Efficacy and safety of immune checkpoint inhibitors for advanced non-small cell lung cancer with leptomeningeal metastases harboring targetable mutations
Highlight box
Key findings
• In our study, the median age of the enrolled patients was 54 years (range, 33–70 years) and 62.2% were female.
• Following immune checkpoint inhibitor (ICI) administration, the intracranial objective response rate and intracranial disease control rate for all enrolled patients were 18.9% and 62.2%, respectively. The median progression-free survival of all patients was 2.5 months [95% confidence interval (CI): 2.166–2.834 months], and the median overall survival (mOS) was 5.8 months (95% CI: 5.087–6.513 months).
• Both univariate and multivariate analyses revealed a significant increase in mOS for individuals who had previously undergone cranial radiation therapy compared to those who had not (P=0.02). Furthermore, different histology molecular types were found to be potentially associated with survival time.
What is known and what is new?
• Leptomeningeal metastases (LM) were more prevalent in non-small cell lung cancer (NSCLC) individuals harboring targetable gene mutations. Currently, the efficacy of immunotherapy in NSCLC cases harboring targetable mutations is controversial.
• Little data have been reported on the efficacy of ICI therapy in patients with NSCLC and LM, particularly those with targetable gene alterations and a history of failed tyrosine kinase inhibitor therapy. Our study showed the potential role of ICI therapy in these populations.
What is the implication, and what should change now?
• While ICI treatment improved survival in certain patients in our study and produced acceptable adverse events, the use of ICIs in patients with LM from driver gene-positive NSCLC still needs significant advancement. This is especially important due to the limited availability of curative drugs for patients with LM.
Introduction
Leptomeningeal metastases (LM) occur when cancer cells spread to the compartments that contain cerebrospinal fluid (CSF). The incidence of LM from solid tumors has risen due to advancements in systemic treatments for individuals with solid tumors, leading to extended patient survival, along with the refinement of more sensitive detection techniques (1). Non-small cell lung cancer (NSCLC) is a frequent contributor to LM, accounting for 3–5% of cases of advanced NSCLC. The incidence is even higher in specific patient subgroups with targetable mutations due to their extended lifespan resulting from novel molecular treatments (2) and may still be underestimated by virtue of the nonspecific symptoms and signs. Although the use of tyrosine kinase inhibitors (TKIs) has been shown to extend the lifespan of individuals with targetable mutations, the efficacy of TKIs in eradicating micro-metastases is compromised by insufficient TKI levels in the CSF, leading to a higher frequency of LM (3).
In previous retrospective studies (4,5), it was found that LM was more prevalent in individuals with epidermal growth factor receptor (EGFR) mutations than in those without EGFR mutations. Moreover, LM has been found in approximately 10.3% of cases with anaplastic lymphoma kinase (ALK) rearrangements and consistently presents as a late complication (6). Other genetic alterations may potentially yield benefit from targeted therapy, including the fusion of ROS proto-oncogene 1 (ROS1); alterations or amplification of Kirsten rat sarcoma virus (KRAS) G12C, proto-oncogene B-Raf (BRAF) V600E, and mesenchymal-epithelial transition factor (MET) exon 14; rearrangement of RET proto-oncogene (RET) and neurotrophic tyrosine receptor kinase type 1–3 (NTRK 1–3); and insertion mutations of human epidermal growth factor receptor 2 (HER2) exon 20 (7,8). However, there is no standard treatment regimen for patients with LM from NSCLC who harbor genomic alterations and have developed resistance to targeted therapy.
The diagnosis and monitoring of LM are challenging, as LM is a severe complication related to an unfavorable prognosis and a diminished quality of life for patients. A diagnostic flowchart, developed by the European Association for Neuro-Oncology-European Society for Medical Oncology (EAN-ESMO) group, includes neurologic symptoms, imaging, and CSF cytology (9,10). Initial clinical manifestations can be subtle, with typical indications comprising headache, nausea, vomiting, impaired vision, neurological impairments, unsteady walking, stupor, and even cerebral herniation (11). Brain and spinal cord magnetic resonance imaging (MRI) are standard methods for evaluating LM. Characteristic MRI findings include linear ependymal enhancement, enhancement of cranial nerve roots, and nodular leptomeningeal enhancement (12,13). The primary diagnostic criterion for LM is detecting tumor cells in the CSF. Although the specificity of CSF cytology methods is high, their quality and sensitivity are low. Huashan Hospital has created a mature platform dedicated to improving the positive rate of CSF cytology by accumulating practical experience and adjusting various procedural details. To optimize results, it is recommended to process recently obtained CSF samples within a 30-minute timeframe. In cases where the initial CSF cytology yields negative results, a second lumbar puncture should be considered. Additional immunocytochemical staining of cytokeratin (CK) 7, thyroid transcription factor 1 (TTF1), and CK20 may help to confirm the diagnosis when CSF cytology reports are equivocal, suspicious, or atypical of the CSF.
The prognosis of LM remains poor despite systemic treatment. Immune checkpoint inhibitors (ICIs) have emerged as the preferred initial therapy for patients with driver gene-negative NSCLC regardless of programmed cell death-ligand 1 (PD-L1) expression (14). However, for driver gene-positive patients, platinum-based chemotherapy remains the standard treatment option when TKI therapies prove ineffective (15). Currently, the efficacy of immunotherapy in NSCLC cases harboring targetable mutations is controversial (16). Subgroup analyses from the IMpower150 study (17) indicated that the combination of atezolizumab (PD-L1 antibody), bevacizumab, and chemotherapy improved overall survival (OS) in patients with EGFR-mutated NSCLC previously treated with TKIs. However, it is worth noting that this subgroup analysis involved a relatively small number of patients (n=35). In addition, ICIs have demonstrated encouraging efficacy in treating brain metastases from lung cancer, and it has been shown that the immune response can help these immune inhibitory molecules cross the blood-brain barrier (18-20). Unfortunately, there are limited data on the efficacy of ICIs for patients with LM, as these patients are often excluded from clinical trials. The LM population remains an understudied cohort and may have an opportunity for improved outcomes in the era of immunotherapy.
