Immunohistochemistry identifies E-cadherin, N-cadherin and focal adhesion kinase (FAK) as predictors of stage I non-small cell lung carcinoma spread through the air spaces (STAS), and the combinations as prognostic factors
Highlight box
Key findings
• E-cadherin, N-cadherin, and focal adhesion kinase (FAK) are predictors of Stage I non-small cell lung carcinoma spread through the air spaces (STAS), and their combinations serve as prognostic factors.
What is known and what is new?
• STAS is one of the multiple modes of lung cancer dissemination, yet its molecular and clinicopathological characterization remains poorly studied.
• In patients with stage I non-small cell lung cancer (NSCLC), our study identified significant associations between the presence of E-cadherin, N-cadherin, and FAK with the occurrence of STAS. The combined risk indices of these markers were highly correlated with an increased risk of recurrence. Additionally, high N-cadherin/FAK expression in tumors provides information about a higher risk of recurrence in STAS-positive stage I lung carcinoma.
What is the implication, and what should change now?
• Utilizing immunohistochemistry to detect E-cadherin, N-cadherin, and FAK enables the enhanced identification of patients requiring adjuvant therapy or close postoperative monitoring.
Introduction
Spread through air spaces (STAS) is a new mode of invasion that has received widespread attention since Kadota et al. defined STAS in 2015 as the spread of tumor cells through the airspace into the lung parenchyma near the margins of the main tumor (1). Recent studies have increasingly validated the prognostic significance of STAS in predicting adverse clinical outcomes for patients with resected lung adenocarcinoma (2,3) and squamous cell carcinoma (4-6). Nevertheless, the correlation between STAS and the molecular profile of lung carcinoma remains underexplored.
Epithelial-mesenchymal transition (EMT) is a critical physiological process in embryonic development and tissue regeneration (7). Abnormal reactivation of EMT is associated with heightened tumor cell malignancy, characterized by elevated migration and invasiveness (7-9). Many independent studies have investigated markers indicative of EMT activation, suggesting a potential association between EMT and the development of tumor STAS, alongside adverse prognostic implications (6,10,11). The defining characteristic of EMT is the disruption of epithelial cell integrity, manifested by reduced adhesion junctions and resulting in cell detachment and enhanced motility (12). Cell adhesion molecules are cell surface proteins responsible for cell-cell interactions, and a variety of adhesion molecules play a crucial role in tumor progression, encompassing growth, invasion, and metastasis (13-16). Building upon the findings of prior research on EMT-related markers, we hypothesize that the incidence of STAS may be linked to altered intercellular adhesion junctions and increased migratory capabilities.
This study was designed to assess the impact of adhesion molecule expression on the incidence of STAS and postoperative recurrence in patients with stage I lung carcinoma undergoing radical resection. We present this article in accordance with the TRIPOD reporting checklist (available at https://tlcr.amegroups.com/article/view/10.21037/tlcr-24-247/rc).
Methods
Patients
The study was conducted in accordance with the Declaration of Helsinki (as revised in 2013). The study was approved by the Institutional Review Board of Nanjing Jinling Hospital (No. DBNJ20241) and individual consent for this retrospective analysis was waived. Patients with stage I primary squamous and adenocarcinoma of the lung who underwent sublobar resection or lobectomy with systemic lymph node dissection between January 2021 and December 2021 at Jinling Hospital were enrolled in the study. Exclusion criteria encompassed: patients with pathology reports from external hospitals (due to the absence of clinical and imaging data in our records); lack of available tumor specimens; tumors exceeding 4 cm in diameter; with regional lymph node metastasis; positive surgical margins; a history of neoadjuvant therapy; and loss to follow-up. The tumor node metastasis (TNM) staging was based on the 8th edition of the American Cancer Society’s TNM Staging Manual.
Histologic evaluation
Lung cancer sections stained with hematoxylin and eosin (H&E) were independently assessed by the pathologist who was blinded to the patients’ clinical data. The identification of STAS was based on the presence of small tumor cell clusters or nests within the lung parenchyma, extending beyond the primary tumor margins, a feature typically noted under low-power magnification in histopathological examinations. The tumors were classified based on the 2021 World Health Organization (WHO) lung cancer classification criteria (17), utilizing pathological characteristics for classification into highly differentiated (characterized by predominant adherent growth pattern without a high-grade component or with a high-grade component constituting less than 20%), moderately differentiated (marked by predominantly vesicular or papillary growth without a high-grade component or with a high-grade component less than 20%), and poorly differentiated types (defined by the presence of a high-grade component constituting 20% or more, with the high-grade component comprising solid, micropapillary, and complex glandular structures) (18). The poorly differentiated type was defined as having a high-risk growth pattern, and the remaining highly and moderately differentiated were defined as having a low-risk growth pattern. Lymphovascular invasion was identified by the detection of at least one cluster of cells within a lymphatic vessel or vein. STAS was defined by the occurrence of micropapillary clusters, small solid tumor nests, or individual cells within the air spaces of the surrounding lung parenchyma. To differentiate from artificially isolated cells during tumor dissection, a minimum of three tumor sections were examined microscopically, with initial identification at low magnification focusing on the boundaries. The presence of STAS was confirmed with tumor cells located beyond the primary tumor margins (1,2,17).
