Association between strenuous sports or other exercises and lung cancer risk: a mendelian randomization study
Highlight box
Key findings
• The study findings indicate that the total odds ratio (OR) for lung cancer from strenuous sports or other exercises (SSOE) was estimated at 0.129 [95% confidence interval (CI): 0.021–00.779], suggesting a significant protective effect. Furthermore, the combined OR for lung cancer from SSOE was estimated at 0.054 (95% CI: 0.01–0.302), indicating a significant inverse relationship.
What is known and what is new?
• Voluntary exercise has been shown in various rodent models to boost immune cells in tumors and significantly reduce tumorigenesis and progression.
• Studying the relationship between SSOE and lung cancer risk remains underexplored.
What is the implication, and what should change now?
• SSOE is beneficial in reducing the risk of lung cancer and helping to develop individualized exercise treatment plans for cancer patients to optimize their quality of life and treatment outcomes.
Introduction
Lung cancer is a global and common aggressive disease that affects people all over the world. Although morbidity and mortality rates vary greatly between men and women in different parts of the world, lung cancer is the cancer that causes the most deaths in men and the second most deaths in women. Men are about twice as likely as women to develop and die from lung cancer (1). Despite recent therapeutic advancements, lung cancer remains a major public health issue in the United States and globally (2). With a 5-year survival rate of only 19% (3), the identification of preventable and modifiable factors that effectively mitigate the risk of lung cancer is of paramount importance.
Consumption of fruit and vegetables, breakfast cereals, and dietary fiber have been inversely associated with the risk of lung cancer (4). In contrast, there are numerous factors that are positively associated with the risk of lung cancer, including occupational exposures, smoking, and radiation exposure, to mention a few. Smoking is a widely recognized catalyst for lung cancer, profoundly impacting individual susceptibility. Extensive studies since the 1950s have unequivocally demonstrated the carcinogenic effects of tobacco smoke on lung health (5). Persistent smokers face a 20- to 50-fold greater risk of developing lung cancer compared to nonsmokers. The duration of smoking plays a pivotal role in determining this risk (6-9). Furthermore, exercise has been suggested as a potential preventive factor against lung cancer (5). Potential biological factors may underlie the effectiveness of exercise in the prevention and treatment of lung cancer. These mechanisms include promoting p53-mediated apoptosis, supporting immune cell infiltration, suppressing lung cancer cell growth and survival, improving the tumor microenvironment, reducing chronic inflammation, turning on DNA repair enzymes, and reducing oxidative stress (7-16).
Recent studies have demonstrated that voluntary exercise can increase immune cells in tumors and significantly reduce tumor incidence and development across multiple rodent models (13). It is hypothesized that this relationship between exercise and the immune system could be leveraged in cancer treatment, particularly in conjunction with immunotherapy, given the similarity in immune cell activation processes during exercise in both rodents and humans (17). In addition, exercise is often employed as a therapy for lung cancer (18).
However, the associations between these factors remain uncertain due to inconsistent findings and potential biases such as residual confounding, misclassification, and reverse causality.
Mendelian randomization (MR) (Figure 1) design can enhance causal inference by utilizing genetic variants as instrumental variables or proxies for exposures (19), such as exercise (20). As genetic variants are randomly distributed at conception, confounding is minimized, and one trait is typically unrelated to other traits. Moreover, genetic variants are not influenced by the onset or progression of the disease, thereby reducing the risk of reverse causation bias (21). Herein, we employed MR design to explore whether strenuous sports or other exercises (SSOE) are associated with a protective effect against lung cancer (22). To increase accuracy and lower potential bias and confounders, our study concentrated more on intense exercise, used a larger dataset, and employed the most recent genetic methods for physical activity features. We also removed a number of factors. What sets us apart from other studies (23) is the unique methodology we employ to enhance the validity and trustworthiness of our results. We present this article in accordance with the STROBE-MR reporting checklist (available at https://tlcr.amegroups.com/article/view/10.21037/tlcr-23-810/rc) (24).
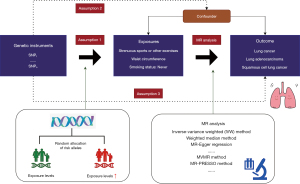
Methods
Study design
MR refers to a methodological approach in epidemiology and genetics that utilizes genetic variants as instrumental variables to investigate causal relationships between an exposure and an outcome. This approach leverages the random assortment of genetic alleles during meiosis, similar to Mendel’s laws of inheritance, to provide unbiased estimates of causal effects in observational studies. Essentially, it mimics the random assignment of individuals to different levels of exposure in a randomized controlled trial, thereby reducing the risk of confounding and reverse causation biases commonly encountered in observational studies. The research design of the MR study investigating the relationship between smoke exposure (SSOE) and lung cancer is illustrated in Figure 1. The study sought to ascertain the correlation between SSOE and lung cancer, encompassing distinct subtypes such as lung adenocarcinoma and squamous cell lung cancer. It accounted for etiological heterogeneity stemming from cancer pathologic subtypes or clinical characteristics. To enhance analytical capability, we merged estimates from various data sources and introduced two additional variables, waist circumference (25) and smoking status, to adjust for the impact of SSOE. The study employed both mediation analysis and the multivariable MR approach. We utilized summary-level data from published genome-wide association studies (GWASs) for our analysis. The study was conducted in accordance with the Declaration of Helsinki (as revised in 2013) (26).
