The current landscape and prospects of antibody-drug conjugates for lung cancer brain metastases: a narrative review
Introduction
Lung cancer is the most common cancer worldwide, both in terms of incidence and mortality, posing a serious threat to human health (1). In 2022, there were an estimated 870,982 new cases of lung cancer and 766,898 deaths due to lung cancer in China, while in the United States, there were 238,032 new cases and 144,913 deaths (2). With the development of medical diagnostics and therapeutics, the survival period of patients has been prolonged, and the occurrence and diagnosis rate of lung cancer brain metastases (BMs) have also gradually increased. Distant metastasis, especially BM, is the main cause of death for patients with lung cancer. As the most common cancer to progress to BMs, lung cancer presents with intracranial involvement in approximately 20% of patients at the time of diagnosis (3). An estimated 20% to 65% of patients with lung cancer will develop BMs during the course of disease (4). A long-term follow-up study based on the Surveillance, Epidemiology, and End Results (SEER) database showed that in patients with nonmetastatic non-small cell lung cancer (NSCLC), the BM incidence of lung adenocarcinoma, squamous cell carcinoma, large-cell carcinoma, and small-cell lung cancer (SCLC) is 11%, 6%, 12%, and 18% respectively (5). Moreover, it has been found that 40–50% of patients with SCLC develop BM during the disease process, with only 60–80% of these patients surviving more than 2 years (5,6).
Lung cancer patients with BM have a rather dismal prognosis, with a median survival of merely 1 to 2 months in the absence of treatment. The current therapeutic approach often entails a synergistic combination of local treatments and systemic therapies including chemotherapy, targeted therapy, immunotherapy, and antiangiogenic therapy. Local treatments include whole-brain radiotherapy, stereotactic radiosurgery, and surgical resection. The management of brain cancers is predominantly constrained by the delivery barriers posed by the blood-brain barrier (BBB). The current treatment paradigm for BM is dependent upon the patient’s overall health status, the number and location of brain lesions, and the extent of extracranial cancer activity. The treatment of this condition necessitates the involvement of multiple related disciplines and the implementation of diverse therapeutic approaches. To foster a multidisciplinary approach in the treatment of lung cancer patients with BM, the collaboration of multidisciplinary experts is required to develop treatment plans that will enhance the quality of life of patients and extend their survival time (4,6). Despite the advent of diverse therapeutic modalities, the median overall survival (OS) for patients with BMs remains at 6 months or less, is under 4 months for those aged 65 years and above, and is as high as 16 months in patients with epidermal growth factor receptor (EGFR) mutations, which underscores an urgent need for innovative treatments (7,8).
In 1913, Paul Ehrlich introduced the concept of the “magic bullet”, now known as antibody-drug conjugates (ADCs) (9). With the emergence of new ADCs, most notably trastuzumab deruxtecan (T-DXd), in cancer treatment, exploration of ADCs in patients with lung cancer BMs has also achieved some encouraging results. In this review, we briefly summarize existing systemic treatments for lung cancer BMs, mainly focusing on the latest advances of ADCs for BMs. Furthermore, we discuss the factors that may influence the differential effects of various ADCs and examine the feasibility of combining ADCs with other treatment modalities in patients with lung cancer BMs. We present this article in accordance with the Narrative Review reporting checklist (available at https://tlcr.amegroups.com/article/view/10.21037/tlcr-24-964/rc).
Methods
This review consists of relevant data on ADCs in lung cancer BMs retrieved on the PubMed database as well as American Association for Cancer Research (AACR), American Society of Clinical Oncology (ASCO), European Society for Medical Oncology (ESMO), and World Conference on Lung Cancer (WCLC) meetings. The keyword search terms included lung cancer, brain metastases and antibody-drug conjugate, among others. In addition, the literature reviewed was required to be published in the past 10 years and be in English. The details of the literature search process are listed in Table 1.
Table 1
Items | Specification |
---|---|
Date of search | July 2024; October 2024 |
Databases and other sources searched | PubMed, AACR, ASCO, ESMO, WCLC meetings |
Search terms used | Lung cancer, brain metastases, antibody-drug conjugate |
Timeframe | From 2015 to 2024 |
Inclusion criteria | English-language literature |
Selection process | Studies were initially screened independently by H.Z. and Y.Z. and reviewed by X.D.; consensus was reached via discussion among all authors |
AACR, American Association for Cancer Research; ASCO, American Society of Clinical Oncology; ESMO, European Society for Medical Oncology; WCLC, World Conference on Lung Cancer.
Current treatment strategies for lung cancer BMs
Chemotherapy remains a crucial and essential component of systemic treatment for patients with lung cancer. However, the charged nature of chemotherapeutic agents and their propensity to bind with albumin make it challenging to cross the BBB and exert antitumor effects on intracranial metastatic lesions. Despite evidence suggesting that the integrity of the BBB can be compromised by primary or metastatic brain cancers, the therapeutic efficacy of various chemotherapy combinations has been less than optimal (10,11). The poor efficacy of chemotherapeutic drugs may be due to their limited intracranial antitumor activities and extrusion from the blood tumor barrier (11). Clinical trials investigating the potential for synergy between different chemotherapy drugs, such as cisplatin with pemetrexed, cisplatin with vinorelbine, paclitaxel with cisplatin, pemetrexed with cisplatin, irinotecan with carboplatin, and etoposide with cisplatin, and etoposide with temozolomide, have yielded unsatisfactory results. This underscores the need for alternative therapeutic approaches that can effectively overcome the obstacles posed by the BBB and enhance the treatment of BM in patients with lung cancer (12).
Molecular targeted therapy in mutations such as EGFR, ALK, and ROS1 has significantly changed the treatment paradigm for driver gene-positive NSCLC BMs due to the high BBB permeability and intracranial activity. The intracranial overall response rate (ORR-ic) of third-generation EGFR tyrosine kinase inhibitors (TKIs) has reached 82.8–91% (13-15) while in ALK, lorlatinib, a third-generation TKI for patients with advanced anaplastic lymphoma kinase (ALK)-positive NSCLC, was found to yield an ORR-ic of 82% and an intracranial complete response (CR) rate of 71% (16). Despite the promising efficacy of molecularly targeted therapy in patients with NSCLC with targetable driver mutations, these individuals remain highly susceptible to recurrence of BMs and even meningeal metastases. Research indicates that patients with NSCLC and EGFR mutations have an increased risk of developing BMs, with cumulative incidences at 1, 3 and 5 years of 46%, 64%, and 71%, respectively with similar outcomes in ALK and ROS1 mutations (17,18). Although the combination of targeted therapy with local radiotherapy can alleviate patient symptoms and prolong the duration of targeted drug effectiveness, the absence of effective subsequent treatment options after intracranial recurrence presents a significant clinical challenge.
The use of immune checkpoint blockade (ICB) therapy has demonstrated efficacy in the treatment of BMs from lung cancer. In the first-line treatment of patients with NSCLC BMs and PD-L1 expression ≥1%, the ORR-ic of single-agent therapy with pembrolizumab was reported to be 29.7% (19). A subgroup analysis of patients with BMs in the KEYNOTE-189 study showed that the combination of pembrolizumab with pemetrexed-platinum significantly prolonged the OS of patients with BM (19.2 months) compared with placebo plus pemetrexed-platinum (7.5 months) [hazard ratio (HR) =0.41, 95% confidence interval (CI): 0.24–0.67] (20). However, the majority of research on immunotherapy for lung cancer BMs is based on retrospective analyses with small sample sizes. Furthermore, the mechanism by which ICB drugs penetrate the BBB remains unclear. Nivolumab plus ipilimumab is a promising therapeutic option in patients with BMs (21). Further research is necessary to provide a more solid foundation for this treatment approach.
Antiangiogenic drugs, including bevacizumab and ramucirumab, have the potential to be efficacious in the treatment of lung cancer BMs. By targeting vascular endothelial growth factor (VEGF) or its receptor, antiangiogenic drugs inhibit endothelial cell proliferation and new blood vessel formation, leading to vascular normalization of BMs. This process is often considered to exert its effects without crossing the BBB (22). Multiple studies have found that the combination of chemotherapy with antiangiogenic drugs is an effective and safe and has the potential to prevent the occurrence of new BMs (23,24). For instance, the CTONG 1509 study demonstrated that the therapeutic regimen combining bevacizumab with erlotinib not only enhanced patient progression-free survival (PFS) but also reduced the risk of BM, offering a superior alternative to single-agent therapies while providing tolerable adverse effects (25). Most recently, ivonescimab, a bispecific antibody with cooperative binding to PD-1 and VEGF, demonstrated a 34% intracranial response rate with a median PFS of 19.3 months (26). Consequently, the investigation of the potential for antiangiogenic agents to act in conjunction with other therapeutic strategies has emerged as a pivotal direction in modern research.
