GNGT1 remodels the tumor microenvironment and promotes immune escape through enhancing tumor stemness and modulating the fibrinogen beta chain-neutrophil extracellular trap signaling axis in lung adenocarcinoma
Highlight box
Key findings
• GNGT1 plays a key role in the early diagnosis of lung adenocarcinoma (LUAD). This finding introduces a novel biomarker for the early detection of LUAD, underscoring its substantial potential for clinical translation.
• A lung-specific GNGT1 transgenic spontaneous tumor formation mouse model was successfully constructed for the first time, which provides a crucial tool for further investigation.
• This study holds significant potential for guiding the development of novel therapeutic strategies targeting LUAD, particularly for specific patient populations.
What is known and what is new?
• Identifying markers for early diagnosis or predict treatment has been a consistent challenge in the diagnosis of lung cancer. This study is the first to demonstrate that the oncogenic factor GNGT1 is integral to the early diagnosis of LUAD. In this study, GNGT1 was found to be significantly associated with the onset, progression, and prognosis of lung cancer, indicating that GNGT1 overexpression may constitute an early driving event in the pathogenesis of LUAD.
• The G protein family plays an important role in tumors, but the mechanism of action of GNGT1 in lung cancer has not been elucidated. This study investigated the molecular mechanisms by which GNGT1 regulates the occurrence and progression of lung tumors, thereby addressing a significant gap in knowledge in this field.
What is the implication, and what should change now?
• The clarification of these mechanisms offers a theoretical foundation for the development of pharmacological agents targeting GNGT1 and its associated pathways. However, the direct regulation and the specific intermolecular mode of GNGT1 on tumor cells and the tumor microenvironment remain to be further studied.
Introduction
Lung cancer is the leading cause of cancer-associated mortality worldwide and accounts for 18% of cancer-related mortality and 12.4% of cancer incidence (1). Lung adenocarcinoma (LUAD), as the most common type of lung cancer, has been linked to millions of deaths over the past several decades and has an increasing incidence (2). Although a variety of treatment options have been implemented for LUAD, the overall survival (OS) rate remains still low due to the latency of distal metastasis and aggressiveness disease (3). Traditional options for LUAD therapy, including surgical resection, radiation treatment, and oral dosing with chemotherapy agents, remain unsatisfactory and are associated with severe adverse side effects (4). As an alternative, molecular targeted therapies have shown promise as a strategy for improving cancer symptoms with less intense side effects, and immunological therapy has shown success in treating some solid tumors (5). Despite the vast potential of immunological therapy and the widespread success of immune checkpoint inhibitors (ICIs) in patients with non-small cell lung cancer (NSCLC), approximately half of patients experience immune-related adverse events (6), with distal metastases remaining an unaddressed challenge. The identification of novel core genes associated with LUAD progression holds immense promise for the development of new diagnostic and treatment approaches.
Metastasis in LUAD is a complex, multistep interaction between tumor cells and the tumor microenvironment (TME) (7). First, the change in biological traits of tumor cells is a direct promoting factor. For example, tumor cells increase stemness to enhance its growth and proliferation capacity (8), epithelial-mesenchymal transition (EMT) confers migratory and invasive capability to tumor cells, the decreased expression of adhesion molecules on tumor cells weakens the adhesion effect between them (9), and tumor cells secrete matrix metalloproteinases (MMPs) promote the degradation of the extracellular matrix (10). All these processes can contribute to the degradation of the histological barrier, which induces the migration and metastasis of tumor cells. Moreover, the emergence of the TME has been shown to be critical to tumor progression (11-13). The TME encompasses a wide variety of cells, including neutrophils, lymphocytes, macrophages, and other stromal cell (14).
A large number of growth factors, chemokines, and matrix degradation enzymes are released in recruitment of tumor cells, which may induce angiogenesis, inhibit immune response, generate tumor stem cells, and change the extracellular matrix components to provide favorable conditions for the invasion and migration of tumor cells (15). It follows that the progression of lung cancer depends on the functional alteration of lung cancer cells and their interaction with the TME. In view of this, exploring the key molecules and mechanisms that affect the biological traits of lung cancer cells and regulate the interaction between lung cancer cells and the TME is expected to provide new targets for intervening in lung cancer progression and a provide a theoretical basis for the establishment of new strategies for the prevention and treatment of lung cancer.
Heterotrimeric G-proteins are composed of a Gα subunit and a Gβγ dimer. G protein binds to the G protein-coupled receptor (GPCR) family, which consists of more than 800 members and accounts for 4% of human genes, enacting a variety of structural and signal transduction functions (16). As the oncogenic driver of a variety of common cancers, G proteins are considered to be part of the cancer-related genome (17). The role of Gα and Gβ in the tumorigenesis of tumors is attracting increased attention in the field of clinical oncology (18,19), but despite being a close partner of β, Gγ, along with its potential function, has been less intensely studied. GNGT1 encodes the gamma subunit of transduction, which also known as GMPase, and mediates the activation of cyclic guanosine triphosphate-specific phosphodiesterase. GNGT1 exerts a regulatory influence on LUAD, and we emphasize the significant role of G proteins and their associated pathways in tumor biology. We anticipate further exploration of targeted therapeutics to enhance the clinical applicability of these findings. We screened for tumor-specific differentially expressed genes (DEGs), including GNGT1. Furthermore, we performed functional enrichment and gene enrichment analysis to identify the roles of GNGT1-associated hub genes in LUAD. Finally, we validated the expression levels of these genes via quantitative polymerase chain reaction (qPCR), immunohistochemistry (IHC), and immunofluorescence (IF) staining in genetically modified mouse lung tissues and the LUAD tissue of patients. Taken together, our results indicate that GNGT1 interacts with tumor-driver genes and enhances the stemness and EMT of tumor cells. Meanwhile, increased expression of GNGT1 upregulates the secretion of fibrinogen beta chain (FGB), which subsequently induces human neutrophils to release neutrophils extracellular traps (NETs) and remodel the TME, thus promoting the biological alteration and immune escape of cancer cells critical to metastasis. We present this article in accordance with the ARRIVE and MDAR reporting checklists (available at https://tlcr.amegroups.com/article/view/10.21037/tlcr-2024-1200/rc).
Methods
Data collection and processing
The transcriptome sequencing profiles and clinical information for LUAD and normal tissues were obtained from The Cancer Genome Atlas (TCGA; https://www.cancer.gov/tcga) database and Gene Expression Omnibus (GEO; https://www.ncbi.nlm.nih.gov/geo/) database. TCGA database contains 515 LUAD samples and 58 paired normal samples that were evaluated on the HiSeq platform (Illumina, San Diego, CA, USA). Raw data were standardized according to the transcripts per kilobase million (TPM). The GSE7670, GSE81089, and GSE118370 datasets were selected to obtain the differential genes between LUAD and normal tissue. GES30219 and GSE10072 were used to further verify the expression of GNGT1 in LUAD and adjacent normal tissues, while GSE41271 was used to validate the effect of GNGT1 expression on OS. The analysis of expression data related to lymph nodes metastasis and T stage was performed via the online University of Alabama at Birmingham Cancer (UALCAN; https://ualcan.path.uab.edu) database and validated by IHC and follow-up analysis of patients with LUAD. The expression heatmap of the key DEGs were visualized with the “ComplexHeatmap” (v. 2.13.1) package in R (The R Foundation of Statistical Computing). The study was conducted in accordance with the Declaration of Helsinki (as revised in 2013).
