A novel bioassay reflecting response to immune checkpoint inhibitor therapy in non-small cell lung cancer with malignant pleural effusion
Highlight box
Key findings
• The aim of this study was to identify prognostic and predictive biomarkers for immune checkpoint inhibitor (ICI) therapy in patients with advanced lung cancer by analyzing malignant pleural effusion (MPE).
• We developed an in vitro assay to measure interferon-gamma (IFN-γ) levels following administration of nivolumab to the whole-cell component of MPE from patients with advanced non-small cell lung cancer.
What is known and what is new?
• There were few research studies regarding parameters in MPE. We proposed several biomarkers in pleural effusion as prognostic factors, including levels of albumin, vascular endothelial growth factor (VEGF), high mobility group box 1 (HMGB1), and the percentage of lymphocytes.
• Furthermore, we developed an in vitro bioassay employing the whole-cell component of MPE and reflecting the immune environment of the pleural cavity. ICI-binding T cells predominantly produced IFN-γ, and the levels of IFN-γ may be correlated with the therapeutic efficacy of ICI treatment.
What is the implication, and what should change now?
• This methodology is very simple because it employs the whole-cell component of the pleural effusion without requiring separation of individual cell fractions. The levels of IFN-γ in pleural effusion, as induced by ICIs, may serve as a novel biomarker for predicting the efficacy of ICI treatment.
Introduction
Background
Programmed cell death-1 (PD-1) and cytotoxic T-lymphocyte-associated protein-4 (CTLA-4) are immune checkpoint molecules (1,2). They act as inhibitory co-receptors and are essential for the regulation of T cells. Cancer evades the immune system by inactivation of T cells through expression of the ligand of these checkpoint molecules (3). Immune checkpoint inhibitor (ICI) therapy involves antibodies preventing the binding of ligands and promoting the activities of T cells. In 2015, the anti-PD-1 inhibitor nivolumab became the first approved immunotherapy for the treatment of non-small cell lung cancer (NSCLC) (4).
Rationale and knowledge gap
ICI has prolonged the survival of a proportion of patients with advanced NSCLC. However, almost 20% of patients treated with ICI experience immune-related adverse effects with or without tumor shrinkage. At present, histological quantification of the tumor proportion score of programmed cell death-ligand 1 (PD-L1) expression in tumor cells is a widely adopted biomarker used to predict the efficacy of ICI in NSCLC. In the KEYNOTE-024 trial, which enrolled patients with tumors with a PD-L1 expression status >50%, pembrolizumab (an anti-PD-1 antibody) significantly prolonged progression-free survival and overall survival (OS) (5). Several reports showed a positive correlation between PD-L1 expression and objective response rate (4,5).
However, we occasionally encounter a discrepancy between the tumor proportion score of PD-L1 and actual clinical response to ICI. Furthermore, evaluation of PD-L1 expression is impossible in cases where tissue sampling is difficult. Malignant pleural effusion (MPE) is considered an inappropriate sample for the evaluation of PD-L1 expression. The presence of MPE in NSCLC is associated with poor outcomes, the estimated median survival of patients with MPE is 4–9 months (6). Despite the advances achieved in the development of therapeutic agents, pleural drainage remains the standard strategy for the treatment of MPE.
Objective
In this study, we initially investigated the association of biomarkers in MPE with disease outcomes. Next, we sought to establish a new bioassay that reflects the clinical response to ICI treatment and investigated the possibility of clinical application. We present this article in accordance with the TRIPOD reporting checklist (available at https://tlcr.amegroups.com/article/view/10.21037/tlcr-24-559/rc).
Methods
Study design
This single-center retrospective study was conducted at Jichi Medical University Hospital (Shimotsuke, Japan). This study was approved by the Ethics Committee of Human Research at Jichi Medical University (No. A16-132). The study was conducted in accordance with the Declaration of Helsinki (as revised in 2013). Individual consent for this retrospective analysis was waived. We included consecutive patients with non-squamous NSCLC with MPE between May 2018 and August 2020. Clinical data were collected from electronic medical records.