Genetic instrument selection
Two-sample MR
We identified single-nucleotide polymorphisms (SNPs) linked to SSOE (22) at the genome-wide significance level (P≤5×10−8) from corresponding GWASs. Linkage disequilibrium among these SNPs was assessed using the 1000 genomes linkage disequilibrium European panel as reference (27). In this MR investigation, 12 independent SNPs with the most significant P values for SSOE were chosen as genetic instruments, while excluding SNPs in moderate linkage disequilibrium (r2>0.01 and clump window <10,000 kb).
Multivariable MR
SNPs associated with SSOE, waist circumference, and smoking status: never at the genome-wide significance level (P≤5×10−8) were obtained from corresponding GWASs. Linkage disequilibrium among these SNPs for each exposure was estimated utilizing the PLINK clumping method, within the context of the European population as defined by the 1000 Genomes reference panel (27). We excluded SNPs with linkage disequilibrium (r2>0.01 and clump window <10,000 kb) and retained the SNP with the lowest P value. In this MR study, we applied 8, 17, and 57 SNPs associated with SSOE, waist circumference, and smoking status: never, respectively, as genetic instruments.
Data sources for lung cancer, squamous cell lung cancer, and lung adenocarcinoma
We extracted summary-level data for lung cancer, squamous cell lung cancer, and lung adenocarcinoma from GWASs for the associations of exposure-associated SNPs, including 11,348 lung cancer cases and 15,861 non-cases (controls) in lung cancer, 3,275 squamous cell lung cancer cases, and 15,038 non-cases (controls) in squamous cell lung cancer, and 3,442 lung adenocarcinoma cases and 14,894 non-cases (controls) in squamous cell lung cancer (28). Age, sex, and major genetic principal components were adjusted for association tests.
Physical activity data selection
Information on physical activity for the experimental component was collected through self-report questionnaires and objective assessments. Self-report surveys measure the frequency, duration, and intensity of various types of exercise through interviews or self-administered questionnaires. In addition, subjects are assessed objectively over several days or weeks using an accelerometer or wearable activity monitor, a machine that monitors daily activity patterns and provides objective measurements such as step counts, moderate-to-vigorous physical activity (MVPA) duration, and sedentary behavior. We classified intense exercise using recognized intensity and duration criteria. Strenuous activities involved a considerable increase in heart rate and respiratory rate. Running, swimming, cycling, and other strenuous activities were given as examples. We also assessed the duration of activities, with a minimum of 30 minutes of continuous high-intensity activity. This ensured that only continuous vigorous activity was classified as demanding exercise. Furthermore, we recognized that different types of physical activity contribute to total activity levels but may not match the requirements for high intensity. This category included moderate-intensity activities such as brisk strolling, recreational sports, and moderate aerobic exercise.
Statistical analysis
Two-sample MR
Inverse variance-weighting (IVW) is a statistical method commonly used in meta-analysis and MR studies. In the context of MR, IVW is used to combine individual genetic variant-exposure and genetic variant-outcome associations into a single estimate of the causal effect. For the two-sample MR analysis, we employed the IVW method with random effects as our main analysis, as it has been widely used and has good performance (29). We also conducted supplementary analyses using other methods, including the weighted median method (30), MR-Egger regression (31), the simple mode, and the weighted mode. Furthermore, we conducted a leave-one-out analysis by omitting a single SNP at a time to examine whether the MR outcome was influenced or biased by any single SNP. Additionally, we performed pleiotropy and heterogeneity tests to assess the validity of our results.
Multivariable MR
Regarding the multivariable MR analysis, we employed the IVW method with random effects, MR-Egger regression, the multivariable median method, and the MR pleiotropy residual sum and outlier (MR-PRESSO) model. The weighted median method was also utilized to provide consistent estimates, assuming that more than 50% of the weight comes from valid SNPs (30). In instances where the intercept test identified significant horizontal pleiotropy (P for intercept <0.05), the MR-Egger analysis was conducted to produce pleiotropy-corrected estimates, although this model is often characterized by limited statistical power (31). The multivariable median method, on the other hand, was similar to the univariable weighted median method but was implemented using quantile regression. The regression model was multivariable and weighted by the inverse of the variances of the variant-specific estimates. Finally, the MR-PRESSO approach was employed to detect any possible outliers and to generate estimates after their removal, and its embedded distortion test could distinguish the differences between estimates before and after outlier removal (32). Horizontal pleiotropy was evaluated using a modified form of Cochran’s Q statistic, which assessed differences in MR estimates across the set of instruments. All analyses were conducted using the TwoSampleMR (33) and MR-PRESSO (32) packages in R software (version 4.2.2).