In this retrospective study, we conducted a thorough literature review and collected data from NSCLC patients harboring targetable mutations who were diagnosed with LM and treated with ICIs at Huashan Hospital. The aim of this study was to assess the efficacy and safety of ICI treatment in patients with advanced NSCLC harboring targetable mutations who have been diagnosed with LM. We present this article in accordance with the STROBE reporting checklist (available at https://tlcr.amegroups.com/article/view/10.21037/tlcr-24-477/rc).
Methods
Data collection and patients
Data from patients with NSCLC harboring targetable alterations and diagnosed with LM between January 2018 and May 2022 were collected. All patients were diagnosed and treated at the Department of Oncology, Huashan Hospital, Fudan University. A systematic search of the scholarly works published from January 2017 to May 2022 was carried out on the PubMed, Cochrane, and Embase Library databases with the specified search terms “leptomeningeal metastases”, “lung cancer”, “immune checkpoint inhibitor”, “PD-1/PD-L1 antibody”, “nivolumab”, “pembrolizumab”, “atezolizumab”, “avelumab”, “sintilimab”, and “camrelizumab”. We selected clinical studies that specifically investigated individuals with LM harboring targetable mutations treated with ICIs. Only studies with complete and comprehensive clinical information were included. Ultimately, six extensive multicenter series were selected from which 23 patients with NSCLC with targetable driver mutations were included. The flow diagram of patient enrollment is shown in Figure 1. The study was conducted in accordance with the Declaration of Helsinki (as revised in 2013). This study was approved by the Ethics Committee of Huashan Hospital, Fudan University (approval No. 2022-634). Informed consent was taken from all the patients.
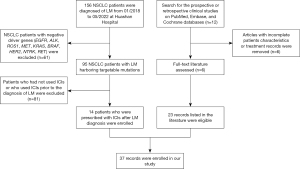
Inclusion and exclusion criteria
LM is diagnosed by the detection of tumor cells in the CSF or by the observation of characteristic findings on the MRI of the brain/spinal cord that are accompanied by typical neurological symptoms. In this study, the neuroimaging findings were confirmed by at least two experienced radiologists. Only patients treated with ICI monotherapy or combination therapy after the development of LM were included in the study. ICIs included PD-1 or PD-L1 antibodies such as nivolumab, pembrolizumab, atezolizumab, toripalimab, camrelizumab, and sintilimab. With the integration of molecular testing into advanced NSCLC management, identifying actionable genetic changes has become an essential part of the diagnostic criteria for NSCLC. The alterations that were targeted included deletions in EGFR exon 19; mutations in L858R/S768I/L861Q/G719X/T790M, KRAS G12C, and BRAF V600E; rearrangements in ALK, ROS1, and RET; rearrangements or alterations in MET exon 14; fusions in NTRK 1–3; and insertion of exon 20 in HER2 (ERBB2). These gene alterations were defined as targetable mutations due to the approval of corresponding targeted therapies for NSCLC by the U.S. Food and Drug Administration (FDA) or the European Medicines Agency (EMA).
The inclusion and exclusion criteria for the published series data were identical. Patients diagnosed with NSCLC and LM based on positive CSF analysis and/or imaging before beginning ICI therapy were included in all six prospective studies (14,20-24). Of these patients, those harboring targetable mutations were screened, and those with inadequate data were excluded. We ensured that all enrolled patients had been treated with ICIs after LM diagnosis and administration of standard treatment. These selected studies had the largest and most detailed medical records of patients with NSCLC and LM.
Assessment and statistical analysis
Data on demographics, clinical features, pathology, molecular analysis, treatment strategies, and survival outcomes were obtained retrospectively from the medical records and follow-up. Detailed neurological examinations, cerebrospinal MRI, computed tomography (CT) scans, and CSF tests were performed every 8–10 weeks or when patients complained of new neurological symptoms or signs. Apart from non-measurable lesions such as LM, other lesions were assessed based on the Response Evaluation Criteria in Solid Tumors (version 1.1) (25). For LM, the evaluation followed the Response Assessment in Neuro-Oncology (RANO) LM criteria (26), which involve a comprehensive assessment combining neurological system evaluation, neuroimaging evaluation, and standard CSF cytology, with the outcomes categorized as response, stable disease (SD), or progression. Both intracranial and extracranial conditions were evaluated according to these criteria.
Progression-free survival (PFS) started from the initiation of ICI treatment until the occurrence of progressive disease (PD) or death from any cause. OS was considered to be the time interval from the start of ICI treatment to death for any reason. The main outcomes of the study were the following: intracranial objective response rate (iORR), defined as the proportion of patients who achieved a complete response (CR) or a partial response (PR) with intracranial measurable lesions after treatment or those with LM showing a response; intracranial disease control rate (iDCR), defined as the proportion of patients with objective response and those with an SD of intracranial lesions for a certain period; PFS; OS; and adverse events (AEs). We employed the Kaplan-Meier method to compute survival curves and employed the log-rank test to compare variables.
In addition, we used a Cox proportional hazards regression model to assess the potential correlation between the clinical factors we analyzed and survival time. Statistical analyses were performed using SPSS 27.0 (IBM Corp.).