Pleural effusion samples were obtained by thoracentesis. Clinical staging was based on the eighth edition of the tumor-node-metastasis (TNM) staging system. Systemic response was assessed according to the Response Evaluation Criteria in Solid Tumors (RECIST) version 1.1. The duration of treatment was defined from the time of ICI treatment initiation until its termination or the occurrence of cancer-related death. OS was defined from the time of diagnosis until death or the last follow-up. PD-L1 expression was determined by immunostaining with Dako PD-L1 IHC 22C3 PharmDx Assay (Agilent, Santa Clara, CA, USA). Driver gene mutations were analyzed using clinically utilized diagnostic methods on an outsourcing basis.
In vitro ICI bioassay
The MPE samples obtained from patients with NSCLC were filtered using a 70-µm cell strainer (Corning, Corning, NY, USA) and centrifuged at 1,200 rpm for 10 min at 4 ℃. Supernatants were collected and stored at −80 ℃. Each cell pellet was dissolved in 5 mL of RPMI 1640 (Sigma-Aldrich, St. Louis, MO, USA) containing 10% (volume/volume) fetal bovine serum (Sigma-Aldrich). The total number of cells was determined, and cells (1×106 per well) were placed in 300 µL of RPMI 1640 in a 96-well flat bottom microplate with nivolumab (Selleck Biotech, Yokohama, Japan) or control immunoglobulin G4 (IgG4) (Fully Human IgG4 Isotype Control; EUREKA, Emeryville, CA, USA) for 48 h. The concentrations of nivolumab and control IgG4 were adjusted to 60 µg/mL based on the average serum concentration in patients treated with nivolumab. After 48 h, cell supernatants were aspirated and centrifuged at 5,000 rpm for 10 min at 4 ℃. Subsequently, measurement of IFN-γ was performed. Cells were also cultured for over 6 h with Cell Activation Cocktail with Brefeldin A (BioLegend, San Diego, CA, USA) and harvested for flow cytometry.
Flow cytometry
Harvested cells were pre-incubated with anti-CD16/32 antibody (clone 2.4G2; BD Biosciences, Franklin Lakes, NJ, USA). Fixable Viability Stain (BD Biosciences) was used to exclude dead cells. Cell surface staining was performed with the following antibodies: anti-CD45; anti-CD3; anti-CD4; anti-CD8; and anti-CD279 (PD-1) (BD Biosciences); as well as anti-IgG4 (Abcam, Cambridge, UK). For intracellular staining, cells were fixed with a Transcription Factor Buffer Set (BD Biosciences) according to the instructions provided by the manufacturer. Intracellular staining was performed with anti-IFN-γ antibody (BD Biosciences). All samples were analyzed using the FACS LSR FortessaX-20 (BD Biosciences) and Flow Jo (Tree Star Inc., San Carlos, CA, USA) software programs.
Measurement of IFN-γ and soluble factors in pleural effusion samples
The stored supernatants of cultured samples were thawed and analyzed by sandwich-enzyme-linked immunosorbent assay (ELISA) using an IFN-γ Human ELISA kit (Thermo Fisher Scientific, Waltham, MA, USA). The stored supernatants of the original pleural effusion samples were sent to the laboratory of Shino-Test Corporation (Tokyo, Japen); several soluble factors [PD-L1, high mobility group box 1 (HMGB1), vascular endothelial growth factor (VEGF)] were assessed by ELISA.
Statistical analysis
The quantitative data were described by mean ± standard deviation. Mann-Whitney U test, one-way analysis of variance, and Pearson’s correlation test were performed for statistical analysis with GraphPad Prism version 5.0 (GraphPad Software, Inc., San Diego, CA, USA). P values <0.05 indicate statistically significant differences. OS was estimated using the Kaplan-Meier method with EZR (Saitama Medical Center, Jichi Medical University, Saitama, Japan), which is a graphical user interface for R (The Foundation for Statistical Computing, Vienna, Austria).
Results
Patient characteristics
Between May 17, 2018, and August 8, 2020, 32 patients with MPE caused by NSCLC were eligible for participation in the study. Initially, patients with small cell lung cancer, cancer of other organs, or mesothelioma were excluded. Next, although patients were diagnosed with NSCLC based on the primary tumor lesion, cytology-negative effusion samples were excluded. Finally, 22 patients (10 males and 12 females) were included in the investigation (Figure 1). The baseline characteristics are shown in Table 1. The median age was 69 years (range, 52–85 years). The majority of patients had stage IV disease (stage IVA: n=5; stage IVB: n=14), and only three patients had experienced recurrence after radical chemoradiotherapy. All patients were histologically diagnosed with adenocarcinoma. Driver gene mutations were detected in half of the patients, i.e., epidermal growth factor receptor (EGFR) mutation in 10 patients and anaplastic lymphoma kinase (ALK) rearrangement in two patients. PD-L1 expression was evaluated by immunostaining of specimens obtained from the primary tumor or cell blocks prepared from pleural effusion samples. The status of PD-L1 expression was as follows: ≥50%, 0; 1–49%, 7; <1%, 4; unknown, 11. ICI therapy was administered in half of the patients.
