Transbronchial microwave ablation of lung nodules with electromagnetic navigation bronchoscopy guidance—a novel technique and initial experience with 30 cases
Introduction
With strong evidence for low-dose computer tomography (CT) scans screening in high risk populations (1), incidental discovery of lung nodule is becoming more common. Many of them are small, sub-solid, and harbour pre-malignant or early stage tumours. Local therapies as definitive treatment of these lesions is among the standard managements, especially in patients with surgical contraindications, for instance prohibitive cardiorespiratory risks or inadequate lung function. Among patients newly diagnosed with early stage lung cancer, 5–10% have absolute or relative contraindications to surgery (2,3), but the percentage could be as high as 28% in octogenarians (4). Treatment modalities such as stereotactic body radiation therapy (SBRT) (5), percutaneous ablation techniques for instance radiofrequency (RFA) (6), microwave (MWA) (7) and cryotherapy (8) are highly effective and are associated with reasonable local control rates ranging from 64% to 69.8% at 2 years. However, these procedures are also associated with complications: SBRT carries up to 22.3% risk of radiation pneumonitis and pneumonia (9), while percutaneous ablation techniques carry 11–52% risk of pneumothorax and bronchopleural fistula (BPF) (10). In terms of energy source, radiofrequency relies heavily on electrical conductance of tissues, thus the high impedance of human lung tissue may limit its effectiveness (11).
Bronchoscopic transbronchial ablation techniques utilizing radiofrequency energy for eradication of tumor cell have been described (12), with theoretical advantages of less pneumothorax/BPF due to non-trans-pleural route of entry, being able to reach peri-bronchial tissues easily, and having access to particular locations in lung which are otherwise difficult or impossible to reach. Meanwhile, microwave energy produces a larger and more predictable ablation zone, as it is minimally affected by impedance and has less heat-sink effects (11). Building upon our institute’s experience in lung nodule dye-marking and biopsy via electromagnetic navigation bronchoscopy (ENB) (13,14), we identified a selected group of cases which are suitable for bronchoscopic transbronchial microwave ablation under ENB guidance. Being one of the first centres to perform ENB microwave ablation in clinical setting, we retrospectively analyze data from the first cohort of patients who had completed the procedure. Primary objective of this study is to assess the safety, feasibility and efficacy in reference to ablative effects and treatment outcomes. We present the following article in accordance with the STROBE reporting checklist (available at http://dx.doi.org/10.21037/tlcr-20-1231).
Methods
Trial design
This study is a single-centre retrospective analysis of patients who underwent bronchoscopic transbronchial microwave ablation of malignant or suspicious lung nodules. The study was conducted in compliance with the Declaration of Helsinki (as revised in 2013) and approved by local institutional review board (the Joint Chinese University of Hong Kong – New Territories East Cluster Clinical Research Ethics Committee), CREC reference number of 2020.524. All cases were discussed in multi-disciplinary meetings with oncologists’ input, and all study participants gave informed consent before taking part in the study.
Enrolment criteria
Patients with radiologically suspicious lung nodules on CT scans and carried high surgical risk or have refused surgery were eligible for evaluation for ENB microwave ablation. Favourable radiologic features for selection included presence of bronchus sign (presence of bronchus directly leading to nodule), peripheral location, tumor size less than 3 cm, and at least 5 mm away from large blood vessels or mediastinal structures to avoid significant heat sink effect or thermal injury to other visceral organs. Special attention should be paid to avoid including brachial plexus or phrenic nerve into the designed ablation zone. Patients with biopsy-proven lung cancer must have CT or PET/CT scans for exclusion of distant metastases, while nodal involvements were ruled out with endobronchial ultrasound biopsy if lymph nodes were large or hypermetabolic, such that eligible nodules are effectively T1N0M0. Ablation was offered to biopsy-proven lung metastasis if the primary tumour was treated with curative intent and all oligometastases in body could be treated. For the nodules which did not have histology either due to failed or refused biopsy, ablation was only offered if they were radiologically suspicious on serial imaging, and with patients’ prior informed consent. During the period from March 2019 to June 2020, 25 patients with 30 nodules were included in the study.
ENB microwave ablation procedure
All patients had a plain fine-cut CT scan within 3 months from the scheduled ablation date for pre-operative planning of ENB pathway.
