Hsp90 inhibitors enhance the antitumoral effect of osimertinib in parental and osimertinib-resistant non-small cell lung cancer cell lines
Introduction
Epidermal growth factor receptor tyrosine kinase inhibitors (EGFR-TKIs) are one of the most important treatments in non-small cell lung cancer (NSCLC) EGFR mutated patients. Osimertinib (also named AZD9291 or Tagrisso) is one EGFR-TKI that has been approved by the Food Drug Administration (FDA) for treating patients with EGFR T790 mutant NSCLC with disease progression on or after EGFR TKI progression. Although first-generation EGFR TKIs provide significant clinical benefit, acquired resistance often occurs, most commonly via a T790M resistance mutation. Osimertinib is selective for both T790M and activating EGFR mutations over wild-type EGFR, but it is highly active when T790M is present, especially EGFR(L858R/T790M), and modestly active when T790M is absent (1,2). Despite good initial response, resistance to osimertinib appears (3-6). The mechanisms of resistance could be divided into two groups: EGFR dependent and EGFR independent. In the first group, C797S mutation in EGFR exon 20 is the main cause of resistance. In addition, T790 mutation reduction or disappearance, EGFR amplification and high EGF levels are involved in resistance to osimertinib. EGFR-independent mechanisms that cause osimertinib-resistance have been described, such as, HER2 (7) or Met amplification, PIK3CA, KRas, NRas or BRAF mutations, PTEN loss, activation of RAS-MAPK or IGF-1R pathways, as well as the FGF-FGFR1 autocrine loop and epithelial-mesenchymal transition (EMT) (8).
An important therapeutic strategy for osimertinib-resistant NSCLC due to EGFR-dependent resistance mechanisms, is the use of anti-EGFR monoclonal antibodies, such as, cetuximab or panitumumab, alone, or in combination with other drugs, like, EA1045 or Brigatinib (6). When EGFR-independent resistance mechanisms are evident, the therapeutic strategies are to combine osimertinib plus crizotinib or inhibitors of MET, MEK, BRAF, PI3K, SFK, FAK or AXL (6). In addition, osimertinib resistant patients with SCLC transformation were treated with platinum-based doublet chemotherapy (9). It has also been described that the PD-L1 expression was increased in osimertinib-resistant NSCLC (10) and it has been postulated that PS-L1/PD-1 antibodies might be a new promising treatment for osimertinib-resistant patients with high PD-L1 expression (6).
Heat shock protein 90 (Hsp90) is highly expressed in most tumors (11). Because tumors cells are often protein misfolded, it implies the need for correct folding and more Hsp90 activity (12) and, for this reason, tumors tend to be more sensitive to Hsp90 inhibition (13). Hsp90 plays a crucial role in maintaining cellular protein homeostasis because it regulates protein maturation, folding and stability, and plays a critical role in differentiation, cell growth and survival (14). Thus, Hsp90 could be an attractive therapeutic target and several clinical trials are being opened or are in progress with Hsp90 inhibitors alone or in combination with other drugs (15).
Hsp90 inhibitors induce the proteosomal degradation of Hsp90 client proteins such as EGFR, HER2, IGF-1R, Stat3, Akt, Raf-1, IKK, c-Kit, v-Src, NPM-Alk, p53, HIF-1 and CD4/6 (16,17). In addition, Akt-activated tumors are sensitive to Hsp90 inhibitors (18) and indirectly downregulate Mcl1 expression by inhibiting Akt and Erk1/2 signaling (19).
Hsp90 inhibitors, such as Ganetespib and Luminespib, have been assayed in clinical trials in several tumor types, such as NSCLC (20,21), colon (22), breast (23), prostate (24,25), myeloma (26,27) and melanoma (28). It has been demonstrated that they overcome resistance to EGFR-kinase inhibitors (18).
Unfortunately, these inhibitors showed limited efficacy as a single agent, however, they are still in various stages of clinical trials as a component for combination therapies (16,29). Luminespib used as a single agent in a phase II study in lung cancer patients showed poor effectiveness (16,29).
Recently, the results have been published of a phase II study with Luminespib in pretreated patients with stage IV NSCLC. This compound is active in these patients, particularly among patients with ALK rearrangements and EGFR mutations (30). In addition, EGFR exon 20 insertion mutations display sensitivity to Hsp90 inhibition in lung adenocarcinomas and cellular models (31,32).
In our study we investigate whether the Hsp90 inhibitors, luminespib and ganetespib, could overcome osimertinib-resistance in EGFR-mutated NSCLC cell lines.