Immunohistochemical staining
Collected samples were tissue sectioned using whole block tumors. Tumor tissue sections were dewaxed and then hydrated, followed by antigen repair using high-pressure steam. Endogenous peroxidase was quenched with endogenous peroxidase blocker (Fuzhou Maixin Biotech, Fuzhou, China) for 30 min at room temperature. Next, nonspecific proteins were blocked with 5% goat serum (BOSTER, Wuhan, China) for 30 min. Subsequently, sections were incubated with E-cadherin-specific antibody (20874-1-AP, Proteintech; dilution ratio 1:10,000), P-cadherin antibody (13773-1-AP, Proteintech; dilution ratio 1:200), N-cadherin antibody (22018-1-AP, Proteintech; dilution ratio 1: 600), focal adhesion kinase (FAK) antibody (66258-1-Ig, Proteintech; dilution ratio 1: 100), epithelial cell adhesion molecule (EpCAM) antibody (66316-1-Ig, Proteintech; dilution 1: 200), neural cell adhesion molecule 1 (NCAM1) antibody (14255-1-AP, Proteintech; dilution 1: 600), and vascular cell adhesion molecule 1 (VCAM1) antibody (66294-1-Ig, Proteintech; dilution ratio 1:600), intercellular cell adhesion molecule-1 (ICAM-1) antibody (60299-1-Ig, Proteintech; dilution ratio 1:500) was incubated at 4 ℃ overnight. Color development was performed using the diaminobenzidine (DAB) horseradish peroxidase color development kit (Fuzhou Maixin Biotech). Finally, the sections were restained with hematoxylin, dehydrated, and sealed with neutral gum.
Immunohistochemical evaluation and quantification of adhesion molecule markers
Immunohistochemical analysis was employed to assess the presence of adhesion molecules within the membrane and cytoplasm of tumor cells. The assessment involved evaluating the staining distribution and intensity across primary tumors, with five tumor areas selected for analysis under an Olympus microscope featuring an EP50 digital camera (Olympus Corp., Tokyo, Japan) and a 20× objective lens. Depending on the percentage of positively stained cells, a score of 0 (0–25% of cells were positive), 1 (26–50% of cells were positive), 2 (51–75% of cells were positive) and 3 (76–100% of cells were positive) was assigned. Similarly, staining intensity was classified from 0 (no expression) to 3 (strong expression) (Figure 1). The average expression score for each patient was derived by aggregating the scores for distribution and intensity, resulting in a composite score between 0 and 6. Expression levels were deemed high or low relative to the median score; scores above the median indicated high expression, while those below indicated low expression.
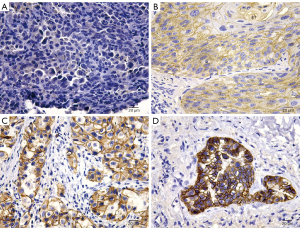
Statistical analysis
Correlations among variables were analyzed through using Chi-squared tests for categorical data and logistic regression to determine the independent correlations between STAS, clinicopathological features, and adhesion molecule expressions within a multivariate framework. Recurrence-free probability (RFP) was defined as the duration from surgical resection to the onset of disease recurrence and was calculated using the Kaplan-Meier estimator, with comparisons between groups conducted via the log-rank test. Furthermore, the impact of adhesion molecules on RFP, while adjusting for clinicopathological variables, was analyzed using Cox proportional hazards regression. Statistical tests were two-sided with a significance level set at 5%. Pearson correlation tests were utilized to explore the relationships between variables, ensuring that only variables without strong intercorrelations were included in the regression model to avoid redundancy. All statistical analyses were executed using SPSS for Windows, version 25.0 (IBM Corporation, Armonk, NY, USA).
Results
Association of STAS with clinicopathological features
Table S1 outlines the clinical and pathological features of 60 stage I lung carcinoma patients. The cohort’s median age was 64 (range, 37–87) years, with males comprising 52% (31 patients) of the population. The vast majority, 92% (55 patients), were classified as pathological stage Ia. Surgical procedures consisted of lobectomy in 80% (48 patients) and limited resection (sub-lobar resection) in 20% (12 patients). During the observation period, recurrence occurred in 20% (12 patients) of the cases. The median follow-up time for patients without recurrence was 27 months (average ± standard deviation: 28±4 months). Furthermore, 50% of the cases (30 patients) exhibited STAS, which was significantly associated with histologic growth patterns (P<0.001).
Association of STAS with adhesion molecules expression
Table 1 delineates the correlations between STAS presence and adhesion molecule expression. Significant associations were noted with E-cadherin, N-cadherin, FAK, and EpCAM (P=0.002, P=0.01, P=0.01, and P=0.002, respectively). After excluding variables that are strongly correlated or have a subgroup number of zero, multivariate logistic regression, controlling for sex, age, clinical stage, and tumor size, identified sex, E-cadherin, N-cadherin, and FAK as independent predictors of STAS (P=0.03, P=0.002, P=0.02, and P=0.03) (Table 2). Figure 2 shows representative images of STAS regarding E-cadherin, N-cadherin, and FAK. Univariate analysis revealed a significant association between the expression levels of E-cadherin, N-cadherin, and FAK with growth patterns and STAS, while no significant correlations were observed with sex, age, smoking status, clinical stage, surgical procedures, tumor size, lymphovascular invasion, or visceral pleural invasion (Table S2).