DEGs and functional enrichment and survival analysis
Three GEO datasets (GSE7670, GSE810809, and GSE11837) were selected to analyze the DEGs between tumor tissues and adjacent normal tissues. The DEGs were filtered using a cutoff value of log fold change (FC) >1, 2, and 3 in GSE7670, GSE810809, and GSE11837 respectively and P<0.05 to confirm the numbers of DEGs between 1,000 and 2,000. Intersection of the DEGs in these three datasets was obtained to determine a gene set, and then the intersection between this gene set and the top 300 DEGs in TCGA database was obtained to confirm the hub genes in LUAD. Subsequently, 515 patients with LUAD from TCGA were stratified based on the GNGT1 expression level into a high-GNGT1 group (GH) and low-GNGT1 group (GL). An expression value of 2.0 was used as cutoff criterion to distinguish the DEGs between the GH group and GL group, which were filtered using thresholds of log FC >1 and P value <0.05. The DEGs of TCGA and GEO were analyzed and visualized with the R software (version 4.2.2) packages “DESeq2”, “ggplot2”, and “Venn Diagram” to evaluate overlapping DEGs. Gene Ontology (GO) analysis was employed to provide a standardized description of gene products in terms of molecular function (MF), biological process (BP), and cellular component (CC). Meanwhile, the Kyoto Encyclopedia of Genes and Genomes (KEGG) database was used to clarify the function and protein expression of DEGs. GO and KEGG enrichment analyses were conducted and visualized with the R package “cluster Profiler” and “ggplot2”, with P<0.05 indicating statistical significance. We further performed functional enrichment in Metascape (https://metascape.org/gp/index.html) for each gene that was associated with OS. Gene set enrichment analysis (GSEA) was performed with GSEA software version 4.3.2 (http://www.gsea-msigdb.org/gsea/index.jsp), with P<0.05 indicating statistical significance. Single-cell analysis of 14 LUAD function states (angiogenesis, apoptosis, cell cycle, differentiation, DNA damage, DNA repair, EMT, hypoxia, inflammation, invasion, metastasis, proliferation, quiescence, and stemness) and GNGT1 expression was performed via the Cancer SEA online database (https://ngdc.cncb.ac.cn/databasecommons/). The OS of patients with LUAD in TCGA and GSE41271 was analyzed and visualized with R package using ‘‘survminer” (v. 3.3.1) and ‘‘survival” (v. 3.2.10). A log-rank P value <0.05 was considered statistically significant. To evaluate the risk score and subsequently construct a nomogram, Cox univariate and multivariate analyses were performed with the R packages ‘‘survival” (v. 3.2.10) and “rms”.
Gene expression, methylation, and mutation analysis
The expression of GNGT1 in pancancer analysis data was collected in TCGA and visualized with the R package “ggplot2”, and the expression data of GNGT1 in LUAD with paired normal tissue were verified in GEO datasets (GSE30219 and GSE10072). The tumor samples of LUAD were collected from The First Affiliated Hospital of Naval Medical University (8 pairs of frozen tissues for qPCR and 58 pairs of paraffin-embedded tissue for IHC). The details of patients with LUAD are provided in Table S1. Gene alteration and methylation data were collected from OncoVar (https://oncovar.org/), cBioportal (https://www.cbioportal.org), MEXPRESS (https://mexpress.ugent.be) and UALCAN (https://ualcan.path.uab.edu/) in LUAD and adjacent normal lung tissue. P<0.05 was considered statistically significant. The expression and function of GNGT1 were further verified in LUAD patient tissues and in transgenic mice overexpressing GNGT1 under control of the lung-specific promoter (GNGT1fl/+ mice).
Immune cell infiltration analysis
Comparisons of immune cell infiltration levels among LUAD with different somatic copy number alterations (including deep deletion, arm-level deletion, diploid/normal, arm-level gain, and high amplification) for GNGT1 were visualized with Tumor Immune Estimation Resource (TIMER; https://cistrome.shinyapps.io/timer/). The R packages ‘‘GSVA” and “ggplot2” (v. 1.46.0) were applied to generate lollipop diagrams and boxplots for visualization of the correlations between GNGT1 expression and 24 immune cell types, which included activated dendritic cells (aDCs), B cells, cytotoxic cells, dendritic cells (DCs), eosinophils, immature DCs (iDCs), macrophages, mast cells, neutrophils, CD56 bright natural killer (NK) cells, CD56 dim NK cells, NK cells, plasmacytoid DCs (pDCs), T cells, T helper (Th) cells, T central memory (Tcm) cells, T effector memory (Tem) cells, T follicular helper (TFH) cells, γδ T cells, Th1 cells, Th17 cells, Th2 cells, and T regulatory cells (Tregs) across TCGA database. The Wilcoxon rank-sum test, conducted via the R packages “stats” and “car”, was used to analyze the statistical data, with a P value <0.05 being considered statistically significant.
Real time qPCR, IHC staining, and IF analyses
The tumor tissue and adjacent normal tissue from patients with LUAD were collected, and real time qPCR (RT-qPCR) and IHC staining were performed to compare the messenger RNA (mRNA) and protein expression levels between tumor and normal tissues. Mice under stop code (LSL-GNGT1) were crossbred with Scgb1a1-Cre mice raised in a standard specific pathogen free (SPF) environment to generate LSL-GNGT1; Scgb1a1-Cre mice (GNGT1fl/+ mice). The animals were purchased from Southern Model Biology, Shanghai, China). Lung tissues were randomly collected from male GNGT1fl/+ mice and their double-negative C57BL/6J littermates at 4, 12, and 16 weeks of age, respectively to ascertain the function of GNGT1. Animals were excluded from the study if they died prematurely, thereby precluding the collection of histological data. The primers for qPCR are provided in Table S2. Rabbit anti-GNGT1 antibodies (cat. no. bs-13470R; Bioss Antibodies, Beijing, China) were diluted to 1:200, and IF staining was performed and scanned by Servicebio (Wuhan, China).
IHC was scored based on expression intensity and proportion. The expression intensity was categorized as negative (no coloring at all, score 0), weakly positive (scattered cytoplasm, score 1), moderately positive (score 2), strongly positive (score 3); meanwhile, proportion was scored based on the area of positive colored cells to total tumor cells as follows: <1%, score 0; 1–50%, score 1; and >50%, score 2. All results were scored by three pathologists in accordance with the double-blind random principle and the average score was taken, then the two scores were summed. An overall score greater than 3 was defined as high expression, while a score lower 3 was defined as low expression.
Ethical statement
Animal experiments were performed under a project license (No. CHEC2021-061) granted by Navy Military Medical University Affiliated Changhai Hospital Ethics Committee, in compliance with institutional guidelines for the care and use of animals. A protocol was prepared before the study without registration.