Treatment procedure is summarized in Table S1. On the day of procedure, the patient was intubated inside our hybrid theatre with cone-beam CT (CBCT) and fluoroscopy capabilities. After the first CBCT, navigation to the target lung lesions was performed under ENB with SuperDimensionTM Navigation System (CovidienTM, Plymouth, MN, USA) as per previously described (15) (Figure S1). Upon reaching segmental bronchus, a locatable guide (LG) within an extended working channel (EWC) was advanced through the bronchoscope following the navigational route until reaching the target (Figure S2). In cases where bronchus sign was absent and transbronchial access was required, CrossCountryTM Transbronchial Access Tool (CovidienTM, Plymouth, MN, USA) was utilized (Video 1). A needle was deployed into the lesion and a second CBCT was done to confirm desired location of needle tip within or closely abutting lesion. The CrossCountryTM dilator was then used to create the transbronchial access (Video 2). Biopsy was performed at this stage if necessary.
The needle and dilator were then exchanged to the EmprintTM Ablation Catheter with ThermosphereTM technology (CovidienTM, Plymouth, MN, USA) (Figure S3). The EWC was retracted to unsheathe the tip of microwave catheter (Videos 3,4), and a third CBCT was performed to confirm accurate positioning of the catheter. An appropriate ablation energy was determined and predicted margin was measured. Microwave ablation was then carried out (Figure 1, Figure S3). After cooling for 10 minutes, the first post-ablation CT scan was done to assess the inclusion of lesion into ablation zone, the size of ablation zone and the minimal margin achieved. Double ablation may be performed by either re-ablating in same position, pull-back and re-ablate, or re-navigation and re-ablate (termed as “bracket ablation”) (Figure S4).
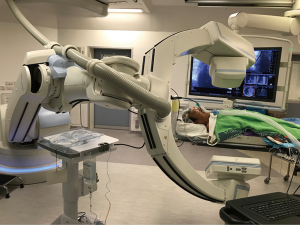
Follow up, response evaluation and data analysis
Chest X-rays were performed on post-operative day 0 and 1, and patients were discharged earliest at 1 day after ablation if no complications arose. Post-ablation fine-cut plain CT scans were arranged at first month, 3rd month, 6th month, 9th month and 12th month, but the full set was not possible for some cases due to patient default or resource restrictions in our public hospital centre. Additional CT scans were done in case of any significant complications. Private scans including PET/CT or CT thorax with contrast were also evaluated if they were available for review. The response to ablation was evaluated by qualified radiologists for local control using the modified Response Evaluation Criteria in Solid Tumours (mRECIST). Statistical analyses were performed with Microsoft Excel 2010 and IBM® SPSS® Statistics 20. Sample size was not estimated due to the retrospective nature of study. Patient and nodule characteristics were summarized with descriptive statistics, while post-ablation CT appearances and clinical outcomes were compared using Student’s t test and chi-square test as appropriate. A P value of ≤0.05 was considered significant.
Results
Baseline characteristics
Total of 25 patients were enrolled between March 2019 and June 2020. Patient characteristics are summarized in Table 1 and Table S2. Thirty lung nodules were ablated, in which 5 patients had more than one lung nodule ablated. Concomitant ENB biopsy of target lesion was performed in 6 cases.
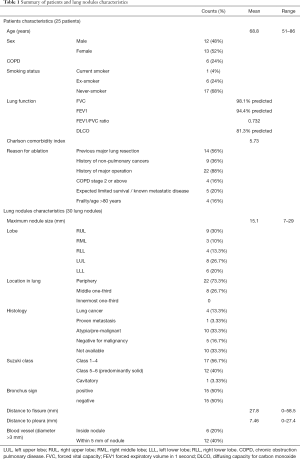
Full table
All patients had absolute or relative contraindications to surgery, including poor lung reserve or previous contralateral lobectomy, or comorbidities limiting survival, for instance significant cardiopulmonary disease or know metastatic disease. Fourteen patients (56%) had previous lung operations, 78.6% of those had previous contralateral lobectomies making single-lung ventilation potentially problematic. Patients included in the study had significant multiple comorbidities and the median Charlson comorbidity index was 6.
The mean maximal diameter of lung nodule size was 15.1 mm (range, 7 to 29 mm). Biopsy of lung nodules were either performed pre-operatively using CT-guided percutaneous biopsy, or during ENB procedure just before ablation. Pre-operative biopsy was not performed for 10 lung nodules mostly due to difficult access or patient refusal of biopsy. The histological types included lung adenocarcinomas (13.3%), atypia/pre-malignant (33.3%) and metastatic cancer (3.33%). Total of 15 nodules (50%) were negative for malignancy or no biopsy done but still radiologically suspicious. The mean distance between nodule and pleura is only 7.46 mm. Total of 6 nodules were abutting the pleura and 14 nodules were within 5 mm from pleura, making pneumothorax a potential concern.