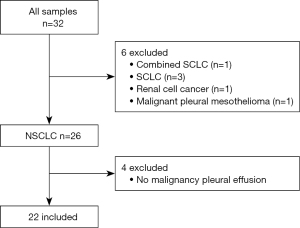
Table 1
Variables | Value (n=22) |
---|---|
Age (years) | 69 [52–85] |
Gender | |
Male | 10 |
Female | 12 |
Smoking status | |
Ex- or current smoker | 11 |
Never smoker | 11 |
Stage | |
IV | 19 |
Recurrence after CRT or surgery | 3 |
Histology | |
Adenocarcinoma | 22 |
Others | 0 |
PD-L1 status | |
≥50% | 0 |
1–49% | 7 |
<1% | 4 |
Unknown | 11 |
Driver mutation | |
EGFR | 10 |
ALK | 2 |
Unknown or wild type | 10 |
Immune checkpoint inhibitor therapy | |
No | 11 |
Yes | 11 |
Data are presented as median [range] or number. CRT, chemoradiotherapy; PD-L1, programmed cell death-ligand 1; EGFR, epidermal growth factor receptor; ALK, anaplastic lymphoma kinase.
OS and prognostic biomarkers in pleural effusion samples
OS of participants from this study was shown in Figure 2. The median OS was 20.4 months (95% confidence interval: 11.5–41.0). This long survival was observed in half of the patients with EGFR mutations. Next, several parameters were selected to identify biomarkers that correlate with OS: total cell numbers; each fraction of cells (neutrophils, lymphocytes, monocytes, atypical cells); and the concentration of albumin, lactate dehydrogenase (LDH), soluble PD-L1, HMBG1, and VEGF121 in pleural effusion samples (Figure S1 and Figure 3A). We found a significant positive correlation with albumin levels and a significant negative correlation with the number of lymphocytes (Figure 3A). Since OS was highly influenced by the presence of a driver gene mutation, we classified the patients into driver gene mutation-positive (EGFR, ALK) or -negative groups, and the correlation with OS was evaluated in the same manner. In the EGFR and ALK-positive group, we also found positive correlations between OS and albumin levels, and negative correlation between OS and the percentage of lymphocytes. Additionally, HMGB1 and VEGF121 showed a significant negative correlation (Figure 3B). In contrast, there were no useful parameters detected in the driver gene mutation-negative group (Figure 3C).
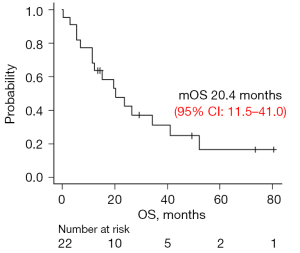
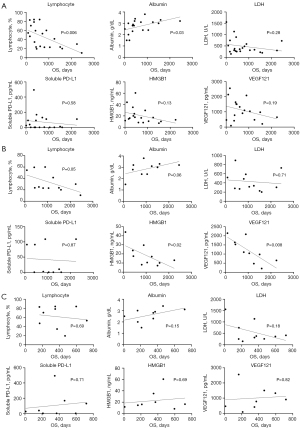
Establishment of in vitro ICI bioassay
To reconstruct the immune environment in vitro, we designed the original culture system, in which tumor cells and immune cells obtained from pleural effusion samples were co-cultured with an ICI (nivolumab) (Figure 4). Initially, we identified T cells (CD45+CD3+ cells) and distinguished CD4+ and CD8+ T cells in the pleural effusion samples (Figure S2A). In MPE, CD4+ T cells were dominant and the number of T cells and proportion of CD4+ and CD8+ T cells were almost similar in individuals with or without ICI (Figure S2B). We successfully demonstrated the binding of nivolumab on the cell surface of both CD4+ and CD8+ T cells; such binding was not observed with the control IgG4 antibody (Figure 5, Figure S3A). In addition, the expressions of CD279 (PD-1) on the surface of T cells were decreased by the binding of nivolumab (Figure 5B, Figure S3B). Next, we examined whether nivolumab would enhance the anti-tumor activities of T cells. Nivolumab-binding T cells showed predominant production of IFN-γ compared with control IgG4 antibody (Figure 5A, Figure S3A). In particular, nivolumab amplified IFN-γ-release from CD8+ compared with CD4+ T cells. On the other hand, it is well established that IFN-γ is also produced by monocytes; however, we could not detect both binding of nivolumab on CD45+ CD14+ monocytes and IFN-γ-release in this assay (Figure S4). Therefore, IFN-γ production in this assay was considered to be derived from T cells in response to nivolumab.