Table 1
Variables | Total, n [%] |
STAS, n [%] | P value | |
---|---|---|---|---|
Absent | Present | |||
E-cadherin expression | ||||
Low | 32 [53] | 10 [33] | 22 [73] | 0.002 |
High | 28 [47] | 20 [67] | 8 [27] | |
P-cadherin expression | ||||
Low | 32 [53] | 19 [63] | 13 [43] | 0.12 |
High | 28 [47] | 11 [37] | 17 [57] | |
N-cadherin expression | ||||
Low | 32 [53] | 21 [70] | 11 [37] | 0.01 |
High | 28 [47] | 9 [30] | 19 [63] | |
FAK expression | ||||
Low | 30 [50] | 20 [67] | 10 [33] | 0.01 |
High | 30 [50] | 10 [33] | 20 [67] | |
EpCAM expression | ||||
Low | 30 [50] | 21 [70] | 9 [30] | 0.002 |
High | 30 [50] | 9 [30] | 21 [70] | |
NCAM1 expression | ||||
Low | 32 [53] | 14 [47] | 18 [60] | 0.30 |
High | 28 [47] | 16 [53] | 12 [40] | |
VCAM-1 expression | ||||
Low | 30 [50] | 14 [47] | 16 [53] | 0.61 |
High | 30 [50] | 16 [53] | 14 [47] | |
ICAM-1 expression | ||||
Low | 30 [50] | 12 [40] | 18 [60] | 0.12 |
High | 30 [50] | 18 [60] | 12 [40] |
STAS, spread through air spaces; FAK, focal adhesion kinase; EpCAM, epithelial cell adhesion molecule; NCAM1, neural cell adhesion molecule 1; VCAM1, vascular cell adhesion molecule 1; ICAM-1, intercellular cell adhesion molecule-1.
Table 2
Variables | OR | 95% CI | P value |
---|---|---|---|
Sex (male vs. female) | 5.686 | 1.234–26.197 | 0.03 |
Age (≥65 vs. <65 years) | 1.022 | 0.228–4.588 | 0.98 |
Stage (Ib vs. Ia) | 1.676 | 0.061–45.970 | 0.76 |
Tumor size (>2 vs. ≤2 cm) | 2.544 | 0.573–11.292 | 0.22 |
E-cadherin expression (high vs. low) | 0.078 | 0.016–0.382 | 0.002 |
N-cadherin expression (high vs. low) | 6.247 | 1.374–28.403 | 0.02 |
FAK expression (high vs. low) | 4.912 | 1.194–20.203 | 0.03 |
STAS, spread through air spaces; OR, odds ratio; CI, confidence interval; FAK, focal adhesion kinase.
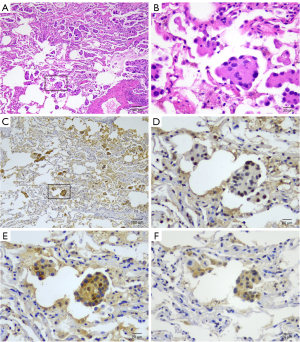
Associations between RFP and adhesion molecules marker expression
Table S3 presents the correlations between patient clinicopathological characteristics and RFP. Among the evaluated clinicopathological factors, clinical stage (P=0.02), growth patterns (P=0.03), and the presence of STAS (P=0.03) exhibited significant associations with recurrence rates. Patients with low E-cadherin expression (n=32; RFP, 69%) demonstrated significantly poorer RFP compared to those with high E-cadherin expression (n=28; RFP, 93%) (P=0.04). Similarly, high N-cadherin expression (n=28; RFP, 68%) correlated with significantly lower RFP than low N-cadherin expression (n=32; RFP, 91%) (P=0.03), and high FAK expression (n=30; RFP, 67%) was significantly associated with reduced RFP compared to low FAK expression (n=30; RFP, 93%) (P=0.01). No other individual adhesion molecule markers showed a significant correlation with RFP. Analysis of combinations of adhesion molecules, selected based on their significance in the univariate analysis, indicated significant prognostic implications for E-cadherin/N-cadherin, E-cadherin/FAK, and N-cadherin/FAK combinations (P=0.03, P=0.008, and P=0.004, respectively) (Table S4).
Based on the above results, paired adhesion molecules were stratified into four categories based on their combined expression levels: ‘low/low’, ‘high/low’, ‘low/high’, and ‘high/high’. The ‘low/high’ (E-cadherin/FAK, E-cadherin/N-cadherin) and ‘high/high’ (N-cadherin/FAK) category was designated as ‘high-risk’ due to its lowest RFP, while the other three categories were considered ‘low-risk’. Patients characterized by high-risk combinations of E-cadherin/N-cadherin, E-cadherin/FAK, and N-cadherin/FAK exhibited significantly inferior RFP compared to those in the low-risk groups (P=0.007, P=0.001, and P<0.001, respectively) (Figure 3). The results indicated that the prognostic significance of E-cadherin/N-cadherin and E-cadherin/FAK combinations was comparable to that of the N-cadherin/FAK combination.
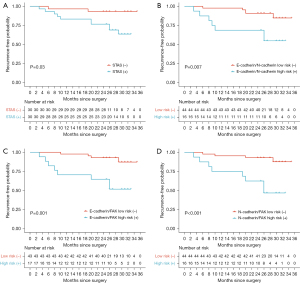
Multivariate survival analyses were conducted across four datasets, with redundancy minimized by retaining only one variable in cases of strong inter-variable correlations. Adjusting for sex, age, tumor size, and clinical stage, the analysis of the initial dataset revealed that STAS was independently predictive of an increased recurrence risk [hazard ratio (HR) =7.689, P=0.03]. Similarly, in the subsequent datasets, the E-cadherin/N-cadherin, E-cadherin/FAK, and N-cadherin/FAK risk indices were each found to be independently associated with elevated recurrence rates (P=0.04, P=0.03, and P=0.003, respectively) (Table 3).