Procedural characteristics
Procedural characteristics were summarized in Table 2. After excluding cases with concomitant biopsy, the mean total procedural time (from intubation to extubation) was 126.3 minutes. Intra-operative CBCT scans were used to plan, guide and adjust catheter locations, and the median number of CBCT required is 7.
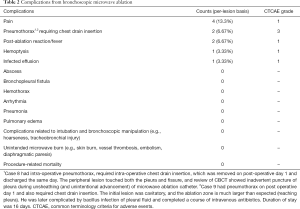
Full table
Four nodules (13.3%) required planned or unplanned double ablation to include the nodule in a satisfactory ablation zone with adequate margin. Mean procedure time for single ablation was 116 minutes while that for double ablation was 160 minutes. Concomitant biopsy also significantly lengthens procedural time to mean of 165 minutes.
Immediate post-ablation statistics
A minimum of 5mm margin was planned for each ablated nodule. The expected ablation zone border was drawn using PURE® platform (Siemens Heathineers, Germany) software and the minimum predicted margin was measured (Figure 2), which had a mean (±SD) of 6.63 (±2.89) mm. The mean (±SD) minimal actual margin after ablation was 5.51 (±2.48) mm (Table 3), and there was no significant difference between the predicted and actual minimal margin (P=0.086). Technical success rate, defined as inclusion of lesion into ablation zone with adequate margin, was 100%.
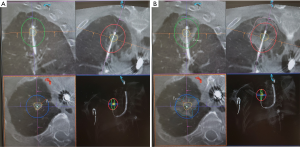
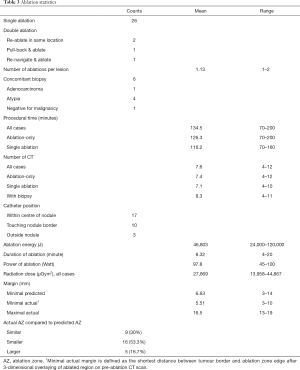
Full table
Tissue contractions occur after ablation, and should be taken into consideration when determining whether adequate margin had been achieved. The contraction percentage is not a constant, but rather is inversely proportional to the distance from the centre of ablation (Figure 3A). This is the so-called “black hole” theory, meaning that contraction is more pronounced when a reference point is closer to the centre of ablation, in analogy of a black hole where objects closer to the centre experience a stronger attraction force. Each patient had different contraction curves, with examples shown in Figure 3B. When all patient’s data was pooled on a scatter plot (Figure 3C), there is a rough maximal contraction percentage for each distance, for example a maximum of 40% contraction for a 20 mm distance from centre. In general, the contraction percentage ranges from 0–43%.
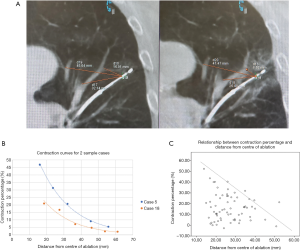
The post-ablation zone volume was generally smaller than the predicted volume, with a mean of −21.4% (SD =38.9%), but this was without consideration of contraction. The actual ablation zones were as predicted (9 cases) or larger (5 cases) in 46.7% of cases. The cases with smaller-than-predicted ablation zone were analyzed against possible factors affecting the ablation zone size, including presence of COPD, solidity of lesion, and presence of ≥3 mm blood vessels close to nodules, but none show statistically significant results.
Regarding heat sink effect, there is no statistically significant correlation between a smaller ablation zone with the presence of a sizeable blood vessel (diameter ≥3 mm) within 5mm of nodule. Presence or absence of bronchus sign does not significantly affect fluoroscopy time, radiation dose, procedure time or the number of CBCT performed. In terms of learning curve, the first and second half of 22 ablation-only cases were compared. There was a trend towards shorter mean procedural time in the latter half of cases (139 vs. 111 minutes, P=0.075) (Figure S5).
Discharge statistics, safety and complications
The median length of hospital stay is 1 day, while the mean is 1.73 days. The great majority of cases (23 cases) were discharged on post-operative day 1, while 6 cases stayed for 2–3 days due to frailty or unrelated complaints.