Results
Two-sample MR
Our two-sample MR analysis revealed that genetic predisposition to SSOE is inversely associated with lung cancer risk. The total OR for lung cancer from SSOE was estimated at 0.129 [95% confidence interval (CI): 0.021–0.779, P=0.03], indicating a significant protective effect. To further validate the robustness of our findings, we conducted several supplementary analyses, including the weighted median method and MR-Egger regression. The weighted median analysis generated an OR estimate of 0.281 (95% CI: 0.033–2.377; P=0.24), which was consistent with the IVW method. MR-Egger regression analysis detected a non-significant causal estimate with an OR of 3.471 (95% CI: 0.001–1.24×104; P=0.77), which suggests the absence of pleiotropy bias. When we compared the association of SSOE with different lung cancer subtypes, we observed a stronger inverse relationship with squamous cell lung cancer (OR =0.045; 95% CI: 0.003–0.677; P=0.03) than with lung adenocarcinoma (OR =0.161; 95% CI: 0.012–2.102; P=0.16) (Figures 2,3, Tables 1,2). Sensitivity analysis by omitting individual SNPs reveal that no influence on the causal effect of SSOE on lung cancer, squamous cell lung cancer, or lung adenocarcinoma (Figure 3). Additionally, the heterogeneity and pleiotropy tests did not indicate any significant impact of horizontal pleiotropy or heterogeneity (P=0.44 and P=0.11, respectively), suggesting that the effect of SSOE on lung cancer was mainly through a single pathway (Table 3).
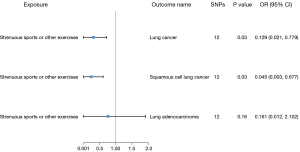
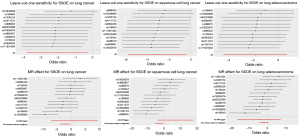
Table 1
Exposure or outcome | Unit | Author | Sample size | Year | PMID | Population |
---|---|---|---|---|---|---|
Strenuous sports or other exercises | NA | Klimentidis YC | 350,492 | 2018 | 29899525 | European |
Lung cancer | Log-odds | Wang Y | 27,209 | 2014 | 24880342 | European |
Squamous cell lung cancer | Log-odds | Wang Y | 18,313 | 2014 | 24880342 | European |
Lung adenocarcinoma | Log-odds | Wang Y | 18,336 | 2014 | 24880342 | European |
NA, not available.
Table 2
Exposure | Outcome | Method | SNPs | P value | OR | 95% CI | |
---|---|---|---|---|---|---|---|
Lower limit | Upper limit | ||||||
Strenuous sports or other exercises | Lung adenocarcinoma | MR Egger | 12 | 0.47 | 0.011 | 0.000 | 1.370×103 |
Weighted median | 12 | 0.11 | 0.086 | 0.004 | 1.700 | ||
Inverse variance-weighted | 12 | 0.16 | 0.161 | 0.012 | 2.102 | ||
Simple mode | 12 | 0.49 | 0.142 | 0.001 | 29.791 | ||
Weighted mode | 12 | 0.28 | 0.096 | 0.002 | 5.612 | ||
Strenuous sports or other exercises | Lung cancer | MR Egger | 12 | 0.77 | 3.471 | 0.001 | 1.240×104 |
Weighted median | 12 | 0.24 | 0.281 | 0.033 | 2.377 | ||
Inverse variance-weighted | 12 | 0.03 | 0.129 | 0.021 | 0.779 | ||
Simple mode | 12 | 0.58 | 0.422 | 0.022 | 8.142 | ||
Weighted mode | 12 | 0.46 | 0.330 | 0.020 | 5.481 | ||
Strenuous sports or other exercises | Squamous cell lung cancer | MR Egger | 12 | 0.24 | 1.220×103 | 0.016 | 9.295×107 |
Weighted median | 12 | 0.11 | 0.066 | 0.002 | 1.769 | ||
Inverse variance weighted | 12 | 0.03 | 0.045 | 0.003 | 0.677 | ||
Simple mode | 12 | 0.24 | 0.018 | 0.000 | 9.736 | ||
Weighted mode | 12 | 0.27 | 0.051 | 0.000 | 7.617 |
MR, Mendelian randomization; SNPs, single nucleotide polymorphisms; OR, odds ratio; CI, confidence interval.
