Predictive value of longitudinal systemic inflammatory markers for pathologic response to neoadjuvant PD-1 blockade in resectable non-small cell lung cancer
Highlight box
Key findings
• This study proposed that combined systemic inflammatory markers demonstrated predictive value in stratifying pathologic response to neoadjuvant sintilimab in resectable non-small cell lung cancer (NSCLC). The high inflammatory burden group had the lowest pathologic regression and the pre-treatment immunosuppressive microenvironment with macrophage enrichment.
What is known and what is new?
• Cancer-related inflammation plays a key role in tumor progression. A few studies have reported that patients with high systemic inflammatory burden are associated with poor efficacy and prognosis.
• Based on the hypothesis that circulating and imaging inflammatory markers could serve as indicators for the anti-tumor immune responses, we reported the predictive value of longitudinal systemic inflammatory markers for pathologic response to resectable NSCLC in the neoadjuvant immunotherapy setting, and explored the relationship between host inflammatory burden and tumor-infiltrating immune cells in the microenvironment.
What is the implication, and what should change now?
• Longitudinal systemic inflammatory markers from peripheral blood and positron emission tomography/computed tomography can stratify pathologic response to resectable NSCLC in neoadjuvant immunotherapy setting, assisting in patients selection with potential benefits. Our findings serve as preliminary data to encourage prospective studies to further explore the pathophysiological mechanisms involving systemic inflammatory markers and their association with the tumor immune microenvironment and clinical outcomes.
Introduction
Lung cancer, particularly non-small cell lung cancer (NSCLC), is the most common cause of cancer-related death worldwide (1). In recent years, clinical trials on neoadjuvant immunotherapy for resectable NSCLC have been conducted with encouraging results (2-5), but not all patients responded satisfactorily. The major pathologic response (MPR) is considered as a vital short-term endpoint that has potential to replace survival outcomes in neoadjuvant immunotherapy setting. Identification of biomarkers to predict responses has become an area of intensive researches.
The links among cancer, inflammation, and immunosuppression are well recognised (6), and cancer-related inflammation plays a key role in tumor progression (7-9). A few studies have reported that a high systemic inflammatory burden is associated with poor efficacy and prognosis (10-13), whereas immune activation is associated with better efficacy and prognosis (14,15). However, the role of inflammatory markers in neoadjuvant immunotherapy remains inconclusive. In addition, tumor-infiltrating immune cells within the tumor immune microenvironment (TIME) play important roles in anti-tumor immunity, particularly CD8+ T cell (16). However, overall and dynamic detection of TIME biomarkers remains challenging.
We have previously demonstrated that metabolic parameters of primary tumor can predict MPR to neoadjuvant sintilimab in resectable NSCLC (17). Considering the interplay between cancer, inflammation, and immunosuppression, we further conducted an exploratory study to reveal the predictive value of combining longitudinal systemic inflammatory markers in stratifying pathologic response. We hypothesised that circulating and imaging inflammatory markers would serve as indicators for the anti-tumor immune responses. Peripheral blood tests and 18F-fluorodeoxyglucose (18F-FDG) positron emission tomography/computed tomography (PET/CT) scans were used to assess the systemic inflammatory burden. Notably, as peripheral blood is susceptible to infection and other factors, we focused on the combined effect of lymphoid organs metabolism (spleen and bone marrow) to comprehensively reflect the systemic inflammatory burden of the host.
Based on peripheral blood tests and 18F-FDG PET/CT scans, the objective of this study was to explore the predictive value of longitudinal systemic inflammatory markers to stratify the pathologic response to resectable NSCLC in neoadjuvant immunotherapy setting. Moreover, we explored the relationship between systemic inflammatory markers and tumor-infiltrating immune cells to provide potential non-invasive surrogate markers for TIME information. We present this article in accordance with the TRIPOD reporting checklist (available at https://tlcr.amegroups.com/article/view/10.21037/tlcr-24-598/rc).
Methods
Data source
Patients were selected from a prospective, single-center, single-arm, phase Ib trial (registration number: ChiCTR-OIC-17013726). From March 6, 2018 to March 8, 2019, a total of 40 patients with resectable NSCLC were enrolled in the trial and 36 patients who underwent pre- and post-treatment peripheral blood tests and 18F-FDG PET/CT scans before and after two cycles of neoadjuvant sintilimab were enrolled in this study. Four patients were excluded: two of them for the baseline PET/CT which was conducted in other hospital, and two of them for the pathologic regression of primary tumor that could not be assessed by the exploratory surgery. Eligible patients were those aged 18–75 years who had histologically or cytologically confirmed NSCLC [stage IA–IIIB, American Joint Committee on Cancer (AJCC) 8th] that was surgically resectable. All patients were treatment-naive and had a primary tumor with diameter ≥2 cm. The exclusion criteria were previous anti-tumor therapy; epidermal growth factor receptor-sensitive mutation; systemic immunosuppressive therapy within 4 weeks prior to study treatment; active and uncontrolled infection; history of interstitial lung disease; known or suspected active autoimmune diseases; hypersensitivity to any monoclonal antibodies; and presence of other known malignant tumor. The complete eligibility criteria (inclusion and exclusion criteria) were available in our previous publications (3,17). The eligible patients received two cycles of sintilimab (200 mg, intravenously, day 1 and 22), and complete tumor resection or biopsy (to confirm tumor progression) was performed for pathological evaluation within 29–43 days after the first dose. Data were extracted from the medical records.
The study was conducted in accordance with the Declaration of Helsinki (as revised in 2013) and was approved by the Ethics Committee and Institutional Review Board of the National Cancer Center/Cancer Hospital, Chinese Academy of Medical Sciences and Peking Union Medical College (No. 17-151/1407). Written informed consent was obtained from all patients.