Results
Patient selection and characteristics
A total of 156 patients with stage IV or recurrent metastatic NSCLC were diagnosed with LM between January 2018 and May 2022 at Huashan Hospital, Fudan University. Among them, 95 patients harbored targetable mutations, and 14 patients were treated with ICIs after LM diagnosis. All patients experienced continued disease progression or intolerable side effects during standard treatments, particularly those treated with TKIs. Our literature search further filtered six prospective clinical studies with the largest populations and detailed multicenter records, with a focus on cases of NSCLC with LM. In the literature, 29 out of the 51 patients with NSCLC and LM possessing targetable mutations were treated with ICIs. After excluding six patients with incomplete records, 37 patients were ultimately included. The median follow-up duration after the initial ICI treatment was 10 months.
The characteristics of the 37 patients in our study are summarized in Table 1. The median age of the cohort was 54 years (range, 33–70 years), and 23 patients (62.2%) were female. More than half (19/37) of the patients were former or current smokers. During ICI therapy, brain metastases were found in 64.9% (24/37) of the individuals. Only five patients did not show typical neurological symptoms, while the remaining 32 patients did show these symptoms, varying from mild headache to severe neurological symptoms, such as visual disorders, headache, vomiting, facial paralysis, sensory disorders, and unstable walking. Although tumor cells were found in the CSF of 21 patients (56.8%), the patients from our institution had a higher percentage of positive results from CSF cytology at 78.6% (11/14), owing to the higher sensitivity of our CSF cytology diagnostic platform.
Table 1
Case No. | Age at start of ICI (years) | Gender | Smoking history | ECOG PS score | Histology/molecular status | PD-L1 | BM | Symptoms before ICI treatment |
---|---|---|---|---|---|---|---|---|
1 | 54 | F | N | 2 | EGFR | + | Y | Headache, vomiting |
2 | 59 | M | N | 2 | EGFR | − | N | Headache, epileptic seizure |
3 | 56 | F | N | 2 | EGFR | − | Y | Headache, blindness |
4 | 70 | M | Y | 2 | EGFR | − | Y | Facial paralysis |
5 | 47 | F | N | 1 | ALK | + | Y | Headache, neck pain |
6 | 69 | F | N | 2 | EGFR | UA | Y | Headache, nausea |
7 | 55 | F | N | 2 | EGFR | UA | Y | Headache, vomiting |
8 | 70 | M | Y | 2 | EGFR, MET | UA | Y | Visual disturbances |
9 | 50 | F | N | 2 | ERBB2 | UA | Y | Visual disturbances |
10 | 52 | M | N | 2 | EGFR | UA | N | Headache, walking problems |
11 | 33 | M | Y | 2 | EGFR | UA | N | Headache, vomiting |
12 | 57 | M | Y | 2 | EGFR | UA | Y | Nausea, walking problems |
13 | 65 | M | N | 2 | EGFR | UA | Y | Dysarthria, walking problems |
14 | 51 | F | N | 1 | ALK, MET | + | Y | Headache, nausea |
15 | 65 | F | Y | 1 | KRAS | + | N | Visual disturbances |
16 | 53 | M | Y | 1 | KRAS | UA | N | Facial paralysis |
17 | 63 | F | UA | 1 | KRAS | + | Y | Headache |
18 | 69 | M | Y | 1 | ALK | UA | N | Light headache, vertigo |
19 | 52 | F | Y | 2 | EGFR | UA | Y | Blindness, nausea |
20 | 55 | F | Y | 2 | KRAS | UA | Y | Headache, nausea |
21 | 51 | F | Y | 1 | MET | + | Y | Sensory loss, pain in legs |
22 | 69 | F | N | 2 | EGFR | UA | Y | None |
23 | 53 | F | Y | 1 | BRAF | + | N | Light headache |
24 | 44 | F | N | 1 | EGFR | + | Y | None |
25 | 56 | M | Y | 1 | EGFR | + | Y | None |
26 | 38 | M | N | 1 | EGFR | UA | N | None |
27 | 34 | F | N | 2 | EGFR | + | N | Headache, nausea, vomiting |
28 | 45 | M | Y | 1 | ERBB2 | − | Y | Headache |
29 | 45 | F | N | 1 | EGFR | UA | N | Dizziness, speech disorder |
30 | 66 | F | Y | 2 | KRAS | + | N | Visual disorder |
31 | 54 | M | Y | 2 | KRAS | UA | Y | Facial paralysis |
32 | 64 | F | UA | 1 | KRAS | + | Y | Headache |
33 | 69 | M | Y | 2 | ALK | UA | N | Light headache, vertigo |
34 | 52 | F | Y | 2 | EGFR | − | Y | Visual disorder, nausea |
35 | 51 | F | Y | 2 | MET | + | Y | Sensory loss |
36 | 69 | F | N | 2 | EGFR | UA | Y | None |
37 | 54 | F | Y | 2 | BRAF | + | N | Light headache |
ICI, immune checkpoint inhibitor; F, female; M, male; N, no; Y, yes; UA, unavailable; ECOG PS, Eastern Cooperative Oncology Group performance status; EGFR, epidermal growth factor receptor; ALK, anaplastic lymphoma kinase; MET, mesenchymal-epithelial transition factor; KRAS, Kirsten rat sarcoma virus; BRAF, proto-oncogene B-Raf; PD-L1, programmed cell death-ligand 1; BM, brain metastasis.
The patients harbored different gene subtypes at baseline: 20 (54.1%) harbored EGFR mutations, including the more common EGFR mutations such as exon 19 deletion or exon 21 L858R point mutation, and the less common EGFR mutations such as the exon 18 G719X point mutation. Some of these patients acquired the EGFR T790M mutation after first- or second-generation EGFR-TKI therapy. One patient acquired an additional C797S mutation after treatment with a third-generation EGFR-TKI and benefited from the combined treatment with first- and third-generation inhibitors. Furthermore, two patients acquired MET exon 14 alterations after developing resistance to EGFR-TKIs, two had ERBB2 E20 mutations, and seven had KRAS mutations. In addition to these driver genes, alteration of the TP53 gene was the most common combined mutation.