Local therapy, including radiotherapy and surgery, is delivered for purposes of local control and the alleviation of intracranial symptoms. However, it can also have a systemic effect on remote tumor deposits and improve median survival time, making it an important treatment option for BMs. Nevertheless, the application of this treatment approach is constrained by several factors, including the number and location of intracranial metastases, the status of extracranial metastases, the overall condition of the patient, and the tolerance of organs at risk to the reirradiation dose. Although the majority of patients are eligible to receive initial radiotherapy or surgical intervention in combination with systemic anticancer therapy, a minority of these patients undergo reirradiation or surgical procedures following the recurrence of intracranial lesions. Currently, the selection of systemic anticancer treatments for lung cancer BMs remains limited, underscoring the pressing need for innovative therapeutic breakthroughs.
Design of different ADCs
Generally, ADCs consist of three integral components: monoclonal antibodies, payload, and linkers. Monoclonal antibodies provide targeting precision by specifically binding to antigens present on the surface of the target cells. Subsequently, ADCs are internalized into cancer cells via clathrin-mediated endocytosis. Once inside the cells, ADCs are degraded by lysosomal enzymes, the low pH of the acidic tumor microenvironment, or the high level of glutathione in cytoplasm and release their cytotoxic payload, which effectively eliminates the targeted cancer cells. This elegant design of ADCs combines the precision of targeted therapy with the potency of chemotherapy, representing an optimal synergy in drug design (27). However, due to limitations in the process and technology employed, the first ADC drug, gemtuzumab ozogamicin, was not approved for clinical use until the year 2000. Its clinical impact was underwhelming due to factors such as the insufficient toxicity of the payload, instability of the linkers, and immunoreactivity triggered by murine monoclonal antibodies, leading to suboptimal efficacy and intolerable toxicity levels.
Subsequent iterations in the design of ADCs have improved the targeting of tumor cells by refining the selection of monoclonal antibodies and mitigating immunogenicity. Additionally, the discovery of more potent small-molecule agents has increased the cytotoxicity of the payload drugs. A prime example is trastuzumab emtansine (T-DM1), an ADC that pairs trastuzumab, a humanized monoclonal antibody targeting human epidermal growth factor receptor 2 (HER2), with the highly toxic microtubule inhibitor maytansine, linked together through an uncleavable thioether linker. This advancement represents a significant stride in the evolution of ADC therapies. T-DM1 has demonstrated effective anticancer activity across a spectrum of solid tumors, notably breast cancer, with manageable adverse reactions. Nonetheless, the design of T-DM1 is associated with certain constraints. The reliance on traditional chemical conjugation techniques can result in variability in the drug:antibody ratio (DAR), complicating the standardization of quality control measures. It may be that the quantity of payload drugs delivered by ADCs that successfully breach the tumor cell barrier is a direct determinant of the anticancer efficacy. T-DM1 has a DAR of 3–4, which may limit its potential for tumor eradication. Furthermore, suboptimal stability due to technological limitations can lead to the partial disassembly of T-DM1 in the bloodstream, potentially releasing minute quantities of highly toxic maytansine, precipitating severe adverse effects.
The latest generation of ADCs employs fully humanized antibodies or engineered monoclonal antibodies to significantly diminish immunogenicity. These advancements are accompanied by refined conjugation methodologies that yield ADCs with a uniform DAR, enhancing the precision of therapeutic delivery. Additionally, the investigation of novel payload drugs within this novel class of ADCs has resulted in a reduced off-target toxicity profile and improved pharmacokinetic efficiency, representing a promising evolution in targeted cancer therapies.
T-DXd is an advanced ADC drug that pairs trastuzumab with the topoisomerase I inhibitor deruxtecan through a cleavable linker. The anti-HER2 antibody belongs to the IgG1 subtype, which is known for its extended half-life, stable configuration, and potent induction of antibody-dependent cell-mediated cytotoxicity and complement-dependent cytotoxicity. The payload, the optimized topoisomerase I inhibitor DXd, is used to minimize the risk of cross-resistance with frequently employed chemotherapeutic agents. DXd is characterized by its moderate toxicity and a brief systemic half-life of merely 1.37 hours when unbound, facilitating the management of systemic toxicity after payload release and thereby expanding the therapeutic window (28). P-glycoprotein-mediated efflux of cytotoxic drugs represents a significant mechanism of drug resistance. However, studies indicate that DXd is efficacious against P-glycoprotein-expressing cells and that T-DXd is a poor substrate for P-glycoprotein, fundamentally reducing the potential for T-DXd resistance (28-30). It is frequently observed that a higher DAR in ADCs correlates with enhanced anticancer activity but that this also accelerates the drug’s systemic clearance (31). Achieving a balance between a high and uniform DAR with favorable pharmacokinetics is thus a critical challenge in ADC design. T-DXd addresses this by employing cysteine as a conjugation site and leveraging refined conjugation techniques to attain a DAR of 7–8 with greater uniformity, facilitating quality control and surpassing the efficacy of other ADCs by delivering more potent payloads to tumor cells (28). T-DXd incorporates an enzymatically cleavable maleimide glycyn-glycyn-phenylalanyn-glycyn (GGFG) peptide linker and introduces a novel self-immolative linker system using an aminomethylene (AM) moiety, which, compared to traditional p-aminobenzyl spacers, effectively diminishes hydrophobicity and drug aggregation. This enhancement aids in maintaining stability within the bloodstream, reduces the likelihood of premature dissociation, and preserves superior pharmacokinetic properties (32). A series of studies have shown that after 21 days, the levels of DXd and trastuzumab levels remain consistently low. This high stability is of crucial importance for controlling the drug’s systemic toxicity, enhancing its therapeutic window and index, and enabling T-DXd to operate effectively at higher dosages (32). Furthermore, the lipophilicity of the payload enhances membrane permeability, and the cleavable linker’s deaminated design generates a robust bystander effect, enabling the destruction of tumor cells that are negative for the target antigen (33). Patritumab deruxtecan (HER3-DXd), which will be discussed later, shares a similar drug design to that of T-DXd. Both have demonstrated promising anticancer activity and safety profiles in clinical trials for patients with lung cancer with BM (34), which is attributable to their structural superiority.
Preclinical research of ADCs in BM
The natural BBB typically restricts the entry of large molecules, such as antibodies, into the brain, resulting in brain antibody concentrations that are only about 0.01% of those found in the bloodstream (35). However, the presence of primary or secondary brain tumors can significantly disrupt the BBB’s integrity (10,36), leading to the formation of the blood-tumor barrier (BTB) (10). The BTB, while compromised compared to the BBB, is characterized by high heterogeneity and variable permeability, which continues to impede drug entry into the brain. A study has observed an increased uptake of radiolabeled anti-HER2 monoclonal antibodies, such as trastuzumab, in BMs of patients with HER2-positive breast cancer (36). This suggests that large molecules, including ADCs, may have enhanced access to BMs through the compromised BBB. However, despite this increased permeability, the BTB still presents challenges for effective drug delivery due to its irregular and heterogeneous nature, which can lead to uneven distribution of therapeutics within the brain (10). Additionally, other mechanisms of drug resistance, such as increased efflux transporter activity and the unique tumor microenvironment, can further limit the efficacy of ADCs in treating BMs (11). Understanding these complexities is crucial for developing strategies to improve the delivery and efficacy of ADCs in the treatment of BMs.
ADCs may infiltrate the central nervous system via endothelial cell phagocytosis. A comparative analysis of the inhibitory effects between trastuzumab and T-DM1 using a mouse model of breast cancer BM and organotypic brain slice cultures of tumor cells demonstrated that T-DM1 markedly surpassed trastuzumab in inducing tumor cell apoptosis, conferring substantial survival advantages (112 vs. 28 days; HR =6.2). Notably, no disparities were observed between trastuzumab and T-DM1 regarding drug distribution or modulation of HER2 signaling, which may signify a promising avenue for overcoming trastuzumab-resistant breast cancer BMs (37).
A study using a mouse patient-derived xenograft (PDX) model for HER2-positive breast cancer BM demonstrated that administration of T-DXd significantly suppressed tumor volume and markedly extended survival duration. Although there was no discernible change in the proliferation marker Ki-67 within T-DXd-treated cancers when compared to control tumors, there was a pronounced increase in the apoptotic marker, caspase 3. This indicates that T-DXd potentially eradicates intracranial cancer cells and extends the survival time in mice via the induction of apoptosis. Analogous anticancer efficacy was noted in PDX models with HER2-low expression [immunohistochemistry (IHC) 2+]. Further investigation in a T-DM1-resistant mouse PDX model revealed that T-DXd treatment significantly extended survival when compared to both the T-DM1-continued and control conditions (38). These in vivo findings indicate that T-DXd may exert favorable therapeutic effects in patients with HER2-positive or HER2-low malignant BMs and may also be efficacious in those who are refractory to T-DM1, thus providing a scientific rationale for the further clinical investigation of T-DXd in BMs.