Table 3
Exposure | Outcome | Heterogeneity P value | Pleiotropy P value |
---|---|---|---|
Strenuous sports or other exercises | Lung cancer | 0.11 | 0.44 |
Strenuous sports or other exercises | Squamous cell lung cancer | 0.14 | 0.10 |
Strenuous sports or other exercises | Lung adenocarcinoma | 0.20 | 0.66 |
Multivariable MR
We conducted a multivariable MR analysis to investigate the effect of SSOE on lung cancer after adjusting for waist circumference and smoking status (never). The combined OR for lung cancer from SSOE was estimated at 0.054 (95% CI: 0.010–0.302, P<0.001), which indicates a significant inverse relationship (Figure 4). We also conducted an MR-Egger regression analysis (P=0.44), which showed a non-significant pleiotropic effect. To further test the robustness of our findings, we performed Cochran’s Q statistic and MR-PRESSO model to detect possible outliers. The P value of the Q statistic was 0.055, indicating no significant heterogeneity. Moreover, no outliers were detected in the MR-PRESSO model (P=0.07), suggesting the absence of bias in the multivariable MR analysis (Table 4). Overall, our multivariable MR analysis confirms that genetic predisposition to SSOE is inversely associated with lung cancer risk, even after adjusting for waist circumference and smoking status.
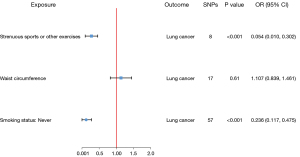
Table 4
Exposure | Outcome | Egger- intercept |
P value | Q-statistic P value | 95% CI of MV medium | P value of MV medium | MR-PRESSO results in P value |
---|---|---|---|---|---|---|---|
Strenuous sports or other exercises | Lung cancer | −0.027 | 0.44 | 0.055 | −5.455, −1.120 | 0.003 | 0.07 |
Waist circumference | Lung cancer | 0.009 | 0.61 | −0.360, 0.305 | 0.87 | ||
Smoking status: never | Lung cancer | −0.005 | 0.71 | −2.146, −0.306 | 0.009 |
MVMR, multivariable Mendelian randomization; CI, confidence interval; MV, multivariable; MR-PRESSO, Mendelian randomization pleiotropy residual sum and outlier.
Discussion
The current MR analysis has revealed a favorable association between engagement in SSOE and a reduced risk of lung cancer. Even after adjusting for waist circumference and excluding never-smokers, the MR analysis still identified an inverse relationship between SSOE and lung cancer risk. Waist circumference has been previously linked to lung cancer, even in individuals who have never smoked (34). Therefore, the exclusion of never-smokers and the impact of waist circumference was necessary to enhance the accuracy of the study.
Individuals who engaged in SSOE for 2–3 days or more each week, for a duration of 15–30 minutes or greater, were included in the study, while those who had not participated in SSOE in the previous four weeks were used as controls. Extreme values were chosen due to the highly skewed and zero-inflated distribution of these variables (22).
While the precise biological pathways linking SSOE to tumorigenesis remain incompletely elucidated, it is widely acknowledged that SSOE plays a role in maintaining a healthy body weight, thereby reducing the risk of metabolic disorders, chronic low-grade inflammation, and excessive endogenous sex hormone activity. There is accumulating evidence indicating that promoting physical activity and reducing sedentary behaviors confer significant preventive health advantages against cancer. Moreover, the accumulation of ectopic fat tissue, characterized by the storage of triglycerides outside of adipose tissue in areas such as the liver, skeletal muscle, heart, and pancreas, poses a notable concern due to its potential disruption of normal cellular and organ functions, thereby heightening the risk of various chronic diseases, including cancer (35).
Exercise has been shown to be beneficial for cancer patients, as it can reduce the risk of developing cancer and inhibit tumor progression. Numerous studies have found that exercise can reduce the incidence of lung cancer by generating changes in tumor microenvironmental categories (36,37). There are several mechanisms for this, including the possibility that exercise improves systemic immunity by increasing the number of circulating immune cells, or that exercise influences immune cell activity within the tumor by modulating local environmental conditions (e.g., oxygen and nutrient supply), as well as the degree of inflammation and stress response (37,38). Furthermore, exercise can reduce the levels of inflammatory factors (e.g., TNF-a, IL-6, IL-1b) and reactive oxygen species in tumor tissues, reducing tumor growth (39-41). Exercise has an epigenetic influence on telomeres, and preventing telomere dysregulation by protecting telomeres from noncoding RNA transcription mistakes that occur during cell division is another mechanism being studied (42). The recent focus in the scientific community has been on investigating the interactions between SSOE and metabolic genes, particularly mitochondrial markers such as ACOT11 and PDHB, in relation to lung cancer. These studies have garnered significant attention in the field (43-45). Other mechanisms that may be talked about include p53-mediated apoptosis, inhibition of lung cancer cell proliferation and survival, enhancement of host immunity, promotion of immune cell infiltration, improvement of the tumor microenvironment, attenuation of chronic inflammation, activation of DNA repair enzymes, and enhancement of anti-oxidative stress (7-16). Preclinical studies have demonstrated that exercise can directly slow malignant progression in mouse models of lung cancer. A pooled analysis of prospective studies has found a direct association between exercise frequency and a reduced risk of seven different cancers, further supporting the notion that regular exercise and healthy lifestyle choices can lower the risk of cancer (46).