Methods
Cell lines
Human lung adenocarcinoma PC-9 cells, harboring EGFR exon 19 deletion (E746-A750) were provided by F. Hoffmann-La Roche Ltd. with the authorization of Dr. Mayumi Ono (Kyushu University, Fukuoka, Japan). Human lung adenocarcinoma H1975 cells, harboring both sensitizing L858R and resistant T790M mutation, were purchased from the American Type Culture Collection (ATCC). PC9-OR2 and PC9-OR4 are osimertinib-resistant cells, the two lines were generated in our laboratory by exposing PC9 cells to increasing concentrations of the osimertinib (33). All cell lines were maintained in Roswell Park Memorial Institute (RPMI) 1640 Medium supplemented with 1% penicillin/streptomycin/glutamine (Gibco) and 10% fetal bovine serum (FBS) (Gibco) in 5% CO2, 37 °C cell culture incubator and were routinely evaluated for mycoplasma contamination. Media and supplements were obtained from Life Technologies (Gaithersburg, MD, USA).
Chemical and reagents
Osimertinib (AZD9291), luminespib (AUY922) and ganetespib (STA9090) were purchased from Selleck Chemicals (Houston, TX, USA). Drugs were prepared in dimethyl sulfoxide (DMSO) at a concentration of 10–100 mmol/L stock solutions and stored at −20 °C. Further dilutions were made in culture medium to final concentration before use. The following antibodies used were from Cell Signaling Technology (Beverly, MA, USA): EGFR (Cat#4267); phospho-EGFR (Tyr 1068) (Cat#3777); STAT3 (Cat#9139); phospho-STAT3 (Tyr705) (Cat#9145); AKT (Cat#9272); phospho-AKT (Ser473) (Cat#9271); ERK1/2 (Cat#9102); phospho-ERK 1/2 (Thr202/Tyr204) (Cat#9101); Bmi1 (Cat#6964); HES1 (Cat#11988); IGF-1R (Cat#9750); phospho-IGF-1R (Tyr 136) (Cat#6113) EphA2 (Cat#6997); phospho-EphA2 (Ser 897) (Cat#6347); Met (Cat#8198); Axl (Cat#8661); Src (Cat#2109); phospho-Src (Tyr 416) (Cat#6943); Yap (Cat#12395); mTOR (Cat#2983); Bcl2 (Cat#15071); Bim (Cat#2819). phospho-Yap (Tyr 357) (Cat#ab62751), h3 (Cat#ab1791) were purchased from Abcam (Cambridge, UK). phospho-h3 (Ser 10) (Cat#06-570) was purchased from Millipore and α-Tubulin from Sigma-Aldrich (Cat#T9026). Secondary antibodies horseradish linked peroxidase anti-rabbit (Cat#NA934), and horseradish linked peroxidase anti-mouse (Cat#NX931) antibodies were purchased from GE Healthcare.
Cell viability assay
The effects of different compounds on the cell viability of PC9, H1975, PC9-OR2 and PC9-OR4 were studied by use of the colorimetric (MTT). Two thousand (PC9, H1975) or 4000 (PC9-GR4, PC9-OR4) cells were seeded in 100 µL 0.1% FBS media and incubated for 24 hours before treatment; 100 µL of various 2× drug concentration (1× final concentration) were added to the wells, and the cells were incubated for 72 hours.
Cell viability was assessed by the Thiazolyl Blue Tetrazolium Bromide (MTT) (Sigma, St Louis, MO, USA) assay. Cells from each cell line were seeded at 2,000 to 4,000 cells per well in 96-well plates, allowed to attach for 24 h and treated for 72 h (for details of the combination experiments, see below). After treatment, cells were incubated with medium containing MTT (0.75 mg/mL in medium) for 1–2 h at 37 °C. Culture medium with MTT was removed and formazan crystals reabsorbed in 100 µL DMSO (Sigma). Cell numbers were estimated by measuring the absorbance at 495 nm, using a microplate reader (BioWhittaker, Walkersville, MD, USA). The data reported represent the mean ± SD of a minimum of 3 experiments of each drug concentration tested.