Datopotamab deruxtecan (Dato-DXd) is an ADC composed of a humanized trophoblast cell-surface antigen 2 (TROP-2) antibody and DXd, which are connected by a cleavable linker. A comparative analysis (39) revealed that Dato-DXd, when administered intravenously, exhibited substantially deeper penetration into brain tumor tissues than did a nontargeting control ADC, which predominantly accumulated at the tumor periphery in the intracranial tumor models. Consequently, the Dato-DXd treatment group registered significantly superior tumor growth inhibition rates and extended survival times in comparison to the nontargeting ADC group. These findings indicate that Dato-DXd is capable of traversing the BBB and owing to the targeted guidance effect of TROP-2, is able to navigate the tumor’s physical barriers more effectively, thus achieving a heightened tumoricidal impact. Moreover, this suggests that Dato-DXd can penetrate the BBB and, due to the targeted guiding effect of TROP-2, is able to more easily penetrate the physical barriers of the tumor into the tumor microenvironment, exerting a more effective tumor-killing effect (39). Thus, future strategies will focus on developing ADCs that will be able to have easier penetration through the BBB. Another strategy that may enhance intracranial response in BM patients can be to target the immune tumor microenvironment. For example, in glioblastoma, a combination of EphA3 ADC with radioimmunotherapy was able to show effective delivery through the BBB (40).
Clinical research of ADCs in treating BM
ADCs represent one of the most rapidly evolving areas in the field of anticancer drug development in recent years. Numerous clinical trials have demonstrated that ADCs, despite being large molecules, can exert significant therapeutic effects on brain tumors (Table 2). T-DM1 is the first ADC to receive approval for the treatment of breast cancer. Although T-DM1 may share similar challenges with trastuzumab in penetrating the BBB, it has still shown notable intracranial activity. The EMILIA study, which included 95 patients with breast cancer and baseline BMs, reported a median OS of 26.8 months for the T-DM1 group, representing a significant improvement to the 12.9 months observed in the lapatinib-plus-capecitabine group. However, the median PFS (mPFS) for the two groups did not significantly differ (5.9 vs. 5.7 months; P>0.99) (41). In the KAMILLA study, 398 patients with breast cancer and baseline BMs were treated with T-DM1, achieving an mPFS of 5.5 months (95% CI: 5.3–5.6) and a median OS of 18.9 months (95% CI: 17.1–21.3). Among these patients, 126 had measurable intracranial metastatic lesions, and an ORR of 21.4% was observed (95% CI: 14.6–29.6%) (42). Although T-DM1’s application in patients with breast cancer BM did not yield statistically significant improvements in PFS over chemotherapy, the clinical data confirms that ADCs have the potential to exert anticancer effects in patients with malignant BMs. This provides reasons for optimism and the basic rationale for the continued development of novel ADCs for BMs.
Table 2
Drug name | Type of study (trial name) | Cancer type related | BMs type related | No. of patients with BMs | Outcome data | References |
---|---|---|---|---|---|---|
T-DM1 (HER2) | Exploratory post hoc analysis of phase III trial (EMILIA) | Breast cancer | Asymptomatic BMs previously treated with radiotherapy | T-DM1: 45 | T-DM1 vs. chemotherapy: | (41) |
chemotherapy: 50 | mOS: 26.8 vs. 12.9 months (HR =0.38, P=0.008) | |||||
mPFS: 5.9 vs. 5.7 months per BICR (HR =1.00, P=1.000) | ||||||
Exploratory post hoc analysis of phase III trial (KAMILLA) | Breast cancer | Asymptomatic, untreated or controlled BMs | BMs: 398; measurable BMs: 126 | Measurable BMs: | (42) | |
ORR: 21.4%, CBR 42.9%; | ||||||
Patients with BMs vs. without BMs: | ||||||
mPFS: 5.5 vs. 7.7 months | ||||||
mOS: 18.9 vs. 30.0 months | ||||||
T-DXd (HER2) | Exploratory post hoc analysis of phase II trial (DESTINY-Breast01) | Breast cancer | Untreated, asymptomatic, or controlled BMs | 24 (5 untreated) | ORR-ic: 47.1% | (43) |
BM subgroup vs. those without BMs | ||||||
cORR: 58.3% for 61.3% | ||||||
DoR: 16.9 vs. 14.8 months | ||||||
mPFS: 18.1 vs. 16.4 months | ||||||
Exploratory post hoc analysis of phase III trial (DESTINY-Breast03) | Breast cancer | Untreated, asymptomatic BMs not requiring treatment | 82 | T-DXd vs. T-DM1 | (44) | |
T-DXd: 43 | ORR: 67.4% vs. 20.5% | |||||
T-DM1: 39 | mPFS: 15.0 vs. 3.0 months, HR =0.25 | |||||
Single-arm phase II trial (TUXEDO-1) | Breast cancer | Active BMs (newly diagnosed BMs or progressing after previous local therapy) | 15 (ITT) | ITT: ORR-ic: 73.3% (RANO criteria) | (45,46) | |
14 (per protocol) | Per protocol population (RANO criteria) | |||||
ORR-ic: 78.6% | ||||||
CBR: 92.9% | ||||||
mPFS: 21.0 months | ||||||
mOS months: not reached (95% CI: 22.2–NR) | ||||||
Single-arm phase II trial (DEBBRAH) | Breast cancer | Asymptomatic untreated; stable or progressing; BMs after local treatment for analysed population | 21: cohort 1: 4 patients BM absent and 4 BM stable but non-measurable after local treatment; cohort 2: 4 patients asymptomatic untreated; cohort 3: 9 patients BM progressing after local treatment | The 16-weeks PFS rate for cohort 1 was 87.5% | (47) | |
ORR-ic for cohort 2: 50.0% (RANO criteria) | ||||||
ORR-ic for cohort 3: 44.4% (RANO criteria) | ||||||
ORR-ic in patients with active BMs (cohorts 2 and 3) was 46.2% (RANO criteria) | ||||||
The overall ORR: | ||||||
Cohort 1: 66.7% | ||||||
Cohort 2: 80.0% | ||||||
Cohort 3: 50.0% | ||||||
Retrospective study | Breast cancer | Active BMs (untreated or progressive after local treatment) and stable BMs | 18 (ITT); 1 (EAS) | ORR-ic: 73% | (38) | |
3: untreated | 12m CNS PFS rate 74.7% | |||||
7: progressive after local treatment | 12m overall PFS rate 57.8% | |||||
7: stable after local treatment | Active BMs: ORR-ic: 70% (7/10) | |||||
Phase II trial (DESTINY-Lung01) | HER2mut NSCLC | Asymptomatic BMs who were not receiving ongoing glucocorticoid or anticonvulsant therapy | 33 | ORR: 54.5% mPFS: 7.1 months; mOS: 13.8 months | (48) | |
Exploratory Pooled analysis of DESTINY-Lung01 & DESTINY-Lung02 | HER2mut NSCLC | Asymptomatic BMs who were not receiving ongoing glucocorticoid or anticonvulsant therapy | T-DXd 5.4 mg/kg: 32 | T-DXd 5.4 mg/kg with BM: systemic cORR: 46.9%, DCR: 90.6%, mDoR: 4.6 months, mPFS: 7.1 months, mOS: 13.6 months | (49) | |
T-DXd 6.4 mg/kg: 54 | T-DXd 6.4 mg/kg with BM: systemic cORR: 50.0%, DCR: 92.6%, mDoR: 7.2 months, mPFS: 7.1 months, mOS: 13.8 months | |||||
Measurable BM at baseline: | Measurable BM at baseline: | |||||
T-DXd 5.4 mg/kg: 14 | T-DXd 5.4 mg/kg: cORR-ic: 50.0%, DCR-ic: 92.9%, mDoR-ic: 9.5 months | |||||
T-DXd 6.4 mg/kg: 30 | T-DXd 6.4 mg/kg: cORR-ic: 30.0%, DCR-ic: 73.3%, mDoR-ic: 4.4 months | |||||
Patritumab deruxtecan (HER3) | Phase II trial (HERTHENA-Lung01) |
EGFR-mut NSCLC | Stable or asymptomatic pre-treated BMs | 115 had a history of BMs, 30 had radiologic evidence of BMs at baseline by BICR |
Patients with a history of BMs vs. without BMs: | (34) |
cORR: 28.7% vs. 30.9% | ||||||
DCR: 70.4% vs. 77.3% | ||||||
mDoR: 5.5 vs. 6.9 months; mPFS: 4.3 vs. 6.2 months | ||||||
mOS: 11.6 vs. 12.9 months | ||||||
BMs had radiologic evidence of BMs (BICR): | ||||||
ORR-ic: 33.3% | ||||||
mDoR: 8.4 months | ||||||
Dato-DXd (TROP2) | Exploratory post hoc analysis of phase II TROPION-Lung05 trial | NSCLC | Stable pre-treated BMs | 53; measurable BMs: 18 | mPFS: 6.9 months | (50) |
Measurable BMs: | ||||||
ORR-ic: 22%, | ||||||
DCR-ic: 72% | ||||||
CBR-ic: 44% | ||||||
BL-B01D1 (EGFR/HER3) | Phase I clinical trial | NSCLC | No BMs; treated but stable BMs; untreated and asymptomatic BMs | Treated/no BMs: 75 | Untreated BMs: | (51) |
Untreated BMs: 27 | ORR: 48.1%, cORR: 33.3%, mDoR: 4.2 months | |||||
DCR: 88.9%, mPFS: 4.1 months | ||||||
Treated/no BMs: | ||||||
ORR: 52.0%, cORR: 41.3%, mDoR: 12.3 months | ||||||
DCR: 86.7%, mPFS: 6.8 months |
ADCs, antibody-drug conjugates; BMs, brain metastases; T-DM1, trastuzumab emtansine; HER2, human epidermal growth factor receptor 2; mOS, median overall survival; HR, hazard ratio; mPFS, median progression-free survival; BICR, blinded independent central review; ORR, objective response rate; CBR, clinical benefit rate; ORR-ic, ORR intracranial; cORR, confirmed ORR; DoR, duration of response; T-DXd, trastuzumab deruxtecan; RANO, response assessment in neuro-oncology; ITT, intention-to-treat; CI, confidence interval; NR, not reported; EAS, efficacy analysis set; CNS, central nervous system; NSCLC, non-small cell lung cancer; DCR, disease control rate.