Patients’ treatment modalities
The history of radiotherapy (RT) was unavailable for ten patients, while 16 underwent brain RT either before or during their ICI treatments. None of the patients received ICIs as their initial treatment. Most patients opted for ICIs in backline therapy, with a median of fourth treatment lines. In our study, the ICI therapies comprised PD-1 and PD-L1 antibodies as a monotherapy (18/37, 48.6%) or in combination (19/37, 51.4%) with chemotherapy, bevacizumab, anlotinib, or other agents. The most commonly prescribed ICI was nivolumab, taken by 19 patients, followed by pembrolizumab, which eight patients took. Additionally, four patients received camrelizumab, two received toripalimab, and one received sintilimab. Three patients received the PD-L1 antibody atezolizumab. As the patients with LM consistently presented with symptoms of high intracranial pressure, five received ventriculoperitoneal shunt (VPS) surgery to relieve hydrocephalus. The details of LM, PFS, and OS resulting from ICIs for each patient are listed in Table 2.
Table 2
Case No. | Type of ICI | Mono/combined | CSF | MRI | Brain RT | VPS | Brain RT before ICI | Intracranial response | ICI PFS (m) |
OS (m) |
ICI treatment line | Major AE |
---|---|---|---|---|---|---|---|---|---|---|---|---|
1 | Nivolumab | Combined | + | Y | N | Y | N | PR | 4 | 9.5 | 3 | Arrhythmia |
2 | Nivolumab | Combined | + | Y | N | Y | N | PR | 2.5 | 3.5 | 4 | Myelosuppression |
3 | Nivolumab | Combined | − | Y | Y | N | Y | PR | 4 | 11 | 4 | Encephalitis |
4 | Camrelizumab | Combined | + | Y | Y | N | Y | PR | 7 | 10 | 4 | Eructation |
5 | Camrelizumab | Combined | eq | Y | Y | N | Y | SD | 6 | 10+ | 4 | Vomiting |
6 | Camrelizumab | Combined | + | Y | N | N | N | PD | 2 | 5.5 | 4 | Hypothyroidism |
7 | Sintilimab | Combined | + | Y | N | Y | N | SD | 2 | 5.5 | 7 | Hypothyroidism |
8 | Nivolumab | Combined | + | Y | N | N | N | PD | 1 | 4.2 | 7 | UA |
9 | Toripalimab | Combined | + | N | N | N | N | SD | 2 | 8 | 2 | Encephalitis |
10 | Nivolumab | Combined | + | Y | N | Y | N | PD | 1 | 3.2 | 5 | Eructation |
11 | Toripalimab | Combined | + | Y | N | Y | N | SD | 2 | 2 | 3 | Hypothyroidism |
12 | Nivolumab | Combined | − | Y | Y | N | Y | SD | 2 | 19 | 3 | Encephalitis |
13 | Atezolizumab | Combined | + | Y | N | N | N | SD | 2 | 6 | 4 | Eructation |
14 | Camrelizumab | Combined | + | N | Y | N | Y | SD | 4 | 14+ | 3 | Hypothyroidism |
15 | Pembrolizumab | Mono | − | Y | N | UA | N | SD | 7.3 | 13+ | 3 | UA |
16 | Nivolumab | Mono | + | Y | N | UA | N | PR | 6.4 | 10.7 | 2 | UA |
17 | Pembrolizumab | Mono | − | N | Y | UA | Y | SD | 6.1 | 12.9+ | 2 | UA |
18 | Nivolumab | Mono | + | Y | N | UA | N | PD | 2.8 | 3.7 | 4 | UA |
19 | Nivolumab | Mono | − | Y | Y | UA | Y | SD | 2.5 | 10.6+ | 4 | UA |
20 | Nivolumab | Mono | − | Y | Y | UA | Y | PD | 2 | 2 | 2 | UA |
21 | Pembrolizumab | Mono | + | Y | Y | UA | Y | PD | 1.9 | 2 | 5 | UA |
22 | Nivolumab | Mono | − | Y | Y | UA | Y | PD | 1.7 | 5.8 | 7 | UA |
23 | Nivolumab | Mono | + | Y | N | UA | N | PD | 0.7 | 1.9 | 3 | UA |
24 | Atezolizumab | Combined | + | N | Y | UA | Y | PR | 2.8 | 6.2 | UA | UA |
25 | Pembrolizumab | Combined | − | Y | N | UA | N | SD | 2.2 | 2.1 | UA | UA |
26 | Atezolizumab | Combined | − | Y | N | UA | N | PD | 5 | 5.4 | UA | UA |
27 | Pembrolizumab | Combined | − | N | Y | UA | Y | PD | 0.2 | 3 | UA | UA |
28 | Nivolumab | Combined | + | Y | N | UA | N | PR | 0.6 | 0.8 | UA | UA |
29 | Nivolumab | Combined | + | Y | N | UA | N | SD | 4.7 | 4.8 | UA | UA |
30 | Pembrolizumab | Mono | − | Y | N | UA | N | SD | 7 | 13 | UA | UA |
31 | Nivolumab | Mono | − | Y | N | UA | N | PR | 6 | 11 | UA | UA |
32 | Pembrolizumab | Mono | eq | Y | Y | UA | Y | SD | 5.8 | 13 | UA | UA |
33 | Nivolumab | Mono | + | Y | N | UA | N | PD | 2.8 | 3.7 | UA | UA |
34 | Nivolumab | Mono | − | Y | Y | UA | Y | SD | 2.8 | 11 | UA | UA |
35 | Pembrolizumab | Mono | + | Y | Y | UA | Y | PD | 2 | 2 | UA | UA |
36 | Nivolumab | Mono | UA | Y | Y | UA | Y | PD | 2 | 5.8 | UA | UA |
37 | Nivolumab | Mono | + | Y | N | UA | N | PD | 0.5 | 1.9 | UA | UA |
ICI, immune checkpoint inhibitor; Mono, monotherapy; Combined, combined therapy; CSF, cerebrospinal fluid; eq, equivocal; MRI, magnetic resonance imaging; Y, yes; N, no; RT, radiotherapy; VPS, ventriculoperitoneal shunt; UA, unavailable; PR, partial response; SD, stable disease; PD, progressive disease; PFS, progression-free survival; OS, overall survival; m, months; AE, adverse event.