18F-FDG PET/CT acquisition
Three-dimensional (3D) whole-body PET/CT scans from head to thigh were performed using an integrated PET/CT device (Discovery 690; GE Healthcare, Milwaukee, Wisconsin, USA), according to the standard protocol. Prior to the scan, the patients fasted for at least 6 h, and the blood glucose level was required to be <145 mg/dL. PET/CT scans were acquired approximately 50–70 min after intravenous administration of 18F-FDG at a mean dose of 3.70–4.44 MBq/kg. The difference in the administration dose was <20%, and the difference in uptake time was <15 min between the pre- and post-treatment PET/CT scans. PET images were obtained in 3D image-acquisition mode using 2 min per frame (generally 7–8 beds location) and reconstructed using the VPFX-S algorithm (two iterations, 24 subsets, 4 mm Gaussian post-filter). Spiral CT was performed using a standardised protocol (tube voltage, 120 kV; tube current, 150 mA; slice thickness, 3.75 mm; and rotation speed, 0.8 s) without intravenous contrast administration. A breath-hold thoracic spiral CT scan (tube voltage, 120 kV; tube current using the automatic milliampere-seconds technology; slice thickness, 5 mm; and rotation speed, 0.5 s) was performed after the PET/CT scan with image reconstruction (slice thickness, 1.25 mm; space, 0.8 mm).
Image analysis
The PET/CT images were retrospectively assessed and interpreted by two radiologists who were blinded to clinical data. Disagreements were resolved through consensus. PET/CT images were analysed using PETVCAR (PET Volume Computerized Assisted Reporting), an automated segmentation software system with an iterative adaptive algorithm for detecting the threshold levels on an advantage workstation (version 4.6; GE Healthcare).
Inflammatory markers in spleen and bone marrow metabolism
Spleen and bone marrow metabolism were normalized by computing the spleen-to-liver ratio (SLR) and the bone marrow-to-liver ratio (BLR), respectively. Prigent et al. (18) reported the interobserver variability and confirmed that the robust metrics with strong reproducibility were the mean standardized uptake value (SUV) of the spleen and bone marrow measurements (SLRmean and BLRmean). Therefore, the SLR and BLR were calculated by dividing the spleen SUVmean by the liver SUVmean and the bone marrow SUVmean by liver SUVmean, respectively. The measurement methods of volume of interest (VOI) were consistent with previous studies (Figure S1) (18-20). The SUVmean of the liver and spleen were computed from a spherical VOI 30 mm in diameter on the right liver lobe and a spherical VOI 20 mm in diameter at the center of the spleen. For the bone marrow, four spherical VOIs of 15 mm diameter were placed in the center of the L1 to L4 (lumbar) vertebral bodies, whereas VOIs with vertebral fractures, severe lumbar osteoarthritis, metastatic lesions, hemangiomas, or history of lumbar spine surgery were excluded. The SUVmean of the bone marrow was defined as the average SUVmean of all the vertebral bodies.
Metabolic tumor burden of the primary tumor
The VOI of the primary tumor was auto-contoured and segmented using a 3D cube that contained all FDG PET-positive areas and excluded negative normal tissues in the axial, coronal, and sagittal planes. The following SUVs corrected by lean body mass (SUL) were calculated: SULmax, SULmean, SULpeak, metabolic tumor volume (MTV), and total lesion glycolysis (TLG). Detailed information was available in our previous publication (17).
Immune-related adverse events (irAEs) detected on PET/CT
irAEs on PET/CT were visually defined as a diffuse and homogeneous increase in organ uptake that was newly emerging or markedly increased compared to the baseline image, or an increase in organ uptake that was quantitatively assessed (SUVpeak increase >30%) compared to the baseline image, which was consistent with the definition from a previous study (21). Diffuse inflammatory uptake of FDG for each organ (pneumonitis, thyroiditis, mediastinal granulomatous reaction, gastritis, colitis, hepatitis, pancreatitis, osteoarticular inflammation, cutaneous inflammation, pleuritis, etc.) was collected separately. Notably, there was no correspondence between clinically reported irAEs and irAEs on PET/CT. Clinical irAEs were not the focus of this study.
Inflammatory markers in peripheral blood
Complete blood cell counts were centrally tested in our institutional laboratory in accordance with standardized operative procedures. Records were obtained from the reports. Absolute neutrophil and lymphocyte counts were collected. The neutrophil-to-lymphocyte ratio (NLR) was calculated as the ratio of the absolute neutrophil count to the absolute lymphocyte count.
Pathological assessment
Routine hematoxylin and eosin staining were performed to assess the percentage of viable residual tumors in the primary tumor. The method for assessing pathologic responses was described in our previous publication (22). The degree of pathologic regression was recorded. Tumors with ≤10% viable tumor cells were considered to achieve MPR.
Detection of tumor-infiltrating immune cells
Multiplex immunofluorescence (mIF) staining was used to detect tumor-infiltrating immune cells. The slides were scanned using a PerkinElmer Vectra imaging system (Vectra 3.0.5; PerkinElmer, Waltham, Massachusetts, USA). The images of tissues stained separately with each reagent were used to construct spectral libraries, and the multispectral images were unmixed by the spectral libraries using the inForm Advanced Image Analysis software (inForm 2.3.0; PerkinElmer). The total infiltration abundance of immune cells (percentage of positively stained immune cells) was calculated by dividing the number of positively stained cells by the total number of nuclear cells. The detailed protocol for the mIF staining assay and data on the total region infiltration abundance of immune cells were available in our previous publication (23). Owing to insufficient adhesion causing samples to fall off the slide, among the 36 patients enrolled in the study, paired staining data from pre- and post-neoadjuvant therapy were available for 26 patients in the panel (CD8, PD-1), 23 patients in the panel (CD4, FOXP3), and 23 patients in the panel (CD56, CD19, CD68, CD163).