A review of 25 studies found no significant difference in the risk of lung cancer between nonsmokers who exercised and those who did not (47), while smokers who exercised had a lower risk of lung cancer. These findings are consistent with the results of our MR study. Exercise is often used as a complementary therapy for lung cancer patients (48), with pre- and post-surgery exercise interventions demonstrating beneficial effects for patients undergoing surgery (49). In a preliminary randomized clinical trial, it was observed that a combination of aerobic exercise and high-intensity respiratory muscle training enhanced exercise capacity, respiratory muscle strength, and blood IGFBP-3 levels among non-small cell lung cancer patients after undergoing lung resection (50). The current MR analysis has revealed a favorable association between engagement in SSOE and a reduced risk of lung cancer. Even after adjusting for waist circumference and excluding never-smokers, the MR analysis still identified an inverse relationship between SSOE and lung cancer risk. Waist circumference has been previously linked to lung cancer, even in individuals who have never smoked (34). Therefore, the exclusion of never-smokers and the impact of waist circumference was necessary to enhance the accuracy of the study. Additionally, increasing physical activity among smokers can lower their risk of developing lung cancer. One possible explanation for this could be that exercise lowers the risk of lung cancer by reducing the level of chronic inflammation, particularly C-reactive protein (51), since chronic inflammation is known to accelerate the development of cancer in a number of cancers, including lung cancer, by interfering with processes like cell migration, survival, and proliferation (52-54). Even though exercise has demonstrated encouraging results in the treatment of lung cancer, more research is necessary to examine any potential synergistic anticancer effects of exercise in addition to immunotherapy (7,11).
The utilization of the MR design in this study represents a significant strength, as it minimizes residual confounding and reverse causality, thereby enhancing causal inference regarding the association between SSOE and lung cancer. Furthermore, the multivariable MR analysis method was applied to account for pleiotropic effects stemming from waist circumference and never-smokers, both of which are genetically linked to SSOE. The primary hurdle encountered in this study is the issue of horizontal pleiotropy, whereby certain genetic instrument characteristics may impact the risk of the outcome not through exposure but via alternative pathways. However, it is unlikely that this pleiotropic effect has biased our findings. The majority of associations in the current MR analysis did not reveal any evidence of pleiotropy as indicated by the MR-Egger intercept test. Although a few outliers were identified by the MR-PRESSO analysis for some associations with significant hints of horizontal pleiotropy, the relationship persisted or strengthened after the removal of outlying SNPs. Moreover, the consistency of the results was maintained even after adjustment for factors with high phenotypic and genetic correlations using the multivariable MR method.
It is worth noting that Xian et al. (23) used a MR approach and did not find a causally protective relationship between physical activity and lung cancer risk, in contrast to our study, which performed a more detailed analysis. Our research extracted summary-level data from GWASs. We meticulously adjusted for age, sex, and major genetic principal components in our association tests. In our statistical research, we used the two-sample MR approach, principally the IVW method with random effects, which has proven to be robust and widely accepted. Supplementary analyses included weighted median, MR-Egger regression, simple mode, and weighted mode. To ensure the trustworthiness of our findings, we performed leave-one-out analyses and examined pleiotropy and heterogeneity. Furthermore, we investigated multivariable MR analysis using a variety of methods, including the IVW method with random effects, MR-Egger regression, the multivariable median method, and the MR-PRESSO model. We addressed potential pleiotropy issues using the weighted median approach and MR-Egger analysis, as well as outlier detection and adjustment analyses. Our comprehensive approach enabled a complete analysis of the relationship between physical activity and lung cancer risk, offering a deeper and more precise knowledge than earlier studies with a broader scope, such as those of Xian et al.
It is worth noting that our studies were conducted solely on European populations to minimize population structure bias, but this limits the generalizability of our findings to other populations. While we accounted for waist circumference and smoking status, important lifestyle factors such as diet, alcohol consumption, and occupational exposures, which are recognized influencers of lung cancer risk, were not encompassed in our analysis. For example, different dietary habits in different populations may have different effects on the development of lung cancer. High intake of meat, especially fried or fully cooked red meat, may increase the risk of lung cancer (55). This omission may introduce residual confounding and undermine the strength of our causal inferences with the STROBE-MR reporting checklist. Furthermore, the intricacies of cancer development demand a more profound exploration of gene-environment interactions and their potential to modulate the association between exercise and lung cancer risk. In forthcoming investigations, we intend to rectify these limitations by incorporating a comprehensive array of confounding factors and delving into gene-environment interactions. As such, our results must be considered in light of their limitations. In our future research endeavors, we aim to broaden our scope by incorporating a diverse range of populations. This will enhance the robustness and applicability of our findings, ensuring they are more representative and generalizable. While pursuing direct validation is critical for increasing the trustworthiness of our results, it is critical to appreciate the intricacies and practical limitations associated with reaching this goal. In future research, we will make a greater effort to confirm the effects of other confounding factors on lung cancer in various populations with varied characteristics of the disease. This is also a problem in our article, and by highlighting these limits, we hope to pique others’ interest in future research areas. We will continue to develop new and efficient data collection approaches that offer possible solutions to these problems in future investigations. Ultimately, the underlying objective of our research endeavors lies in gaining a profound comprehension of lung cancer, enabling us to enhance our preventive measures and therapeutic interventions.