Western blotting
For Western blot assays, cells were cultured in cell culture flasks and left untreated or treated as indicated in each experiment. Cells were lysed in ice-cold RIPA buffer [20 mM Tris-HCl (pH:7.5), 150 mM NaCl, 1 mM EDTA, 1 mM EGTA, 1% NP40, 1% sodium deoxycholate, 2.5 mM sodium pyrophosphate, 1 mM beta-glycerophosphate, 1 mM Na3VO4, 1 µg/mL leupeptin, 1 mM PMSF]. After incubating for 20 min at 4 °C, the samples were centrifuged, and the supernatant was kept at −80 °C. Protein concentration was determined by bicinchoninic acid protein assay. Equal amounts of protein from each cell lysate (30 µg/lane) were subjected to SDS polyacrylamide gel electrophoresis (SDS/PAGE) and transferred onto polyvinylidene difluoride membranes (Millipore, New Bedford, MA, USA). The membranes were blocked in Tris-buffered saline containing 5% fat free dry milk and then probed with primary antibodies at 4 °C overnight. After washing, the membrane was incubated with horseradish peroxidase-conjugated secondary antibodies for 2 hours at room temperature. Specific proteins were visualized with enhanced chemiluminescence detection reagent according to the manufacturer’s instructions (Pierce Biotechnology, Rockford, IL, USA) and analyzed by Chemidoc MP Imaging System (Bio Rad). β-actin was used as an internal control to confirm equal gel loading.
Colony formation assay
PC9, H1975, PC9-OR2 and PC9-OR4 cells were seeded at 200 cells per well in a 6-well plate and incubated overnight. The cells were treated with compounds, Osimertinib, Ganetespib or Luminespib, or DMSO as vehicle control. The culture media and compounds were changed every three days. On day 12 cell culture medium was removed and each well was washed with phosphate buffered saline (PBS). Then the colonies were stained with 0.5% of violet crystal at room temperature for 15 min and distilled water was used to wash the plates several times until the dye stops coming off and allowed to dry overnight. Images were captured and the number of colonies in each well was counted using J software.
Patient and case report
The clinical and radiographic data for this study was obtained from the medical reports of a patient treated in our institution. Patient signed and IRB-validated informed consent authorizing the anonymized use of her medical images for publication purposes following institutional and regional regulations and the review of data was conducted in accordance with recognized ethical guidelines. The Institutional Review Board was the Ethical Committee of Drug Research of Quirónsalud Hospitality Group (Barcelona, Spain).
Results
Protein characterization of osimertinib-resistant cell lines
We established five osimertinib-resistant cell lines from the parental NSCLC cell line, PC9. This cell line was treated with increased concentrations of osimertinib, as described, thus generating the osimertinib-resistant cell lines. PC9 cells were treated with an increased concentration of osimertinib for 10 cycles and the 10th generation resistant cells were named PC9-OR1, PC9-OR2, PC9-OR3, PC9-OR4 and PC9-OR5 (PC9-ORs). The IC50 values for osimertinib of these cell lines are described in Figure 1.
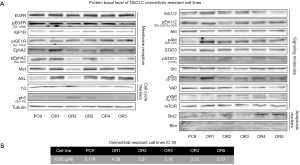
We studied the basal level expression and activation of several proteins, such as, membrane receptors, protein receptor tyrosine kinase signaling, cancer stem cell markers, and proteins involved in apoptosis and cell cycle, to investigate whether the PC9-ORs cell lines influence protein expression and activation. The most important change when comparing the PC9 parental cell line with PC9-ORs was: in all five PC9-ORs cells AKT was activated, in three, Stat3 was activated, and in four, YAP was activated. There was overexpression of EMT marker AXL and IGF1R activation in two of them. There is also overexpression of antiapoptotic protein bcl-2 in three PC9-ORs (Figure 1). We described that the CSC marker Hes1 is overexpressed in four PC9-ORs (34).
Hsp90 inhibitors potentiate the effects of osimertinib in cell viability and colony formation inhibition
It has previously been described that ganetespib exhibits cytotoxic activity against NSCLC lines with EGFR mutations and also showed activity against erlotinib-resistant NSCLC tumor phenotypes in vitro (35,36).
To determine the ganetespib and luminespib antitumoral activity in the context of EGFR-mutated cell lines (PC9, H1975) and osimertinib-resistant cell lines (PC9-OR2 and PC9-OR4), cells were treated for 72 h with the Hsp90 inhibitors, ganetespib and luminespib, in a dose-dependent manner to determine if they inhibit cell viability in theses cancer cell lines.
As shown in Figure 2A, the cell viability of both resistant and parental cell lines was similarly affected by each Hsp90 inhibitor. Ganetespib and luminespib caused a dose-dependent, cell viability decrease of 12.5–15 nM in both compounds.