Statistical analysis
All statistical analyses were processed using R software version 4.2.2 and GraphPad version 8.0.1 (GraphPad Software, La Jolla, CA, USA). The Student’s t-test was used for comparing the differences between two groups. Correlations were analyzed with a Pearson correlation coefficients or the Fisher exact test. Kaplan-Meier curves and the log-rank test were used to assess the OS of patients with LUAD. Data are presented as the mean ± standard deviation. A P value <0.05 was considered statistically significant.
Results
The critical role of GNGT1 in the development and progression of LUAD
The DEGs between tumor and normal tissue in the GSE7670, GSE81089, and GSE118370 datasets were identified, respectively (Figure 1A). We then sought to identify the overlapping DEGs between the three datasets, and 63 DEGs were observed in LUAD (Figure 1B). In the LUAD datasets of TCGA, 3,647 up- and 1,076 downregulated DEGs were observed (Figure 1C). Based on the change of log FC, the top 300 change genes were chosen and the intersections between TCGA and GEO obtained, with GNGT1, SLC6A4, AGER, CST1, CYP24A1, MMP11, PCP4, KRT6A, COL11A1, and SPINK1 showing differential expression in LUAD (Figure 1D).
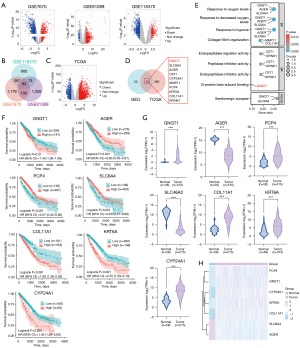
The function analysis of the gene set showed that the DEGs were primarily related to pathways such as response to decreased oxygen levels, response to hypoxia, and extracellular matrix structural constituent conferring tensile strength (Figure 1E). In the top BPs, the enriched genes were GNGT1, SLC6A4, and AGER. Hypoxia and matrix remodeling are important features of the TME. This suggested that the hub genes may affect the TME and further impact the biological properties of LUAD. Following this, the OS and the expression of the 10 genes were analyzed, respectively. GNGT1, CYP24A1, KRT6A, and COL11A1 were found to decrease the OS of patients with LUAD, while SLC6A4, AGER and PCP4 can increase the OS (Figure 1F and Figure S1A). In LUAD, the expression of SLC6A4 and AGER was downregulated, while that of GNGT1, CYP24A1, PCP4, KRT6A, and COL11A1 was upregulated (Figure 1G,1H and Figure S1B). The function of each gene was further analyzed with the online Metascape tool (Table S3). GNGT1 was found to be related with the RAS pathway, PI3K, AKT, and mTOR signaling, which may be highly correlated with tumorigenesis and development.
GNGT1 was upregulated in LUAD and was associated with poorer prognosis and higher N stage
The pancancer expression analysis of GNGT1 indicated that it was overexpressed in a variety of tumors, including LUAD (Figure 2A and Figure S2), further suggesting that GNGT1 may be an important gene in regulating the development and progression of tumors. To determine the reliability of the data, GNGT1 expression levels in LUAD were validated in GEO datasets (GSE30219, GSE10072; Figure 2B,2C), with the results being consistent with those of TCGA database. Furthermore, we examined the expression of GNGT1 in frozen (Figure 2D) and formalin-fixed human LUAD tissues (Figure 2E) and found that the expression of GNGT1 was significantly increased in LUAD relative to adjacent normal tissues.
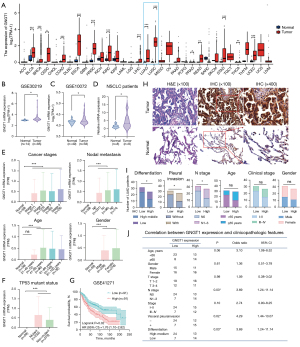
We further analyzed the relationship between GNGT1 mRNA expression and other clinicopathological features via the UALCAN online database. We found that high GNGT1 expression was associated with a greater degree lymph node metastasis (20) (Figure 2F) and not affected by TP53 mutation status (Figure 2G). Univariate and multivariate Cox regression analysis of TCGA data showed that GNGT1 was an independent prognostic factor for LUAD (Figure S3A,S3B and Tables S4,S5), and the expression of GNGT1 is not associated with standard biomarkers, such as EGFR, ALK, ROS, BRAF and HER2 (Figure S3C). Poor prognosis was associated with higher GNGT1 expression in TCGA, which was further verified in the GSE41271 dataset (Figure 2H). To clarify the importance of GNGT1, we analyzed clinicopathological features, including pathological stage, pleural invasion, T stage, lymph node metastasis, age, gender, and pathological differentiation of the enrolled patients with LUAD according to the IHC expression of GNGT1. We found that higher GNGT1 expression was significantly associated with lymph node metastasis, pleural invasion, and lower differentiation. No significance was observed between T stage (P>0.99) and pathological stage (P=0.10), potentially due to the limited sample size. GNGT1 expression was not associated with age or gender (Figure 2I,2J and Figure S3D).
Upregulation of GNGT1 expression in LUAD was associated with the regulation of network of gene alteration and methylation
Genetic alterations and methylation modifications can affect the final expression of genes. We used the online database OncoVar to study the genetic alterations in LUAD and found a large number of genes are altered in various forms (Figure S4A-S4H), and the gene alteration of the seven hub genes in LUAD were analyzed via cBioportal (https://www.cbioportal.org/). The alteration rate of GNGT1 in LUAD was 2.2% (Figure S4I). The GNGT1 gene demonstrated multiple change patterns in various cancers, including LUAD and lung squamous cell carcinoma (LUSC), such as mutation, structural variants, amplification, and deep deletion (Figure S5A). GNGT1 mRNA expression was positively correlated with the mutation count (Figure S5B) and could be changed by putative copy-number alterations (Figure S5C).
To further evaluate the relationship between methylation and gene transcriptional profiles, we implemented MEXPRESS (20), which detected 21 sites for GNGT1 that were hypermethylated in LUAD (Figure S6A). According to the cBioportal database, GNGT1 mRNA expression was also significantly negatively correlated with the methylation of ATP2A1 (cg000002920) (Figure S6B). and MEOX2 (cg00003994) (Figure S6C). The promoter methylation levels of GNGT1 in LUAD were significantly downregulated compared to those of paired normal tissue (Figure S6D). Therefore, we confirmed the upregulated expression of GNGT1 in LUAD was related to the regulation networks of the gene alteration and methylation.
Functional enrichment analysis of GNGT1 in LUAD
To elucidate the underlying molecular mechanisms of GNGT1 in LUAD, we sorted the tumor samples into the GH and GL groups using an expression value of 2 as cutoff criterion to identify DEGs that are associated with GNGT1 upregulation in LUAD. Gene expression levels of 309 patients with LUAD in the GL group and 206 patients in the GH group were analyzed. A total of 1,136 DEGs were identified, in which 928 genes were upregulated and 208 downregulated (cutoffs of P<0.05 and log FC >1) (Figure 3A). The correlation analysis showed that SPERT, SLC5A12, DVWA, POU6F2, and SLC06A2 were the top five genes related to GNGT1 (Figure 3B). The expression of these five genes were analyzed and were found to be significantly altered in LUAD (Figure 3C) and LUSC (Figure 3D) according to GNGT1 expression level.