Conclusions
In summary, our MR analysis suggests that SSOE may be a protective factor against lung cancer, highlighting the importance of exercise in cancer prevention and treatment. Further research is needed to fully understand the molecular processes of exercise, including its impact on redox state and the interplay between metabolism and immune modulation in cancer. Nonetheless, exercise remains an essential component of treatment for various illnesses, including cancer. Personalized exercise treatment plans should be developed for patients with cancer to optimize their quality of life and treatment outcomes.
Acknowledgments
We would like to extend sincere gratitude to the nurses and clinical staff who provided care for the patients and the Guangzhou Institute of Respiratory Health, which provided us with a platform to conduct research into the respiratory system.
Funding: This work was supported by
Footnote
Reporting Checklist: The authors have completed the STROBE-MR reporting checklist. Available at https://tlcr.amegroups.com/article/view/10.21037/tlcr-23-810/rc
Peer Review File: Available at https://tlcr.amegroups.com/article/view/10.21037/tlcr-23-810/prf
Conflicts of Interest: All authors have completed the ICMJE uniform disclosure form (available at https://tlcr.amegroups.com/article/view/10.21037/tlcr-23-810/coif). The authors have no conflicts of interest to declare.
Ethical Statement: The authors are accountable for all aspects of the work in ensuring that questions related to the accuracy or integrity of any part of the work are appropriately investigated and resolved. The study was conducted in accordance with the Declaration of Helsinki (as revised in 2013).
Open Access Statement: This is an Open Access article distributed in accordance with the Creative Commons Attribution-NonCommercial-NoDerivs 4.0 International License (CC BY-NC-ND 4.0), which permits the non-commercial replication and distribution of the article with the strict proviso that no changes or edits are made and the original work is properly cited (including links to both the formal publication through the relevant DOI and the license). See: https://creativecommons.org/licenses/by-nc-nd/4.0/.
References
- Sung H, Ferlay J, Siegel RL, et al. Global Cancer Statistics 2020: GLOBOCAN Estimates of Incidence and Mortality Worldwide for 36 Cancers in 185 Countries. CA Cancer J Clin 2021;71:209-49. [Crossref] [PubMed]
- Bade BC, Dela Cruz CS. Lung Cancer 2020: Epidemiology, Etiology, and Prevention. Clin Chest Med 2020;41:1-24. [Crossref] [PubMed]
- Siegel RL, Miller KD, Jemal A. Cancer statistics, 2020. CA Cancer J Clin 2020;70:7-30. [Crossref] [PubMed]
- Wei X, Zhu C, Ji M, et al. Diet and Risk of Incident Lung Cancer: A Large Prospective Cohort Study in UK Biobank. Am J Clin Nutr 2021;114:2043-51. [Crossref] [PubMed]
- Patel AV, Friedenreich CM, Moore SC, et al. American College of Sports Medicine Roundtable Report on Physical Activity, Sedentary Behavior, and Cancer Prevention and Control. Med Sci Sports Exerc 2019;51:2391-402. [Crossref] [PubMed]
- Malhotra J, Malvezzi M, Negri E, et al. Risk factors for lung cancer worldwide. Eur Respir J 2016;48:889-902. [Crossref] [PubMed]
- Martín-Ruiz A, Fiuza-Luces C, Rincón-Castanedo C, et al. Benefits of exercise and immunotherapy in a murine model of human non-small-cell lung carcinoma. Exerc Immunol Rev 2020;26:100-15. [PubMed]
- Higgins KA, Park D, Lee GY, et al. Exercise-induced lung cancer regression: mechanistic findings from a mouse model. Cancer 2014;120:3302-10. [Crossref] [PubMed]
- Bade BC, Thomas DD, Scott JB, et al. Increasing physical activity and exercise in lung cancer: reviewing safety, benefits, and application. J Thorac Oncol 2015;10:861-71. [Crossref] [PubMed]
- Huang Q, Wu M, Wu X, et al. Muscle-to-tumor crosstalk: The effect of exercise-induced myokine on cancer progression. Biochim Biophys Acta Rev Cancer 2022;1877:188761. [Crossref] [PubMed]
- Ge Z, Wu S, Qi Z, et al. Exercise modulates polarization of TAMs and expression of related immune checkpoints in mice with lung cancer. J Cancer 2022;13:3297-307. [Crossref] [PubMed]