Table 3
Variables | Model 1 | Model 2 | Model 3 | Model 4 | |||||||
---|---|---|---|---|---|---|---|---|---|---|---|
HR (95% CI) | P | HR (95% CI) | P | HR (95% CI) | P | HR (95% CI) | P | ||||
Sex | 0.267 (0.062–1.145) | 0.08 | 0.488 (0.140–1.700) | 0.26 | 0.593 (0.181–1.938) | 0.39 | 0.303 (0.077–1.192) | 0.09 | |||
Age | 3.724 (0.833–16.639) | 0.09 | 3.768 (0.908–15.641) | 0.07 | 2.251 (0.613–8.273) | 0.22 | 3.924 (0.982–15.691) | 0.053 | |||
Tumor size | 0.235 (0.046–1.201) | 0.08 | 0.385 (0.076–1.952) | 0.25 | 0.366 (0.077–1.729) | 0.20 | 0.462 (0.088–2.418) | 0.36 | |||
Stage | 4.127 (0.798–21.336) | 0.09 | 2.770 (0.374–20.507) | 0.32 | 3.737 (0.682–20.472) | 0.13 | 1.360 (0.167–11.072) | 0.77 | |||
STAS | 7.689 (1.284–46.031) | 0.03 | |||||||||
E-cadherin/ N-cadherin risk |
4.544 (1.091–18.922) | 0.04 | |||||||||
E-cadherin/FAK risk | 4.390 (1.206–15.986) | 0.03 | |||||||||
N-cadherin/FAK risk | 10.180 (2.197–47.170) | 0.003 |
STAS, spread through air spaces; HR, hazard ratio; CI, confidence interval; FAK, focal adhesion kinase.
Further examination focused on the interplay between clinicopathological features, adhesion molecule expression, and recurrence risk among STAS-positive patients. Notably, male patients with STAS exhibited significantly better RFP (n=19; RFP, 79%) compared to females (n=11; RFP, 45%) (P=0.048) (Table S5). Additionally, patients with a high N-cadherin/FAK risk index (n=13; RFP, 46%) demonstrated significantly poorer RFP than those with a low-risk index (n=17; RFP, 82%) (P=0.03) (Table S6). After adjusting for age, surgical procedures, and visceral pleural invasion, sex and the N-cadherin/FAK risk index were identified as independent predictors of RFP in STAS patients (P=0.03 and P=0.01, respectively) (Table S7).
Associations of clinicopathological features with E-cadherin/N-cadherin, E-cadherin/FAK, and N-cadherin/FAK high-risk index
Univariate analysis of the clinicopathologic characteristics of the E-cadherin/N-cadherin, E-cadherin/FAK, and N-cadherin/FAK risk indices revealed that they were significantly correlated with histological growth patterns as well as with STAS, with the N-cadherin/FAK risk index being significantly correlated with clinical staging (P=0.03). Furthermore, none of the three risk indices showed significant correlations with sex, age, smoking status, clinical stage, surgical procedures, STAS, histologic growth patterns, tumor size, lymphovascular invasion, or visceral pleural invasion (Table S8).
Discussion
This research entailed a detailed examination of various adhesion molecular markers, including E-cadherin, P-cadherin, N-cadherin, FAK, EpCAM, NCAM1, VCAM-1, and ICAM-1, in patients with stage I lung cancer who underwent surgical resection. The analysis identified low E-cadherin expression, high N-cadherin expression, and high FAK expression as independent predictors of a higher incidence of STAS. Furthermore, high-risk indices involving combinations of E-cadherin/N-cadherin, E-cadherin/FAK, and N-cadherin/FAK, significantly predicted RFP. In addition, a high risk of N-cadherin/FAK was found to be associated with the risk of recurrence in patients with STAS, after adjusting for sex, age, visceral pleural invasion, and surgical procedures.
STAS was recognized as a novel invasive pattern in the 2015 WHO classification of lung tumors (17). STAS has been identified as a detrimental prognostic indicator for recurrence and survival in non-small cell lung cancer (NSCLC) patients, a finding supported by meta-analyses and validated across large, independent cohorts internationally (2,3,17,19-21). Prior research indicates that patients exhibiting STAS and identified with high-risk factors for recurrence derive a survival advantage from adjuvant chemotherapy (22). Thus, more precise identification of STAS patients with high-risk factors can better guide clinical treatment planning.
Cell adhesion molecules, located on the cell surface, facilitate cell-cell interactions and include cadherins, the immunoglobulin superfamily, integrins, selectins, and other non-classical adhesion molecules. These molecules play pivotal roles in mediating tumor growth, invasion, and metastasis (13,15,16).
FAK, a crucial tyrosine kinase within the integrin signaling cascade, becomes phosphorylated on tyrosine (Tyr397) following activation. This leads to the formation of a FAK/Src complex, which orchestrates the remodeling of the actin cytoskeleton via phosphorylation. Consequently, it triggers focal adhesion relaxation, exerting a substantial impact on cellular migration, cytoskeletal rearrangement, and adhesion dynamics (23-28).