Efficacy of ICI therapy
Seven patients (18.9%) demonstrated PR consisting of intracranial involvement after a comprehensive assessment, which included an evaluation of the combined neurological system, neuroimaging, and CSF cytology. Moreover, 16 patients (43.2%) exhibited stable symptoms of intracranial metastases, while 14 patients (37.8%) experienced PD with deteriorating symptoms after immunotherapy. As a result, the iORR and iDCR for all enrolled patients were 18.9% and 62.2%, respectively. All 14 patients from our institute received at least two cycles of ICIs and baseline brain MRI imaging. Two patients (patient #5 and patient #7) were excluded due to progression of extracranial lesions (including liver and lymph nodes), while the other 12 patients were excluded due to poor control of LM lesions. Of these, one patient benefited from ICIs with symptom relief and extended PFS (patient #4). Among the seven patients with SD as the best central nervous system response, one experienced PR as the best extracranial response, and two patients did not experience intracranial PD according to MRI but did experience extracranial PD during follow-up.
As shown in Figure 2, the median PFS (mPFS) of all included patients was 2.5 months [95% confidence interval (CI): 2.166–2.834 months], and the median overall survival (mOS) was 5.8 months (95% CI: 5.087–6.513 months). Notably, better mPFS and mOS were observed in the subgroup of patients with KRAS mutations than in the subgroups with EGFR alterations, ALK fusion variants, and those with other mutations (such as BRAF, MET, and ERBB2); specifically, the mPFS were 6.1, 2.1, 3.4, and 1.3 months for these subgroups, respectively, while the mOS were 12.9, 5.65, 3.7, and 1.9 months, respectively. The 3-month PFS and OS rates were 48.1% and 75.7%, respectively. Only three patients obtained a PFS exceeding 6 months (3/37), with one experiencing gradual symptomatic improvement. Furthermore, the 6-month OS rate and 12-month OS rate were 43.2% and 16.2%, respectively. Throughout the treatment course, 13 patients had the opportunity to receive subsequent therapy such as chemotherapy, bevacizumab, or anlotinib in combination with or without ICIs or TKIs, along with the best supportive care.
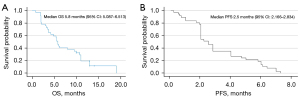
Univariate analysis revealed that a history of cranial radiation therapy was significantly associated with a longer OS (P=0.02) (Figure 3A). However, mPFS between the groups that underwent RT or without RT showed no statistical significance (P=0.94) (Figure 3B). In addition, different histology molecular types were associated with the PFS and OS. As previously mentioned, patients in the KRAS group had the longest OS and PFS among the four genomic groups, as illustrated in the survival curves (Figure 3C,3D). Meanwhile, monotherapy/combination therapy, age, gender, smoking history, performance status (PS) score, and presence or absence of brain metastasis were not statistically associated with PFS or OS. After adjusting for the other variables, multivariate analysis (Figure 4) confirmed the associations between a history of cranial radiation therapy, different genomic groups, and OS.
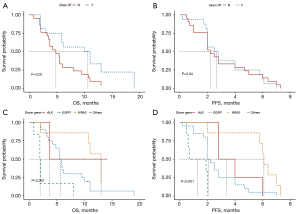
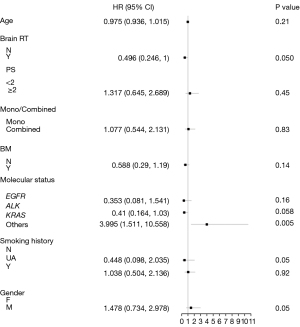
AEs and safety
AEs of the 14 patients from our institution were recorded in Table 2. Eructation, vomiting, myelosuppression, and constipation, were attributable to chemotherapy. The majority of AEs was hypothyroidism, which was determined to be related to immunotherapy. In one patient (patient #3), a post-treatment psychiatric abnormality was assessed by a neurologist, who identified it as autoimmune encephalitis, which was potentially linked to the ICI treatments. Certain AEs, such as interstitial pneumonia, were absent in the examined 14 patients.
Discussion
TKIs are the optimal treatment option for patients with LM harboring targetable gene alterations (21,27). Unfortunately, upon failure of TKI therapies, these patients typically have very few alternative therapeutic options. Although little data have been reported on the efficacy of ICI therapy in patients with NSCLC and LM, particularly those with targetable gene alterations and a history of failed TKI therapy, our study showed the potential role of ICI therapy in these populations. In this study, we analyzed 37 cases from multiple centers in detail.
There is currently a lack of agreement regarding the potential benefits of intracranial RT for patients with LM (28,29). Notably, there has been no implementation of randomized clinical trials to evaluate the efficacy and tolerance of RT in LM populations. A retrospective analysis found that NSCLC patients with LM (n=125) had a poor mOS of only 3 months. Furthermore, no therapeutic advantage was observed with whole-brain RT (30), and retrospective studies have failed to reveal any correlation between whole-brain RT and survival in patients with LM (31,32). However, our study found that the mOS of patients with a history of brain RT was longer than that of those lacking such a history.