- Murphy EA, Davis JM, Brown AS, et al. Effects of moderate exercise and oat beta-glucan on lung tumor metastases and macrophage antitumor cytotoxicity. J Appl Physiol (1985) 2004;97:955-9. [Crossref] [PubMed]
- Pedersen L, Idorn M, Olofsson GH, et al. Voluntary Running Suppresses Tumor Growth through Epinephrine- and IL-6-Dependent NK Cell Mobilization and Redistribution. Cell Metab 2016;23:554-62. [Crossref] [PubMed]
- Elisia I, Cho B, Hay M, et al. The effect of diet and exercise on tobacco carcinogen-induced lung cancer. Carcinogenesis 2019;40:448-60. [Crossref] [PubMed]
- Ge Z, Wu S, Qi Z, et al. Compared with High-intensity Interval Exercise, Moderate Intensity Constant Load Exercise is more effective in curbing the Growth and Metastasis of Lung Cancer. J Cancer 2022;13:1468-79. [Crossref] [PubMed]
- Washington TA, Schrems ER, Haynie WS, et al. Development of skeletal muscle fibrosis in a rodent model of cancer cachexia. Cell Biochem Funct 2023;41:478-89. [Crossref] [PubMed]
- Idorn M, Thor Straten P. Exercise and cancer: from "healthy" to "therapeutic"? Cancer Immunol Immunother 2017;66:667-71. [Crossref] [PubMed]
- Avancini A, Sartori G, Gkountakos A, et al. Physical Activity and Exercise in Lung Cancer Care: Will Promises Be Fulfilled? Oncologist 2020;25:e555-69. [Crossref] [PubMed]
- Burgess S, Thompson SG. Mendelian Randomization: Methods for Using Genetic Variants in Causal Estimation. J R Stat Soc Ser A Stat Soc 2018;181:549-50. [Crossref]
- Larsson SC. Mendelian randomization as a tool for causal inference in human nutrition and metabolism. Curr Opin Lipidol 2021;32:1-8. [Crossref] [PubMed]
- Angrist JD, Imbens GW, Rubin DB. Identification of Causal Effects Using Instrumental Variables. J Am Stat Assoc 1996;91:444-55. [Crossref]
- Klimentidis YC, Raichlen DA, Bea J, et al. Genome-wide association study of habitual physical activity in over 377,000 UK Biobank participants identifies multiple variants including CADM2 and APOE. Int J Obes (Lond) 2018;42:1161-76. [Crossref] [PubMed]
- Xian W, Shen J, Zhou H, et al. Mendelian randomization study indicates lack of causal relationship between physical activity and lung cancer. J Cancer Res Clin Oncol 2021;147:177-81. [Crossref] [PubMed]
- Skrivankova VW, Richmond RC, Woolf BAR, et al. Strengthening the Reporting of Observational Studies in Epidemiology Using Mendelian Randomization: The STROBE-MR Statement. JAMA 2021;326:1614-21. [Crossref] [PubMed]
- Shungin D, Winkler TW, Croteau-Chonka DC, et al. New genetic loci link adipose and insulin biology to body fat distribution. Nature 2015;518:187-96. [Crossref] [PubMed]
- World Medical Association Declaration of Helsinki. ethical principles for medical research involving human subjects. JAMA 2013;310:2191-4. [Crossref] [PubMed]
- Clarke L, Zheng-Bradley X, Smith R, et al. The 1000 Genomes Project: data management and community access. Nat Methods 2012;9:459-62. [Crossref] [PubMed]
- Wang Y, McKay JD, Rafnar T, et al. Rare variants of large effect in BRCA2 and CHEK2 affect risk of lung cancer. Nat Genet 2014;46:736-41. [Crossref] [PubMed]
- Burgess S, Bowden J, Fall T, et al. Sensitivity Analyses for Robust Causal Inference from Mendelian Randomization Analyses with Multiple Genetic Variants. Epidemiology 2017;28:30-42. [Crossref] [PubMed]
- Bowden J, Davey Smith G, Haycock PC, et al. Consistent Estimation in Mendelian Randomization with Some Invalid Instruments Using a Weighted Median Estimator. Genet Epidemiol 2016;40:304-14. [Crossref] [PubMed]
- Bowden J, Davey Smith G, Burgess S. Mendelian randomization with invalid instruments: effect estimation and bias detection through Egger regression. Int J Epidemiol 2015;44:512-25. [Crossref] [PubMed]
- Verbanck M, Chen CY, Neale B, et al. Detection of widespread horizontal pleiotropy in causal relationships inferred from Mendelian randomization between complex traits and diseases. Nat Genet 2018;50:693-8. [Crossref] [PubMed]