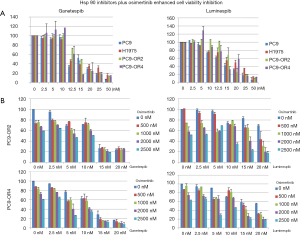
To determine whether Hsp90 inhibitors could overcome intrinsic resistance to osimertinib and synergize with osimertinib in PC9-OR cells, we exposed PC9-OR2 and PC9-OR4 cells to different doses of osimertinib plus ganetespib or luminespib. In these studies, the combination of luminespib plus osimertinib in both cell lines and ganetespib plus osimertinib in PC9-OR4 cell line clearly potentiates a decrease of cell viability (Figure 2).
Furthermore, colony formation assays indicate that PC9, H1975, PC9-OR2 and PC9-OR4 cells incubated with osimertinib and luminespib, in a dose-dependent experiment, were sensitive to the combination, significantly reducing the number of colonies better than single luminespib treatment, showing a clear effect at 2 nM of luminespib plus osimertinib. Thus, combination treatment reduces the formation of new colonies greater than single luminespib or osimertinib strategy (Figure 3).
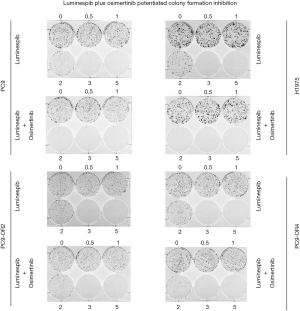
Luminespib decreases expression and activation of proteins involved in osimertinib-resistance
To assess the effect of luminespib in expression and activation of proteins related to osimertinib-resistance, Western blotting analyses were conducted. In a dose-response experiment with PC9-OR2 and PC9-OR4 cells exposed to increasing luminespib concentrations, it was observed that the Hsp90 inhibitor attenuated protein expression, in a dose-dependent manner, of membrane receptors EGFR, MET and AXL, that are protein clients of Hsp90 (16,17) and, in a lesser manner, the downstream molecules of signaling pathways related to membrane receptors, such as, AKT and Stat3, which are described as Hsp90 client proteins (16,17), and YAP (Figure 4). We described that the CSC marker Hes1 is overexpressed in four PC9-ORs (35).
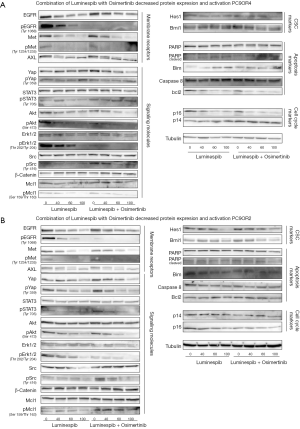
Concomitant reduction has also been observed in the phosphorylation of the membrane receptors EGFR, MET, downstream signaling molecules Stat3, AKT, Erk1/2 YAP and Src (Figure 4).
There were no protein level changes of signaling molecules Src, Erk1/2, β-catenin at the doses of luminespib used (Figure 4).
In apoptotic related proteins, there are no changes in PARP cleavage in the PC9-OR2 cell line, but an increase of PARP cleaved protein was appreciated in the PC9-OR4 cell line. There is a decrease in bcl2 expression that is clearer in the PC9-OR4 cell line. There are no significant changes in caspase 8 expression (Figure 4). Finally, a slight protein expression decrease was observed in cell cycle related proteins, p14 in PC9-OR2 and p16 in PC9-OR4 (Figure 4).
Luminespib sensitizes osimertinib-resistant cell lines to osimertinib
As we have observed before that luminespib was able to decrease expression or activation of several proteins involved in osimertinib-resistance, we wanted to study the capacity of luminespib to potentiate the effect of osimertinib in osimertinib-resistant cell lines. Then, to determine whether luminespib can enhance the antitumor effect of osimertinib, we treated PC9-OR2 and PC9-OR4 cells with increasing doses of luminespib alone, or in combination with a constant dose of osimertinib (50 nM), for 24 h, and analyzed the effect of the luminespib plus osimertinib combination.
We assessed that the osimertinib and luminespib combination causes and important effect in protein expression of YAP and AKT in PC9-OR4 and bcl2 in PC9-OR2 and the CSC marker Bmi1 in PC9-OR4. There is a clear protein expression downregulation of p14 in PC9-OR2 and p16 in PC9-OR4 cell cycle markers (Figure 4). Also, we have to note that osimertinib increases the phosphorylation of Stat3, AKT and Src in both cell lines and YAP in PC9-OR2, and luminespib was able to reverse this activation (Figure 4).