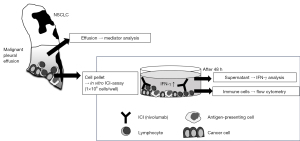
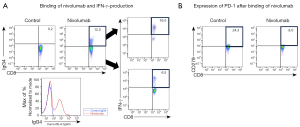
Release of IFN-γ in in vitro bioassay and correlation analysis
Subsequently, we analyzed the released IFN-γ in the supernatant of the bioassay system in 19 patients (Figure 6A). We found significant individual variation in the levels of IFN-γ production. Among 19 patients, 12 showed increase in IFN-γ after treatment with nivolumab; however, the remaining seven patients did not show an increase in IFN-γ. This is considered individual variability in response to treatment with nivolumab and may be comparable to the response observed after systemic nivolumab treatment. In addition, we analyzed the correlation between released IFN-γ and several biomarkers. The concentration of released IFN-γ was positively correlated with soluble PD-L1 and negatively correlated with VEGF121 (Figure 6B). Next, we investigated the relationship between IFN-γ and clinical parameters, specifically OS and the duration of ICI treatment, in patients receiving ICI (n=10). This analysis did not reveal significant correlations. Although the differences were not statistically significant, the duration of ICI treatment tended to be longer in patients with IFN-γ release (>0 pg/mL) (n=7) versus those without IFN-γ release (n=3) (Figure 7A,7B). The present findings suggest that evaluation of IFN-γ release after treatment with nivolumab through our immune bioassay may help identify responders prior to ICI treatment.
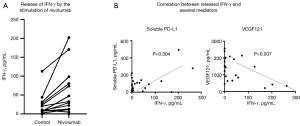
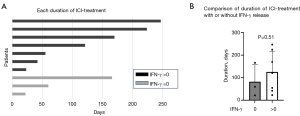
Discussion
In this study, we explored markers in MPE associated with the efficacy of ICI therapy and prognosis of NSCLC that could be useful in clinical practice. OS and the percentage of lymphocytes and the levels of albumin in MPE were statistically correlated in all eligible patients. In addition, the levels of VEGF were negatively correlated with OS only in patients with driver gene mutations. Furthermore, we established an in vitro bioassay to assess the immune activation status of patients by measuring IFN-γ levels following the co-culture of ICI with the cell component of MPE. The duration of ICI treatment tended to be longer in patients with IFN-γ release, suggesting that this method has the potential to predict the efficacy of ICI.
Biomarkers of lung cancer are generally identified in blood, and there is currently a low number of pleural effusion-derived candidates. Thus far, it has been reported that high levels of LDH (7), low levels of protein (8), a high neutrophil-to-lymphocyte ratio (9), and an increase in the number of CD4+ T cells (10) in MPE are correlated with poor prognosis of NSCLC. In our study, the positive correlation of albumin with OS was in line with previous findings, implying that nutritional status and systemic metabolic function were also important for prognosis. Unexpectedly, the percentage of lymphocytes in MPE exhibited a negative correlation with OS, in contrast to the results of a previous study (9). We suspected that an increase in regulatory T cells might influence the anti-tumor activity, or highly inflammatory effusion might exaggerate cachexia and lead to poor prognosis. In this study, we could not classify the immune phenotype of lymphocytes; hence, further analyses of T cells in MPE are warranted to clarify the precise role of lymphocytes in MPE.