- Hemani G, Zheng J, Elsworth B, et al. The MR-Base platform supports systematic causal inference across the human phenome. Elife 2018;7:e34408. [Crossref] [PubMed]
- Lam TK, Moore SC, Brinton LA, et al. Anthropometric measures and physical activity and the risk of lung cancer in never-smokers: a prospective cohort study. PLoS One 2013;8:e70672. [Crossref] [PubMed]
- Friedenreich CM, Ryder-Burbidge C, McNeil J. Physical activity, obesity and sedentary behavior in cancer etiology: epidemiologic evidence and biologic mechanisms. Mol Oncol 2021;15:790-800. [Crossref] [PubMed]
- Jones LW, Alfano CM. Exercise-oncology research: past, present, and future. Acta Oncol 2013;52:195-215. [Crossref] [PubMed]
- Jia N, Zhou Y, Dong X, et al. The antitumor mechanisms of aerobic exercise: A review of recent preclinical studies. Cancer Med 2021;10:6365-73. [Crossref] [PubMed]
- Esteves M, Monteiro MP, Duarte JA. Role of Regular Physical Exercise in Tumor Vasculature: Favorable Modulator of Tumor Milieu. Int J Sports Med 2021;42:389-406. [Crossref] [PubMed]
- Luo Z, Wan R, Liu S, et al. Mechanisms of exercise in the treatment of lung cancer - a mini-review. Front Immunol 2023;14:1244764. [Crossref] [PubMed]
- Thomas RJ, Kenfield SA, Jimenez A. Exercise-induced biochemical changes and their potential influence on cancer: a scientific review. Br J Sports Med 2017;51:640-4. [Crossref] [PubMed]
- Scheffer DDL, Latini A. Exercise-induced immune system response: Anti-inflammatory status on peripheral and central organs. Biochim Biophys Acta Mol Basis Dis 2020;1866:165823. [Crossref] [PubMed]
- Ntanasis-Stathopoulos J, Tzanninis JG, Philippou A, et al. Epigenetic regulation on gene expression induced by physical exercise. J Musculoskelet Neuronal Interact 2013;13:133-46. [PubMed]
- Jin X, Liu D, Kong D, et al. Dissecting the alternation landscape of mitochondrial metabolism-related genes in lung adenocarcinoma and their latent mechanisms. Aging (Albany NY) 2023;15:5482-96. [Crossref] [PubMed]
- Giannos P, Kechagias KS, Gal A. Identification of Prognostic Gene Biomarkers in Non-Small Cell Lung Cancer Progression by Integrated Bioinformatics Analysis. Biology (Basel) 2021;10:1200. [Crossref] [PubMed]
- Li YP, Liu GX, Wu ZL, et al. A Novel Mitochondrial-Related Gene Signature for the Tumor Immune Microenvironment Evaluation and Prognosis Prediction in Lung Adenocarcinoma. J Immunol Res 2022;2022:5366185. [Crossref] [PubMed]
- Matthews CE, Moore SC, Arem H, et al. Amount and Intensity of Leisure-Time Physical Activity and Lower Cancer Risk. J Clin Oncol 2020;38:686-97. [Crossref] [PubMed]
- Schmid D, Ricci C, Behrens G, et al. Does smoking influence the physical activity and lung cancer relation? A systematic review and meta-analysis. Eur J Epidemiol 2016;31:1173-90. [Crossref] [PubMed]
- Cavalheri V, Granger CL. Exercise training as part of lung cancer therapy. Respirology 2020;25:80-7. [Crossref] [PubMed]
- Himbert C, Klossner N, Coletta AM, et al. Exercise and lung cancer surgery: A systematic review of randomized-controlled trials. Crit Rev Oncol Hematol 2020;156:103086. [Crossref] [PubMed]
- Messaggi-Sartor M, Marco E, Martínez-Téllez E, et al. Combined aerobic exercise and high-intensity respiratory muscle training in patients surgically treated for non-small cell lung cancer: a pilot randomized clinical trial. Eur J Phys Rehabil Med 2019;55:113-22. [Crossref] [PubMed]
- Kasapis C, Thompson PD. The effects of physical activity on serum C-reactive protein and inflammatory markers: a systematic review. J Am Coll Cardiol 2005;45:1563-9. [Crossref] [PubMed]
- Coussens LM, Werb Z. Inflammation and cancer. Nature 2002;420:860-7. [Crossref] [PubMed]
- Balkwill F, Mantovani A. Inflammation and cancer: back to Virchow? Lancet 2001;357:539-45. [Crossref] [PubMed]
- O'Byrne KJ, Dalgleish AG. Chronic immune activation and inflammation as the cause of malignancy. Br J Cancer 2001;85:473-83. [Crossref] [PubMed]
- Sinha R, Kulldorff M, Curtin J, et al. Fried, well-done red meat and risk of lung cancer in women (United States). Cancer Causes Control 1998;9:621-30. [Crossref] [PubMed]