. Biology of MET: a double life between normal tissue repair and tumor progression. Ann Transl Med 2015;3:82. [PubMed]
- Guo R, Berry LD, Aisner DL, et al. MET IHC Is a Poor Screen for MET Amplification or MET Exon 14 Mutations in Lung Adenocarcinomas: Data from a Tri-Institutional Cohort of the Lung Cancer Mutation Consortium. J Thorac Oncol 2019;14:1666-71. [Crossref] [PubMed]
- Xu Z, Li H, Dong Y, et al. Incidence and PD-L1 Expression of MET 14 Skipping in Chinese Population: A Non-Selective NSCLC Cohort Study Using RNA-Based Sequencing. Onco Targets Ther 2020;13:6245-53. [Crossref] [PubMed]
- Guo J, Yuan H, Zhu Y, et al. PD-L1 expression and its correlation with clinicopathological and molecular characteristics in Chinese patients with non-small cell lung cancer. Medicine (Baltimore) 2024;103:e36770. [Crossref] [PubMed]
- Paik PK, Felip E, Veillon R, et al. Tepotinib in Non-Small-Cell Lung Cancer with MET Exon 14 Skipping Mutations. N Engl J Med 2020;383:931-43. [Crossref] [PubMed]
- Veillon R, Sakai H, Le X, et al. Safety of Tepotinib in Patients With MET Exon 14 Skipping NSCLC and Recommendations for Management. Clin Lung Cancer 2022;23:320-32. [Crossref] [PubMed]
- Mazieres J, Paik PK, Garassino MC, et al. Tepotinib Treatment in Patients With MET Exon 14-Skipping Non-Small Cell Lung Cancer: Long-term Follow-up of the VISION Phase 2 Nonrandomized Clinical Trial. JAMA Oncol 2023;9:1260-6. [Crossref] [PubMed]
- Rolfo C, Mack P, Scagliotti GV, et al. Liquid Biopsy for Advanced NSCLC: A Consensus Statement From the International Association for the Study of Lung Cancer. J Thorac Oncol 2021;16:1647-62. [Crossref] [PubMed]
- Wolf J, Seto T, Han JY, et al. Capmatinib in MET Exon 14-Mutated or MET-Amplified Non-Small-Cell Lung Cancer. N Engl J Med 2020;383:944-57. [Crossref] [PubMed]
- Makimoto G, Shimonishi A, Ohashi K, et al. Successful and Prompt Treatment with Tepotinib for Lung Adenocarcinoma Harboring MET Exon 14 Skipping Mutation Combined with Lung Abscess Formation: A Case Report. Case Rep Oncol 2022;15:494-8. [Crossref] [PubMed]
- Wu YL, Smit EF, Bauer TM. Capmatinib for patients with non-small cell lung cancer with MET exon 14 skipping mutations: A review of preclinical and clinical studies. Cancer Treat Rev 2021;95:102173. [Crossref] [PubMed]
- Kunimasa K, Tamiya M, Inoue T, et al. Clinical application of the Lung Cancer Compact Panel(TM) using various types of cytological specimens in patients with lung cancer. Lung Cancer 2024;189:107498. [Crossref] [PubMed]
- Morikawa K, Kida H, Handa H, et al. A Prospective Validation Study of Lung Cancer Gene Panel Testing Using Cytological Specimens. Cancers (Basel) 2022;14:3784. [Crossref] [PubMed]