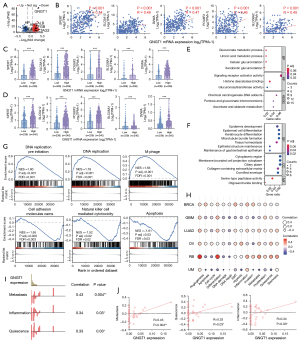
We then performed GO and KEGG enrichment analyses of these 1,136 differential genes using R software version 4.2.2. We discovered that the upregulated genes in the GH group were associated with pathways related to chemical carcinogenesis and metabolism (Figure 3E), while downregulated genes were associated with tissue homeostasis, epithelial structure maintenance, and extracellular matrix structural constituent (Figure 3F). The GSEA showed that the GH group genes were mainly enriched in DNA replication preinitiation, DNA replication, and M phase pathway, while genes related to cell adhesion, apoptosis, and NK cell-mediated cytotoxicity were downregulated (Figure 3G and Figure S7).
The single-cell function state analysis indicated that GNGT1 expression was correlated with many function states in a variety of cancers, such as LUAD, breast cancer (BRCA), glioblastoma (GBM), ovarian cancer (OV), retinoblastoma (RB), and uveal melanoma (UM) (Figure 3H). The expression of GNGT1 was significantly correlated with metastasis, quiescence, and inflammation in LUAD (Figure 3I,3J).
GNGT1 could promote lung cancer progression by enhancing the stemness of cancer cells
To gain a better understanding of the potential mechanisms of GNGT1 in LUAD, we established a lung-specific GNGT1 overexpression mouse model. Mice under stop code (LSL-GNGT1) were crossbred with Scgb1a1-Cre mice raised in a standard SPF environment to generate LSL-GNGT1; Scgb1a1-Cre mice (GNGT1fl/+ mice; Figure 4A,4B). The overexpression of GNGT1 in the lung was verified by RT-qPCR (Figure 4C) and IHC (Figure 4D). Histopathological assessment of lung tissue confirmed an increase in alveolar space fluid leakage and bronchial epithelial damage, thereby highlighting the significant epithelial structure disruption in GNGT1fl/+ mice. In hematoxylin and eosin (H&E) staining, GNGT1 expression of the other important organs showed no significant differences between GNGT1fl/+ and wild-type mice (C57BL/6) (Figure 4D). In addition, the expressions of Ki-67 (Figure 4E) and PCNA (Figure 4F), which are classical markers of cell proliferation, were upregulated, while the lower expression of caspase-3 (Figure 4G), an apoptosis marker, was also observed in the lung tissue of GNGT1fl/+ mice relative to wild-type controls. This result, along with GSEA, suggested that GNGT1 exerts a key effect in tumor proliferation and apoptosis. We further considered how GNGT1 induces a state of high proliferation of epithelium cells. TCGA database was used to detect the correlation between LUAD stemness genes and GNGT1 expression, which indicated that SOX2, CD44, and KLF4 were significantly correlated with GNGT1 (Figure 5A). The results of RT-qPCR (Figure 5B) and IF (Figure 5C) verification also indicated that the expression of tumor stem genes was significantly upregulated in the lung tissues of GNGT1fl/+ mice, suggesting that GNGT1 could enhance tumor progression by increasing the stemness of tumor cells.
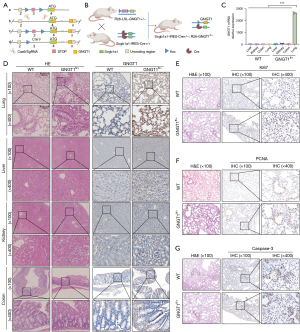
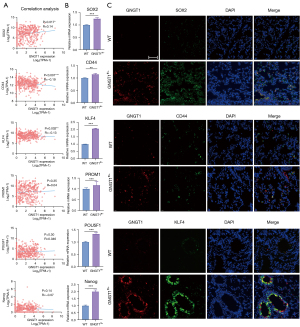
GNGT1 interacted with driver genes and regulated the EMT function of tumor cells
The epithelial structure polar imbalance in histological staining was consistent with the downregulation of epithelial structure maintenance and homeostasis in the GH group. GSEA also showed that DNA replication preinitiation was upregulated in the GH group. We thus considered whether the high expression of GNGT1 induces the changes of tumor driver genes, thereby causing morphological and function changes in the transformation of normal epithelium cells to atypical cells or tumor cells. Correlation analysis and mRNA detection of common LUAD-related driver genes confirmed this speculation (Figure 6A,6B). The mRNA expression of B2M, KRAS, STK11, PTEN, KEAP1, and NEF2L2 was significantly upregulated in the lung tissues of GNGT1fl+ mice (Figure 6C). Dysfunction of tumor-driver gene function and increased tumor stemness can both induce the EMT of tumor cells, promote tumor invasion and migration, and further promote tumor occurrence. We further found that E-cadherin and CDH2 mRNA were downregulated and upregulated in GNGT1fl+ mice, respectively (Figure 6C), which was consistent with the functional analysis and suggested that EMT is altered in cells with high GNGT1 expression.
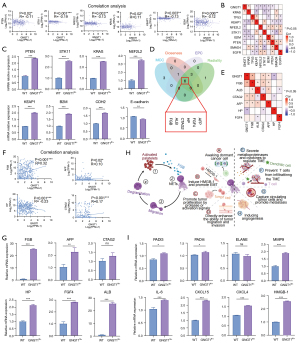
GNGT1 promoted FGB-NET axis activation to remodel the TME
The protein-protein interaction (PPI) network of DEGs was constructed using the Search Tool for the Retrieval of Interacting Genes/Proteins (STRING) database and visualized in Cytoscape. The cytoCNA app was used to calculate the betweenness centrality of the DEGs, and then the top 100 genes in terms of betweenness centrality score were selected to construct the PPI network. The cytoHubba app was used to obtain the hub genes. The overlapping genes selected by cytoHubba with four different algorithms [maximal clique centrality (MCC), closeness, edge percolated component (EPC), and radiality] were FGB, albumin (ALB), cancer/testis antigen 2 (CTAG2), alpha-fetoprotein (AFP), haptoglobin (HP), and fibroblast growth factor 4 (FGF4), which were considered the hub genes (Figure 6D). The correlation between the hub genes and GNGT1 was analyzed, and FGB, CTAG2, AFP, and HP, demonstrated a significant correlation with GNGT1 (Figure 6E,6F). Moreover, in GNGT1fl/+ and wild-type mice, we detected the mRNA expression levels of the hub genes (Figure 6G) and the most-changed DEGs related to the structural integrity of epithelial cells (Figure S8A), extracellular matrix organization (Figure S8B), cell-cell communication (Figure S8C), transcriptional regulation and differentiation of pluripotent stem cells (Figure S8D), regulation of autophagy (Figure S8E), transcriptional regulation and posttranscriptional modification (Figure S9A), immune regulation (Figure S9B) and among other DEGs (Figure S9C). Ultimately, FGB showed the most significant change among all the detected genes.