There was a low rate of complications (Table 2). Mild immediate or delayed pleuritic chest pain was present in 4 cases (13.3%) and responded to common oral analgesics and did not prolong hospital stay. All 4 of the lesions were close to pleura, and the mechanism of pain could be due to pleural irritation after ablation of peripherally located lung nodules. No referred pain to shoulder due to phrenic nerve injury, or referred pain to arm due to brachial plexus injury occurred. Pneumothorax occurred in 2 cases (6.67%) and both required chest drain insertion. Post ablation reaction including fever occurred in 2 cases (6.67%); while mild hemoptysis was noted in 1 case (3.33%). There were only 2 CTCAE (common terminology criteria for adverse events) grade 3 events.
Follow up and response
Median duration of follow up was 349 days (range from 132 to 615 days). Fifteen cases have completed at least 6-month post-ablation CT scans, with 8 of them already had post-ablation 1 year CT (Table 4). Complete response was achieved in 1 patient, partial response in 12 patients, and stable disease in the remaining 2 patients. There was no progressive disease identified. One unrelated death (case 2) occurred at 12 months after ablation. Morphology of ablated tumour on CT at one month post-ablation is summarized in Table S3.
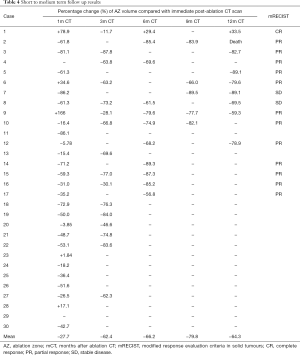
Full table
Regarding the ablation zone volumes in follow up CTs, there is a mean shrinkage of 27.8% when comparing first month CT to immediate post-ablation CT, however the variance is quite large. 60% of cases became smaller, 23.3% had static sizes. For the 10% of cases which got larger, on average there was a 93.4% increase in size, and were mostly those showing morphology of cavities. After that, the ablation zone continued to shrink, at a faster rate initially and slowing with time, eventually stabilizing in size by 9th to 12th month. In our series, compared to first month CT, ablation zone volume reduced by 66.2% at 6th month, and 64.3% by 12 months (Figure 4).
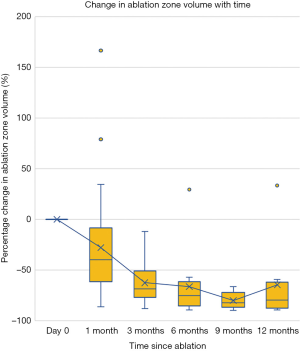
Discussion
This retrospective study has demonstrated safety and feasibility of bronchoscopic transbronchial microwave ablation in management of lung nodules. Microwave energy for lung ablation demonstrates superior properties over its predecessor radiofrequency energy. Although both are electromagnetic energy that utilizes thermal heat to achieve tissue coagulation necrosis and cell death, radiofrequency energy induces frictional heating which occurs within only a few millimeters of the electrode, and the ablation zone size is mainly enlarged by thermal conductance, but charred and desiccated tissue surrounding the electrode increases electrical impedance (16). In contrast, microwave energy devices directly heat tissue to lethal temperatures through dielectric hysteresis (17). Being less dependent on electrical conductance, microwave energy deposition has less heat-sink effect (17), is less susceptible to tissue impedance, and is able to produce faster, larger and more predictable ablation zones (11). In our series, the presence of nearby blood vessel did not significantly affect ablation zone volumes, although a mild local reduction in ablated area can sometimes be seen in certain cases.
Percutaneous microwave ablation for lung tumours produced reasonable results, with cancer-specific survivals ranging between 69–84.3% at 1 year, 42.1–61% at 3 years, recurrence rate of 44% at 3 years, while median time to local tumour recurrence was between 22.6–62 months (7,18-22). In a retrospective study comparing percutaneous microwave ablation and lobectomy for stage 1 non-small cell lung cancers (NSCLC), there was no significant difference in overall survival and disease-free survival (23).