In recent years, retrospective studies have shown that a combination of radiation with immunotherapy can improve locoregional and distant control by stimulating immune response and result in better survival outcomes (33-35). Radiation appears to boost the immune system by activating T cells, dendritic cells, and other immune cells in the tumor microenvironment (TME), releasing tumor-associated antigens and improving antigen presentation (36,37). In addition, brain RT can potentially disturb the blood-brain barrier, thereby facilitating the entry of additional peripheral effector T cells into the CSF.
Based on our results, we concluded that the clinical response to ICI therapy varies depending on the presence of diverse driver mutations. Regarding these molecular subgroups, KRAS-mutated patients with LM appeared to benefit the most from ICI treatment compared to those with EGFR-, ALK-, BRAF-, or HER2-mutated tumors. Consistent with the results of the IMMUNOTARGET study (38), patients with KRAS-mutated tumors (n=271) exhibited the highest percentage of PR or CR. The ORR reached 26%, with a mPFS of 3.2 months. This may be attributed to the higher likelihood of PD-1 and PD-L1 expression in KRAS-mutated NSCLC (39).
However, due to the limited sample size and missing data, we could not identify a substantial association between the clinical benefits of ICIs in the KRAS-mutated group and the degree of PD-L1 expression.
Given the significantly elevated incidence of LM in individuals with NSCLC harboring EGFR mutations, exploring the potential advantages of ICI treatment for EGFR-mutated patients with LM is imperative. In our restricted cohort, 20 out of 37 patients with LM harbored EGFR mutations. Among them, four showed symptomatic relief after ICI treatments (patient #7 might have benefited from VPS surgery), and two achieved a PFS exceeding 5 months (patients #4 and #27 received combined therapy). Despite the ongoing debate regarding the efficacy of immunotherapy in individuals with EGFR-mutated NSCLCs, our findings suggest that certain patients, including those with LM, can receive positive clinical results. A greater understanding of these cases may help us to identify patient populations harboring targetable mutations that would benefit from ICI therapy. A recent analysis summarized the impact of various oncogenic drivers of NSCLC on the immune TME and the response to ICIs (37). Further investigations are necessary to uncover the mechanisms responsible for the response to the therapy.
Several studies have confirmed the favorable tolerability of immune monotherapy, immune-combination platinum-containing chemotherapy, immune-combination chemotherapy, and anti-hematopoietic therapy after resistance to EGFR-TKI treatment. Additionally, the adverse effect profile was similar to the driver gene-negative NSCLC patients. The ATLANTIC study’s results (40) showed that EGFR+ or ALK+ patients treated with durvalumab monotherapy had a similar adverse effect profile to those of EGFR− and ALK− patients. The ORIENT-31 (41) and ORIENT-11 (42) studies explored the use of sintilimab in combination with chemotherapy in patients with resistant EGFR-mutated and primary driver gene-negative nonsquamous NSCLC. They found that both the efficacy and safety were generally consistent in both studies. In the EGFR mutation subgroup analysis of the IMpower150 study, AEs observed in the EGFR+ subgroup treated with atezolizumab combined with chemotherapy and bevacizumab were consistent with those of the overall population. In this study, only AEs from 14 patients at our institute—who were prescribed combination immunotherapy with chemotherapy and antiangiogenic therapy—were recorded. Generally, the treatment was relatively well tolerated, even in patients with poor PS scores. However, special attention is warranted for patients diagnosed with autoimmune encephalitis after ICI therapy, especially in those with intracranial metastases.
As discussed above, although ICI treatment could improve survival in some specific patients in our study and provide acceptable AEs, the application of ICIs in patients with LM from driver gene-positive NSCLC still requires substantial development. This is particularly crucial given the limited availability of curable drugs for patients with LM. Moving forward, an in-depth investigation into the underlying mechanisms and the identification of the most suitable treatment populations is warranted. Ongoing studies on LM are anticipated to provide valuable guidelines, shaping the trajectory of ICI application in this challenging clinical context.
Conclusions
The study found that some NSCLC patients with LM harboring targetable mutations might benefit from immunotherapy and have relatively good tolerance. For patients with LM who are resistant to targeted therapy, PD1/PD-L1 drugs, either alone or in combination, can be tried. However, more research is needed to accurately identify the benefiting population.
Acknowledgments
We thank all the individuals who took part in this research.
Funding: This study was funded by
Footnote
Reporting Checklist: The authors have completed the STROBE reporting checklist. Available at https://tlcr.amegroups.com/article/view/10.21037/tlcr-24-477/rc
Data Sharing Statement: Available at https://tlcr.amegroups.com/article/view/10.21037/tlcr-24-477/dss
Peer Review File: Available at https://tlcr.amegroups.com/article/view/10.21037/tlcr-24-477/prf
Conflicts of Interest: All authors have completed the ICMJE uniform disclosure form (available at https://tlcr.amegroups.com/article/view/10.21037/tlcr-24-477/coif). M.P. serves as an unpaid editorial board member of Translational Lung Cancer Research from October 2023 to September 2025. The other authors have no conflicts of interest to declare.
Ethical Statement: The authors are accountable for all aspects of the work in ensuring that questions related to the accuracy or integrity of any part of the work are appropriately investigated and resolved. The study was conducted in accordance with the Declaration of Helsinki (as revised in 2013). This study was approved by the Ethics Committee of Huashan Hospital, Fudan University (approval No. 2022-634). Informed consent was taken from all the patients.