By analyzing the KEGG pathway of FGB, we found that FGB acts on NETs (pathway: map04613) and that NETs may promote tumor progression through multiple pathways (Figure 6H). RT-qPCR verified the expression of neutrophil-related inflammatory factors platelet factor 4 (CXCL4), chemokine (C-X-C motif) ligand 15 (CXCL15), interleukin 6 (IL-6), and peptidylarginine deiminases (PADs) family members, along with matrix metallopeptidase 9 (MMP9) and high mobility group protein B1 (HGMB1), which are all important molecules in the NETs process, and their expression was all significantly increased in GNGT1fl+ mice. However, neutrophil elastase (ELANE) expression presented no alteration (Figure 6I). These results suggest that GNGT1 may upregulate the secretion of FGB to activate the release of NETs and further affect tumor immunity and the stromal microenvironment to induce the metastasis of tumor cells.
Immune infiltration status and tumor mutation burden (TMB) was correlated with GNGT1 expression in NSCLC
To further evaluate the potential effects of GNGT1 on the tumor-immune environment in NSCLC, we quantified immune infiltration signatures in LUAD and LUSC from TCGA in the TIMER database (Figure 7A and Figure S10A-S10D) and with R package “GSVA” and “ggplot2”. The SCNA module in TIMER was used to compare the tumor infiltration levels among tumors with different somatic copy number alterations for GNGT1. It was found that the infiltration of neutrophils was significantly associated with the arm-level deletion of GNGT1 and that the enrichment score of a variety of immune cells was changed according to the expression level of GNGT1 (Figure 7B). For confirmation, we analyzed 24 types of immune cell infiltration in LUAD using the R package ‘‘GSVA”. Multiple immune cells exhibited a significant correlation with GNGT1 expression, including Th2 cells [P<0.001; correlation (cor) =0.161], γδ T cells, (P<0.001; cor =0.16), T cells (P=0.003; cor =−0.13); aDCs (P<0.001; cor =−0.17), cytotoxic cells (P<0.001; cor =−0.16), DCs (P<0.001; cor =−0.30), eosinophils (P<0.001; cor =−0.26), iDCs (P<0.001; cor =−0.30), macrophages (P<0.001; cor =−0.22), mast cells (P<0.001; cor =−0.26), neutrophils (P<0.001; cor =−0.24), NK cells (P<0.001; cor =−0.15), pDCs (P<0.001; cor =−0.27), and Th17 cells (P<0.001, cor =−0.15) (Figure 7C,7D and Figure S10E).
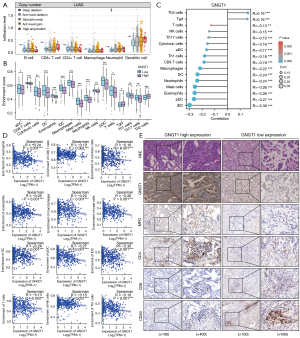
Further investigation into the potential effects of GNGT1 in LUAD on TMB and microsatellite instability (MSI) revealed a positive correlation between GNGT1 expression and TMB (P=0.01, cor =−0.12; Figure S11A), whereas no correlation was observed with MSI (P=0.15, cor =0.05; Figure S11B).
GNGT1fl/+ mouse experiments and pancancer analysis of immune infiltration both confirmed the importance of GNGT1 in remodeling TME. Pathway analysis also showed that GNGT1 was related to extracellular matrix structural constituent and the interaction of immune cells with the TME. We next determined whether the TME features observed in the preclinical models also occurs in patients with a high expression of GNGT1. H&E stains tissues sections of patients with LUAD were reassessed, which indicated a higher degree of immune cell infiltration in the tumor stroma of patients with high GNGT1 expression compared to those with low expression (Figure 7E, red arrow). IHC staining showed that the abundance of CD4+ T lymphocytes and neutrophils was increased in patients with high GNGT1 expression, while that of CD20+ B lymphocytes and CD8+ T lymphocytes was decreased, which further verified the regulatory effects of GNGT1 on TME remodeling.
Discussion
Due to its high degree of metastasis and aggressiveness, NSCLC remains a major challenge for cancer therapy and has a high mortality rate worldwide (21). Metastasis is one of the malignant markers and characteristics of lung cancer and the leading cause of treatment failure and death in patients with lung cancer (22). About 30% of these patients already have distant metastases at diagnosis, and 50–60% develop distant metastases during the course of treatment (23). Although there are numerous traditional clinical management options for NSCLC and the survival time of patients with advanced metastasis have been improved to some extent, metastasis still results in 80–90% of those with lung cancer (24). Thus, it is imperative to explore the pathological mechanism of lung cancer metastasis and identify effective intervention targets to prevent the malignant process of lung cancer and improve the survival rate of patients.
The GPCR superfamily, including G protein subunit γ transduction protein 1 (GNGT1), participates in the regulation of a series of cell biological behaviors by activating various secondary signaling pathways such as PI3K, MAPK, and ion channels after binding with the corresponding ligands (25-30). Aberrant expression and activation of GPCR and its downstream signaling pathways have been shown to be important markers of the tumor malignancy process. GPR143 is elevated in multiple cancers and promotes cancer metastasis (16), and GPR30 overexpression is associated with worse prognosis in patients with endometrial or ovarian cancer and increases the risk of metastasis in those with breast cancer (27,31). Chemokine (C-X-C motif) receptor 4 (CXCR4), the most well-known GPCR, has proliferative, survival, and metastatic effects (32-34) and is abnormally expressed in many cancers. Lymph nodes, the lungs, bone marrow, and the liver are the most common sites of tumor metastasis, which usually express the ligand of CXCR4, namely chemokine (C-X-C motif) ligand 12 (CXCL12) (35,36). The cascade of signaling pathways such as PI3K/AKT/mTOR is also important for the proliferation, survival, migration, and metabolism of tumor cells (37). However, previous studies on this subject have focused only on Gα and β (16,26-28), and the research into the expression and function of GNGT1 in tissues and organs, including in the field of cancers, is still in its infancy. GNGT1 was found to be localized to human chromosome 7 and may be associated with various forms of cancer to regulate cell proliferation, migration, adhesion, and apoptosis. GNGT1 has been reported to be highly expressed in tumor tissues of patients with nasopharyngeal carcinoma, and the rate of distant metastasis is significantly increased in patients with higher expression (38). Moreover, GNGT1 has been demonstrated to be highly expressed in tumor tissues of patients with adrenocortical carcinoma and to be positively correlated with tumor malignancy (39). However, its influence on the occurrence and development of NSCLC and the related molecular mechanisms are still unknown.
Our study found that GNGT1 overexpression is an important mechanism in the malignant process of LUAD, especially for metastasis. To identify GNGT1-related targets, we identified 928 mRNAs that were upregulated in the GH group. GSEA, GO and KEGG analyses revealed that they were enriched in functions such as cell cycle, DNA replication, and M phase but were downregulated in cell adhesion and tissue homeostasis. The single-cell analysis showed that the status of functions of cancer cells in LUAD, such as metastasis, was significantly positively correlated with GNGT1 expression.