T-DXd, with its elevated DAR and bystander effect, surpasses T-DM1 in augmenting the potency of its anticancer action. Meta-analysis has demonstrated the intracranial efficacy of T-DXd in patients with both stable and active BMs (52). In the DESTINY-Breast01 trial, T-DXd demonstrated an ORR of 58.3%, an mPFS of 18.1 months, and an ORR-ic of 47.1% among 24 patients with stable BMs from breast cancer, supporting T-DXd’s substantial potential in this context (43). The DESTINY-Breast03 trial further substantiated T-DXd’s efficacy. In the trial, for 82 patients with stable breast cancer BMs treated with T-DXd, the ORR was 67.4% while the mPFS was 15.0 months, both of which were superior to the 20.5% and 3.0 months (HR =0.25) , respectively, yielded by T-DM1 (44), confirming T-DXd’s marked benefit in patients with advanced-stage breast cancer and stable BMs. The TUXEDO-1 study, which included 15 patients with HER2-positive breast cancer and active BMs, demonstrated comparable outcomes to those observed in the DESTINY-Breast03 trial. The ORR-ic was 78.6%, and the mPFS was 21.0 months in the per protocol population (n=14 patients)(45,46). Additionally, the DEBBRAH study, which enrolled 21 patients with breast cancer BMs, 23 of whom had active BMs, reported an ORR-ic of 46.2% for those with active disease (47). Kabraji et al. conducted a retrospective analysis which included 10 cases of active BMs and 7 cases of stable intracranial lesions in patients with late-stage breast cancer and reported an overall ORR-ic of 73%, with an ORR-ic of 70% specifically for active BMs (38). Notably, among these patients, one with low HER2 expression achieved a partial response (PR) for intracranial tumors with a duration of response (DoR) of 3.2 months, highlighting T-DXd’s therapeutic versatility across the spectrum of HER2 expression levels. In the DESTINY-Breast12 study, T-DXd achieved a 12-month CNS-specific PFS of 58.9% (95% CI: 51.9–65.3%) and an ORR of 71.7% (95% CI: 64.2–79.3%) in breast cancer patients with HER2-positive BMs (53). The substantial intracranial antitumor activities of T-DXd highlights its unique capacity to overcome the BBB in controlling HER2-positive BMs. T-DXd has been recently approved for the treatment of HER2+ solid tumors (54). With this pan-tumor approval, it is important to explore the intracranial antitumor activities of T-DXd in HER2+ lung cancer patients with BMs. It is of note that HER2 expression may differ between primary and BMs, due to tumor heterogeneity and evolution, raising the prospect of treating HER2- lung cancer patients with BMs.
Numerous clinical trials are currently investigating the efficacy of ADCs in the treatment of specific cancer types, aiming to offer novel therapeutic options for patients. Notable examples include MRG003 (an EGFR-targeting ADC), CPO301 (another EGFR-targeting ADC), and NPX267 (an HHLA-2-targeting ADC), which are being evaluated in ongoing studies involving patients with EGFR-positive advanced non-small-cell lung cancer (NCT04838548), adult patients with advanced or metastatic solid tumors (NCT05948865), and individuals with solid tumors expressing HHLA-2 (NCT05958199), respectively.
ADCs are also demonstrating significant clinical promise in the context of lung cancer BMs. ADCs that target a variety of receptors, including HER2, HER3, human trophoblast cell-surface antigen 2 (TROP2), and cellular-mesenchymal epithelial transition factor (c-MET), have exhibited initial efficacy in clinical studies for lung cancer. It is worth noting that positive therapeutic effects have been observed in patients with lung cancer and BMs, indicating the potential broadening of therapeutic horizons for this patient population.
The DESTINY-Lung01 study examined the viability of T-DXd in patients with HER2-mutated NSCLC who also had BMs. The trial included 33 asymptomatic patients with BMs, achieving an ORR of 54.5%, which was comparable to the 55.2% ORR observed in patients without BMs. The mPFS was 7.1 months for those with BMs and was 8.2 months for those without, while the OS was 13.8 and 17.8 months, respectively (48). These data provide evidence that T-DXd is effective in treating intracranial tumors in patients with HER2-mutated NSCLC. The DESTINY-Lung02 study demonstrated that T-DXd 5.4 and 6.4 mg/kg once every 3 weeks both elicited profound and sustained responses, which are consistent across various factors, including HER2 mutation type and amplification status, baseline central nervous system metastasis, and prior treatment history (55). A comprehensive analysis presented at the European Society for Medical Oncology (ESMO) Congress 2023, which pooled data from the DESTINY-Lung01 and DESTINY-Lung02 studies, evaluated the efficacy of T-DXd 5.4 and 6.4 mg/kg in patients with HER2-mutant NSCLC. The study comprised 32 patients in the 5.4 mg/kg cohort and 54 in the 6.4 mg/kg cohort. Both dosages exhibited favorable systemic therapeutic benefits for patients irrespective of whether they had BMs. In the 5.4 mg/kg group, the confirmed ORR (cORRs) for patients with and without BMs were 46.9% and 50.0%, respectively, while the disease control rates (DCRs) were 90.6% and 94.3%, respectively. The mPFS for patients with BMs in both dosage groups was identical at 7.1 months, and was 18.0 months (5.4 mg/kg group) and 11.9 months (6.4 mg/kg group) in patients without baseline BMs. Fourteen patients in the 5.4 mg/kg group and 30 patients in the 6.4 mg/kg group had measurable brain metastatic lesions at baseline. The intracranial cORR was 50% for the 5.4 mg/kg group and 30.0% for the 6.4 mg/kg group (95% CI: 14.7–49.4%). Three patients (21.4%) in the 5.4 mg/kg group achieved CR, while 4 (28.6%) in the 5.4 mg/kg group and 9 (30.0%) in the 6.4 mg/kg group achieved PR. Intracranial response rates were consistent across patients, regardless of their baseline BM treatment status (49). Based on the positive findings from the DESTINY-Lung01 and DESTINY-Lung02 studies, the US Food and Drug Administration approved T-DXd as a second-line treatment for HER2-mutated NSCLC. Mutations in HER2 occur in approximately 3% of nonsquamous NSCLCs and are associated with a higher incidence of BM compared to NSCLC without HER2 mutations (56,57). T-DXd is not only effective for HER2 mutated NSCLC patients but also exhibits promising antitumor activities in patients with NSCLC with HER2 expression. In the Destiny-Lung03 study, T-DXd may also have a good efficacy in patients with HER2-expressing NSCLC BMs (58), but more data are needed.