Taken together, our studies indicate that the dual treatment of Hsp90 inhibitors plus osimertinib partially overcomes osimertinib-resistance in these tumor cell lines.
Clinical activity of ganetespib in one lung cancer patient harboring an exon 20 EGFR mutation
A 29-year-old female, never smoker, was diagnosed with advanced NSCLC patient after presenting with cough, dyspnea and hemoptysis. Imaging studies revealed metastatic involvement of the mediastinal lymph nodes, multiple pleural implants and severe pleural effusion. A pleural biopsy allowed confirmatory diagnosis of metastatic lung adenocarcinoma and EGFR testing was performed detecting an exon 20 T790M mutation. No known EGFR sensitizing mutation was detected. Other actionable alterations were not routinely tested at our institution at that time.
Initial therapy with erlotinib was ineffective and resulted in symptomatic tumor progression within the first month of treatment. Patient was then enrolled in an afatinib phase 2 clinical trial but again, disease progression was the best response after 8 weeks of treatment. Three cytotoxic chemotherapy lines of treatment were subsequently administered (including platinum-gemcitabine, pemetrexed and paclitaxel-bevacizumab) with short-lived clinical benefit allowing 2 years of survival at the cost several chronic sequels due to cumulative toxicity. By April 2012 the disease had spread with new brain and bone metastases. At that point, patient started ganetespib treatment under a compassionate use program. The best response assessment showed brain and femur metastasis partial response (Figure 5) and the duration of the response was 8 months. Ganetespib tolerance was adequate with asthenia and mild diarrhea as only reported side effects (Figure 5).
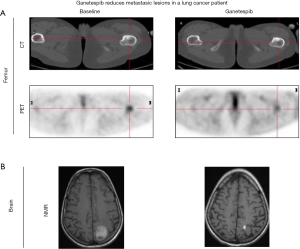
Discussion
Osimertinib has been approved by FDA for treating patients with EGFR T790M mutant NSCLC with disease progression on or after EGFR TKI treatment, however, resistance to this drug emerged. The mechanisms of resistance could be EGFR dependent or EGFR independent, and several strategies to overcome osimertinib-resistance have been assayed.
Many of the proteins involved in mutant EGFR proteins are particularly reliant on the chaperone activity on Hsp90 for their conformational stability and function (18,37). For this reason, the use of Hsp90 inhibitors that leads to the degradation of mutant proteins involved in EGFRTKI resistance, has been assayed to treat EGFR mutant tumors, as well as WT EGFR tumors (38). However, the clinical results with Hsp90 inhibitors revealed a modest single-agent activity in WT or mutant EGFR NSCLC patients (39,40). As a result, Hsp90 inhibitors have been used in combination with other drugs. For example, ganetespib has shown potent chemosensitizing activity when combined with taxanes in NSCLC (20,41). Also, Hsp90 inhibitors have shown good efficiency in EGFR-TKI resistant cells (18). It has been described that the Hsp90 inhibitor luminespib was active in patients with NSCLC, particularly among patients with ALK rearrangements and EGFR mutations (33) KRAS-mutant cell lines with LKB loss showed enhanced sensitivity to Hsp90 inhibition (42,43).
Acquired resistance to EGFR TKIs is usually due to the development of a T790M mutation in EGFR exon 20. Luminespib and ganetespib showed antitumor activity against H1975, which harbors T790M and L858R mutations (8).
We have generated five osimertinib-resistant cell lines from the EGFR mutant NSCLC cell line, PC9 and analyzed the molecular profile of these cell models. Many of the proteins overexpressed or activated in these osimertinib-resistant cell lines are client proteins of Hsp90, and, for this reason, we hypothesize that the combination of Hsp90 inhibitors and osimertinib could be an adequate strategy to overcome osimertinib-resistance.
Our results showed synergistic effect of the combination of osimertinib and the Hsp90 inhibitors, ganetespib and luminespib, on inhibiting the growth of lung cancer cells, and similar effects have been seen in colony-formation assays. Therefore, the combination of Hsp90 inhibitors with osimertinib could be a promising therapeutic strategy for osimertinib-resistant NSCLC patients. Hsp90 inhibition was seen to induce sensitivity to osimertinib in osimertinib-resistant cell lines. The concurrent treatment with luminespib and osimertinib resulted in a significantly improved antitumor response, indicating that dual treatment may provide a good strategy to overcome osimertinib-resistance. This should be evaluated further in clinical trials.