Statistical analysis
The variation was defined as the percentage change from pre- to post-neoadjuvant sintilimab. The calculation formula is as follows: the variation = (XPost − XPre)/XPre × 100%. Comparisons between MPR and non-MPR patients were evaluated using Fisher’s exact test for categorical variables and the Mann-Whitney U test for continuous variables. The predictive value of systemic inflammatory markers for MPR was calculated using receiver operating characteristic (ROC) analysis. The area under the ROC curve (AUC), sensitivity, specificity, and accuracy were calculated. Moreover, significant variables divided patients into three groups (low, intermediate, and high inflammatory burden groups). The Kruskal-Wallis test was used to compare differences in pathologic regression and pre-treatment infiltration abundance of tumor-infiltrating immune cells among the three groups. A post-hoc test with Bonferroni correction was performed for multiple comparisons.
The correlation between systemic inflammatory markers, primary tumor metabolism, and tumor-infiltrating immune cells abundance at various time points (pre-treatment, post-treatment, and variation) was determined using Spearman’s correlation analysis. Available case analyses were used for the missing data.
Statistical analyses were performed using SPSS (version 25.0; IBM Corp.) and R (version 4.0.3). All reported P values were two-sided, and P values <0.05 were considered statistically significant. Considering the preliminary and exploratory nature of the study with its small sample size, the data obtained should be interpreted with caution and regarded as hypothesis-generating.
Results
Clinical characteristics of the patients
Among the 36 enrolled patients (29 male and 7 female; median age, 61 years), 35 patients underwent radical resection of the primary tumor after neoadjuvant sintilimab with different degrees of pathologic regression. None of the 35 patients had distant metastases. Only one patient did not undergo primary tumor resection, and was confirmed tumor progression by pleural biopsy of a new metastasis with the pathologic regression considered as 0. The median pathologic regression rate for all enrolled patients was 42.5% (0–100%). Overall, 36.1% (13/36) patients achieved MPR and 63.9% (23/36) patients did not achieve MPR. The patient characteristics are summarized in Table 1, and the study flowchart is presented in Figure 1. Descriptive statistics on the distribution and variation of systemic inflammatory markers and primary tumor metabolism before and after neoadjuvant sintilimab were detailed in Figure S2. According to our previous study, there was no significant correlation between the baseline characteristics of the patients in terms of age, sex, histology, smoking history, clinical stage, and MPR (17).
Table 1
Clinical characteristics | Median [range]/n (%) |
---|---|
Age (years) | 61 [48–70] |
Sex | |
Male | 29 (80.6) |
Female | 7 (19.4) |
Histology | |
Adenocarcinoma | 6 (16.7) |
Squamous cell carcinoma | 29 (80.6) |
Mixed | 1 (2.8) |
Smoking history | |
Never | 8 (22.2) |
Former or current | 28 (77.8) |
Pack-years | 25 [0–100] |
Clinical stage at baseline† | |
Ia | 1 (2.8) |
Ib | 6 (16.7) |
IIb | 12 (33.3) |
IIIa | 9 (25.0) |
IIIb | 8 (22.2) |
†, the clinical stage at baseline was based on the criteria of the American Joint Committee on Cancer, eighth edition.
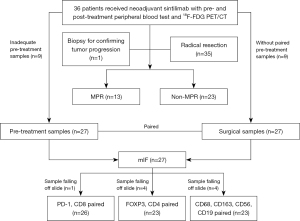
ΔNLR% and pre- and post-treatment SLRs correlate with both MPR and pathologic regression
The relationship between pre- and post-treatment systemic inflammatory markers and pathologic responses is summarized in Table 2 and Table S1. The ΔNLR% in the MPR group was significantly lower than that in the non-MPR group (P=0.047). The ΔNLR% was significantly negatively correlated with pathologic regression (r=−0.34, P=0.045). Further analyses indicated that the dominant significant effect was attributable to the absolute neutrophil count (Δ%). The absolute neutrophil count (Δ%) had significant effects on both MPR and pathologic regression (both P=0.03; Tables S2,S3). The pre- and post-treatment SLRs in patients with MPR tended to be significantly lower than those in patients without MPR, which almost reached significance (P=0.06; P=0.055). The pre- and post-treatment SLRs tended to be negatively correlated with pathologic regression, which almost reached significance (r=−0.30, P=0.07; r=−0.31, P=0.06).
Table 2
Systemic inflammatory markers | Patients with MPR (n=13), median [range] | Patients without MPR (n=23), median [range] |
P value |
---|---|---|---|
Pre-treatment | |||
NLR | 2.9 [1.5, 6.6] | 2.8 [1.0, 10.3] | >0.99 |
SLR | 0.8 [0.7, 1.1] | 0.9 [0.7, 1.3] | 0.06 |
BLR | 0.8 [0.6, 1.6] | 0.9 [0.4, 2.3] | 0.18 |
Post-treatment | |||
NLR | 2.1 [0.9, 4.2] | 2.3 [1.2, 12.0] | 0.23 |
SLR | 0.8 [0.7, 0.9] | 0.9 [0.5, 1.1] | 0.055 |
BLR | 0.8 [0.6, 0.9] | 0.8 [0.5, 2.4] | 0.33 |
Percentage variation (Δ%) | |||
ΔNLR% | −22.7 [−74.6, 33.0] | −8.0 [−60.3, 224.5] | 0.047 |
ΔSLR% | 0.1 [−27.6, 9.8] | −1.1 [−38.8, 19.4] | 0.95 |
ΔBLR% | −4.9 [−42.3, 46.2] | −5.8 [−44.2, 47.7] | 0.63 |
MPR, major pathologic response; NLR, neutrophil-to-lymphocyte ratio; SLR, spleen-to-liver ratio; BLR, bone marrow-to-liver ratio.