To validate our analysis, we designed a lung-specific GNGT1 overexpression mouse model (LSL-GNGT1; Scgb1a1-Cre mouse, GNGT1fl/+ mouse). The mRNA expression of key gene-related pathway were detected. The EMT-related molecule E-cadherin and CDH2 were significantly altered, while stemness-related molecules SOX2, KLF4, CD44, OCT4, and Nanog were upregulated, which confirmed our findings related to the functional properties of GNGT 1 in LUAD. The driver genes of LUAD, including KRAS, B2M, TP53, KEAP1, NFE2L2, SMAD4, and EGFR were also upregulated. Aberrant GNGT1 expression cooperates with the imbalance of driver genes to promote cancer progression in the lung. All of these changes enhance the proliferation and invasion ability of lung cancer cells; however, tumor progression and metastasis is also under the regulation of the microenvironment.
To clarify the effect of GNGT1 on remodeling the TME, we identified 1,326 mRNAs that were differentially expressed in the GH group compared with the GL group and performed intersection analysis, which yielded 6 mRNAs (FGB, ALB, HP, AFP, CTAG2, and FGF4). We analyzed the correlation of the hub genes and found that FGB had the most significant FC with GNGT1.
The FGB gene encodes the beta component of FGB, which is a blood-borne glycoprotein comprised of three pairs of nonidentical polypeptide chains. Mutations in this gene lead to several disorders (40). In addition, various cleavage products of FGB and fibrin regulate cell adhesion and spreading. A recent study revealed that FGB facilities tumor growth and metastasis through the integrin/AKT signaling pathway in lung cancer (41). To confirm the functional roles of FGB in LUAD, we repeated KEGG analysis, which verified that FGB is enriched in the NET pathway. An increasing amount of evidence is indicating that immune cell infiltration in tumor tissues affects cancer progression and patient prognosis (42-45). NETs is composed of chromatin coated with histones, granule proteases, and cytosolic proteins, and is an important mechanism and function of neutrophils (46). NETs can capture circulating tumor cells and increase the adhesion of tumor cells with blood vessels to promote the migration of tumors (47-51). In addition, the formation of NETs further prevents cytotoxic T cells from infiltrating the TME and inducing the immune escape of tumor cells (52). In the TME of LUAD develops many types of immune cells, such as neutrophils and macrophages. Existing studies show that immune cells also present different types under different conditions and are dynamically changing processes (21). By regulating FGB production, GNGT1 promotes the recruitment and functional status of neutrophils, and then regulates the dynamic changes of TME. Tumor-associated neutrophils (TANs) are important immune cells, and cancer-promoting effects of TANs include the acceleration of angiogenesis, cancer cell migration, and EMT (53-58). Activated TANs can release NETs, and proteolytic enzymes and MMPs which are contained in NETs can dissolve the extracellular matrix and enhance the aggressiveness of tumor cells (59,60). We examined the expression of inflammation markers (CXCL4, CXCL15, IL-6), peptidyl arginine deiminase (PADI4 and PADI3), matrix metalloproteinase (MMP9), and alpha-1-B glycoprotein (HGMB-1), which are all key NET-related factors, in the lungs of GNGT1fl/+ mice and confirmed the significant increase in the expression of these genes and further validated the activation of NETs. IHC staining of LUAD patients with high GNGT1 expression showed a greater infiltration of neutrophils and CD4+ T cells but a lower degree of cytotoxic CD8+ T lymphocytes and CD20+ B cells. Taken together, these finding suggest that GNGT1 may have a pronounced inhibitory effect on the immune function in the TME in LUAD.
In this study, a greater emphasis was placed on biogenic analysis, while the number of clinical samples was relatively limited. Further observation is required to determine whether the GNGT1 conditioned overexpression in lung tissue of mice will progress to invasive tumors.
Early marker diagnosis is always a difficult problem in the diagnosis of lung cancer. This study is the first to demonstrate that the oncogenic factor GNGT1 is integral to the early diagnosis of LUAD. It is significantly associated with the onset, progression, and prognosis of lung cancer, indicating that GNGT1 overexpression may constitute an early driving event in the pathogenesis of LUAD. The G protein family plays an important role in tumors, but the mechanism of action of GNGT1 in lung cancer has been unclear. This study investigated the molecular mechanisms by which GNGT1 regulates the occurrence and progression of lung tumors, thereby addressing a significant research gap in this domain. This study holds significant potential for guiding the development of novel therapeutic strategies targeting LUAD, particularly for specific patient populations.
However, further research is required to elucidate the direct interactions between GNGT1 and associated molecules, as well as its impact on specific infiltration patterns of TANs and the underlying mechanisms of action. Specific mutations in genes have been identified in the plasma of patients with several types of cancer, which highlights circulating tumor DNA (ctDNA) as a possible cancer biomarker (61). Further studies are still necessary to achieve the relationship of GNGT1 in tissue and ctDNA for diagnostic and treatment monitoring for NSCLC and other types of cancers.
A series of bioinformatic and machine learning approaches, such as enriched pathways, genomic and copy number alterations were combined to establish effective signatures to predict immune checkpoint blockade (ICB) therapy response in LUAD patients. In the future, the development of more sophisticated analytical methodologies is anticipated to enhance the precision of target identification for predicting the efficacy of targeting and immunotherapeutic strategies in lung cancer.
Conclusions
We confirmed that a high expression of GNGT1 enhances the stemness and EMT of tumor cells. We found that GNGT1 can impact tumor TME remodeling by increasing FGB secretion to regulate NETs. These events eventually result in poor prognosis and a high degree of lymph node metastasis in LUAD. These findings were verified across three different dimensions, including through online databases, a preclinical transgenic mice model, and the tissue of patients with LUAD. These results provide additional evidence for the potential integral roles of GNGT1 in the development, progression, and metastasis of NSCLC, which may provide new strategies for the treatment of NSCLC.
Acknowledgments
We would like to thank Professor Hou Jin of Navy Military Medical University for advice and useful comments that helped finalize this article.
Footnote
Reporting Checklist: The authors have completed the ARRIVE and MDAR reporting checklists. Available at https://tlcr.amegroups.com/article/view/10.21037/tlcr-2024-1200/rc
Data Sharing Statement: Available at https://tlcr.amegroups.com/article/view/10.21037/tlcr-2024-1200/dss
Peer Review File: Available at https://tlcr.amegroups.com/article/view/10.21037/tlcr-2024-1200/prf
Funding: This study was supported in part by grants from
Conflicts of Interest: All authors have completed the ICMJE uniform disclosure form (available at https://tlcr.amegroups.com/article/view/10.21037/tlcr-2024-1200/coif). R.A.d.M. serves as an unpaid editorial board member of Translational Lung Cancer Research from January 2024 to December 2025. The other authors have no conflicts of interest to declare.