Most of the thermal ablative techniques in literature applied the electrode in a percutaneous manner. Lately, transbronchial ablation techniques using different energy source have been researched in animal lung models (24-26). In humans, bronchoscopy-guided transbronchial ablation have been attempted by a Japanese and a Chinese group, utilizing RFA energy with reported safety (27-29). More recently, small case series of image-guided transbronchial microwave ablation of lung tumours have been reported by 2 separate groups with favourable benefit-risk profiles (30,31). Compared to percutaneous techniques, a major advantage of bronchoscopic ablation is avoidance of pleural puncture, and hence fewer pleural-based complications, including pneumothorax (6,10,32,33), bronchopleural fistulae (34) and needle tract pleural seeding (35). Another edge of bronchoscopic ablation is its ability to reach certain regions of lung which are otherwise difficult or dangerous for percutaneous route, for instance areas near mediastinal pleura or diaphragm, lung apex, and areas shielded by scapula. With evidence of safety and technical success of bronchoscopic microwave ablation in animal models (36), the authors’ institute is one of the first to have performed ENB-guided microwave ablation on patients.
Our data supports the safety of bronchoscopic ablation compared to percutaneous route. Only 2 cases (6.67%) developed pneumothorax, and one of the pneumothorax may have been avoided if the catheter had not been advanced so far in as to puncture the pleura. The other pneumothorax likely resulted from rupture of a rapidly-enlarging cavity. The ablation zone reached pleura in 66.7% of our cases, indicating that an ablated pleura, as long as not punctured, is generally safe and does not produce pneumothorax. There was only 1 case (3.33%) of pleural effusion necessitating drainage, lower than the 6–19% reported in percutaneous ablation (10). The rate of other complications, 6.67% for post-ablation reaction, 3.33% for hemoptysis/bronchopulmonary haemorrhage, and 3.33% for infective complications, were comparable to percutaneous ablation (10).
Only selective cases are suitable for bronchoscopic transbronchial microwave ablation. Numerous lung nodule ablation studies have correlated risk of local recurrence with nodule size, with cut-off of 1.5, 2 and 3 cm respectively (6,7,19). It should be noted that the typical early post-ablative morphology of concentric GGOs on CT actually contains an outer rim of denser GGO containing congested lung tissue that retains viability (37). The size seen on CT scan overestimate the area of true coagulation necrosis by 4.1 mm (38) or even up to 8–9 mm (39). Therefore, multiple authors recommended a margin of at least 5 mm on CT scan assessment to ensure adequate tumour kill (18,38,40). For the Emprint microwave catheter used in the present study, the spheroidal maximal ablation zone width is 35 mm at 100 W for 10 minutes, being derived from in-vivo experiments in healthy porcine lungs (Figure S6). Taking into consideration a desired margin of at least 5mm on either side of lesion, the maximal nodule size suitable for ablation should be about 25mm, unless double ablation is planned.
The actual ablation zone volumes immediately post-ablation were generally smaller by a mean of −21.4% when compared to the predicted ablation zone dimensions provided by the manufacturer. A further analysis of the one-month ablation zone volume revealed a large variance in ablation zone sizes, ranging from −86.2% to +166%. The size of tumors, solidity of nodules and the presence of nearby blood vessels were not predictive of ablation zone sizes. The predicted ablation zone dimensions were derived from manufacturer’s pre-clinical study in living healthy swine. The mismatch between predicted and actual ablation zone may be due to variations in lung properties between human and porcine lung (41), and differences in impedance and conductivity between normal lungs and tumour tissues.
It has been observed that tissue contraction occurred in most thermal ablation in different organs (16). To date only limited information on tissue contraction in human lung ablation is available. We found that contraction percentage is higher in the centre as opposed to periphery (black hole theory) (Figure 3B), but exact contraction percentage varies between individuals (Figure 3C). This may be explained by the differences in architecture and water content (16), lung nodule characteristics, and presence of surrounding blood vessels. Our findings may have significant implications on ablative margin and ultimately the technical success. By taking this phenomenon into account, clinicians may determine the ablative volume more accurately and reduce the risk of overcompensation with additional ablations.
Our study is limited by its retrospective nature, absence of parallel arm, and lack of histology for some lesions. Moreover, the contrasting effects of underestimation of ablation zone by CT due to tissue contraction and the overestimation of ablation zone by CT appearance indicates that CT is only fairly reliable in assessing and monitoring patient’s response to ablation. Multi-phase contrast-enhanced CT, PET/CT and dynamic contrast-enhanced MRI are better imaging modalities (37,42) but are not employed in the current study due to their limited availability. Nevertheless, current data is encouraging and support future prospective study on bronchoscopic microwave ablation. It will also be important to investigate the key parameters affecting ablation zone size such as lung densitometry and water content measurements. Long term results including local control and survival rates are also key endpoints to observe. Combined with simultaneous intra-tumoural chemotherapy (43), or enhanced permeability and retention (EPR) effect via hyperthermia induced by ablation (44), transbronchial lung cancer ablation will likely become an important part of the armamentarium in an exciting era of personalized cancer treatment.