ROC analyses indicated that the ΔNLR% (AUC =0.702), pre-treatment SLR (AUC =0.689), and post-treatment SLR (AUC =0.694) exhibited the best differentiation ability for MPR at thresholds of −17%, 0.83, and 0.88, respectively (Table 3). Significant variables with convenient integration into clinical routines (ΔNLR% and pre-treatment SLR) divided patients into three groups. Considering the significant collinearity between pre- and post-treatment SLRs, the post-treatment SLR was not included in the classification. Patients were divided into three groups as follows: (I) the low inflammatory burden group (pre-treatment SLR ≤0.83 and ΔNLR% ≤−17%); (II) the intermediate inflammatory burden group (pre-treatment SLR ≤0.83 and ΔNLR% >−17%; pre-treatment SLR >0.83 and ΔNLR% ≤−17%); and (III) the high inflammatory burden group (pre-treatment SLR >0.83 and ΔNLR% >−17%). As shown in Figure 2A, there were significant differences in pathologic regression among the three groups (P=0.01). The high inflammatory burden group exhibited significantly lower pathologic regression than the low inflammatory burden group (P=0.009).
Table 3
Systemic inflammatory markers | Threshold | AUC (95% CI) | Sensitivity | Specificity | Accuracy | P value |
---|---|---|---|---|---|---|
ΔNLR% | −17 | 0.702 (0.529–0.876) | 0.692 | 0.696 | 0.694 | 0.046 |
Pre-treatment SLR | 0.83 | 0.689 (0.496–0.882) | 0.615 | 0.783 | 0.722 | 0.06 |
Post-treatment SLR | 0.88 | 0.694 (0.522–0.866) | 0.846 | 0.565 | 0.667 | 0.056 |
MPR, major pathologic response; AUC, area under the receiver operating characteristic curve; CI, confidence interval; NLR, neutrophil-to-lymphocyte ratio; SLR, spleen-to-liver ratio.
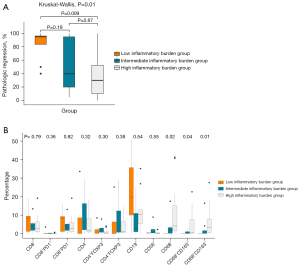
Evaluation of tumor-infiltrating immune cells in the TIME
We sought to determine whether pre-treatment tumor-infiltrating immune cells in the TIME differed among the three groups. As shown in Figure 2B, the infiltration abundance of macrophages (CD68+, CD68+CD163+, and CD68+CD163−) differed significantly among the three groups (P=0.01–0.04). In particular, the infiltration abundance of macrophages (CD68+, CD68+CD163+, and CD68+CD163−) was significantly higher in the high than in the low inflammatory burden group. There were no significant differences in the infiltration of CD8+ T cells, CD4+ T cells, CD19+ B cells, and CD56+ NK cells among the three groups. Figure 3 showed the representative PET/CT and pre-treatment CD68 staining images of patients with the low and high inflammatory burden.
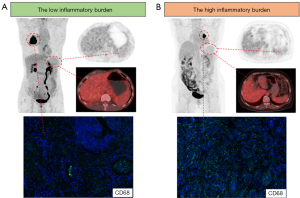
Subsequently, we explored the correlation of tumor-infiltrating immune cells abundance with systemic inflammatory markers and primary tumor metabolism at various time points (pre-treatment, post-treatment, and variation). As shown in Figure 4, the post-treatment SLR showed a significant positive correlation with CD68+ and CD68+CD163− macrophages (r=0.53 and 0.52, P=0.009 and 0.01). The ΔBLR% was significantly negatively correlated with ΔCD19+ B cells (r=−0.63, P=0.001). The ΔSULmean% was significantly negatively correlated with ΔCD8+ and ΔCD8+PD1− T cells (r=−0.40 and −0.42, P=0.04 and 0.03).
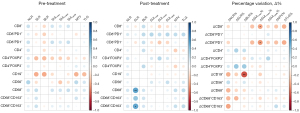
Correlations between systemic inflammatory markers and primary tumor metabolism
The correlations between systemic inflammatory markers and primary tumor metabolism at various time points (pre-treatment, post-treatment, and variation) were detailed in Figure S3. For systemic inflammatory markers, the pre-treatment BLR was positively correlated with the pre-treatment SLR, and ΔBLR% was positively correlated with both ΔSLR% and ΔNLR%. Various metabolic parameters of the primary tumor exhibited significant positive correlations with systemic inflammatory markers. Specifically, in the pre-treatment phase, MTV and TLG were positively correlated with the NLR, SLR, and BLR; in the post-treatment phase, the SULmax, SULmean, SULpeak, MTV, and TLG were positively correlated with the SLR; during the variation phase, ΔSULmax%, ΔSULmean%, ΔSULpeak%, and ΔTLG% were positively correlated with ΔNLR%. Significant collinearity was observed among the primary tumor metabolic parameters.
Association between irAEs on PET/CT with MPR and pathologic regression
Of the 36 enrolled patients, 24 (66.7%) displayed at least one site of irAEs, including 10 (27.8%) with thyroiditis, 9 (25.0%) with pneumonitis, 8 (22.2%) with mediastinal granulomatous reaction, 4 (11.1%) with gastritis, 5 (13.9%) with colitis, 2 (5.6%) with osteo-articular inflammation, 2 (5.6%) with cutaneous inflammation, and 1 (2.8%) with pleuritis. Occurrence of irAEs on PET/CT did not have significant effects on both MPR and pathologic regression in the overall and per-organ statistical analyses (Tables 4,5).