Aberrant activation of EMT contributes to increased cell migration, invasiveness, and resistance to apoptosis (29). Central to this process is the epithelial cell marker E-cadherin and the mesenchymal marker N-cadherin, which play pivotal roles in EMT by facilitating the replacement of epithelial adhesion proteins (e.g., E-cadherin) with those offering greater junctional flexibility (e.g., N-cadherin), thereby promoting cell detachment and migration (12).
Previous studies in malignancies such as pancreatic cancer and colorectal cancer have demonstrated that a mesenchymal phenotype, characterized by reduced expression of epithelial markers and elevated expression of mesenchymal markers, is linked to tumor progression and adverse outcomes (5,30,31). Regarding the mechanism of STAS occurrence, Jia et al. found that the presence of STAS in squamous cell carcinoma and adenocarcinoma of the lung is associated with low expression of E-cadherin (32). Ikeda et al. found that the occurrence of STAS in NSCLC is associated with non-epithelial features, such as low expression or loss of E-cadherin (6). The study by Liu et al. confirmed the association between twist and slug (which act as transcription factors inducing EMT) and the occurrence of STAS (10). Yoshida et al. found that the tumor-associated CD68+ macrophages, CD163+ macrophages, and CD25+ lymphocytes were independently associated with the occurrence of STAS. CD163 serves as a specific marker for M2 macrophages, and the M2 polarization of tumor-associated macrophages (TAMs) promotes the EMT and invasive capacity of cancer cells (33-36). The above studies all indicate a close relationship between the occurrence of STAS and the process of EMT, which may be influenced and regulated by the immune microenvironment and upstream relevant transcription factors. Our study also confirms this viewpoint. Additionally, our study expands on the finding that high expression of N-cadherin is also an independent predictor of STAS, indicating that in lung cancer, not only the loss of epithelial markers but also the increase in mesenchymal cell markers is associated with STAS.
Moreover, previous research has shown that activation of FAK is accompanied by decreased expression of E-cadherin and increased expression of Slug and N-cadherin. Conversely, inhibition of FAK results in the opposite changes in the aforementioned three markers (37-39). Phosphorylation of FAK exacerbates adhesive disruption mediated by E-cadherin, while inhibition of E-cadherin function can further enhance Src activity by promoting FAK phosphorylation, thereby relieving E-cadherin-mediated adhesion and subsequently enhancing tumor cell motility. These findings collectively suggest active involvement of the FAK pathway in the EMT process (40). Based on our study findings, we observed that STAS is not only associated with the expression levels of E-cadherin and N-cadherin but also independently correlated with high FAK expression. This suggests that the development and progression of STAS may be related not only to the abnormal activation of the EMT process and alterations in epithelial adhesion function but also to increased cell migration and cytoskeletal reorganization mediated by the integrin-FAK pathway. FAK may serve as an upstream regulatory molecule associated with the occurrence of STAS, but caution is warranted in interpreting these results. Further in-depth research is warranted to elucidate the specific mechanisms by which FAK regulates EMT activation and promotes STAS occurrence in patients with NSCLC.
Sub-lobar resection is a recognized factor influencing poor prognosis in STAS-positive patients (1,41,42). Although our study did not observe any differences in RFP associated with surgical procedures, we still believe that the results of previous studies should be prioritized. Additionally, our study developed novel risk indices. We found that high-risk indices (comprising low E-cadherin/high N-cadherin, low E-cadherin/high FAK, and high N-cadherin/high FAK combinations) independently predict recurrence. Furthermore, the high N-cadherin/high FAK combination remains a risk factor for recurrence in NSCLC patients with STAS after adjusting for confounding factors such as surgical procedures, visceral pleural invasion, age, and sex. Moreover, we did not observe any correlation between the risk indices, composed of E-cadherin, N-cadherin, FAK, and their combinations, and surgical procedures.
Building on previous research, we speculated that in the future, combining FAK inhibitors with monoclonal antibodies or modulators targeting relevant adhesion molecules (such as upregulators or activators of E-cadherin) may have potential applications for patients with STAS-positive NSCLC (43-45). Small molecule FAK inhibitors may exert their effects by directly or indirectly inhibiting aberrant EMT activation. Additionally, FAK inhibitors may impact the immune microenvironment, enhancing tumor sensitivity to T cells and promoting the infiltration of anti-tumor immune cells into the tumor, thereby reshaping the tumor microenvironment (46,47). However, these potential applications still face many challenges that need to be addressed. Currently, FAK inhibitors are still in the early stages of research, with limited studies on their use in early-stage NSCLC treatment. Additionally, the production of human monoclonal antibodies as therapeutic agents poses numerous challenges and is costly. Further research is needed in the future to validate the possibility of these applications.
Many studies have indicated an association between STAS and the EMT process, consistent with our findings. However, Yagi et al. observed E-cadherin expression in the STAS regions attached to the alveolar wall in three micropapillary (MIP) samples and suggested that tumor cells in STAS may reattach to the alveolar wall via a vascular co-selection mechanism (48). This challenges the view that STAS cells undergo EMT. We speculate that when STAS lesions detach from the primary tumor and form free tumor cell clusters, they may undergo abnormal EMT activation processes, leading to reduced cell adhesion and tumor budding, indicative of characteristic EMT activation. However, upon reattachment to the alveolar wall, STAS lesions may undergo a mesenchymal-epithelial transition (MET), promoting tumor growth, hence the expression of E-cadherin observed in the attached STAS regions (49,50). Therefore, elucidating the mechanisms underlying STAS occurrence and re-implantation, which lead to increased risk of recurrence in NSCLC patients, remains an area requiring further investigation.