Conclusions
Bronchoscopic transbronchial microwave ablation is safe, feasible and potentially effective. This novel technique may represent a future treatment modality for early stage lung cancers and lung metastases. Together with ENB biopsy and intra-operative pathological evaluation, bronchoscopic microwave ablation in the hybrid theater is capable of providing a one-stop diagnosis and treatment for suspicious lung nodules (45).
Acknowledgments
Funding: This work was supported by Research Grants Council (RGC) Hong Kong, General Research Fund (GRF) (no: 14119019). The Fund has no influence on the content of the present article.
Footnote
Reporting Checklist: The authors have completed the STROBE reporting checklist. Available at http://dx.doi.org/10.21037/tlcr-20-1231
Data Sharing Statement: Available at Available at http://dx.doi.org/10.21037/tlcr-20-1231
Peer Review File: Available at Available at http://dx.doi.org/10.21037/tlcr-20-1231
Conflicts of Interest: All authors have completed the ICMJE uniform disclosure form (available at Available at http://dx.doi.org/10.21037/tlcr-20-1231). CSHN is a consultant for Johnson and Johnson; Medtronic, USA; and Siemens Healthineer. RWHL is a consultant for Medtronic, USA; and Siemens Healthineer. TSKM has no potential conflicts of interest that exist with companies/organizations whose products or services are discussed in this article. The other authors have no conflicts of interest to declare.
Ethical Statement: The authors are accountable for all aspects of the work in ensuring that questions related to the accuracy or integrity of any part of the work are appropriately investigated and resolved. The study was conducted in compliance with the Declaration of Helsinki (as revised in 2013) and approved by local institutional review board (the Joint Chinese University of Hong Kong – New Territories East Cluster Clinical Research Ethics Committee), CREC reference number of 2020.524. All cases were discussed in multi-disciplinary meetings with oncologists’ input, and all study participants gave informed consent before taking part in the study.
Open Access Statement: This is an Open Access article distributed in accordance with the Creative Commons Attribution-NonCommercial-NoDerivs 4.0 International License (CC BY-NC-ND 4.0), which permits the non-commercial replication and distribution of the article with the strict proviso that no changes or edits are made and the original work is properly cited (including links to both the formal publication through the relevant DOI and the license). See: https://creativecommons.org/licenses/by-nc-nd/4.0/.
References
- Aberle DR, Adams AM, Berg CD, et al. Reduced Lung-Cancer Mortality with Low-Dose Computed Tomographic Screening. N Engl J Med 2011;365:395-409. [Crossref] [PubMed]
- Bryant AK, Mundt RC, Sandhu AP, et al. Stereotactic Body Radiation Therapy Versus Surgery for Early Lung Cancer Among US Veterans. Ann Thorac Surg 2018;105:425-31. [Crossref] [PubMed]
- Puri V, Crabtree TD, Bell JM, et al. Treatment Outcomes in Stage I Lung Cancer: A Comparison of Surgery and Stereotactic Body Radiation Therapy. J Thorac Oncol 2015;10:1776-84. [Crossref] [PubMed]
- Lee K, Kim HO, Choi HK, et al. Real-world treatment patterns for patients 80 years and older with early lung cancer: A nationwide claims study. BMC Pulm Med 2018;18:127. [Crossref] [PubMed]
- Senthi S, Lagerwaard FJ, Haasbeek CJA, et al. Patterns of disease recurrence after stereotactic ablative radiotherapy for early stage non-small-cell lung cancer: A retrospective analysis. Lancet Oncol 2012;13:802-9. [Crossref] [PubMed]