Table 4
irAEs on PET/CT | All patients (n=36) | Patients with MPR (n=13) | Patients without MPR (n=23) | P value |
---|---|---|---|---|
All organs | 0.47 | |||
Yes | 24 | 10 | 14 | |
No | 12 | 3 | 9 | |
Pneumonitis | 0.44 | |||
Yes | 9 | 2 | 7 | |
No | 27 | 11 | 16 | |
Thyroiditis | 0.12 | |||
Yes | 10 | 6 | 4 | |
No | 26 | 7 | 19 | |
Mediastinal granulomatous reaction | 0.42 | |||
Yes | 8 | 4 | 4 | |
No | 28 | 9 | 19 | |
Gastritis | >0.99 | |||
Yes | 4 | 1 | 3 | |
No | 32 | 12 | 20 | |
Colitis | >0.99 | |||
Yes | 5 | 2 | 3 | |
No | 31 | 11 | 20 | |
Other organs | 0.14 | |||
Yes | 5 | 0 | 5 | |
No | 31 | 13 | 18 |
All organs: refer to the occurrence of irAEs on PET/CT displayed at least one organ. Other organs: 2 patients had osteo-articular inflammation; 2 patients had cutaneous inflammation; 1 patient had pleuritis. irAEs, immune-related adverse events; PET/CT, positron emission tomography/computed tomography; MPR, major pathologic response.
Table 5
irAEs on PET/CT | Pathologic regression (%), median [range] |
P value |
---|---|---|
All organs | 0.34 | |
Yes | 55 [0–100] | |
No | 40 [5–100] | |
Pneumonitis | 0.57 | |
Yes | 30 [10–100] | |
No | 45 [0–100] | |
Thyroiditis | 0.45 | |
Yes | 92.5 [0–100] | |
No | 40 [5–100] | |
Mediastinal granulomatous reaction | 0.27 | |
Yes | 75 [10–100] | |
No | 40 [0–100] | |
Gastritis | 0.54 | |
Yes | 37.5 [5–95] | |
No | 47.5 [0–100] | |
Colitis | 0.97 | |
Yes | 40 [5–100] | |
No | 45 [0–100] | |
Other organs | 0.06 | |
Yes | 20 [0–60] | |
No | 50 [5–100] |
All organs: refer to the occurrence of irAEs on PET/CT displayed at least one organ. Other organs: 2 patients had osteo-articular inflammation; 2 patients had cutaneous inflammation; 1 patient had pleuritis. irAEs, immune-related adverse events; PET/CT, positron emission tomography/computed tomography.
Discussion
This study provided a promising novel insight into the predictive value of longitudinal systemic inflammatory markers to stratify pathologic response to neoadjuvant immunotherapy. The main findings were as follows: first, ΔNLR% emerged as a significant negative predictor of MPR and negatively correlated with pathologic regression. Pre- and post-treatment SLRs were potential negative predictors of MPR and negatively correlated with pathologic regression. Second, the combination of pre-treatment SLR and ΔNLR% allowed oncologists to noninvasively classify patients into three groups, and patients in the high inflammatory burden group exhibiting the lowest pathologic regression showing the pre-treatment immunosuppressive microenvironment with macrophage enrichment. Finally, the emergence of irAEs on PET/CT did not have significant effects on MPR and pathologic regression in the overall and per-organ analyses.
An elevated NLR indicates neutrophilia, lymphopenia, or both, physiologically indicating the relative absence of anti-tumor immunity (24). Studies have shown that the NLR was an important negative biomarker for the prediction and prognosis of immunotherapy response, as a high NLR (both pre- and post-treatment) and elevated NLR during variation were associated with poor clinical outcomes (10,15). In our study, ΔNLR% was a significant negative predictor of pathologic regression after neoadjuvant immunotherapy. Further analyses indicated that the dominant significant effect was attributable to the absolute neutrophil count (Δ%). Studies have also supported the negative prognostic role of pre-treatment and variations in absolute neutrophil counts for immunotherapy (25,26). Recently, Kargl et al. (27) reported that neutrophils dominated the immune landscape of NSCLC; although many mechanisms were unknown, high levels of neutrophils were thought to be primarily involved in tumorigenesis and cancer proliferation and were responsible for treatment failure of immunotherapy (28).
Determining the role of lymphoid organ metabolism in immunotherapy has proven challenging because the results published to date have been inconclusive for various tumors and have not been fully studied in NSCLC. Our study showed a trend that higher pre- and post-treatment SLRs appeared to be associated with poorer pathologic response, almost reaching statistical significance. This finding was consistent with those of previous studies demonstrating that a high SLR was associated with unfavorable patient outcomes (13,29). In contrast, Jin et al. (11) reported that an elevated SLR after immunotherapy was significantly correlated with longer survival. Lang et al. (12) proposed that a high BLR, rather than a high SLR, was associated with poor clinical outcomes. Some studies have also suggested that both a high SLR and BLR were associated with poor clinical outcomes (11,30,31). Indeed, the SLR and BLR presented a strong positive correlation (30), supporting the hypothesis of a pathophysiological mechanism and a common systemic inflammatory state throughout the whole body.
This exploratory study proposed a promising new method that combined the pre-treatment SLR and ΔNLR% to effectively stratify pathologic response to neoadjuvant immunotherapy in resectable NSCLC. This method emphasized the combined effect of peripheral blood tests and PET/CT scans in comprehensively assessing the host immune status and immune activation. Results showed that the high inflammatory burden group, characterized by a pre-treatment SLR >0.83 and ΔNLR% >−17%, had the lowest pathologic regression and a pre-treatment TIME with CD68+ macrophages enrichment. There is persuasive evidence that macrophages promote cancer growth and malignant progression (32). Macrophages create a mutagenic and growth-promoting inflammatory environment during tumor initiation, stimulate angiogenesis, enhance tumor cell invasion, and suppress antitumor immunity during tumor progress (32). Therefore, the high inflammatory burden group exhibited CD68+ macrophages enrichment indicating an immunosuppressive microenvironment. The results provide complementary information for predictive markers, suggesting that in addition to the primary tumor, the host immune status also significantly influences the effect of immunotherapy. Notably, assessing host immune responses from peripheral blood alone can be unreliable because blood is susceptible to multiple factors, such as infection, steroid treatment, or other stress triggers. Our study specifically included lymphoid organ metabolism as an imaging marker of systemic inflammation and emphasized their combined effect. Both the pre- and post-treatment SLRs were potential negative predictors of pathologic response. Given the significant collinearity, we only included the pre-treatment SLR in the classification and did not include the post-treatment SLR. Moreover, post-immunotherapy PET/CT scan is not mandatory in routine clinical practice despite the non-negligible significance. Recurrent PET/CT scans are not cost-effective and have problems with cumulative radiation doses. In summary, our study suggests that combining pre-treatment PET/CT scan with longitudinal peripheral blood tests can serve as promising tools for stratifying pathologic response to neoadjuvant immunotherapy in resectable NSCLC. This approach offers easy integration into clinical practice, involves no additional expenditure, and allows for straightforward calculations.