This study has several limitations. Firstly, it is retrospective and covers a limited sample size from a single center, potentially introducing selection bias. Therefore, expanding the study to a multicenter environment may enhance the external validity and generalizability of the findings. Additionally, the method of assessing adhesion molecule expression using immunohistochemistry alone for patient stratification in clinical settings may be constrained by the time limitations of surgical approach selection, thus affecting a comprehensive evaluation of treatment plans. Future research could employ methods such as real-time quantitative polymerase chain reaction (PCR), ribonucleic acid (RNA) sequencing, mass spectrometry, etc., to quantitatively assess adhesion molecule expression in preoperative biopsy and postoperative tumor specimens, to ascertain the feasibility of patient risk stratification before surgery. Thirdly, our study suggests an association between the occurrence of STAS and tumor recurrence with the expression levels of relevant adhesion molecules. However, previous research has indicated that inhibiting E-cadherin function may also induce the occurrence of the EMT process. Therefore, basic and clinical research on inhibiting or activating the function of relevant adhesion molecules may be a future research direction, such as using specific antibodies for blockade or activation, to comprehensively explore the relationship between the mechanism of STAS occurrence and adhesion molecules.
Conclusions
In patients with stage I NSCLC, our study identified significant associations between the presence of E-cadherin, N-cadherin, and FAK with the occurrence of STAS. The combined high-risk indices of these markers were highly correlated with an increased risk of recurrence. Additionally, high N-cadherin/FAK expression in tumors provides information about a higher risk of recurrence in STAS-positive stage I lung carcinoma. Utilizing immunohistochemistry to detect these biomarkers enables the enhanced identification of patients requiring adjuvant therapy or close postoperative monitoring. However, the validation of these findings necessitates extended follow-up periods.
Acknowledgments
We are grateful to the reviewers for their efforts in reviewing this manuscript.
Funding: This work was supported by
Footnote
Reporting Checklist: The authors have completed the TRIPOD reporting checklist. Available at https://tlcr.amegroups.com/article/view/10.21037/tlcr-24-247/rc
Data Sharing Statement: Available at https://tlcr.amegroups.com/article/view/10.21037/tlcr-24-247/dss
Peer Review File: Available at https://tlcr.amegroups.com/article/view/10.21037/tlcr-24-247/prf
Conflicts of Interest: All authors have completed the ICMJE uniform disclosure form (available at https://tlcr.amegroups.com/article/view/10.21037/tlcr-24-247/coif). The authors have no conflicts of interest to declare.
Ethical Statement: The authors are accountable for all aspects of the work in ensuring that questions related to the accuracy or integrity of any part of the work are appropriately investigated and resolved. The study was conducted in accordance with the Declaration of Helsinki (as revised in 2013). The study was approved by the Institutional Review Board of Nanjing Jinling Hospital (No. DBNJ20241) and individual consent for this retrospective analysis was waived.
Open Access Statement: This is an Open Access article distributed in accordance with the Creative Commons Attribution-NonCommercial-NoDerivs 4.0 International License (CC BY-NC-ND 4.0), which permits the non-commercial replication and distribution of the article with the strict proviso that no changes or edits are made and the original work is properly cited (including links to both the formal publication through the relevant DOI and the license). See: https://creativecommons.org/licenses/by-nc-nd/4.0/.
References
- Kadota K, Nitadori JI, Sima CS, et al. Tumor Spread through Air Spaces is an Important Pattern of Invasion and Impacts the Frequency and Location of Recurrences after Limited Resection for Small Stage I Lung Adenocarcinomas. J Thorac Oncol 2015;10:806-14. [Crossref] [PubMed]
- Warth A, Muley T, Kossakowski CA, et al. Prognostic Impact of Intra-alveolar Tumor Spread in Pulmonary Adenocarcinoma. Am J Surg Pathol 2015;39:793-801. [Crossref] [PubMed]
- Masai K, Sakurai H, Sukeda A, et al. Prognostic Impact of Margin Distance and Tumor Spread Through Air Spaces in Limited Resection for Primary Lung Cancer. J Thorac Oncol 2017;12:1788-97. [Crossref] [PubMed]
- Kadota K, Kushida Y, Katsuki N, et al. Tumor Spread Through Air Spaces Is an Independent Predictor of Recurrence-free Survival in Patients With Resected Lung Squamous Cell Carcinoma. Am J Surg Pathol 2017;41:1077-86. [Crossref] [PubMed]
- Chouat E, Zehani A, Chelly I, et al. Tumor budding is a prognostic factor linked to epithelial mesenchymal transition in pancreatic ductal adenocarcinoma. Study report and literature review. Pancreatology 2018;18:79-84. [Crossref] [PubMed]