- Dupuy DE, Fernando HC, Hillman S, et al. Radiofrequency ablation of stage IA non-small cell lung cancer in medically inoperable patients: Results from the American College of Surgeons Oncology Group Z4033 (Alliance) trial. Cancer 2015;121:3491-8. [Crossref] [PubMed]
- Healey TT, March BT, Baird G, et al. Microwave Ablation for Lung Neoplasms: A Retrospective Analysis of Long-Term Results. J Vasc Interv Radiol 2017;28:206-11. [Crossref] [PubMed]
- Niu L, Xu K, Mu F. Cryosurgery for lung cancer. J Thorac Dis 2012;4:408-19. [PubMed]
- Onishi H, Marino K, Yamashita H, et al. Case series of 23 patients who developed fatal radiation pneumonitis after stereotactic body radiotherapy for lung cancer. Technol Cancer Res Treat 2018;17:1533033818801323 [Crossref] [PubMed]
- Hiraki T, Gobara H, Fujiwara H, et al. Lung cancer ablation: Complications. Semin Intervent Radiol 2013;30:169-75. [Crossref] [PubMed]
- Brace CL, Hinshaw JL, Laeseke PF, et al. Pulmonary thermal ablation: Comparison of radiofrequency and microwave devices by using gross pathologic and CT findings in a swine model. Radiology 2009;251:705-11. [Crossref] [PubMed]
- Harris K, Puchalski J, Sterman D. Recent Advances in Bronchoscopic Treatment of Peripheral Lung Cancers. Chest 2017;151:674-85. [Crossref] [PubMed]
- Ng CS, Zhao Z, Long H, et al. Electromagnetic Navigation Bronchoscopy Triple Contrast Dye Marking for Lung Nodule Localization. Thorac Cardiovasc Surg 2020;68:253-5. [Crossref] [PubMed]
- Zhao ZR, Lau RWH, Ng CSH. Electromagnetic navigation bronchoscopy in hybrid theater. Front Surg 2019;6:10. [Crossref] [PubMed]
- Zhao ZR, Lau RWH, Yu PSY, et al. Image-guided localization of small lung nodules in video-assisted thoracic surgery. J Thorac Dis 2016;8:S731-7. [Crossref] [PubMed]
- Kim C. Understanding the nuances of microwave ablation for more accurate post-treatment assessment. Future Oncol 2018;14:1755-64. [Crossref] [PubMed]
- Lubner MG, Brace CL, Hinshaw JL, et al. Microwave tumor ablation: Mechanism of action, clinical results, and devices. J Vasc Interv Radiol 2010;21:S192. [Crossref] [PubMed]
- Wolf FJ, Grand DJ, Machan JT, et al. Microwave ablation of lung malignancies: Effectiveness, CT findings, and safety in 50 patients. Radiology 2008;247:871-9. [Crossref] [PubMed]
- Vogl TJ, Worst TS, Naguib NNN, et al. Factors influencing local tumor control in patients with neoplastic pulmonary nodules treated with microwave ablation: A risk-factor analysis. AJR Am J Roentgenol 2013;200:665-72. [Crossref] [PubMed]
- Belfiore G, Ronza F, Belfiore MP, et al. Patients’ survival in lung malignancies treated by microwave ablation: Our experience on 56 patients. Eur J Radiol 2013;82:177-81. [Crossref] [PubMed]
- Pusceddu C, Melis L, Sotgia B, et al. Usefulness of percutaneous microwave ablation for large non-small cell lung cancer: A preliminary report. Oncol Lett 2019;18:659-66. [Crossref] [PubMed]
- Ko WC, Lee YF, Chen YC, et al. CT-guided percutaneous microwave ablation of pulmonary malignant tumors. J Thorac Dis 2016;8:S659-65. [Crossref] [PubMed]
- Yao W, Lu M, Fan W, et al. Comparison between microwave ablation and lobectomy for stage I non-small cell lung cancer: a propensity score analysis. Int J Hyperthermia 2018;34:1329-36. [Crossref] [PubMed]
- Yoneda KY, Herth F, Spangler T, et al. Long-term Survival Results following Endobronchial RF Ablation in a Healthy-Porcine Model. Annu Int Conf IEEE Eng Med Biol Soc 2020;2020:5252-8. [Crossref] [PubMed]
- Sebek J, Kramer S, Rocha R, et al. Bronchoscopically delivered microwave ablation in an in vivo porcine lung model. ERJ Open Res 2020;6:00146-2020. [Crossref] [PubMed]
- Casal RF. Cone Beam CT-Guided Bronchoscopy. J Bronchology Interv Pulmonol 2018;25:255-6. [Crossref] [PubMed]