Theoretical evidence suggests the existence of crosstalk among tumor-infiltrating immune cells, circulating immune cells, and lymphatic organs (13,33). The spleen and bone marrow regulate the balance between the pro-tumoral circulating and infiltrating myeloid-derived suppressor cells and anti-tumoral-activated CD8+ T cells (13,34). Seban et al. (20) reported that a high baseline SLR was an independent factor for low stromal tumor-infiltrating lymphocytes with a significant negative correlation. They also found that an increased BLR was significantly associated with the upregulation of transcriptomic profiles, including regulatory T cell markers (30). Studies have shown that the spleen and bone marrow accumulated a large number of immunosuppressive cells, and a high SLR and BLR might represent the enriched mobilization of tumor-associated immunosuppressive cells, which migrated to the peripheral lymphoid organs and tumor sites and mediated a suppressive immune microenvironment (35-37). In our study, the high inflammatory burden group represented a pre-treatment immunosuppressive TIME with CD68+ macrophages enrichment, in consistency with previous studies of high SLR- and high NLR-mediated immunosuppressive TIMEs (20,24,38). Furthermore, blood and PET/CT imaging comprehensively assessed systemic inflammation status from different perspectives of peripheral circulating cells and organs metabolism. Significant positive correlations between blood and PET/CT inflammatory markers have been reported in several studies (31,39,40), suggesting the potential of diffuse spleen and bone marrow uptake as integrated imaging-derived biomarkers reflecting hematologic imbalance. In this study, other than the significant positive correlation between ΔNLR% and ΔBLR%, the correlation analyses between blood and PET/CT inflammatory markers were not significant, which may be due to the limited sample size. A larger sample size may yield statistically significant results. In addition, Wang et al. (41) demonstrated significant correlations between primary tumor metabolic parameters and various immune cells in the TIME. Our results showed that the ΔSULmean% was negatively correlated with ΔCD8+ and ΔCD8+PD1− T cells. Although these correlations remain uncertain, they may reveal the potential of tumor and lymphoid tissue metabolism to characterize the information of tumor-infiltrating immune cells in the TIME. Advanced radiomic methods based on PET/CT texture analysis will be used to characterize the landscape of the tumor microenvironment in future studies.
PET/CT is an effective imaging method for the early detection of potential irAEs before clinical diagnosis with high detection rates among multiple imaging modalities, regardless of whether the patient is symptomatic or not (42,43). In our study, 24 patients (66.7%) had at least one site of irAEs on PET/CT, with thyroiditis being the most common manifestation. Studies have reported that clinical irAEs are related to the anti-tumor effects, with better efficacy and longer survival rates (14,44,45). However, the value of irAEs detected by PET/CT has been poorly investigated. Humbert et al. (21) demonstrated that immune-induced gastritis on PET/CT was a novel and strong imaging biomarker of improved overall survival. However, the emergence of irAEs on PET/CT did not have significant effects on both MPR and pathologic regression in the overall and per-organ statistical analyses in our study. The relatively short interval between pre- and post-treatment PET/CT scans (approximately 1 month) might be insufficient to detect delayed organ inflammation uptake, as most irAEs occurred during the first 3 months.
There are some limitations in this study. First, this was a single-center retrospective analysis of prospectively acquired data from a phase Ib trial. The preliminary and exploratory study had a limited sample size, and not all biopsy tumor tissues were available. Second, the small sample size posed challenges for comprehensive analyses of histological subgroups. Finally, we did not evaluate the long-term clinical endpoints such as progression-free survival and overall survival, as our study focused on pathologic response and MPR, which is a vital short-term endpoint that has potential to replace survival outcomes in neoadjuvant immunotherapy setting. Future studies with large sample sizes, long-term follow-up, and comprehensive evaluation are warranted to provide more conclusive insights.
Conclusions
In conclusion, the combination of systemic inflammatory markers from pre-treatment PET/CT scan and longitudinal peripheral blood tests are promising tools for stratifying pathologic response to resectable NSCLC in neoadjuvant immunotherapy, providing an easily integrable approach in routine clinical practice to help patients selection with potential benefits. The high inflammatory burden group showed the lowest pathologic regression and the pre-treatment immunosuppressive microenvironment with macrophage enrichment. Our findings may serve as preliminary data to encourage prospective studies to further explore the pathophysiological mechanisms involving systemic inflammatory markers and their association with the TIME and clinical outcomes.
Acknowledgments
We thank the patients and their families, and the participating study teams for making this study possible.
Funding: This work was supported by
Footnote
Reporting Checklist: The authors have completed the TRIPOD reporting checklist. Available at https://tlcr.amegroups.com/article/view/10.21037/tlcr-24-598/rc
Data Sharing Statement: Available at https://tlcr.amegroups.com/article/view/10.21037/tlcr-24-598/dss
Peer Review File: Available at https://tlcr.amegroups.com/article/view/10.21037/tlcr-24-598/prf
Conflicts of Interest: All authors have completed the ICMJE uniform disclosure form (available at https://tlcr.amegroups.com/article/view/10.21037/tlcr-24-598/coif). The authors have no conflicts of interest to declare.