We then analyzed, by Western blotting technique, the molecular basis underlying the superior efficacy of the combinatorial strategy over single agent treatment, since Hsp90 inhibition could have a convergent impact, or parallel signaling pathways, to the EGFR pathway.
The Hsp90 inhibitor luminespib, decreases the protein expression of membrane receptors, such as EGFR, MET and AXL, and, in a lesser manner, the downstream molecules, YAP, Stat3, AKT, Src, Mcl1, the apoptosis marker Bim and cell cycle marker p14. This treatment, also decreases the phosphorylation of EGFR and MET, with concomitant effects on downstream pathways, including, Stat3, AKT, YAP, Src and Erk1/2.
The addition of luminespib to osimertinib treatment attenuated the expression of downstream signaling molecules, Stat3 and AKT, and the CSC marker, Bmi1. It also affects the activation of EGFR and Erk1/2. One remarkable effect is that luminespib decreases the activation caused by osimertinib of MET, Stat3, AKT and Src.
As an example of the potential clinical activity of Hsp90 inhibitors in EGFR mutant patients we described the evolution of a patient case that showed significant benefit from ganetespib monotherapy. At that moment, osimertinib was not available but based on our results the combined treatment would have been an excellent opportunity for our patient.
In conclusion, our results focused on the role of Hsp90 inhibition as a potential therapeutic option in combination with osimertinib against osimertinib-resistant NSCLC.
Acknowledgments
We acknowledge Ms. Stephanie Davies for language editing assistance.
Funding: Work in Dr. Rosell’s laboratory is partially supported by a Marie Skłodowska-Curie Innovative Training Networks18 European Grant (ELBA No. 765492) and European Union’s Horizon 2020 research and innovation programme under the Marie Skłodowska-Curie grant agreement No. 712949 (TECNIOspring PLUS) and from the Agency for Business Competitiveness of the Government of Catalonia.
Footnote
Conflicts of Interest: The authors have no conflicts of interest to declare.
Ethical Statement: The authors are accountable for all aspects of the work in ensuring that questions related to the accuracy or integrity of any part of the work are appropriately investigated and resolved. Patient signed and IRB-validated informed consent authorizing the anonymized use of her medical images for publication purposes following institutional and regional regulations and the review of data was conducted in accordance with recognized ethical guidelines. The Institutional Review Board was the Ethical Committee of Drug Research of Quirónsalud Hospitality Group (Barcelona, Spain).
References
- Greig SL. Osimertinib: First Global Approval. Drugs 2016;76:263-73. [Crossref] [PubMed]
- Popat S. Osimertinib as First-Line Treatment in EGFR-Mutated Non-Small-Cell Lung Cancer. N Engl J Med 2018;378:192-3. [Crossref] [PubMed]
- Paz-Ares L, Soulieres D, Melezinek I, et al. Clinical outcomes in non-small-cell lung cancer patients with EGFR mutations: pooled analysis. J Cell Mol Med 2010;14:51-69. [Crossref] [PubMed]
- Pérez-Soler R, Chachoua A, Hammond LA, et al. Determinants of tumor response and survival with erlotinib in patients with non-small-cell lung cancer. J Clin Oncol 2004;22:3238-47. [Crossref] [PubMed]
- Santarpia M, Liguori A, Karachaliou N, et al. Osimertinib in the treatment of non-small-cell lung cancer: design, development and place in therapy. Lung Cancer (Auckl) 2017;8:109-25. [Crossref] [PubMed]
- Tang ZH, Lu JJ. Osimertinib resistance in non-small cell lung cancer: Mechanisms and therapeutic strategies. Cancer Lett 2018;420:242-6. [Crossref] [PubMed]
- Kim TM, Song A, Kim DW, et al. Mechanisms of acquired resistance to AZD9291: a mutation-selective, irreversible EGFR inhibitor. J Thorac Oncol 2015;10:1736-44. [Crossref] [PubMed]