- Tanabe T, Koizumi T, Tsushima K, et al. Comparative study of three different catheters for CT imaging-bronchoscopy- guided radiofrequency ablation as a potential and novel interventional therapy for lung cancer. Chest 2010;137:890-7. [Crossref] [PubMed]
- Koizumi T, Tsushima K, Tanabe T, et al. Bronchoscopy-Guided Cooled Radiofrequency Ablation as a Novel Intervention Therapy for Peripheral Lung Cancer. Respiration 2015;90:47-55. [Crossref] [PubMed]
- Xie F, Zheng X, Xiao B, et al. Navigation Bronchoscopy-Guided Radiofrequency Ablation for Nonsurgical Peripheral Pulmonary Tumors. Respiration 2017;94:293-8. [Crossref] [PubMed]
- Lau K, Spiers A, Pritchett M, et al. P1.05-06 Bronchoscopic Image-Guided Microwave Ablation of Peripheral Lung Tumours – Early Results. J Thorac Oncol 2018;13:S542. [Crossref]
- Pritchett M, Reisenauer J, Kern R, et al. Image-Guided Transbronchial Microwave Ablation of Peripheral Primary Lung Tumors with a Flexible Probe: First in US Experience. Chest 2020;158:A1452-3. [Crossref]
- Zheng A, Wang X, Yang X, et al. Major complications after lung microwave ablation: A single-center experience on 204 sessions. Ann Thorac Surg 2014;98:243-8. [Crossref] [PubMed]
- Kashima M, Yamakado K, Takaki H, et al. Complications After 1000 Lung Radiofrequency Ablation Sessions in 420 Patients: A Single Center’s Experiences. AJR Am J Roentgenol 2011;197:W576-80 [Crossref] [PubMed]
- Sakurai J, Hiraki T, Mukai T, et al. Intractable Pneumothorax Due to Bronchopleural Fistula after Radiofrequency Ablation of Lung Tumors. J Vasc Interv Radiol 2007;18:141-5. [Crossref] [PubMed]
- Hiraki T, Mimura H, Gobara H, et al. Two Cases of Needle-Tract Seeding after Percutaneous Radiofrequency Ablation for Lung Cancer. J Vasc Interv Radiol 2009;20:415-8. [Crossref] [PubMed]
- Yuan HB, Wang XY, Sun JY, et al. Flexible bronchoscopy-guided microwave ablation in peripheral porcine lung: A new minimally-invasive ablation. Transl Lung Cancer Res 2019;8:787-96. [Crossref] [PubMed]
- Chheang S, Abtin F, Guteirrez A, et al. Imaging features following thermal ablation of lung malignancies. Semin Intervent Radiol 2013;30:157-68. [Crossref] [PubMed]
- Yamamoto A, Nakamura K, Matsuoka T, et al. Radiofrequency Ablation in a Porcine Lung Model: Correlation Between CT and Histopathologic Findings. AJR Am J Roentgenol 2005;185:1299-306. [Crossref] [PubMed]
- Meram E, Longhurst C, Brace CL, et al. Comparison of Conventional and Cone-Beam CT for Monitoring and Assessing Pulmonary Microwave Ablation in a Porcine Model. J Vasc Interv Radiol 2018;29:1447-54. [Crossref] [PubMed]
- Anderson EM, Lees WR, Gillams AR. Early indicators of treatment success after percutaneous radiofrequency of pulmonary tumors. Cardiovasc Intervent Radiol 2009;32:478-83. [Crossref] [PubMed]
- Judge EP, Hughes JML, Egan JJ, et al. Anatomy and bronchoscopy of the porcine lung: A model for translational respiratory medicine. Am J Respir Cell Mol Biol 2014;51:334-43. [Crossref] [PubMed]
- Chen J, Lin ZY, Wu ZB, et al. Magnetic resonance imaging evaluation after radiofrequency ablation for malignant lung tumors. J Cancer Res Ther 2017;13:669-75. [Crossref] [PubMed]
- Ueki A, Okuma T, Hamamoto S, et al. Combination therapy involving radiofrequency ablation and targeted chemotherapy with bevacizumab plus paclitaxel and cisplatin in a rabbit VX2 lung tumor model. BMC Res Notes 2018;11:251. [Crossref] [PubMed]
- Golombek SK, May JN, Theek B, et al. Tumor targeting via EPR: Strategies to enhance patient responses. Adv Drug Deliv Rev 2018;130:17-38. [Crossref] [PubMed]
- Chan JWY, Yu PSY, Lau RWH, et al. Hybrid operating room—one stop for diagnosis, staging and treatment of early stage NSCLC. J Thorac Dis 2020;12:123-31. [Crossref] [PubMed]