Open Access Statement: This is an Open Access article distributed in accordance with the Creative Commons Attribution-NonCommercial-NoDerivs 4.0 International License (CC BY-NC-ND 4.0), which permits the non-commercial replication and distribution of the article with the strict proviso that no changes or edits are made and the original work is properly cited (including links to both the formal publication through the relevant DOI and the license). See: https://creativecommons.org/licenses/by-nc-nd/4.0/.
References
- Ozcan G, Singh M, Vredenburgh JJ. Leptomeningeal Metastasis from Non-Small Cell Lung Cancer and Current Landscape of Treatments. Clin Cancer Res 2023;29:11-29. [Crossref] [PubMed]
- Wen L, Zhen J, Shan C, et al. Efficacy and safety of osimertinib for leptomeningeal metastases from EGFR-mutant non-small cell lung cancer: a pooled analysis. Eur J Med Res 2023;28:267. [Crossref] [PubMed]
- Lee Y, Han JY, Kim HT, et al. Impact of EGFR tyrosine kinase inhibitors versus chemotherapy on the development of leptomeningeal metastasis in never smokers with advanced adenocarcinoma of the lung. J Neurooncol 2013;115:95-101. [Crossref] [PubMed]
- Li YS, Jiang BY, Yang JJ, et al. Leptomeningeal Metastases in Patients with NSCLC with EGFR Mutations. J Thorac Oncol 2016;11:1962-9. [Crossref] [PubMed]
- Pan K, Concannon K, Li J, et al. Emerging therapeutics and evolving assessment criteria for intracranial metastases in patients with oncogene-driven non-small-cell lung cancer. Nat Rev Clin Oncol 2023;20:716-32. [Crossref] [PubMed]
- Zheng MM, Li YS, Jiang BY, et al. Clinical Utility of Cerebrospinal Fluid Cell-Free DNA as Liquid Biopsy for Leptomeningeal Metastases in ALK-Rearranged NSCLC. J Thorac Oncol 2019;14:924-32. [Crossref] [PubMed]
- Fan Y, Qin J, Han N, et al. HER2 exon 20 insertion mutations in lung adenocarcinoma with leptomeningeal metastasis: a case report and response to poziotinib. Ann Palliat Med 2022;11:1582-8. [Crossref] [PubMed]
- Rabold K, Zoodsma M, Grondman I, et al. Reprogramming of myeloid cells and their progenitors in patients with non-medullary thyroid carcinoma. Nat Commun 2022;13:6149. [Crossref] [PubMed]
- Le Rhun E, Weller M, Brandsma D, et al. EANO-ESMO Clinical Practice Guidelines for diagnosis, treatment and follow-up of patients with leptomeningeal metastasis from solid tumours. Ann Oncol 2017;28:iv84-99. [Crossref] [PubMed]
- Le Rhun E, Devos P, Boulanger T, et al. The RANO Leptomeningeal Metastasis Group proposal to assess response to treatment: lack of feasibility and clinical utility and a revised proposal. Neuro Oncol 2019;21:648-58. [Crossref] [PubMed]
- Kaplan JG, DeSouza TG, Farkash A, et al. Leptomeningeal metastases: comparison of clinical features and laboratory data of solid tumors, lymphomas and leukemias. J Neurooncol 1990;9:225-9. [Crossref] [PubMed]
- Chamberlain M, Soffietti R, Raizer J, et al. Leptomeningeal metastasis: a Response Assessment in Neuro-Oncology critical review of endpoints and response criteria of published randomized clinical trials. Neuro Oncol 2014;16:1176-85. [Crossref] [PubMed]
- Chamberlain MC. Leptomeningeal metastases in the MRI era. Neurology 2011;76:200-author reply 200-1. [Crossref] [PubMed]
- Hendriks LEL, Bootsma G, Mourlanette J, et al. Survival of patients with non-small cell lung cancer having leptomeningeal metastases treated with immune checkpoint inhibitors. Eur J Cancer 2019;116:182-9. [Crossref] [PubMed]
- Ettinger DS, Wood DE, Aisner DL, et al. Non-Small Cell Lung Cancer, Version 3.2022, NCCN Clinical Practice Guidelines in Oncology. J Natl Compr Canc Netw 2022;20:497-530. [Crossref] [PubMed]
- Borghaei H, Paz-Ares L, Horn L, et al. Nivolumab versus Docetaxel in Advanced Nonsquamous Non-Small-Cell Lung Cancer. N Engl J Med 2015;373:1627-39. [Crossref] [PubMed]
- Reck M, Mok TSK, Nishio M, et al. Atezolizumab plus bevacizumab and chemotherapy in non-small-cell lung cancer (IMpower150): key subgroup analyses of patients with EGFR mutations or baseline liver metastases in a randomised, open-label phase 3 trial. Lancet Respir Med 2019;7:387-401. [Crossref] [PubMed]
- Brastianos PK, Lee EQ, Cohen JV, et al. Single-arm, open-label phase 2 trial of pembrolizumab in patients with leptomeningeal carcinomatosis. Nat Med 2020;26:1280-4. [Crossref] [PubMed]
- Brastianos PK, Strickland MR, Lee EQ, et al. Phase II study of ipilimumab and nivolumab in leptomeningeal carcinomatosis. Nat Commun 2021;12:5954. [Crossref] [PubMed]
- Goldberg SB, Schalper KA, Gettinger SN, et al. Pembrolizumab for management of patients with NSCLC and brain metastases: long-term results and biomarker analysis from a non-randomised, open-label, phase 2 trial. Lancet Oncol 2020;21:655-63. [Crossref] [PubMed]