- Ikeda T, Kadota K, Yoshida C, et al. The epithelial-mesenchymal transition phenotype is associated with the frequency of tumor spread through air spaces (STAS) and a High risk of recurrence after resection of lung carcinoma. Lung Cancer 2021;153:49-55. [Crossref] [PubMed]
- Tan X, Yan Y, Song B, et al. Focal adhesion kinase: from biological functions to therapeutic strategies. Exp Hematol Oncol 2023;12:83. [Crossref] [PubMed]
- Huang Y, Hong W, Wei X. The molecular mechanisms and therapeutic strategies of EMT in tumor progression and metastasis. J Hematol Oncol 2022;15:129. [Crossref] [PubMed]
- Dong B, Li S, Zhu S, et al. MiRNA-mediated EMT and CSCs in cancer chemoresistance. Exp Hematol Oncol 2021;10:12. [Crossref] [PubMed]
- Liu A, Sun X, Xu J, et al. Relevance and prognostic ability of Twist, Slug and tumor spread through air spaces in lung adenocarcinoma. Cancer Med 2020;9:1986-98. [Crossref] [PubMed]
- Niu Y, Han X, Zeng Y, et al. The significance of spread through air spaces in the prognostic assessment model of stage I lung adenocarcinoma and the exploration of its invasion mechanism. J Cancer Res Clin Oncol 2023;149:7125-38. [Crossref] [PubMed]
- Lamouille S, Xu J, Derynck R. Molecular mechanisms of epithelial-mesenchymal transition. Nat Rev Mol Cell Biol 2014;15:178-96. [Crossref] [PubMed]
- Harjunpää H, Llort Asens M, Guenther C, et al. Cell Adhesion Molecules and Their Roles and Regulation in the Immune and Tumor Microenvironment. Front Immunol 2019;10:1078. [Crossref] [PubMed]
- Schnell U, Cirulli V, Giepmans BN. EpCAM: structure and function in health and disease. Biochim Biophys Acta 2013;1828:1989-2001. [Crossref] [PubMed]
- Bui TM, Wiesolek HL, Sumagin R. ICAM-1: A master regulator of cellular responses in inflammation, injury resolution, and tumorigenesis. J Leukoc Biol 2020;108:787-99. [Crossref] [PubMed]
- Kong DH, Kim YK, Kim MR, et al. Emerging Roles of Vascular Cell Adhesion Molecule-1 (VCAM-1) in Immunological Disorders and Cancer. Int J Mol Sci 2018;19:1057. [Crossref] [PubMed]
- Nicholson AG, Tsao MS, Beasley MB, et al. The 2021 WHO Classification of Lung Tumors: Impact of Advances Since 2015. J Thorac Oncol 2022;17:362-87. [Crossref] [PubMed]
- Moreira AL, Ocampo PSS, Xia Y, et al. A Grading System for Invasive Pulmonary Adenocarcinoma: A Proposal From the International Association for the Study of Lung Cancer Pathology Committee. J Thorac Oncol 2020;15:1599-610. [Crossref] [PubMed]
- Uruga H, Fujii T, Fujimori S, et al. Semiquantitative Assessment of Tumor Spread through Air Spaces (STAS) in Early-Stage Lung Adenocarcinomas. J Thorac Oncol 2017;12:1046-51. [Crossref] [PubMed]
- Liu H, Yin Q, Yang G, et al. Prognostic Impact of Tumor Spread Through Air Spaces in Non-small Cell Lung Cancers: a Meta-Analysis Including 3564 Patients. Pathol Oncol Res 2019;25:1303-10. [Crossref] [PubMed]
- Chen D, Mao Y, Wen J, et al. Tumor Spread Through Air Spaces in Non-Small Cell Lung Cancer: A Systematic Review and Meta-Analysis. Ann Thorac Surg 2019;108:945-54. [Crossref] [PubMed]
- Lv Y, Li S, Liu Z, et al. Impact of surgery and adjuvant chemotherapy on the survival of stage I lung adenocarcinoma patients with tumor spread through air spaces. Lung Cancer 2023;177:51-8. [Crossref] [PubMed]
- Shi Q, Boettiger D. A novel mode for integrin-mediated signaling: tethering is required for phosphorylation of FAK Y397. Mol Biol Cell 2003;14:4306-15. [Crossref] [PubMed]
- Avizienyte E, Wyke AW, Jones RJ, et al. Src-induced de-regulation of E-cadherin in colon cancer cells requires integrin signalling. Nat Cell Biol 2002;4:632-8. [Crossref] [PubMed]
- McLean GW, Carragher NO, Avizienyte E, et al. The role of focal-adhesion kinase in cancer - a new therapeutic opportunity. Nat Rev Cancer 2005;5:505-15. [Crossref] [PubMed]
- Yilmaz M, Christofori G. EMT, the cytoskeleton, and cancer cell invasion. Cancer Metastasis Rev 2009;28:15-33. [Crossref] [PubMed]
- Wang T, Rao D, Yu C, et al. RHO GTPase family in hepatocellular carcinoma. Exp Hematol Oncol 2022;11:91. [Crossref] [PubMed]
- Krugmann S, Jordens I, Gevaert K, et al. Cdc42 induces filopodia by promoting the formation of an IRSp53:Mena complex. Curr Biol 2001;11:1645-55. [Crossref] [PubMed]
- Kalluri R, Weinberg RA. The basics of epithelial-mesenchymal transition. J Clin Invest 2009;119:1420-8. [Crossref] [PubMed]
- De Smedt L, Palmans S, Andel D, et al. Expression profiling of budding cells in colorectal cancer reveals an EMT-like phenotype and molecular subtype switching. Br J Cancer 2017;116:58-65. [Crossref] [PubMed]
- Zlobec I, Lugli A. Epithelial mesenchymal transition and tumor budding in aggressive colorectal cancer: tumor budding as oncotarget. Oncotarget 2010;1:651-61. [Crossref] [PubMed]
- Jia M, Yu S, Yu J, et al. Comprehensive analysis of spread through air spaces in lung adenocarcinoma and squamous cell carcinoma using the 8th edition AJCC/UICC staging system. BMC Cancer 2020;20:705.