HER3-DXd, structurally similar to T-DXd, is an ADC that combines a humanized anti-HER3 antibody with the payload DXd through a tetrapeptide linker. The U31402-A-U102 study results indicated that, following response assessment in 52 patients who had previously undergone platinum-based chemotherapy, those with a history of BMs (n=25) exhibited an ORR of 32%, whereas patients without such a history (n=27) demonstrated an ORR of 41% (59). In the HERTHENA-Lung01 study, HER3-DXd demonstrated robust systemic activity in 115 patients with EGFR-mutated NSCLC and a history of BMs, showing a cORR of 28.7%, a DCR of 70.4%, and a median DoR (mDoR) of 5.5 months. These outcomes were comparable to those seen in patients without history of BMs, highlighting the drug’s systemic efficacy. Among the subgroup of 30 patients with EGFR-mutated NSCLC with untreated BMs, HER3-DXd achieved a cORR-ic of 33.3%, including 9 cases of CR and 1 case of PR, with a CNS mDoR of 8.4 months (34). These results highlight the potent anticancer activity HER3-DXd against BMs in EGFR-mutated NSCLC. Moreover, an ongoing clinical trial (NCT05865990) is investigating the potential of HER3-DXd in treating leptomeningeal metastases from breast cancer or NSCLC.
Dato-DXd is an ADC targeting TROP2 and is composed of a humanized anti-TROP2 IgG1 monoclonal antibody linked to a potent topoisomerase I inhibitor payload through a stable tetrapeptide cleavable linker. In the TROPION-Lung01 study, administration of the TROP-2 targeting ADC Dato-DXd resulted in an extended PFS compared to docetaxel, although OS remained unchanged in patients with previously treated NSCLC (60). The post hoc analysis results of the phase II TROPION-Lung05 trial of Dato-DXd in the treatment of NSCLC were presented at the American Society of Clinical Oncology (ASCO) Annual Meeting in 2024, with a particular focus on the patient population that progressed after receiving targeted therapy and platinum-based chemotherapy (with BM). The study included 137 patients, 53 of whom (39%) had baseline BMs, with 55% carrying EGFR mutations. The median intracranial lesion PFS for the 53 patients with baseline BMs was 6.9 months. Among the 18 patients with measurable intracranial lesions, the ORR was 22%, the DCR was 72%, and the clinical benefit rate (CBR) was 44%. The efficacy and safety profiles of Dato-DXd were similar among patients with and without baseline BMs. The study results suggest that Dato-DXd, as a novel TROP2-ADC drug, not only shows potential in controlling intracranial lesions but may also become a postresistance treatment option for patients with NSCLC BMs (50). BL-B01D1, a bispecific ADC targeting both EGFR and HER3, consists of the dual-specificity antibody SI-B001 and the topoisomerase I inhibitor Ed-04, connected by a cleavable linker with a DAR of 8. The potential of BL-B01D1 in NSCLC treatment was presented at the ESMO Congress 2023. The study involved 102 participants, including 75 who had received prior treatment with or without BMs and 27 untreated patients with BMs. The median number of treatment lines was 3, with 90% of the study population having previously received TKI therapy or immunotherapy. The overall cORR was 39.2%, with 33.3% in the untreated BMs group and 41.3% in the treated or non-BM group. The overall mPFS was 5.6 months, with 4.1 months in the untreated BMs group and 6.8 months in the treated or non-BM group. These findings indicate the encouraging efficacy of this bispecific ADC in patients with NSCLC BMs (51).
Discussion
Patients with lung cancer with BM generally have a poor prognosis in terms of OS, especially those with leptomeningeal metastasis. The main treatment mode for lung cancer BM is a combination of systemic therapy and local treatment. However, challenges remain in the selection of systemic therapy due to the barrier of the BBB, resistance to targeted therapy, and limitations on the reuse of local treatment. The emergence of ADCs has overcome the limitations of traditional chemotherapy and acquired resistance to molecular targeted therapy. ADCs directly deliver the drug to the target tumor cells through the specific targeting action of the antibody, enter the CNS through the endocytosis of endothelial cells, and have demonstrated good intracranial anticancer activity in both preclinical and clinical studies, offering new options for the treatment of lung cancer BM.
Studies have shown that the HER2-targeted ADC drug T-DXd has significant intracranial benefits in the treatment of breast cancer and lung cancer BMs (43,44,48,49). However, not all ADCs demonstrate significant efficacy against BM; for instance, the EGFR-targeted ADC depatuxizumab mafodotin (Depatux-M) failed to bring OS benefit to patients with glioblastoma in a phase III clinical trial (61). In an exploratory post hoc analysis of a phase III trial (ASCENT), 61 patients with triple-negative breast cancer and stable BM were enrolled, 31 of whom received sacituzumab govitecan and 29 chemotherapy, with an ORR of 3% and 0% and an mPFS of 2.8 and 1.6 months, respectively. The use of sacituzumab govitecan for treating patients with BMs from breast cancer has not yet shown to confer significant therapeutic benefits as compared to chemotherapy (62).
Research suggests that various factors determine the different efficacies of ADCs in intracranial cancers (63) (Figure 1). First, the structure and design of different drugs play a decisive role in inhibiting intracranial tumors. The new ADC drug T-DXd has undergone significant changes compared to the previous-generation ADC drug T-DM1, such as the use of a potent topoisomerase I inhibitor DXd as the drug payload, which avoids cross-resistance with commonly used microtubule inhibitors such as paclitaxel in breast cancer through leveraging a different mechanism. T-DXd has a higher DAR, better homogeneity, increased payload, and stronger cytotoxicity. Additionally, the cleavable tetrapeptide linker and improved membrane permeability allow for efficient release of the payload within tumor cells and its spread to adjacent tumor cells, resulting in a strong “bystander effect” and overcoming tumor heterogeneity. These advantages have led to a revolutionary improvement in the efficacy of T-DXd. In the phase 3 DESTINY-Breast03 study, a head-to-head comparison of the two generations of ADCs showed that the mPFS of T-DXd was four times that of T-DM1, reaching an unprecedented 29.0 months (64). In patients with BMs, the ORR of those treated with T-DXd was three times that of T-DM1, with the mPFS being five times that of the T-DM1 group (44). Second, the ability of ADCs to penetrate the BBB is another important factor affecting the treatment of BM. The uptake of ADCs by the CNS is heterogeneous, and ADCs with uniform DAR are more likely to pass through the BBB or BTB (63). T-DXd, with its optimized conjugation technology, may facilitate drug entry into the brain due to a more uniform DAR (65). ADCs using substrates of non-ATP-binding cassette transporters or chemically modified payloads can reduce the affinity for drug efflux pumps, which may further increase the accumulation of the payload in the CNS, thereby enhancing the intracranial activity of ADCs (63). T-DXd is a poor substrate for P-glycoprotein, which may help maintain higher intracranial drug concentrations compared to T-DM1 (28). Third, the bystander effect of the payload may play a significant role in the activity of ADCs within the CNS. The payload of ADCs can spread between adjacent cells within the tumor through the bystander effect, exerting anticancer effects on tumor cells with low or no expression of the target antigen. The stability of the chemical bond between the antibody and the linker, the stability of the linker, and the nature of the cytotoxin are critical factors affecting the bystander effect. The realization of a reasonable bystander effect mainly depends on the unique design of ADCs. As previously mentioned, T-DXd and HER3-DXd are based on the enzyme-cleavable linker GGFG carrying a new topoisomerase I inhibitor, which exerts a potent bystander effect, and thus have shown good anticancer activity and safety in clinical trials of patients with lung cancer and BM.
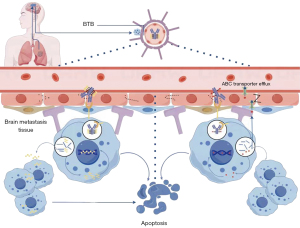
The existence of the BBB is a key reason why substantial therapeutic breakthroughs have not been achieved for treatments involving patients with NSCLC BMs. Another important factor may be the molecular characteristics of brain metastatic tumor cells differing from those of the primary tumor and/or extracranial metastases, which can result in unpredictable volatility in the response of intracranial metastases to targeted drugs (66-68). Studies have shown that the positive expression rates of EGFR and HER3 in primary NSCLC tumors are approximately 52.3% and 82.7%, respectively, while in BMs, these rates are 62.7% and 91.2%, respectively, suggesting that the expression rates of EGFR and HER3 in BMs might be higher than those in the primary tumor (69). A study comparing HER2 expression in primary breast cancer lesions and BMs found that in 21% of patients, the HER2 expression in BMs was inconsistent with that of the primary lesion. The primary lesion had a HER2 expression rate of 66%, while the BM had a rate of 81% (70). Furthermore, the positive rate of HER3 in HER2-positive BMs was significantly higher than that in HER2-negative cases (71). This evidence suggests that novel therapeutic approaches targeting HER2 and HER3, which can cross the BBB, may achieve breakthroughs in patients with BMs from breast and lung cancer. The positive results of T-DXd in patients with BMs from breast and lung cancer, as well as HER3-DXd and BL-B01D1 in those with lung cancer BM, have validated this perspective. Another related aspect is finding the reliable predictive biomarkers for each ADC in NSCLC. For example, HER-2 IHC positivity has been predictive of response rate in NSCLC given that HER-2 positive tumors with 3+ IHC are FDA approved across all solid tumors per results of the DESTINY-PanTumor02 study (72). However, in Trop-2, IHC positivity has not been predictive of response rate and OS (73). Thus, further studies such as utilizing machine learning and looking at membrane and cytoplasm expression to better predict Trop-2 ADC efficacy (74). In determining whether ADCs that are effective for extracranial tumor lesions can achieve similarly significant therapeutic results in the control of BMs, future research may need to consider the differences in molecular characteristics between BMs and the primary tumors as an important factor along with identifying better predictive biomarkers.