Ethical Statement: The authors are accountable for all aspects of the work in ensuring that questions related to the accuracy or integrity of any part of the work are appropriately investigated and resolved. The study was conducted in accordance with the Declaration of Helsinki (as revised in 2013). The Ethics Committee and Institutional Review Board of the National Cancer Center/Cancer Hospital, Chinese Academy of Medical Sciences and Peking Union Medical College approved this prospective study (No. 17-151/1407), and informed consent was obtained from all individual participants.
Open Access Statement: This is an Open Access article distributed in accordance with the Creative Commons Attribution-NonCommercial-NoDerivs 4.0 International License (CC BY-NC-ND 4.0), which permits the non-commercial replication and distribution of the article with the strict proviso that no changes or edits are made and the original work is properly cited (including links to both the formal publication through the relevant DOI and the license). See: https://creativecommons.org/licenses/by-nc-nd/4.0/.
References
- Nasim F, Sabath BF, Eapen GA. Lung Cancer. Med Clin North Am 2019;103:463-73. [Crossref] [PubMed]
- Forde PM, Chaft JE, Smith KN, et al. Neoadjuvant PD-1 Blockade in Resectable Lung Cancer. N Engl J Med 2018;378:1976-86. [Crossref] [PubMed]
- Gao S, Li N, Gao S, et al. Neoadjuvant PD-1 inhibitor (Sintilimab) in NSCLC. J Thorac Oncol 2020;15:816-26. [Crossref] [PubMed]
- Chaft JE, Oezkan F, Kris MG, et al. Neoadjuvant atezolizumab for resectable non-small cell lung cancer: an open-label, single-arm phase II trial. Nat Med 2022;28:2155-61. [Crossref] [PubMed]
- Eichhorn F, Klotz LV, Kriegsmann M, et al. Neoadjuvant anti-programmed death-1 immunotherapy by pembrolizumab in resectable non-small cell lung cancer: First clinical experience. Lung Cancer 2021;153:150-7. [Crossref] [PubMed]
- Mantovani A, Allavena P, Sica A, et al. Cancer-related inflammation. Nature 2008;454:436-44. [Crossref] [PubMed]
- Roxburgh CS, McMillan DC. Cancer and systemic inflammation: treat the tumour and treat the host. Br J Cancer 2014;110:1409-12. [Crossref] [PubMed]
- Munn LL. Cancer and inflammation. Wiley Interdiscip Rev Syst Biol Med 2017;9: [Crossref] [PubMed]
- Hanahan D, Weinberg RA. Hallmarks of cancer: the next generation. Cell 2011;144:646-74. [Crossref] [PubMed]
- Platini H, Ferdinand E, Kohar K, et al. Neutrophil-to-Lymphocyte Ratio and Platelet-to-Lymphocyte Ratio as Prognostic Markers for Advanced Non-Small-Cell Lung Cancer Treated with Immunotherapy: A Systematic Review and Meta-Analysis. Medicina (Kaunas) 2022;58:1069. [Crossref] [PubMed]
- Jin P, Bai M, Liu J, et al. Tumor metabolic and secondary lymphoid organ metabolic markers on 18F-fludeoxyglucose positron emission tomography predict prognosis of immune checkpoint inhibitors in advanced lung cancer. Front Immunol 2022;13:1004351. [Crossref] [PubMed]
- Lang D, Ritzberger L, Rambousek V, et al. First-Line Pembrolizumab Mono- or Combination Therapy of Non-Small Cell Lung Cancer: Baseline Metabolic Biomarkers Predict Outcomes. Cancers (Basel) 2021;13:6096. [Crossref] [PubMed]
- Seban RD, Assié JB, Giroux-Leprieur E, et al. Prognostic value of inflammatory response biomarkers using peripheral blood and 18F-FDG PET/CT in advanced NSCLC patients treated with first-line chemo- or immunotherapy. Lung Cancer 2021;159:45-55. [Crossref] [PubMed]
- Grangeon M, Tomasini P, Chaleat S, et al. Association Between Immune-related Adverse Events and Efficacy of Immune Checkpoint Inhibitors in Non-small-cell Lung Cancer. Clin Lung Cancer 2019;20:201-7. [Crossref] [PubMed]
- Guo Y, Xiang D, Wan J, et al. Focus on the Dynamics of Neutrophil-to-Lymphocyte Ratio in Cancer Patients Treated with Immune Checkpoint Inhibitors: A Meta-Analysis and Systematic Review. Cancers (Basel) 2022;14:5297. [Crossref] [PubMed]
- Gajewski TF, Schreiber H, Fu YX. Innate and adaptive immune cells in the tumor microenvironment. Nat Immunol 2013;14:1014-22. [Crossref] [PubMed]
- Tao X, Li N, Wu N, et al. The efficiency of (18)F-FDG PET-CT for predicting the major pathologic response to the neoadjuvant PD-1 blockade in resectable non-small cell lung cancer. Eur J Nucl Med Mol Imaging 2020;47:1209-19. [Crossref] [PubMed]
- Prigent K, Lasnon C, Ezine E, et al. Assessing immune organs on (18)F-FDG PET/CT imaging for therapy monitoring of immune checkpoint inhibitors: inter-observer variability, prognostic value and evolution during the treatment course of melanoma patients. Eur J Nucl Med Mol Imaging 2021;48:2573-85. [Crossref] [PubMed]
- Seban RD, Moya-Plana A, Antonios L, et al. Prognostic 18F-FDG PET biomarkers in metastatic mucosal and cutaneous melanoma treated with immune checkpoint inhibitors targeting PD-1 and CTLA-4. Eur J Nucl Med Mol Imaging 2020;47:2301-12. [Crossref] [PubMed]
- Seban RD, Rouzier R, Latouche A, et al. Total metabolic tumor volume and spleen metabolism on baseline [18F]-FDG PET/CT as independent prognostic biomarkers of recurrence in resected breast cancer. Eur J Nucl Med Mol Imaging 2021;48:3560-70.