- Suda K, Rivard CJ, Mitsudomi T, et al. Overcoming resistance to EGFR tyrosine kinase inhibitors in lung cancer, focusing on non-T790M mechanisms. Expert Rev Anticancer Ther 2017;17:779-86. [Crossref] [PubMed]
- Ham JS, Kim S, Kim HK, et al. Two cases of small cell lung cancer transformation from EGFR mutant adenocarcinoma during AZD9291 treatment. J Thorac Oncol 2016;11:e1-4. [Crossref] [PubMed]
- Gainor JF, Sequist LV, Shaw AT, et al. Clinical correlation and frequency of programmed death ligand-1 (PD-L1) expression in EGFR-mutant and ALK-rearranged non-small cell lung cancer (NSCLC). J Clin Oncol 2015;33:8012. [Crossref]
- Park KS, Oh B, Lee MH, et al. The HSP90 inhibitor, NVP-AUY922, sensitizes KRAS-mutant non-small cell lung cancer with intrinsic resistance to MEK inhibitor, trametinib. Cancer Lett 2016;372:75-81. [Crossref] [PubMed]
- Kamal A, Thao L, Sensintaffar J, et al. A high-affinity conformation of Hsp90 confers tumour selectivity on Hsp90 inhibitors. Nature 2003;425:407-10. [Crossref] [PubMed]
- Lai CH, Park KS, Lee DH, et al. HSP-90 inhibitor ganetespib is synergistic with doxorubicin in small cell lung cancer. Oncogene 2014;33:4867-76. [Crossref] [PubMed]
- Wandinger SK, Richter K, Buchner J. The Hsp90 chaperone machinery. J Biol Chem 2008;283:18473-7. [Crossref] [PubMed]
- Yuno A, Lee MJ, Lee S, et al. Clinical Evaluation and Biomarker Profiling of Hsp90 Inhibitors. Methods Mol Biol 2018;1709:423-41. [Crossref] [PubMed]
- Garcia-Carbonero R, Carnero A, Paz-Ares L. Inhibition of HSP90 molecular chaperones: moving into the clinic. Lancet Oncol 2013;14:e358-69. [Crossref] [PubMed]
- Tillotson B, Slocum J, Coco N, et al. Hsp90 (heat shock protein 90) inhibitor occupancy is a direct determinant of client protein degradation and tumor growth arrest in vivo. J Biol Chem 2010;285:39835-43. [Crossref] [PubMed]
- Shimamura T, Li D, Ji H, et al. Hsp90 inhibition suppresses mutant EGFR-T790M signaling and overcomes kinase inhibitor resistance. Cancer Res 2008;68:5827-38. [Crossref] [PubMed]
- Lee DH, Sung KS, Bartlett DL, et al. HSP90 inhibitor NVP-AUY922 enhances TRAIL-induced apoptosis by suppressing the JAK2-STAT3-Mcl-1 signal transduction pathway in colorectal cancer cells. Cell Signal 2015;27:293-305. [Crossref] [PubMed]
- Sequist LV, Gettinger S, Senzer NN, et al. Activity of IPI-504, a novel heat-shock protein 90 inhibitor, in patients with molecularly defined non-small-cell lung cancer. J Clin Oncol 2010;28:4953-60. [Crossref] [PubMed]
- Garon EB, Finn RS, Hamidi H, et al. The HSP90 inhibitor NVP-AUY922 potently inhibits non-small cell lung cancer growth. Mol Cancer Ther 2013;12:890-900. [Crossref] [PubMed]
- Chen JS, Hsu YM, Chen CC, et al. Secreted heat shock protein 90alpha induces colorectal cancer cell invasion through CD91/LRP-1 and NF-kappaB-mediated integrin alphaV expression. J Biol Chem 2010;285:25458-66. [Crossref] [PubMed]
- Massey AJ, Schoepfer J, Brough PA, et al. Preclinical antitumor activity of the orally available heat shock protein 90 inhibitor NVP-BEP800. Mol Cancer Ther 2010;9:906-19. [Crossref] [PubMed]
- Solit DB, Zheng FF, Drobnjak M, et al. 17-Allylamino-17- demethoxygeldanamycin induces the degradation of androgen receptor and HER-2/neu and inhibits the growth of prostate cancer xenografts. Clin Cancer Res 2002;8:986-93. [PubMed]
- Lattouf JB, Srinivasan R, Pinto PA, et al. Mechanisms of disease: the role of heat-shock protein 90 in genitourinary malignancy. Nat Clin Pract Urol 2006;3:590-601. [Crossref] [PubMed]
- Okawa Y, Hideshima T, Steed P, et al. SNX-2112, a selective Hsp90 inhibitor, potently inhibits tumor cell growth, angiogenesis, and osteoclastogenesis in multiple myeloma and other hematologic tumors by abrogating signaling via Akt and ERK. Blood 2009;113:846-55. [Crossref] [PubMed]