Radiotherapy, as an important treatment modality for BMs, can effectively and reversibly disrupt the BBB. It also induces immunogenic cell death, increasing the release of tumor antigens and the expression of MHC class I molecules on tumor cells, thereby activating antigen-presenting cells, CD8+ T cells, and other components to initiate the host immune response (75). Studies have shown that radiotherapy has a synergistic effect with immunotherapy and can significantly improve intracranial PFS. Administering radiotherapy and immunotherapy within a 1-month interval may be more beneficial for the control of intracranial diseases (76). In theory, radiotherapy may facilitate the entry of a higher concentration of ADCs into the brain. The tumor antigens released by the destroyed cancer cells could potentially stimulate the host’s immune response and further enhance the therapeutic efficacy of the ADCs. The exploration of the combination of radiotherapy and ADCs in the treatment of BMs is promising and highly anticipated in future research. However, this combination itself presents several issues that need to be addressed. For instance, the concurrent use of stereotactic radiosurgery and ADCs may increase the incidence of symptomatic radiation necrosis (77). Additionally, the optimal sequence and tolerable dosages of radiotherapy in conjunction with ADCs also require further exploration in clinical settings. The combination of ADC with TKIs, immunotherapy (78), and bispecific antibody ADCs in malignant tumors is being explored, such as HER3-DXd in combination with osimertinib (NCT04676477), BL-B01D1 in combination with osimertinib mesylate tablets (NCT05880706) and REGN5093-M114 (METxMET ADC) in combination with cemiplimab (NCT04982224). In a phase 1b study (NCT02099058), patients with c-Met+ NSCLC tolerated the combination therapy of telisotuzumab vedotin plus nivolumab well but had limited antitumor activity, with an objective remission rate of only 7.4% (79). Another study has shown that RC48 (disitamab vedotin)-ADC combined with PD-1 inhibitors demonstrated a longer mPFS (8.2 months), compared to the mPFS (6.9 months) of the RC48-ADC monotherapy group (HR =1.10, 95% CI: 0.31–3.93, P=0.50) in urothelial carcinoma (78). If the optimal balance between efficacy and toxicity can be obtained and the best-structured ADCs and the most effective combination strategies identified, there may be cause for new optimism in patients with lung cancer BMs.
Conclusions
The incidence of lung cancer BM is high, there are few effective treatment options available, leading to poor outcomes. New ADCs, represented by T-DXd and HER3-DXd, have demonstrated excellent antitumor activities and survival benefits in clinical applications, indicating that ADCs can cross the BBB or BTB and hold promise as a new treatment approach for lung cancer with BMs. However, there are also some ADCs that have not demonstrated significant benefits in the treating patients with BMs. An analysis of successful cases and preclinical research results suggests that the design and structure of ADCs, the uniformity of DAR, and the lipophilicity of the payload drug are closely related to the effectiveness of intracranial tumor control. The exploration of more effective ADCs, biomarker and treatment strategies are key to providing hope for patients within lung cancer BMs.
Acknowledgments
Funding: This work was supported by
Footnote
Reporting Checklist: The authors have completed the Narrative Review reporting checklist. Available at https://tlcr.amegroups.com/article/view/10.21037/tlcr-24-964/rc
Peer Review File: Available at https://tlcr.amegroups.com/article/view/10.21037/tlcr-24-964/prf
Conflicts of Interest: All authors have completed the ICMJE uniform disclosure form (available at https://tlcr.amegroups.com/article/view/10.21037/tlcr-24-964/coif). T.H. serves as an unpaid editorial board member of Translational Lung Cancer Research from January 2024 to December 2025. R.H. is a consultant for MJH Life Sciences and Takeda, has received honoraria from DAVA Oncology and The Dedham Group, and has participated in the advisory board meeting for OncoHost. The other authors have no conflicts of interest to declare.
Ethical Statement: The authors are accountable for all aspects of the work in ensuring that questions related to the accuracy or integrity of any part of the work are appropriately investigated and resolved.
Open Access Statement: This is an Open Access article distributed in accordance with the Creative Commons Attribution-NonCommercial-NoDerivs 4.0 International License (CC BY-NC-ND 4.0), which permits the non-commercial replication and distribution of the article with the strict proviso that no changes or edits are made and the original work is properly cited (including links to both the formal publication through the relevant DOI and the license). See: https://creativecommons.org/licenses/by-nc-nd/4.0/.
References
- Siegel RL, Miller KD, Wagle NS, et al. Cancer statistics, 2023. CA Cancer J Clin 2023;73:17-48. [Crossref] [PubMed]
- Xia C, Dong X, Li H, et al. Cancer statistics in China and United States, 2022: profiles, trends, and determinants. Chin Med J (Engl) 2022;135:584-90. [Crossref] [PubMed]
- Nayak L, Lee EQ, Wen PY. Epidemiology of brain metastases. Curr Oncol Rep 2012;14:48-54. [Crossref] [PubMed]
- Vogelbaum MA, Brown PD, Messersmith H, et al. Treatment for Brain Metastases: ASCO-SNO-ASTRO Guideline. J Clin Oncol 2022;40:492-516. [Crossref] [PubMed]
- Goncalves PH, Peterson SL, Vigneau FD, et al. Risk of brain metastases in patients with nonmetastatic lung cancer: Analysis of the Metropolitan Detroit Surveillance, Epidemiology, and End Results (SEER) data. Cancer 2016;122:1921-7. [Crossref] [PubMed]
- Murray N, Sheehan F. Limited stage small cell lung cancer. Curr Treat Options Oncol 2001;2:63-70. [Crossref] [PubMed]
- Lamba N, Wen PY, Aizer AA. Epidemiology of brain metastases and leptomeningeal disease. Neuro Oncol 2021;23:1447-56. [Crossref] [PubMed]
- Nadler E, Espirito JL, Pavilack M, et al. Real-world disease burden and outcomes of brain metastases in EGFR mutation-positive non-small-cell lung cancer. Future Oncol 2020;16:1575-84. [Crossref] [PubMed]
- Strebhardt K, Ullrich A. Paul Ehrlich's magic bullet concept: 100 years of progress. Nat Rev Cancer 2008;8:473-80. [Crossref] [PubMed]
- Steeg PS. The blood-tumour barrier in cancer biology and therapy. Nat Rev Clin Oncol 2021;18:696-714. [Crossref] [PubMed]
- Arvanitis CD, Ferraro GB, Jain RK. The blood-brain barrier and blood-tumour barrier in brain tumours and metastases. Nat Rev Cancer 2020;20:26-41. [Crossref] [PubMed]
- Boire A, Brastianos PK, Garzia L, et al. Brain metastasis. Nat Rev Cancer 2020;20:4-11. [Crossref] [PubMed]
- Soria JC, Ohe Y, Vansteenkiste J, et al. Osimertinib in Untreated EGFR-Mutated Advanced Non-Small-Cell Lung Cancer. N Engl J Med 2018;378:113-25. [Crossref] [PubMed]
- Lu S, Dong X, Jian H, et al. AENEAS: A Randomized Phase III Trial of Aumolertinib Versus Gefitinib as First-Line Therapy for Locally Advanced or MetastaticNon-Small-Cell Lung Cancer With EGFR Exon 19 Deletion or L858R Mutations. J Clin Oncol 2022;40:3162-71. [Crossref] [PubMed]
- Shi Y, Chen G, Wang X, et al. Furmonertinib (AST2818) versus gefitinib as first-line therapy for Chinese patients with locally advanced or metastatic EGFR mutation-positive non-small-cell lung cancer (FURLONG): a multicentre, double-blind, randomised phase 3 study. Lancet Respir Med 2022;10:1019-28. [Crossref] [PubMed]