- Humbert O, Bauckneht M, Gal J, et al. Prognostic value of immunotherapy-induced organ inflammation assessed on (18)FDG PET in patients with metastatic non-small cell lung cancer. Eur J Nucl Med Mol Imaging 2022;49:3878-91. [Crossref] [PubMed]
- Ling Y, Li N, Li L, et al. Different pathologic responses to neoadjuvant anti-PD-1 in primary squamous lung cancer and regional lymph nodes. NPJ Precis Oncol 2020;4:32. [Crossref] [PubMed]
- Wang S, Yuan P, Mao B, et al. Genomic features and tumor immune microenvironment alteration in NSCLC treated with neoadjuvant PD-1 blockade. NPJ Precis Oncol 2022;6:2. [Crossref] [PubMed]
- Gabrilovich DI, Nagaraj S. Myeloid-derived suppressor cells as regulators of the immune system. Nat Rev Immunol 2009;9:162-74. [Crossref] [PubMed]
- Murakami Y, Tamiya A, Taniguchi Y, et al. Retrospective analysis of long-term survival factors in patients with advanced non-small cell lung cancer treated with nivolumab. Thorac Cancer 2022;13:593-601. [Crossref] [PubMed]
- Sibille A, Henket M, Corhay JL, et al. White Blood Cells in Patients Treated with Programmed Cell Death-1 Inhibitors for Non-small Cell Lung Cancer. Lung 2021;199:549-57. [Crossref] [PubMed]
- Kargl J, Busch SE, Yang GH, et al. Neutrophils dominate the immune cell composition in non-small cell lung cancer. Nat Commun 2017;8:14381. [Crossref] [PubMed]
- Coffelt SB, Wellenstein MD, de Visser KE. Neutrophils in cancer: neutral no more. Nat Rev Cancer 2016;16:431-46. [Crossref] [PubMed]
- Sachpekidis C, Weru V, Kopp-Schneider A, et al. The prognostic value of [18F]FDG PET/CT based response monitoring in metastatic melanoma patients undergoing immunotherapy: comparison of different metabolic criteria. Eur J Nucl Med Mol Imaging 2023;50:2699-714.
- Seban RD, Nemer JS, Marabelle A, et al. Prognostic and theranostic 18F-FDG PET biomarkers for anti-PD1 immunotherapy in metastatic melanoma: association with outcome and transcriptomics. Eur J Nucl Med Mol Imaging 2019;46:2298-310. [Crossref] [PubMed]
- Lee JH, Lee HS, Kim S, et al. Prognostic significance of bone marrow and spleen (18)F-FDG uptake in patients with colorectal cancer. Sci Rep 2021;11:12137. [Crossref] [PubMed]
- Qian BZ, Pollard JW. Macrophage diversity enhances tumor progression and metastasis. Cell 2010;141:39-51. [Crossref] [PubMed]
- Morrison SJ, Scadden DT. The bone marrow niche for haematopoietic stem cells. Nature 2014;505:327-34. [Crossref] [PubMed]
- Groth C, Hu X, Weber R, et al. Immunosuppression mediated by myeloid-derived suppressor cells (MDSCs) during tumour progression. Br J Cancer 2019;120:16-25. [Crossref] [PubMed]
- Haverkamp JM, Crist SA, Elzey BD, et al. In vivo suppressive function of myeloid-derived suppressor cells is limited to the inflammatory site. Eur J Immunol 2011;41:749-59. [Crossref] [PubMed]
- Kumar V, Patel S, Tcyganov E, et al. The Nature of Myeloid-Derived Suppressor Cells in the Tumor Microenvironment. Trends Immunol 2016;37:208-20. [Crossref] [PubMed]
- Cortez-Retamozo V, Etzrodt M, Newton A, et al. Origins of tumor-associated macrophages and neutrophils. Proc Natl Acad Sci U S A 2012;109:2491-6. [Crossref] [PubMed]
- Levy L, Mishalian I, Bayuch R, et al. Splenectomy inhibits non-small cell lung cancer growth by modulating anti-tumor adaptive and innate immune response. Oncoimmunology 2015;4:e998469. [Crossref] [PubMed]
- Yoon HJ, Kim BS, Moon CM, et al. Prognostic value of diffuse splenic FDG uptake on PET/CT in patients with gastric cancer. PLoS One 2018;13:e0196110. [Crossref] [PubMed]
- Nam HY, Kim SJ, Kim IJ, et al. The clinical implication and prediction of diffuse splenic FDG uptake during cancer surveillance. Clin Nucl Med 2010;35:759-63. [Crossref] [PubMed]
- Wang Y, Zhao N, Wu Z, et al. New insight on the correlation of metabolic status on (18)F-FDG PET/CT with immune marker expression in patients with non-small cell lung cancer. Eur J Nucl Med Mol Imaging 2020;47:1127-36. [Crossref] [PubMed]
- Mekki A, Dercle L, Lichtenstein P, et al. Detection of immune-related adverse events by medical imaging in patients treated with anti-programmed cell death 1. Eur J Cancer 2018;96:91-104. [Crossref] [PubMed]
- Iravani A, Osman MM, Weppler AM, et al. FDG PET/CT for tumoral and systemic immune response monitoring of advanced melanoma during first-line combination ipilimumab and nivolumab treatment. Eur J Nucl Med Mol Imaging 2020;47:2776-86. [Crossref] [PubMed]
- Kotwal A, Kottschade L, Ryder M. PD-L1 Inhibitor-Induced Thyroiditis Is Associated with Better Overall Survival in Cancer Patients. Thyroid 2020;30:177-84. [Crossref] [PubMed]
- Nobashi T, Baratto L, Reddy SA, et al. Predicting Response to Immunotherapy by Evaluating Tumors, Lymphoid Cell-Rich Organs, and Immune-Related Adverse Events Using FDG-PET/CT. Clin Nucl Med 2019;44:e272-9. [Crossref] [PubMed]