- Zheng MM, Tu HY, Yang JJ, et al. Clinical outcomes of non-small cell lung cancer patients with leptomeningeal metastases after immune checkpoint inhibitor treatments. Eur J Cancer 2021;150:23-30. [Crossref] [PubMed]
- Dudnik E, Yust-Katz S, Nechushtan H, et al. Intracranial response to nivolumab in NSCLC patients with untreated or progressing CNS metastases. Lung Cancer 2016;98:114-7. [Crossref] [PubMed]
- Gauvain C, Vauléon E, Chouaid C, et al. Intracerebral efficacy and tolerance of nivolumab in non-small-cell lung cancer patients with brain metastases. Lung Cancer 2018;116:62-6. [Crossref] [PubMed]
- Gion M, Remon J, Caramella C, et al. Symptomatic leptomeningeal metastasis improvement with nivolumab in advanced non-small cell lung cancer patient. Lung Cancer 2017;108:72-4. [Crossref] [PubMed]
- Schwartz LH, Litière S, de Vries E, et al. RECIST 1.1-Update and clarification: From the RECIST committee. Eur J Cancer 2016;62:132-7. [Crossref] [PubMed]
- Chamberlain M, Junck L, Brandsma D, et al. Leptomeningeal metastases: a RANO proposal for response criteria. Neuro Oncol 2017;19:484-92. [PubMed]
- Yi Y, Cai J, Xu P, et al. Potential benefit of osismertinib plus bevacizumab in leptomeningeal metastasis with EGFR mutant non-small-cell lung cancer. J Transl Med 2022;20:122. [Crossref] [PubMed]
- Yan W, Liu Y, Li J, et al. Whole brain radiation therapy does not improve the overall survival of EGFR-mutant NSCLC patients with leptomeningeal metastasis. Radiat Oncol 2019;14:168. [Crossref] [PubMed]
- Barbour AB, Zaki P, McGranahan TM, et al. Emergent radiotherapy for brain and leptomeningeal metastases: a narrative review. Ann Palliat Med 2023;12:1405-19. [Crossref] [PubMed]
- Morris PG, Reiner AS, Szenberg OR, et al. Leptomeningeal metastasis from non-small cell lung cancer: survival and the impact of whole brain radiotherapy. J Thorac Oncol 2012;7:382-5. [Crossref] [PubMed]
- Gwak HS, Joo J, Kim S, et al. Analysis of treatment outcomes of intraventricular chemotherapy in 105 patients for leptomeningeal carcinomatosis from non-small-cell lung cancer. J Thorac Oncol 2013;8:599-605. [Crossref] [PubMed]
- Kuiper JL, Hendriks LE, van der Wekken AJ, et al. Treatment and survival of patients with EGFR-mutated non-small cell lung cancer and leptomeningeal metastasis: A retrospective cohort analysis. Lung Cancer 2015;89:255-61. [Crossref] [PubMed]
- Zhang I, Formenti SC, Knisely JPS. Immunotherapy Plus Stereotactic Radiosurgery: Building on the Promise of Precision Medicine for CNS Malignancies-PART 1: Principles of Combined Treatment. Oncology (Williston Park) 2018;32:e28-32. [PubMed]
- Qian JM, Yu JB, Kluger HM, et al. Timing and type of immune checkpoint therapy affect the early radiographic response of melanoma brain metastases to stereotactic radiosurgery. Cancer 2016;122:3051-8. [Crossref] [PubMed]
- Tagliaferri L, Lancellotta V, Fionda B, et al. Immunotherapy and radiotherapy in melanoma: a multidisciplinary comprehensive review. Hum Vaccin Immunother 2022;18:1903827. [Crossref] [PubMed]
- Bernstein MB, Krishnan S, Hodge JW, et al. Immunotherapy and stereotactic ablative radiotherapy (ISABR): a curative approach? Nat Rev Clin Oncol 2016;13:516-24. [Crossref] [PubMed]
- Kudo Y, Haymaker C, Zhang J, et al. Suppressed immune microenvironment and repertoire in brain metastases from patients with resected non-small-cell lung cancer. Ann Oncol 2019;30:1521-30. [Crossref] [PubMed]
- Mazieres J, Drilon A, Lusque A, et al. Immune checkpoint inhibitors for patients with advanced lung cancer and oncogenic driver alterations: results from the IMMUNOTARGET registry. Ann Oncol 2019;30:1321-8. [Crossref] [PubMed]
- West HJ, McCleland M, Cappuzzo F, et al. Clinical efficacy of atezolizumab plus bevacizumab and chemotherapy in KRAS-mutated non-small cell lung cancer with STK11, KEAP1, or TP53 comutations: subgroup results from the phase III IMpower150 trial. J Immunother Cancer 2022;10:e003027. [Crossref] [PubMed]
- Garassino MC, Cho BC, Kim JH, et al. Durvalumab as third-line or later treatment for advanced non-small-cell lung cancer (ATLANTIC): an open-label, single-arm, phase 2 study. Lancet Oncol 2018;19:521-36. [Crossref] [PubMed]
- Lu S, Wu L, Jian H, et al. Sintilimab plus bevacizumab biosimilar IBI305 and chemotherapy for patients with EGFR-mutated non-squamous non-small-cell lung cancer who progressed on EGFR tyrosine-kinase inhibitor therapy (ORIENT-31): first interim results from a randomised, double-blind, multicentre, phase 3 trial. Lancet Oncol 2022;23:1167-79. [Crossref] [PubMed]
- Yang Y, Wang Z, Fang J, et al. Efficacy and Safety of Sintilimab Plus Pemetrexed and Platinum as First-Line Treatment for Locally Advanced or Metastatic Nonsquamous NSCLC: a Randomized, Double-Blind, Phase 3 Study (Oncology pRogram by InnovENT anti-PD-1-11). J Thorac Oncol 2020;15:1636-46. [Crossref] [PubMed]