PD-L1 expression in tumor tissue is a well-established marker in NSCLC. Its expression in pleural fluid cell blocks showed high concordance with that recorded in primary solid tumors (11,12). However, our data indicated that soluble PD-L1 in MPE did not show any correlations with OS (Figure 3). Recently, Hayashi et al. reported that soluble PD-L1 and soluble CTLA-4 in blood reflected overactivation or an exhaustion status of antitumor immunity, while patients with high soluble PD-L1 levels were linked to poor outcome (13). Therefore, the impact of soluble PD-L1 in MPE remains controversial. In the in vitro bioassay, PD-L1 showed a significant correlation with the levels of released IFN-γ (Figure 6B). Based on this observation, we speculated that soluble PD-L1 in MPE might reflect a potential of immune reactivity against ICI and may be a predictive factor of the efficacy of ICI in NSCLC with MPE.
NSCLC patients with a driver oncogene mutation, such as EGFR mutations and ALK rearrangements, are considered poor responders to ICI treatment (14). In particular, in EGFR-mutated NSCLC, the clinical response to ICI treatment is insufficient even in cases with high PD-L1 expression (15). This highlights the difficulty in predicting the efficacy of ICI based on PD-L1 testing in MPE of EGFR-mutant NSCLC. VEGF121 were one of the splicing variants of VEGF; thus far, four splicing variants (VEGF121, 165, 189, 206) were identified (16). Particularly, VEGF121 and 165 could be detected in pleural effusion. We found significant correlation with VEGF121. In our study, low levels of VEGF121 in MPE were associated with good OS in patients with EGFR-mutant NSCLC. Moreover, in all patients, the levels of VEGF121 were negatively correlated with IFN-γ release in the ICI bioassay. VEGF generally promotes angiogenesis, increases vascular permeability, and has immune suppressive function by affecting immune cells, including T cells (16). In particular, VEGF is involved in the induction and maintenance of regulatory T cells (17). Clinical trial data demonstrated that the median progression-free survival of EGFR mutation-positive patients was longer after treatment with erlotinib plus bevacizumab versus erlotinib alone, especially in those with pleural effusion (18,19). Therefore, our results reinforced the appropriateness and clinical benefit of combination therapy with an EGFR-tyrosine kinase inhibitor (TKI) and a VEGF inhibitor in EGFR-mutant NSCLC with MPE. Additionally, several investigations demonstrated that extracellular HMGB1 is an immunogenic factor associated with tumor progression and unfavorable prognosis in various types of cancer (20,21). Therefore, the negative correlation of HMGB1 with OS observed in the present study is compatible with this notion. However, further investigation is required to elucidate the role of HMGB1 in driver-mutant NSCLC with MPE.
Next, we developed a new in vitro bioassay to reflect the clinical response to ICI. Thus far, several in vitro bioassays, such as three-dimensional culture and organoid formation, have been proposed for the evaluation of cytotoxic agents in patients with MPE (22,23). Pre-existing bioassays were mainly constructed by cancer cells obtained from each primary site and immune cells from peripheral blood. However, these assays are technically complicated and do not demonstrate the actual immune microenvironment. This is because MPE contains various immune cells and immunomodulators. Therefore, we established this simple co-culture bioassay, in which the whole-cell component of MPE (including cancer cells, immune cells, and stromal cells) was incubated with ICI. Importantly, the cellular component of the pleural fluid varied between cases, and may reflect the immune environment of individual patients.
The cytokine IFN-γ plays a key role in the activation of the anti-tumor immune response. Recognition of tumor antigens by T cells results in the release of IFN-γ, which acts as a cytotoxic cytokine (24). In our in vitro bioassay, we detected an increase in IFN-γ in approximately one-third of the patients in response to ICI. In this respect, our in vitro assay mimics the tumor microenvironment in the pleural cavity, and the increase in IFN-γ-release may reflect the individual anti-tumor activity. Furthermore, the levels of released IFN-γ in the bioassay correlated with those of soluble PD-L1 and VEGF. This evidence suggested that soluble PD-L1 and VEGF are immune modulators and may be associated with the efficacy of ICI in the pleural cavity, despite the absence of a positive correlation with OS. Regarding the association with clinical factors, the duration of ICI treatment tended to be longer in patients with IFN-γ release versus those without IFN-γ release. Thus, screening for IFN-γ release after treatment with nivolumab using our in vitro immune assay may help identify responders prior to ICI treatment among NSCLC patients with MPE with or without driver oncogene mutations.