Ethical Statement: The authors are accountable for all aspects of the work in ensuring that questions related to the accuracy or integrity of any part of the work are appropriately investigated and resolved. The study was conducted in accordance with the Declaration of Helsinki (as revised in 2013). Animal experiments were performed under a project license (No. CHEC2021-061) granted by Navy Military Medical University Affiliated Changhai Hospital Ethics Committee, in compliance with institutional guidelines for the care and use of animals.
Open Access Statement: This is an Open Access article distributed in accordance with the Creative Commons Attribution-NonCommercial-NoDerivs 4.0 International License (CC BY-NC-ND 4.0), which permits the non-commercial replication and distribution of the article with the strict proviso that no changes or edits are made and the original work is properly cited (including links to both the formal publication through the relevant DOI and the license). See: https://creativecommons.org/licenses/by-nc-nd/4.0/.
References
- Bray F, Laversanne M, Sung H, et al. Global cancer statistics 2022: GLOBOCAN estimates of incidence and mortality worldwide for 36 cancers in 185 countries. CA Cancer J Clin 2024;74:229-63. [Crossref] [PubMed]
- Mazzone PJ, Lam L. Evaluating the Patient With a Pulmonary Nodule: A Review. JAMA 2022;327:264-73. [Crossref] [PubMed]
- Hu J, Sánchez-Rivera FJ, Wang Z, et al. STING inhibits the reactivation of dormant metastasis in lung adenocarcinoma. Nature 2023;616:806-13. [Crossref] [PubMed]
- Spicer JD, Cascone T, Wynes MW, et al. Neoadjuvant and Adjuvant Treatments for Early Stage Resectable NSCLC: Consensus Recommendations From the International Association for the Study of Lung Cancer. J Thorac Oncol 2024;19:1373-414. [Crossref] [PubMed]
- Passaro A, Brahmer J, Antonia S, et al. Managing Resistance to Immune Checkpoint Inhibitors in Lung Cancer: Treatment and Novel Strategies. J Clin Oncol 2022;40:598-610. [Crossref] [PubMed]
- Wang J, Zheng Q, Chen J. Immune checkpoint inhibitor-induced autoimmune encephalitis in metastatic squamous cell lung cancer. Immunotherapy 2023;15:409-16. [Crossref] [PubMed]
- Hu Z, Sui Q, Jin X, et al. IL6-STAT3-C/EBPβ-IL6 positive feedback loop in tumor-associated macrophages promotes the EMT and metastasis of lung adenocarcinoma. J Exp Clin Cancer Res 2024;43:63. [Crossref] [PubMed]
- Chen J, Wu Z, Deng W, et al. USP51 promotes non-small cell lung carcinoma cell stemness by deubiquitinating TWIST1. J Transl Med 2023;21:453. [Crossref] [PubMed]
- Xiao GY, Tan X, Rodriguez BL, et al. EMT activates exocytotic Rabs to coordinate invasion and immunosuppression in lung cancer. Proc Natl Acad Sci U S A 2023;120:e2220276120. [Crossref] [PubMed]
- Chen JS, Guo X, Sun JY, et al. Fangchinoline derivatives inhibits PI3K signaling in vitro and in vivo in non-small cell lung cancer. Bioorg Chem 2023;138:106623. [Crossref] [PubMed]
- Zhang F, Ma Y, Li D, et al. Cancer associated fibroblasts and metabolic reprogramming: unraveling the intricate crosstalk in tumor evolution. J Hematol Oncol 2024;17:80. [Crossref] [PubMed]
- You S, Li S, Zeng L, et al. Lymphatic-localized Treg-mregDC crosstalk limits antigen trafficking and restrains anti-tumor immunity. Cancer Cell 2024;42:1415-1433.e12. [Crossref] [PubMed]
- Zhong H, Zhou S, Yin S, et al. Tumor microenvironment as niche constructed by cancer stem cells: Breaking the ecosystem to combat cancer. J Adv Res 2024; Epub ahead of print. [Crossref]
- Elhanani O, Ben-Uri R, Keren L. Spatial profiling technologies illuminate the tumor microenvironment. Cancer Cell 2023;41:404-20. [Crossref] [PubMed]
- Haynes NM, Chadwick TB, Parker BS. The complexity of immune evasion mechanisms throughout the metastatic cascade. Nat Immunol 2024;25:1793-808. [Crossref] [PubMed]
- Chaudhary PK, Kim S. An Insight into GPCR and G-Proteins as Cancer Drivers. Cells 2021;10:3288. [Crossref] [PubMed]
- Lee YJ, Shin KJ, Jang HJ, et al. GPR143 controls ESCRT-dependent exosome biogenesis and promotes cancer metastasis. Dev Cell 2023;58:320-334.e8. [Crossref] [PubMed]
- Arakaki AKS, Pan WA, Wedegaertner H, et al. α-Arrestin ARRDC3 tumor suppressor function is linked to GPCR-induced TAZ activation and breast cancer metastasis. J Cell Sci 2021;134:jcs254888. [Crossref] [PubMed]
- Bagnato A, Rosanò L. New Routes in GPCR/β-Arrestin-Driven Signaling in Cancer Progression and Metastasis. Front Pharmacol 2019;10:114. [Crossref] [PubMed]
- Koch A, De Meyer T, Jeschke J, et al. MEXPRESS: visualizing expression, DNA methylation and clinical TCGA data. BMC Genomics 2015;16:636. [Crossref] [PubMed]
- Martínez-Ruiz C, Black JRM, Puttick C, et al. Genomic-transcriptomic evolution in lung cancer and metastasis. Nature 2023;616:543-52. [Crossref] [PubMed]
- Li Z, Huang J, Shen S, et al. SIRT6 drives epithelial-to-mesenchymal transition and metastasis in non-small cell lung cancer via snail-dependent transrepression of KLF4. J Exp Clin Cancer Res 2018;37:323. [Crossref] [PubMed]
- Gourd E. Osimertinib for leptomeningeal metastases in NSCLC. Lancet Oncol 2020;21:e17. [Crossref] [PubMed]
- Greco L. Patients With Brain Metastases Deserve Better-A Hard-Won Perspective. JAMA Oncol 2023;9:1197-8. [Crossref] [PubMed]
- Laplante M, Sabatini DM. mTOR signaling in growth control and disease. Cell 2012;149:274-93. [Crossref] [PubMed]
- Song Q, Ji Q, Li Q. The role and mechanism of β arrestins in cancer invasion and metastasis Int J Mol Med 2018;41:631-9. (Review). [Crossref] [PubMed]
- Prossnitz ER, Barton M. The G protein-coupled oestrogen receptor GPER in health and disease: an update. Nat Rev Endocrinol 2023;19:407-24. [Crossref] [PubMed]
- Dashti MR, Gorbanzadeh F, Jafari-Gharabaghlou D, et al. G Protein-Coupled Receptor 75 (GPR75) As a Novel Molecule for Targeted Therapy of Cancer and Metabolic Syndrome. Asian Pac J Cancer Prev 2023;24:1817-25. [Crossref] [PubMed]