- Huston A, Leleu X, Jia X, et al. Targeting Akt and heat shock protein 90 produces synergistic multiple myeloma cell cytotoxi city in the bone marrow microenvironment. Clin Cancer Res 2008;14:865-74. [Crossref] [PubMed]
- Haarberg HE, Paraiso KH, Wood E, et al. Inhibition of Wee1, AKT, and CDK4 underlies the efficacy of the HSP90 inhibitor XL888 in an in vivo model of NRAS-mutant melanoma. Mol Cancer Ther 2013;12:901-12. [Crossref] [PubMed]
- Seggewiss-Bernhardt R, Bargou RC, Goh YT, et al. Phase 1/1B trial of the heat shock protein 90 inhibitor NVP-AUY922 as monotherapy or in combination with bortezomib in patients with relapsed or refractory multiple myeloma. Cancer 2015;121:2185-92. [Crossref] [PubMed]
- Rong B, Yang S. Molecular mechanism and targeted therapy of Hsp90 involved in lung cancer: New discoveries and developments Int J Oncol 2018;52:321-36. (Review). [PubMed]
- Felip E, Barlesi F, Besse B, et al. Phase 2 Study of the HSP-90 Inhibitor AUY922 in Previously Treated and Molecularly Defined Patients with Advanced Non-Small Cell Lung Cancer. J Thorac Oncol 2018;13:576-84. [Crossref] [PubMed]
- Jorge SE, Lucena-Araujo AR, Yasuda H, et al. EGFR Exon 20 Insertion Mutations Display Sensitivity to Hsp90 Inhibition in Preclinical Models and Lung Adenocarcinomas. Clin Cancer Res 2018;24:6548-55. [Crossref] [PubMed]
- Piotrowska Z, Costa DB, Oxnard GR, et al. Activity of the Hsp90 inhibitor luminespib among non-small-cell lung cancers harboring EGFR exon 20 insertions. Ann Oncol 2018;29:2092-7. [PubMed]
- Jacobsen K, Bertran-Alamillo J, Molina MA, et al. Convergent Akt activation drives acquired EGFR inhibitor resistance in lung cancer. Nat Commun 2017;8:410. [Crossref] [PubMed]
- Codony-Servat J, Codony-Servat C, Cardona AF, et al. Cancer Stem Cells Biomarkers in EGFR-mutation positive Non-Small Cell Lung Cancer. Clin Lung Cancer 2019;20:167-77. [Crossref] [PubMed]
- Ying W, Du Z, Sun L, et al. Ganetespib, a unique triazolone-containing Hsp90 inhibitor, exhibits potent antitumor activity and a superior safety profile for cancer therapy. Mol Cancer Ther 2012;11:475-84. [Crossref] [PubMed]
- Shimamura T, Perera SA, Foley KP, et al. Ganetespib (STA-9090), a non geldanamycin HSP90 inhibitor, has potent antitumor activity in in vitro and in vivo models of non-small cell lung cancer. Clin Cancer Res 2012;18:4973-85. [Crossref] [PubMed]
- Shimamura T, Lowell AM, Engelman JA, et al. Epidermal growth factor receptors harboring kinase domain mutations associate with the heat shock protein 90 chaperone and are destabilized following exposure to geldanamycins. Cancer Res 2005;65:6401-8. [Crossref] [PubMed]
- Grossi F, Kubota K, Cappuzzo F, et al. Future scenarios for the treatment of advanced non-small cell lung cancer: focus on taxane-containing regimens. Oncologist 2010;15:1102-12. [Crossref] [PubMed]
- Socinski MA, Goldman J, El-Hariry I, et al. A multicenter phase II study of ganetespib monotherapy in patients with genotypically defined advanced non-small cell lung cancer. Clin Cancer Res 2013;19:3068-77. [Crossref] [PubMed]
- Proia DA, Sang J, He S, et al. Synergistic activity of the Hsp90 inhibitor ganetespib with taxanes in non-small cell lung cancer models. Invest New Drugs 2012;30:2201-9. [Crossref] [PubMed]
- Ramalingam S, Goss GD, Andric ZG, et al. A randomized study of ganetespib, a heat shock protein 90 inhibitor, in combination with docetaxel versus docetaxel alone for second-line therapy of lung adenocarcinoma (GALAXY-1). J Clin Oncol 2013;31:abstr CRA8007.
- Skoulidis F, Byers LA, Diao L, et al. Co-occurring genomic alterations define major subsets of KRAS-mutant lung adenocarcinoma with distinct biology, immune profiles, and therapeutic vulnerabilities. Cancer Discov 2015;5:860-77. [Crossref] [PubMed]