The limitations of this study were as follows: the present investigation included a small number of pleural effusion samples; hence, additional studies involving a larger number of cases are required to support our results. Moreover, detailed cellular analysis including regulatory T cells is desirable in this in vitro assay. Further analysis is also required to reveal the value of our assay in treatment with ICI.
Conclusions
This study attempted to predict treatment response and prognosis based on the analysis of pleural fluids obtained from patients. The results revealed several useful factors. We also established a co-culture bioassay to evaluate IFN-γ release following the administration of an ICI, reflecting the immune environment of the pleural cavity under this treatment. This in vitro bioassay of IFN-γ release may help identify responders prior to ICI treatment.
Acknowledgments
We thank Hiroko Hayakawa, Satomi Fujiwara, and Takako Ogura for their excellent technical assistance. BD LSRFortessa (BD Biosciences), a flow cytometer used in this study, was subsidized by JKA through its promotion funds from KEIRIN RACE.
Funding: None.
Footnote
Reporting Checklist: The authors have completed the TRIPOD reporting checklist. Available at https://tlcr.amegroups.com/article/view/10.21037/tlcr-24-559/rc
Data Sharing Statement: Available at https://tlcr.amegroups.com/article/view/10.21037/tlcr-24-559/dss
Peer Review File: Available at https://tlcr.amegroups.com/article/view/10.21037/tlcr-24-559/prf
Conflicts of Interest: All authors have completed the ICMJE uniform disclosure form (available at https://tlcr.amegroups.com/article/view/10.21037/tlcr-24-559/coif). M.M. received lecture fees from Ono Pharma and Bristol-Myers Squibb. The other authors have no conflicts of interest to declare.
Ethical Statement: The authors are accountable for all aspects of the work in ensuring that questions related to the accuracy or integrity of any part of the work are appropriately investigated and resolved. This study was approved by the Ethics Committee of Human Research at Jichi Medical University (No. A16-132). The study was conducted in accordance with the Declaration of Helsinki (as revised in 2013). Individual consent for this retrospective analysis was waived.
Open Access Statement: This is an Open Access article distributed in accordance with the Creative Commons Attribution-NonCommercial-NoDerivs 4.0 International License (CC BY-NC-ND 4.0), which permits the non-commercial replication and distribution of the article with the strict proviso that no changes or edits are made and the original work is properly cited (including links to both the formal publication through the relevant DOI and the license). See: https://creativecommons.org/licenses/by-nc-nd/4.0/.
References
- Ishida Y, Agata Y, Shibahara K, et al. Induced expression of PD-1, a novel member of the immunoglobulin gene superfamily, upon programmed cell death. EMBO J 1992;11:3887-95. [Crossref] [PubMed]
- Alegre ML, Frauwirth KA, Thompson CB. T-cell regulation by CD28 and CTLA-4. Nat Rev Immunol 2001;1:220-8. [Crossref] [PubMed]
- Pardoll DM. The blockade of immune checkpoints in cancer immunotherapy. Nat Rev Cancer 2012;12:252-64. [Crossref] [PubMed]
- Brahmer J, Reckamp KL, Baas P, et al. Nivolumab versus Docetaxel in Advanced Squamous-Cell Non-Small-Cell Lung Cancer. N Engl J Med 2015;373:123-35. [Crossref] [PubMed]
- Reck M, Rodríguez-Abreu D, Robinson AG, et al. Pembrolizumab versus Chemotherapy for PD-L1-Positive Non-Small-Cell Lung Cancer. N Engl J Med 2016;375:1823-33. [Crossref] [PubMed]
- Morgensztern D, Waqar S, Subramanian J, et al. Prognostic impact of malignant pleural effusion at presentation in patients with metastatic non-small-cell lung cancer. J Thorac Oncol 2012;7:1485-9. [Crossref] [PubMed]