- Liu H, Zhu Y, Niu H, et al. Activation of PI3K/Akt pathway by G protein-coupled receptor 37 promotes resistance to cisplatin-induced apoptosis in non-small cell lung cancer. Cancer Med 2023;12:19777-93. [Crossref] [PubMed]
- Khalil BD, Hsueh C, Cao Y, et al. GPCR Signaling Mediates Tumor Metastasis via PI3Kβ. Cancer Res 2016;76:2944-53. [Crossref] [PubMed]
- Wolfson B, Padget MR, Schlom J, et al. Exploiting off-target effects of estrogen deprivation to sensitize estrogen receptor negative breast cancer to immune killing. J Immunother Cancer 2021;9:e002258. [Crossref] [PubMed]
- Nengroo MA, Khan MA, Verma A, et al. Demystifying the CXCR4 conundrum in cancer biology: Beyond the surface signaling paradigm. Biochim Biophys Acta Rev Cancer 2022;1877:188790. [Crossref] [PubMed]
- Korbecki J, Bosiacki M, Kupnicka P, et al. CXCR4 as a therapeutic target in acute myeloid leukemia. Leukemia 2024;38:2303-17. [Crossref] [PubMed]
- Dong L, Hu S, Li X, et al. SPP1(+) TAM Regulates the Metastatic Colonization of CXCR4(+) Metastasis-Associated Tumor Cells by Remodeling the Lymph Node Microenvironment. Adv Sci (Weinh) 2024;11:e2400524. [Crossref] [PubMed]
- Müller A, Homey B, Soto H, et al. Involvement of chemokine receptors in breast cancer metastasis. Nature 2001;410:50-6. [Crossref] [PubMed]
- Meguro S, Johmura Y, Wang TW, et al. Preexisting senescent fibroblasts in the aged bladder create a tumor-permissive niche through CXCL12 secretion. Nat Aging 2024;4:1582-97. [Crossref] [PubMed]
- Ben-Sahra I, Manning BD. mTORC1 signaling and the metabolic control of cell growth. Curr Opin Cell Biol 2017;45:72-82. [Crossref] [PubMed]
- Wang Y, Li M, Guo Y, et al. Key genes affecting the progression of nasopharyngeal carcinoma identified by RNA-sequencing and bioinformatic analysis. Aging (Albany NY) 2021;13:22176-87. [Crossref] [PubMed]
- Ma C, Xiong J, Su H, et al. The underlying molecular mechanism and drugs for treatment in adrenal cortical carcinoma. Int J Med Sci 2021;18:3026-38. [Crossref] [PubMed]
- Chen Y, Zhu Y, Sheng Y, et al. SIRT1 downregulated FGB expression to inhibit RCC tumorigenesis by destabilizing STAT3. Exp Cell Res 2019;382:111466. [Crossref] [PubMed]
- Wang M, Zhang G, Zhang Y, et al. Fibrinogen Alpha Chain Knockout Promotes Tumor Growth and Metastasis through Integrin-AKT Signaling Pathway in Lung Cancer. Mol Cancer Res 2020;18:943-54. [Crossref] [PubMed]
- Hisada Y, Mackman N. Cancer-associated pathways and biomarkers of venous thrombosis. Blood 2017;130:1499-506. [Crossref] [PubMed]
- Cristinziano L, Modestino L, Antonelli A, et al. Neutrophil extracellular traps in cancer. Semin Cancer Biol 2022;79:91-104. [Crossref] [PubMed]
- Khan U, Chowdhury S, Billah MM, et al. Neutrophil Extracellular Traps in Colorectal Cancer Progression and Metastasis. Int J Mol Sci 2021;22:7260. [Crossref] [PubMed]
- De Meo ML, Spicer JD. The role of neutrophil extracellular traps in cancer progression and metastasis. Semin Immunol 2021;57:101595. [Crossref] [PubMed]
- Papayannopoulos V. Neutrophil extracellular traps in immunity and disease. Nat Rev Immunol 2018;18:134-47. [Crossref] [PubMed]
- Shahzad MH, Feng L, Su X, et al. Neutrophil Extracellular Traps in Cancer Therapy Resistance. Cancers (Basel) 2022;14:1359. [Crossref] [PubMed]
- Huang H, Zhang H, Onuma AE, et al. Neutrophil Elastase and Neutrophil Extracellular Traps in the Tumor Microenvironment. Adv Exp Med Biol 2020;1263:13-23. [Crossref] [PubMed]
- Kaltenmeier C, Simmons RL, Tohme S, et al. Neutrophil Extracellular Traps (NETs) in Cancer Metastasis. Cancers (Basel) 2021;13:6131. [Crossref] [PubMed]
- Stehr AM, Wang G, Demmler R, et al. Neutrophil extracellular traps drive epithelial-mesenchymal transition of human colon cancer. J Pathol 2022;256:455-67. [Crossref] [PubMed]
- Wang H, Kim SJ, Lei Y, et al. Neutrophil extracellular traps in homeostasis and disease. Signal Transduct Target Ther 2024;9:235. [Crossref] [PubMed]
- Kaltenmeier C, Yazdani HO, Morder K, et al. Neutrophil Extracellular Traps Promote T Cell Exhaustion in the Tumor Microenvironment. Front Immunol 2021;12:785222. [Crossref] [PubMed]
- Loffredo S, Borriello F, Iannone R, et al. Group V Secreted Phospholipase A(2) Induces the Release of Proangiogenic and Antiangiogenic Factors by Human Neutrophils. Front Immunol 2017;8:443. [Crossref] [PubMed]
- Kim J, Bae JS. Tumor-Associated Macrophages and Neutrophils in Tumor Microenvironment. Mediators Inflamm 2016;2016:6058147. [Crossref] [PubMed]
- Mizuno R, Kawada K, Itatani Y, et al. The Role of Tumor-Associated Neutrophils in Colorectal Cancer. Int J Mol Sci 2019;20:529. [Crossref] [PubMed]
- Li S, Cong X, Gao H, et al. Tumor-associated neutrophils induce EMT by IL-17a to promote migration and invasion in gastric cancer cells. J Exp Clin Cancer Res 2019;38:6. [Crossref] [PubMed]
- Zhou X, Wu C, Wang X, et al. Tumor cell-released autophagosomes (TRAPs) induce PD-L1-decorated NETs that suppress T-cell function to promote breast cancer pulmonary metastasis. J Immunother Cancer 2024;12:e009082. [Crossref] [PubMed]
- Yu Y, Zhang C, Dong B, et al. Neutrophil extracellular traps promote immune escape in hepatocellular carcinoma by up-regulating CD73 through Notch2. Cancer Lett 2024;598:217098. [Crossref] [PubMed]
- Demkow U. Neutrophil Extracellular Traps (NETs) in Cancer Invasion, Evasion and Metastasis. Cancers (Basel) 2021;13:4495. [Crossref] [PubMed]
- Yang L, Liu Q, Zhang X, et al. DNA of neutrophil extracellular traps promotes cancer metastasis via CCDC25. Nature 2020;583:133-8. [Crossref] [PubMed]
- Cohen SA, Liu MC, Aleshin A. Practical recommendations for using ctDNA in clinical decision making. Nature 2023;619:259-68. [Crossref] [PubMed]
(English Language Editor: J. Gray)