- Shaw AT, Bauer TM, de Marinis F, et al. First-Line Lorlatinib or Crizotinib in Advanced ALK-Positive Lung Cancer. N Engl J Med 2020;383:2018-29. [Crossref] [PubMed]
- Han G, Bi J, Tan W, et al. A retrospective analysis in patients with EGFR-mutant lung adenocarcinoma: is EGFR mutation associated with a higher incidence of brain metastasis? Oncotarget 2016;7:56998-7010. [Crossref] [PubMed]
- Griesinger F, Roeper J, Pöttgen C, et al. Brain metastases in ALK-positive NSCLC - time to adjust current treatment algorithms. Oncotarget 2018;9:35181-94. [Crossref] [PubMed]
- Goldberg SB, Schalper KA, Gettinger SN, et al. Pembrolizumab for management of patients with NSCLC and brain metastases: long-term results and biomarker analysis from a non-randomised, open-label, phase 2 trial. Lancet Oncol 2020;21:655-63. [Crossref] [PubMed]
- Gadgeel S, Rodríguez-Abreu D, Speranza G, et al. Updated Analysis From KEYNOTE-189: Pembrolizumab or Placebo Plus Pemetrexed and Platinum for Previously Untreated Metastatic Nonsquamous Non-Small-Cell Lung Cancer. J Clin Oncol 2020;38:1505-17. [Crossref] [PubMed]
- Paz-Ares L, Ciuleanu TE, Cobo M, et al. First-line nivolumab plus ipilimumab combined with two cycles of chemotherapy in patients with non-small-cell lung cancer (CheckMate 9LA): an international, randomised, open-label, phase 3 trial. Lancet Oncol 2021;22:198-211. [Crossref] [PubMed]
- Buttigliero C, Bertaglia V, Novello S. Anti-angiogenetic therapies for central nervous system metastases from non-small cell lung cancer. Transl Lung Cancer Res 2016;5:610-27. [Crossref] [PubMed]
- Ilhan-Mutlu A, Osswald M, Liao Y, et al. Bevacizumab Prevents Brain Metastases Formation in Lung Adenocarcinoma. Mol Cancer Ther 2016;15:702-10. [Crossref] [PubMed]
- Liang P, Wang YD, Wei ZM, et al. Bevacizumab for non-small cell lung cancer patients with brain metastasis: A meta-analysis. Open Med (Wars) 2020;15:589-97. [Crossref] [PubMed]
- Zhou Q, Xu CR, Cheng Y, et al. Bevacizumab plus erlotinib in Chinese patients with untreated, EGFR-mutated, advanced NSCLC (ARTEMIS-CTONG1509): A multicenter phase 3 study. Cancer Cell 2021;39:1279-1291.e3. [Crossref] [PubMed]
- Zhang L, Zhou C, Fang WF, et al. 174P Intracranial (IC) activity of ivonescimab (ivo) alone or in combination with platinum doublet chemotherapy (PC) in patients (Pts) with advanced non-small cell lung cancer (aNSCLC) and brain metastases (BMs). ESMO Open 2024;9:102749.
- Hoffmann RM, Coumbe BGT, Josephs DH, et al. Antibody structure and engineering considerations for the design and function of Antibody Drug Conjugates (ADCs). Oncoimmunology 2018;7:e1395127. [Crossref] [PubMed]
- Ogitani Y, Aida T, Hagihara K, et al. DS-8201a, A Novel HER2-Targeting ADC with a Novel DNA Topoisomerase I Inhibitor, Demonstrates a Promising Antitumor Efficacy with Differentiation from T-DM1. Clin Cancer Res 2016;22:5097-108. [Crossref] [PubMed]
- Fojo T, Menefee M. Mechanisms of multidrug resistance: the potential role of microtubule-stabilizing agents. Ann Oncol 2007;18:v3-8. [Crossref] [PubMed]
- Kovtun YV, Audette CA, Mayo MF, et al. Antibody-maytansinoid conjugates designed to bypass multidrug resistance. Cancer Res 2010;70:2528-37. [Crossref] [PubMed]
- Hamblett KJ, Senter PD, Chace DF, et al. Effects of drug loading on the antitumor activity of a monoclonal antibody drug conjugate. Clin Cancer Res 2004;10:7063-70. [Crossref] [PubMed]
- Nakada T, Sugihara K, Jikoh T, et al. The Latest Research and Development into the Antibody-Drug Conjugate, [fam-] Trastuzumab Deruxtecan (DS-8201a), for HER2 Cancer Therapy. Chem Pharm Bull (Tokyo) 2019;67:173-85.
- Tarantino P, Carmagnani Pestana R, Corti C, et al. Antibody-drug conjugates: Smart chemotherapy delivery across tumor histologies. CA Cancer J Clin 2022;72:165-82. [Crossref] [PubMed]
- Yu HA, Goto Y, Hayashi H, et al. HERTHENA-Lung01, a Phase II Trial of Patritumab Deruxtecan (HER3-DXd) in Epidermal Growth Factor Receptor-Mutated Non-Small-Cell Lung Cancer After Epidermal Growth Factor Receptor Tyrosine Kinase Inhibitor Therapy and Platinum-Based Chemotherapy. J Clin Oncol 2023;41:5363-75. [Crossref] [PubMed]
- St-Amour I, Paré I, Alata W, et al. Brain bioavailability of human intravenous immunoglobulin and its transport through the murine blood-brain barrier. J Cereb Blood Flow Metab 2013;33:1983-92. [Crossref] [PubMed]
- Kurihara H, Hamada A, Yoshida M, et al. (64)Cu-DOTA-trastuzumab PET imaging and HER2 specificity of brain metastases in HER2-positive breast cancer patients. EJNMMI Res 2015;5:8. [Crossref] [PubMed]
- Askoxylakis V, Ferraro GB, Kodack DP, et al. Preclinical Efficacy of Ado-trastuzumab Emtansine in the Brain Microenvironment. J Natl Cancer Inst 2016;108:djv313. [Crossref] [PubMed]
- Kabraji S, Ni J, Sammons S, et al. Preclinical and Clinical Efficacy of Trastuzumab Deruxtecan in Breast Cancer Brain Metastases. Clin Cancer Res 2023;29:174-82. [Crossref] [PubMed]
- Jones KJ, Suksomboon M. Abstract 1911: Dato-DXd mediates anti-tumor activity in preclinical TROP2-expressing intracranial tumor model. Cancer Res 2024;84:1911.
- Offenhäuser C, Al-Ejeh F, Puttick S, et al. EphA3 Pay-Loaded Antibody Therapeutics for the Treatment of Glioblastoma. Cancers (Basel) 2018;10:519. [Crossref] [PubMed]
- Krop IE, Lin NU, Blackwell K, et al. Trastuzumab emtansine (T-DM1) versus lapatinib plus capecitabine in patients with HER2-positive metastatic breast cancer and central nervous system metastases: a retrospective, exploratory analysis in EMILIA. Ann Oncol 2015;26:113-9. [Crossref] [PubMed]
- Montemurro F, Delaloge S, Barrios CH, et al. Trastuzumab emtansine (T-DM1) in patients with HER2-positive metastatic breast cancer and brain metastases: exploratory final analysis of cohort 1 from KAMILLA, a single-arm phase IIIb clinical trial Ann Oncol 2020;31:1350-8. [Crossref] [PubMed]
- Jerusalem G, Park YH, Yamashita T, et al. Trastuzumab Deruxtecan in HER2-Positive Metastatic Breast Cancer Patients with Brain Metastases: A DESTINY-Breast01 Subgroup Analysis. Cancer Discov 2022;12:2754-62. [Crossref] [PubMed]
- Hurvitz S, Kim SB, Chung WP, et al. Abstract GS3-01: Trastuzumab deruxtecan (T-DXd; DS-8201a) vs. trastuzumab emtansine (T-DM1) in patients (pts) with HER2+ metastatic breast cancer (mBC): subgroup analyses from the randomized phase 3 study DESTINY-Breast03. Cancer Res 2022;82:GS3-01.
- Bartsch R, Berghoff AS, Furtner J, et al. Trastuzumab deruxtecan in HER2-positive breast cancer with brain metastases: a single-arm, phase 2 trial. Nat Med 2022;28:1840-7. [Crossref] [PubMed]
- Bartsch R, Berghoff AS, Furtner J, et al. Final outcome analysis from the phase II TUXEDO-1 trial of trastuzumab-deruxtecan in HER2-positive breast cancer patients with active brain metastases. Neuro Oncol 2024;26:2305-15. [Crossref] [PubMed]
- Pérez-García JM, Vaz Batista M, Cortez P, et al. Trastuzumab deruxtecan in patients with central nervous system involvement from HER2-positive breast cancer: The DEBBRAH trial. Neuro Oncol 2023;25:157-66. [Crossref] [PubMed]
- Li BT, Smit EF, Goto Y, et al. Trastuzumab Deruxtecan in HER2-Mutant Non-Small-Cell Lung Cancer. N Engl J Med 2022;386:241-51. [Crossref] [PubMed]
- Li BT, Planchard D, Goto K, et al. 1321MO Trastuzumab deruxtecan (T-DXd) in patients (pts) with HER2 (ERBB2)-mutant (HER2m) metastatic non–small cell lung cancer (NSCLC) with and without brain metastases (BMs): Pooled analyses from DESTINY-Lung01 and DESTINY-Lung02. Ann Oncol 2023;34:S762-3.