- Yoshida C, Kadota K, Ikeda T, et al. Tumor-associated macrophage infiltration is associated with a higher rate of tumor spread through air spaces in resected lung adenocarcinomas. Lung Cancer 2021;158:91-6. [Crossref] [PubMed]
- Yoshida C, Kadota K, Yamada K, et al. Tumor-associated CD163(+) macrophage as a predictor of tumor spread through air spaces and with CD25(+) lymphocyte as a prognostic factor in resected stage I lung adenocarcinoma. Lung Cancer 2022;167:34-40. [Crossref] [PubMed]
- Evrard D, Szturz P, Tijeras-Raballand A, et al. Macrophages in the microenvironment of head and neck cancer: potential targets for cancer therapy. Oral Oncol 2019;88:29-38. [Crossref] [PubMed]
- Xiao P, Long X, Zhang L, et al. Neurotensin/IL-8 pathway orchestrates local inflammatory response and tumor invasion by inducing M2 polarization of Tumor-Associated macrophages and epithelial-mesenchymal transition of hepatocellular carcinoma cells. Oncoimmunology 2018;7:e1440166. [Crossref] [PubMed]
- Chen KS, Shi MD, Chien CS, et al. Pinocembrin suppresses TGF-β1-induced epithelial-mesenchymal transition and metastasis of human Y-79 retinoblastoma cells through inactivating αvβ3 integrin/FAK/p38α signaling pathway. Cell Biosci 2014;4:41. [Crossref] [PubMed]
- Datta A, Loo SY, Huang B, et al. SPHK1 regulates proliferation and survival responses in triple-negative breast cancer. Oncotarget 2014;5:5920-33. [Crossref] [PubMed]
- Xu CY, Liu SQ, Qin MB, et al. SphK1 modulates cell migration and EMT-related marker expression by regulating the expression of p-FAK in colorectal cancer cells. Int J Mol Med 2017;39:1277-84. [Crossref] [PubMed]
- Alt-Holland A, Sowalsky AG, Szwec-Levin Y, et al. Suppression of E-cadherin function drives the early stages of Ras-induced squamous cell carcinoma through upregulation of FAK and Src. J Invest Dermatol 2011;131:2306-15. [Crossref] [PubMed]
- Shiono S, Endo M, Suzuki K, et al. Spread Through Air Spaces Is a Prognostic Factor in Sublobar Resection of Non-Small Cell Lung Cancer. Ann Thorac Surg 2018;106:354-60. [Crossref] [PubMed]
- Zombori T, Sejben A, Tiszlavicz L, et al. Architectural Grade Combined With Spread Through Air Spaces (STAS) Predicts Recurrence and is Suitable for Stratifying Patients Who Might Be Eligible for Lung Sparing Surgery for Stage I Adenocarcinomas. Pathol Oncol Res 2020;26:2451-8. [Crossref] [PubMed]
- Gerber DE, Camidge DR, Morgensztern D, et al. Phase 2 study of the focal adhesion kinase inhibitor defactinib (VS-6063) in previously treated advanced KRAS mutant non-small cell lung cancer. Lung Cancer 2020;139:60-7. [Crossref] [PubMed]
- Yayan J, Franke KJ, Berger M, et al. Adhesion, metastasis, and inhibition of cancer cells: a comprehensive review. Mol Biol Rep 2024;51:165. [Crossref] [PubMed]
- Na TY, Schecterson L, Mendonsa AM, et al. The functional activity of E-cadherin controls tumor cell metastasis at multiple steps. Proc Natl Acad Sci U S A 2020;117:5931-7. [Crossref] [PubMed]
- Qiao M, Zhou F, Liu X, et al. Targeting focal adhesion kinase boosts immune response in KRAS/LKB1 co-mutated lung adenocarcinoma via remodeling the tumor microenvironment. Exp Hematol Oncol 2024;13:11. [Crossref] [PubMed]
- Gao J, Cheng J, Xie W, et al. Prospects of focal adhesion kinase inhibitors as a cancer therapy in preclinical and early phase study. Expert Opin Investig Drugs 2024;33:639-51. [Crossref] [PubMed]
- Yagi Y, Aly RG, Tabata K, et al. Three-Dimensional Histologic, Immunohistochemical, and Multiplex Immunofluorescence Analyses of Dynamic Vessel Co-Option of Spread Through Air Spaces in Lung Adenocarcinoma. J Thorac Oncol 2020;15:589-600. [Crossref] [PubMed]
- Chao YL, Shepard CR, Wells A. Breast carcinoma cells re-express E-cadherin during mesenchymal to epithelial reverting transition. Mol Cancer 2010;9:179. [Crossref] [PubMed]
- Wells A, Yates C, Shepard CR. E-cadherin as an indicator of mesenchymal to epithelial reverting transitions during the metastatic seeding of disseminated carcinomas. Clin Exp Metastasis 2008;25:621-8. [Crossref] [PubMed]