- Verma A, Phua CK, Sim WY, et al. Pleural LDH as a prognostic marker in adenocarcinoma lung with malignant pleural effusion. Medicine (Baltimore) 2016;95:e3996. [Crossref] [PubMed]
- Kasapoglu US, Arınç S, Gungor S, et al. Prognostic factors affecting survival in non-small cell lung carcinoma patients with malignant pleural effusions. Clin Respir J 2016;10:791-9. [Crossref] [PubMed]
- Lee YS, Nam HS, Lim JH, et al. Prognostic impact of a new score using neutrophil-to-lymphocyte ratios in the serum and malignant pleural effusion in lung cancer patients. BMC Cancer 2017;17:557. [Crossref] [PubMed]
- Takahashi K, Saito S, Kamamura Y, et al. Prognostic value of CD4+ lymphocytes in pleural cavity of patients with non-small cell lung cancer. Thorax 2001;56:639-42. [Crossref] [PubMed]
- Zou Y, Xu L, Tang Q, et al. Cytology cell blocks from malignant pleural effusion are good candidates for PD-L1 detection in advanced NSCLC compared with matched histology samples. BMC Cancer 2020;20:344. [Crossref] [PubMed]
- Grosu HB, Arriola A, Stewart J, et al. PD-L1 detection in histology specimens and matched pleural fluid cell blocks of patients with NSCLC. Respirology 2019;24:1198-203. [Crossref] [PubMed]
- Hayashi H, Chamoto K, Hatae R, et al. Soluble immune checkpoint factors reflect exhaustion of antitumor immunity and response to PD-1 blockade. J Clin Invest 2024;134:e168318. [Crossref] [PubMed]
- Gainor JF, Shaw AT, Sequist LV, et al. EGFR Mutations and ALK Rearrangements Are Associated with Low Response Rates to PD-1 Pathway Blockade in Non-Small Cell Lung Cancer: A Retrospective Analysis. Clin Cancer Res 2016;22:4585-93. [Crossref] [PubMed]
- Sato M, Watanabe S, Tanaka H, et al. Retrospective analysis of antitumor effects and biomarkers for nivolumab in NSCLC patients with EGFR mutations. PLoS One 2019;14:e0215292. [Crossref] [PubMed]
- Sun L, Xu G, Liao W, et al. Clinicopathologic and prognostic significance of regulatory T cells in patients with hepatocellular carcinoma: a meta-analysis. Oncotarget 2017;8:39658-72. [Crossref] [PubMed]
- Togashi Y, Shitara K, Nishikawa H. Regulatory T cells in cancer immunosuppression - implications for anticancer therapy. Nat Rev Clin Oncol 2019;16:356-71. [Crossref] [PubMed]
- Saito H, Fukuhara T, Furuya N, et al. Erlotinib plus bevacizumab versus erlotinib alone in patients with EGFR-positive advanced non-squamous non-small-cell lung cancer (NEJ026): interim analysis of an open-label, randomised, multicentre, phase 3 trial. Lancet Oncol 2019;20:625-35. [Crossref] [PubMed]
- Hosomi Y, Seto T, Nishio M, et al. Erlotinib with or without bevacizumab as a first-line therapy for patients with advanced nonsquamous epidermal growth factor receptor-positive non-small cell lung cancer: Exploratory subgroup analyses from the phase II JO25567 study. Thorac Cancer 2022;13:2192-200. [Crossref] [PubMed]
- Liu PL, Tsai JR, Hwang JJ, et al. High-mobility group box 1-mediated matrix metalloproteinase-9 expression in non-small cell lung cancer contributes to tumor cell invasiveness. Am J Respir Cell Mol Biol 2010;43:530-8. [Crossref] [PubMed]
- Ellerman JE, Brown CK, de Vera M, et al. Masquerader: high mobility group box-1 and cancer. Clin Cancer Res 2007;13:2836-48. [Crossref] [PubMed]
- Wu CG, Chiovaro F, Curioni-Fontecedro A, et al. In vitro cell culture of patient derived malignant pleural and peritoneal effusions for personalised drug screening. J Transl Med 2020;18:163. [Crossref] [PubMed]
- Mazzocchi A, Devarasetty M, Herberg S, et al. Pleural Effusion Aspirate for use in 3D Lung Cancer Modeling and Chemotherapy Screening. ACS Biomater Sci Eng 2019;5:1937-43. [Crossref] [PubMed]
- Tau GZ, Cowan SN, Weisburg J, et al. Regulation of IFN-gamma signaling is essential for the cytotoxic activity of CD8(+) T cells. J Immunol 2001;167:5574-82. [Crossref] [PubMed]