- Lisberg A, Ahn MJ, Kitazono S, et al. Intracranial efficacy of datopotamab deruxtecan (Dato-DXd) in patients (pts) with previously treated advanced/metastatic non-small cell lung cancer (a/m NSCLC) with actionable genomic alterations (AGA): Results from TROPION-Lung05. J Clin Oncol 2024;42:8593.
- Zhang L, Ma Y, Zhao Y, et al. BL-B01D1, a first-in-class EGFRxHER3 bispecific antibody-drug conjugate (ADC), in patients with locally advanced or metastatic solid tumor: Results from a first-in-human phase 1 study. J Clin Oncol 2023;41:3001.
- Michelon I, Vilbert M, Marinho AD, et al. Trastuzumab deruxtecan in human epidermal growth factor receptor 2-positive breast cancer brain metastases: a systematic review and meta-analysis. ESMO Open 2024;9:102233. [Crossref] [PubMed]
- Harbeck N, Ciruelos E, Jerusalem GDESTINY-Breast12 study group, et al. Trastuzumab deruxtecan in HER2-positive advanced breast cancer with or without brain metastases: a phase 3b/4 trial. Nat Med 2024; Epub ahead of print. [Crossref]
- AstraZeneca. Enhertu approved in the US as first tumour-agnostic HER2-directed therapy for previously treated patients with metastatic HER2-positive solid tumours. Available online: https://www.astrazeneca.com/media-centre/press-releases/2024/enhertu-approved-in-the-us-as-first-tumour-agnostic-her2-directed-therapy-for-previously-treated-patients-with-metastatic-her2-positive-solid-tumours.html
- Goto K, Goto Y, Kubo T, et al. Trastuzumab Deruxtecan in Patients With HER2-Mutant Metastatic Non-Small-Cell Lung Cancer: Primary Results From the Randomized, Phase II DESTINY-Lung02 Trial. J Clin Oncol 2023;41:4852-63. [Crossref] [PubMed]
- Stephens P, Hunter C, Bignell G, et al. Lung cancer: intragenic ERBB2 kinase mutations in tumours. Nature 2004;431:525-6. [Crossref] [PubMed]
- Offin M, Feldman D, Ni A, et al. Frequency and outcomes of brain metastases in patients with HER2-mutant lung cancers. Cancer 2019;125:4380-7. [Crossref] [PubMed]
- Planchard D, Kim HR, Suksombooncharoen T, et al. OA16.05 Trastuzumab Deruxtecan Monotherapy in Pretreated HER2-overexpressing Nonsquamous Non-Small Cell Lung Cancer: DESTINY-Lung03 Part 1. J Thorac Oncol 2024;19:S46-7.
- Jänne PA, Baik C, Su WC, et al. Efficacy and Safety of Patritumab Deruxtecan (HER3-DXd) in EGFR Inhibitor–Resistant, EGFR-Mutated Non–Small Cell Lung Cancer. Cancer Discov 2022;12:74-89. [Crossref] [PubMed]
- Ahn MJ, Tanaka K, Paz-Ares L, et al. Datopotamab Deruxtecan Versus Docetaxel for Previously Treated Advanced or Metastatic Non-Small Cell Lung Cancer: The Randomized, Open-Label Phase III TROPION-Lung01 Study. J Clin Oncol 2024; Epub ahead of print. [Crossref]
- Lassman AB, Pugh SL, Wang TJC, et al. Depatuxizumab mafodotin in EGFR-amplified newly diagnosed glioblastoma: A phase III randomized clinical trial. Neuro Oncol 2023;25:339-50. [Crossref] [PubMed]
- Diéras V, Weaver R, Tolaney SM, et al. Abstract PD13-07: Subgroup analysis of patients with brain metastases from the phase 3 ASCENT study of sacituzumab govitecan versus chemotherapy in metastatic triple-negative breast cancer. Cancer Res 2021;81:PD13-07.
- Mair MJ, Bartsch R, Le Rhun E, et al. Understanding the activity of antibody-drug conjugates in primary and secondary brain tumours. Nat Rev Clin Oncol 2023;20:372-89. [Crossref] [PubMed]
- Cortés J, Hurvitz SA, Im SA, et al. Trastuzumab deruxtecan versus trastuzumab emtansine in HER2-positive metastatic breast cancer: long-term survival analysis of the DESTINY-Breast03 trial. Nat Med 2024;30:2208-15. [Crossref] [PubMed]
- Li J, Wang M, Xu S, et al. The Strategies and Mechanisms of Immune Checkpoint Inhibitors for Brain Metastases in NSCLC. Front Pharmacol 2022;13:841623. [Crossref] [PubMed]
- Wang M, Ran L, Liu B, et al. Disturbed meningeal lymphatic function associated with malignancy and progression in patients with intracranial malignant tumors. Med 2023;4:898-912.e4. [Crossref] [PubMed]
- Liu D, Bai J, Chen Q, et al. Brain metastases: It takes two factors for a primary cancer to metastasize to brain. Front Oncol 2022;12:1003715. [Crossref] [PubMed]
- Soffietti R, Ahluwalia M, Lin N, et al. Management of brain metastases according to molecular subtypes. Nat Rev Neurol 2020;16:557-74. [Crossref] [PubMed]
- Scharpenseel H, Hanssen A, Loges S, et al. EGFR and HER3 expression in circulating tumor cells and tumor tissue from non-small cell lung cancer patients. Sci Rep 2019;9:7406. [Crossref] [PubMed]
- Moss NS, Singh JM, Reiner AS, et al. Incidence of HER2-expressing brain metastases in patients with HER2-null breast cancer: a matched case analysis. NPJ Breast Cancer 2023;9:86. [Crossref] [PubMed]
- Tomasich E, Steindl A, Paiato C, et al. Frequent Overexpression of HER3 in Brain Metastases from Breast and Lung Cancer. Clin Cancer Res 2023;29:3225-36. [Crossref] [PubMed]
- Meric-Bernstam F, Makker V, Oaknin A, et al. Efficacy and Safety of Trastuzumab Deruxtecan in Patients With HER2-Expressing Solid Tumors: Primary Results From the DESTINY-PanTumor02 Phase II Trial. J Clin Oncol 2024;42:47-58. [Crossref] [PubMed]
- Heist RS, Guarino MJ, Masters G, et al. Therapy of Advanced Non-Small-Cell Lung Cancer With an SN-38-Anti-Trop-2 Drug Conjugate, Sacituzumab Govitecan. J Clin Oncol 2017;35:2790-7. [Crossref] [PubMed]
- Garassino MC, Sands J, Paz-Ares L, et al. Normalized Membrane Ratio of TROP2 by Quantitative Continuous Scoring is Predictive of Clinical Outcomes in TROPION-Lung01. Presented at: 2024 IASLC World Conference on Lung Cancer; 7-11 September 2024; San Diego, CA. Abstract 4037.
- Sato H, Okonogi N, Nakano T. Rationale of combination of anti-PD-1/PD-L1 antibody therapy and radiotherapy for cancer treatment. Int J Clin Oncol 2020;25:801-9. [Crossref] [PubMed]
- Antelo G, Comas S, Casas F, et al. Clinical outcomes and timing on the combination of focal radiation therapy and immunotherapy for the treatment of brain metastases. Front Immunol 2023;14:1236398. [Crossref] [PubMed]
- Lebow ES, Pike LRG, Seidman AD, et al. Symptomatic Necrosis With Antibody-Drug Conjugates and Concurrent Stereotactic Radiotherapy for Brain Metastases. JAMA Oncol 2023;9:1729-33. [Crossref] [PubMed]
- Xu J, Zhang H, Zhang L, et al. Real-world effectiveness and safety of RC48-ADC alone or in combination with PD-1 inhibitors for patients with locally advanced or metastatic urothelial carcinoma: A multicenter, retrospective clinical study. Cancer Med 2023;12:21159-71.
- Camidge DR, Barlesi F, Goldman JW, et al. A Phase 1b Study of Telisotuzumab Vedotin in Combination With Nivolumab in Patients With NSCLC. JTO Clin Res Rep 2022;3:100262. [Crossref] [PubMed]